DOI:
10.1039/C7RA06707K
(Paper)
RSC Adv., 2017,
7, 37839-37843
Copper-catalyzed one-pot reactions of acetyl chloride, o-halobenzoic acids and Wittig reagents toward 3-methyl isocoumarin synthesis†
Received
16th June 2017
, Accepted 24th July 2017
First published on 1st August 2017
Abstract
The one-pot reactions of acetyl chloride, o-halobenzoic acids and Wittig reagents providing 3-methyl isocoumarins have been furnished via tandem Wittig reaction, oxa-Michael addition and C–C cross coupling. Three new chemical bonds including one C–O, one C
C and one C–C bond are generated via the catalysis of a simple copper salt for the heterocycle construction.
Introduction
The copper-catalyzed Ullmann-type C–C cross coupling reaction between aryl/vinyl halides and active carbon coupling partners is an important tool in the generation of new carbon–carbon bonds.1 While significant contribution of such transformation has been furnished in the synthesis of divergent organic products via both direct coupling process or tandem reactions initiated by this coupling transformation, one of the major restrictions in the Ullmann C–C cross coupling reaction is that the scope of the carbon coupling partners is rather narrow. In most of the related known literature, active methylenes such as 1,3-dicarbonyl or analogous compounds are predominantly used as the reaction partners of aryl/vinyl halides,2 and alternative reactants in such reactions are rarely known.3 Accordingly, the limited substrate scope has also hampered the application of this C–C cross coupling in the synthesis of more structurally diversified organic products. Therefore, in order to promote the application of the Ullmann C–C coupling as broad as those equivalent C-heteroatom coupling versions, discovering practical reaction partners constitutes the main present challenge. Our group has previously reported the copper-catalyzed C–C coupling initiated tandem reactions toward the synthesis of benzofurans4 and indoles5 wherein the in situ generated allenes are utilized as the carbon coupling partners of the Ar–halogen bond to enable the product construction. In despite of these successful examples, it should be noted that examples on the C–C coupling reactions employing allenes as coupling partners are still rather scarce.
Isocoumarins are a class of typical heterocycles with broad spectrum biological activity, and this backbone also constitutes the central fragment of many natural products.6 Based on known literature, isocoumarins can be synthesized with different strategies,7 such as the tandem annulations initiated by Ar–H bond addition to alkynes,8 diazo functionalized ketones9 or cyclic alkenyl carbonates,10 the intramolecular cross coupling of Ar–halogen and vinyl C–H bond,11 the addition of Ar–halogen bond to alkynes,12 ring expansion reactions,13 the oxidative coupling of benzoic acids and vinylarene,14 among others.15 Regardless the enriched availability on synthetic methods, it is notable that most of the reported methods on isocoumarin synthesis require the presence of noble metal reagent such as Pd, Ru, Rh, Ag or Au as catalyst with few exception. To our knowledge, a synthetic route via tandem reaction initiated by copper-catalyzed allene-based Ullmann-type C–C coupling has not yet been known. Considering the power of multicomponent reactions16 as well as related cascade reactions17 in providing efficient organic synthesis, and in continuous to our research efforts in developing copper-catalyzed cross coupling and their application in designing tandem reactions,18 we report herein a new tandem assembly consists of the in situ allene generation, the oxa-Michael addition and intramolecular C–C coupling for the synthesis of 3-methyl isocoumarins. The in situ generation and utilization of the unstable allene substrates as well as the low cost copper-catalyst of the present work feature as advantageous over similar known work employing noble metal Ag catalyst and two-step synthesis.11
Results and discussion
The investigation started from the reaction of Wittig reagent 1a, acetyl chloride 2a and o-iodobenzoic acid 3a in the presence of CuI and Cs2CO3. By heating at 100 °C in DMSO, the target product 4a was acquired with 27% yield (entry 1, Table 1). Subsequently, entries employing different copper catalysts such as CuBr, CuO and Cu(OAc)2 were examined, and Cu(OAc)2 displayed much better catalytic efficiency than other candidates (entries 2–4, Table 1). The reactions conducted in the presence of different base additives, including K2CO3, K3PO4, NaHCO3, EtONa and Et3N, however, was not able to give better result (entries 5–9, Table 1). The employment of reaction medium of different polarity such as DMF, toluene and water etc. failed to improve the expect product generation, either (entries 10–13, Table 1). However, attempt in employing oxidative potassium iodate was found to be useful in enhancing the yield of 4a (entries 14–17, Table 2). Finally, the variation on the reaction temperature proved that 90 °C was proper for the reaction (entries 18–19, Table 1).
Table 1 Optimization on reaction conditionsa
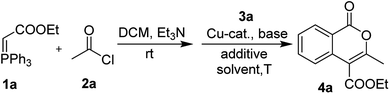
|
Entry |
Catalyst |
Base |
Additive |
Solvent |
Yieldb (%) |
The reactions were generally carried out with stepwise one-pot operation (see Experimental sections for details) 1a (0.6 mmol), 2a (0.9 mmol), 3a (o-iodobenzoic acid, 0.3 mmol), catalyst (0.03 mmol), base (0.75 mmol), additive (0.6 mmol, if applicable) and n-hexane (3 mL) in solvent (2 mL), stirred at 100 °C for 12 h (TLC); commercial Cu(OAc)2·H2O was used in all entries. Yield of isolated product based on 1a. The temperature was 90 °C. The temperature was 110 °C. |
1 |
CuI |
Cs2CO3 |
— |
DMSO |
27 |
2 |
CuBr |
Cs2CO3 |
— |
DMSO |
28 |
3 |
CuO |
Cs2CO3 |
— |
DMSO |
21 |
4 |
Cu(OAc)2 |
Cs2CO3 |
— |
DMSO |
45 |
5 |
Cu(OAc)2 |
K2CO3 |
— |
DMSO |
27 |
6 |
Cu(OAc)2 |
K3PO4 |
|
DMSO |
Trace |
7 |
Cu(OAc)2 |
NaHCO3 |
— |
DMSO |
Trace |
8 |
Cu(OAc)2 |
EtONa |
— |
DMSO |
Trace |
9 |
Cu(OAc)2 |
Et3N |
— |
DMSO |
Trace |
10 |
Cu(OAc)2 |
Cs2CO3 |
— |
DMF |
26 |
11 |
Cu(OAc)2 |
Cs2CO3 |
— |
1,4-Dioxane |
39 |
12 |
Cu(OAc)2 |
Cs2CO3 |
— |
p-Xylene |
NR |
13 |
Cu(OAc)2 |
Cs2CO3 |
— |
H2O |
NR |
14 |
Cu(OAc)2 |
Cs2CO3 |
KBr |
DMSO |
49 |
15 |
Cu(OAc)2 |
Cs2CO3 |
KI |
DMSO |
52 |
16 |
Cu(OAc)2 |
Cs2CO3 |
KIO3 |
DMSO |
56 |
17 |
Cu(OAc)2 |
Cs2CO3 |
MnO2 |
DMSO |
54 |
18c |
Cu(OAc)2 |
Cs2CO3 |
KIO3 |
DMSO |
74 |
19d |
Cu(OAc)2 |
Cs2CO3 |
KIO3 |
DMSO |
53 |
Table 2 Scope in the three-component isocoumarin synthesisa,b
The reactions were generally carried out with one-pot stepwise operation (see Experimental sections for details): 1 (0.6 mmol), 2a (0.9 mmol), 3 (0.3 mmol), Cu(OAc)2 (0.03 mmol), Cs2CO3 (0.75 mmol), KIO3 (0.6 mmol) and n-hexane (3 mL)/DMSO (2 mL), stirred at 90 °C for 12 h. Yield of isolated product. |
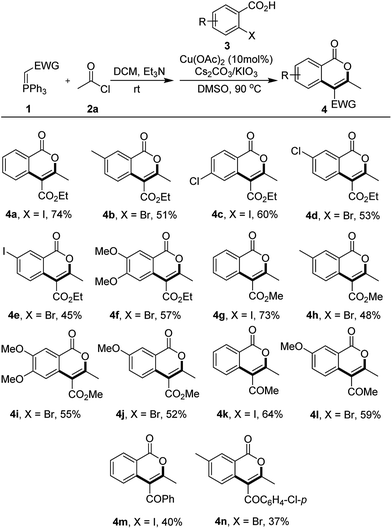 |
In subsequent work, the scope of this three-component tandem reaction on the synthesis of isocoumarins 4 was explored. As showing in Table 2, both o-iodo- and o-bromobenzoic acids could be used as building blocks in this kind of synthesis. The general tendency was that iodinated benzoic acids provided corresponding products with higher yield than those equivalent entries employing o-bromobenzoic acids, suggesting that the reactivity of the Ar–X bond evidently influenced the efficiency of the target product synthesis (comparing 4a, 4c, 4g with 4b, 4d, 4e, 4f, 4h, 4i and 4j in Table 2). On the other hand, the entries employing aryl ketone-based Wittig reagents gave much lower yield of corresponding isocoumarins than those ones constructed by alkyl ketone-based Wittig reagents (comparing 4k, 4l with 4m, 4n in Table 2), which indicated that the electron withdrawing effect resulting from the aryl ring was negative to expect reaction by reducing the nucleophilicity of the α-carbon in the in situ generated allene intermediate. In addition, the attempts in employing other linear acyl chlorides such as propionyl chloride and butyryl chloride for the reaction failed to give the expected isocoumarins. The consequence might be attributed to the additional steric effect resulting from the alkyl substitution with corresponding allene intermediate 5 which hampled the addition of the carboxylic acid ion of weak nucleophilicity.
To illustrate the possible process forming the isocoumarin products via the copper-catalyzed C–C coupling, a plausible mechanism for the present tandem reactions is proposed in Scheme 1. The reaction is supposed to start from the oxa-Michael addition of the carboxylic acid to the in situ generated allene intermediate 5 which provides vinyl ether 6. The tautomerization of 6 leads to the occurrence of the intermediate 7. Under the promotion of the Cs2CO3, the nucleophilic carbon site in intermediate 7 attacks Cu(OAc)2 via a formal nucleophilic substitution to afford Cu(II) complex A. Subsequently, the oxidative addition of the copper site to the Ar–X bond takes place and generates the seven-membered Cu(IV) complex B. Finally, the reductive elimination on B allowed the production of target product 4 and the regeneration of the Cu(II) catalyst. The role of KIO3 in the reaction is not yet clear, a possibility is that the KIO3 can oxidise the halid (X−) produced during the product formation and promote the reaction to run toward the positive direction.
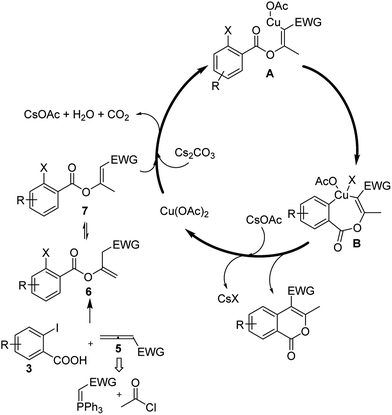 |
| Scheme 1 The plausible reaction mechanism. | |
Conclusions
In summary, we have established a tandem reaction tactic wherein the Wittig reaction, oxa-Michael addition and a copper-catalyzed Ullmann-type C–C coupling have been involved for the facile synthesis of 3-methyl isocoumarins. This synthetic method starts from simple materials and require no noble metal catalyst, which can be a useful complementary approach in the synthesis of valuable isocoumarin scaffolds.
Experimental section
General procedure for the synthesis of isocoumarins 4
In a 25 mL round-bottom flask was charged with phosphorus ylide 1 (0.6 mmol), CH2Cl2 (1 mL), and Et3N (0.66 mmol). A solution of acetyl chloride 2a (0.9 mmol) in CH2Cl2 (1 mL) was added dropwise with stirring. After an additional 4 h stirring, the CH2Cl2 was completely evaporated under reduced pressure. n-Hexane (3 mL), o-halobenzoic acid 3 (0.3 mmol), Cu(OAc)2 (0.03 mmol), Cs2CO3 (0.75 mmol), KIO3 (0.6 mmol) and DMSO (2 mL) were then consequently added. The resulting mixture was heated at 90 °C for 12 h (TLC). The reaction mixture was allowed to cool to rt, and H2O (10 mL) was added. The resulting suspension was extracted with ethyl acetate (3 × 10 mL). The organic phases were combined and dried over Na2SO4. After filtration and removing the solvent at reduced pressure, the residue was subjected to silica gel column chromatography to give the pure product by using mixed petroleum ether/ethyl acetate (v/v = 20
:
1) as the eluent.
Ethyl 3-methyl-1-oxo-1H-isochromene-4-carboxylate (4a)11. Yellow liquid; 1H NMR (400 MHz, CDCl3): δ 8.29 (d, J = 8.0 Hz, 1H), 7.78–7.72 (m, 2H), 7.52 (t, J = 7.2 Hz, 1H), 4.49–4.43 (m, 2H), 2.46 (s, 3H), 1.44 (t, J = 7.2 Hz, 3H); 13C NMR (100 MHz, CDCl3): 165.8, 161.2, 157.7, 135.1, 134.6, 129.7, 128.2, 124.1, 119.5, 110.3, 61.7, 19.3, 14.3.
Ethyl 3,7-dimethyl-1-oxo-1H-isochromene-4-carboxylate (4b). White soild; mp 76–78 °C. 1H NMR (400 MHz, CDCl3): δ 8.07 (s, 1H), 7.67 (d, J = 8.4 Hz, 1H), 7.54 (d, J = 8.4 Hz, 1H), 4.48–4.42 (m, 2H), 2.45 (s, 6H), 1.43 (t, J = 7.2 Hz, 3H); 13C NMR (100 MHz, CDCl3): 165.9, 161.4, 156.9, 138.4, 136.3, 132.1, 129.3, 124.1, 119.4, 110.1, 61.6, 21.2, 19.2, 14.3; ESI-HRMS calcd for C14H15O4 [M + H]+ 247.0965, found 247.0966.
Ethyl 7-chloro-3-methyl-1-oxo-1H-isochromene-4-carboxylate (4d)11. White soild; mp 97–99 °C; 1H NMR (400 MHz, CDCl3): δ 8.25 (d, J = 2.0 Hz, 1H), 7.79 (d, J = 8.8 Hz, 1H), 7.67 (dd, J1 = 8.8 Hz, J2 = 2.4 Hz, 1H), 4.48–4.42 (m, 2H), 2.48 (s, 3H), 1.43 (t, J = 7.2 Hz, 3H); 13C NMR (100 MHz, CDCl3): 165.4, 160.1, 158.5, 135.4, 134.1, 133.1, 129.0, 126.0, 120.9, 109.6, 61.9, 19.5, 14.2.
Methyl 3-methyl-1-oxo-1H-isochromene-4-carboxylate (4g). Colorless liquid. 1H NMR (400 MHz, CDCl3): δ 8.28 (d, J = 7.6 Hz, 1H), 7.75–7.73 (m, 2H), 7.53–7.49 (m, 1H), 3.98 (s, 3H), 2.45 (s, 3H); 13C NMR (100 MHz, CDCl3): 166.2, 161.1, 135.1, 134.5, 129.6, 128.2, 124.2, 119.4, 110.0, 52.4, 19.4; ESI-HRMS calcd for C12H11O4 [M + H]+ 219.0652, found 219.0655.
Methyl 6,7-dimethoxy-3-methyl-1-oxo-1H-isochromene-4-carboxylate (4i). White solid; mp 154–156 °C; 1H NMR (400 MHz, CDCl3): δ 7.64 (s, 1H), 7.33 (s, 1H), 3.99 (s, 3H), 3.98 (s, 3H), 3.97 (s, 3H), 2.48 (s, 3H); 13C NMR (100 MHz, CDCl3): 166.53, 166.52, 158.0, 155.3, 149.6, 130.3, 112.7, 109.5, 109.2, 105.5, 56.3, 56.2, 52.3, 19.8; ESI-HRMS calcd for C14H15O6 [M + H]+ 279.0863, found 279.0862.
Acknowledgements
This work is financially supported by National Natural Science Foundation of China (21562024, 21202064).
Notes and references
- For selected reviews, see:
(a) F. Monnier and M. Taillefer, Angew. Chem., Int. Ed., 2008, 47, 3096 CrossRef CAS PubMed;
(b) F. Monnier and M. Taillefer, Angew. Chem., Int. Ed., 2009, 48, 6954 CrossRef CAS PubMed;
(c) J. Hassan, M. Sévignon, C. Gozzi, E. Schulz and M. Lemaire, Chem. Rev., 2002, 102, 1359 CrossRef CAS PubMed;
(d) C. Sambiagio, S. P. Marsden, A. J. Blacker and P. C. McGowan, Chem. Soc. Rev., 2014, 43, 3525 RSC;
(e) Y. Liu and J.-P. Wan, Chem.–Asian J., 2012, 7, 1488 CrossRef CAS PubMed;
(f) Y. Liu and J.-P. Wan, Org. Biomol. Chem., 2011, 9, 6873 RSC.
-
(a) C. He, G. Zhang, J. Ke, H. Zhang, J. T. Miller, A. J. Kropf and A. Lei, J. Am. Chem. Soc., 2013, 135, 488 CrossRef CAS PubMed;
(b) E. J. Hennessy and S. L. Buchwald, Org. Lett., 2002, 4, 269 CrossRef CAS PubMed;
(c) H.-J. Cristau, P. P. Cellier, J.-F. Spindler and M. Taillefer, Chem.–Eur. J., 2004, 10, 5607 CrossRef CAS PubMed;
(d) Q. Niu, H. Mao, G. Yuan, J. Gao, H. Liu, Y. Tu, X. Wang and X. Lv, Adv. Synth. Catal., 2013, 355, 1185 CrossRef CAS;
(e) S. F. Yip, H. Y. Cheung, Z. Zhou and F. Y. Kwong, Org. Lett., 2007, 9, 3469 CrossRef CAS PubMed;
(f) D. Yang, H. Yang and H. Fu, Chem. Commun., 2011, 47, 2348 RSC;
(g) Y. Fang and C. Li, J. Org. Chem., 2006, 71, 6427 CrossRef CAS PubMed;
(h) B. Lu and D. Ma, Org. Lett., 2006, 8, 6115 CrossRef CAS PubMed;
(i) J. Lu, X. Gong, H. Yang and H. Fu, Chem. Commun., 2010, 46, 4172 RSC;
(j) C. C. Malakar, D. Schmidt, J. Conrad and U. Beifuss, Org. Lett., 2011, 13, 1972 CrossRef CAS PubMed;
(k) M. Jiang, J. Li, F. Wang, Y. Zhao, F. Zhao, X. Dong and W. Zhao, Org. Lett., 2012, 14, 1420 CrossRef CAS PubMed;
(l) Z. Lou, S. Zhang, C. Chen, X. Pan, M. Li and L. Wen, Adv. Synth. Catal., 2014, 356, 153 CrossRef CAS.
-
(a) B. Li, S. Guo, J. Zhang, X. Zhang and X. Fan, J. Org. Chem., 2015, 80, 5444 CrossRef CAS PubMed;
(b) X. Wang, J. Liu, H. Guo, C. Ma, X. Gao, K. Zhou and G. Huang, Synthesis, 2012, 44, 1037 CrossRef CAS;
(c) Q. Cai, J. Yan and K. Ding, Org. Lett., 2012, 41, 3332 CrossRef PubMed.
-
(a) Y. Liu, H. Wang and J.-P. Wan, J. Org. Chem., 2014, 79, 10599 CrossRef CAS PubMed;
(b) J.-P. Wan, H. Wang, Y. Liu and H. Ding, Org. Lett., 2014, 16, 5160 CrossRef CAS PubMed.
- B. Huang, D. Hu, J. Wang, J.-P. Wan and Y. Liu, Tetrahedron Lett., 2015, 56, 2551 CrossRef CAS.
-
(a) S. Pal, V. Chatare and M. Pal, Curr. Org. Chem., 2011, 15, 782 CrossRef CAS;
(b) G. Qadeer, N. H. Rama and S. J. H. Shah, Arkivoc, 2007, XIV, 12 Search PubMed;
(c) A. Saeed, Eur. J. Med. Chem., 2016, 116, 290 CrossRef CAS PubMed;
(d) K. Tianpanich, S. Prachya, S. Wiyakrutta, C. Mahidol, S. Ruchirawat and P. Kittakoop, J. Nat. Prod., 2011, 74, 79 CrossRef CAS PubMed;
(e) K. Nozawa, M. Yamada, Y. Tsuda, K. Kawai and S. Nakajima, Chem. Pharm. Bull., 1981, 29, 2689 CrossRef CAS PubMed;
(f) J. M. Dickinson, Nat. Prod. Rep., 1993, 10, 71 RSC;
(g) J. E. Kerrigan, J. Oleksyszyn, C.-M. Kam, J. Selzler and J. C. Powers, J. Med. Chem., 1995, 38, 544 CrossRef CAS PubMed.
- For reviews, see:
(a) Z. Ashraf, Chem. Heterocycl. Compd., 2016, 52, 149 CrossRef CAS;
(b) A. Saeed, M. Haroon, F. Muhammad, F. A. Larik, E.-S. Hesham and P. A. Channar, J. Organomet. Chem., 2017, 834, 88 CrossRef CAS.
-
(a) J. Mo, L. Wang and X. Cui, Org. Lett., 2015, 17, 4960 CrossRef CAS PubMed;
(b) X. G. Li, K. Liu, G. Zou and P. N. Liu, Adv. Synth. Catal., 2014, 356, 1496 CrossRef CAS;
(c) L. Song, J. Xiao, W. Dong, Z. Peng and D. An, Eur. J. Org. Chem., 2017, 341 CrossRef CAS;
(d) P. B. Dalvi, K.-L. Lin, M. V. Kulkarni and C.-M. Sun, Org. Lett., 2016, 18, 3706 CrossRef CAS PubMed;
(e) K. S. Singh, S. G. Sawant and P. H. Dixneuf, ChemCatChem, 2016, 8, 1046 CrossRef CAS;
(f) Y. Unoh, K. Hirano, T. Satoh and M. Miura, Tetrahedron, 2013, 69, 4454 CrossRef CAS;
(g) L. Ackermann, J. Pospech, K. Graczyk and K. Rauch, Org. Lett., 2012, 14, 930 CrossRef CAS PubMed;
(h) R. K. Chinnagolla and M. Jeganmohan, Chem. Commun., 2012, 48, 2030 RSC.
-
(a) X. G. Li, M. Sun, K. Liu, Q. Jin and P. N. Liu, Chem. Commun., 2015, 51, 2380 RSC;
(b) C. Yang, X. He, L. Zhang, G. Han, Y. Zuo and Y. Shang, J. Org. Chem., 2017, 82, 2081 CrossRef CAS PubMed.
- Y. Hara, S. Onodera, T. Kochi and F. Kakiuchi, Org. Lett., 2015, 17, 4850 CrossRef CAS PubMed.
- N. Panda, P. Mishra and I. Mattan, J. Org. Chem., 2016, 81, 1047 CrossRef CAS PubMed.
-
(a) H. Liu, Y. Yang, J. Wu, X.-N. Wang and J. Chang, Chem. Commun., 2016, 52, 6801 RSC;
(b) X. Guo, J. Org. Chem., 2013, 78, 1660 CrossRef CAS PubMed;
(c) R. C. Larock, M. J. Doty and X. Han, J. Org. Chem., 1999, 64, 8770 CrossRef CAS PubMed;
(d) M. A. Waseem, Shireen, A. A. Abumahdi, A. Srivastava, A. Srivastava, Rahila and I. R. Siddiqui, Catal. Commun., 2014, 55, 70 CrossRef CAS;
(e) M. R. Kumar, F. M. Irudayanathan, J. H. Moon and S. Lee, Adv. Synth. Catal., 2013, 355, 3221 CrossRef CAS.
-
(a) F.-J. Zhang, M.-Y. Sun, Y.-J. Dang, X.-Y. Meng, B. Jiang, W.-J. Hao and S.-J. Tu, Tetrahedron, 2014, 70, 9628 CrossRef;
(b) S. Pathak, D. Das, A. Kundu, S. Maity, N. Guchhait and A. Pramanik, RSC Adv., 2015, 5, 17308 RSC;
(c) P. M. Shpuntov, V. A. Shcherbinin, V. T. Abaev and A. V. Butin, Tetrahedron Lett., 2016, 57, 1483 CrossRef CAS;
(d) P. P. Kaishap, B. Sarma and S. Gogoi, Chem. Commun., 2016, 52, 9809 RSC;
(e) R. Prakash, K. Shekarrao, S. Gogoi and R. C. Boruah, Chem. Commun., 2015, 51, 9972 RSC.
- D. Nandi, D. Ghosh, S.-J. Chen, B.-C. Kuo, N. M. Wang and H. M. Lee, J. Org. Chem., 2013, 78, 3445 CrossRef CAS PubMed.
-
(a) Y. He, X. Zhang, N. Shen and X. Fan, J. Org. Chem., 2013, 78, 10178 CrossRef CAS PubMed;
(b) Q. Yuan, Z.-B. Chen, F.-L. Zhang and Y.-M. Zhu, Org. Biomol. Chem., 2017, 15, 1628 RSC;
(c) K. Sudarshan, M. K. Manna and I. S. Aidhen, Eur. J. Org. Chem., 2015, 1797 CrossRef CAS;
(d) N. A. Mallampudi, G. S. Reddy, S. Maity and D. K. Mohapatra, Org. Lett., 2017, 19, 2074 CrossRef CAS PubMed;
(e) S.-L. Zhang and Z.-L. Yu, Org. Biomol. Chem., 2016, 14, 10511 RSC;
(f) M. Toure, S. Jaime-Figureroa, G. M. Burslem and C. M. Crews, Eur. J. Org. Chem., 2016, 4171 CrossRef CAS;
(g) M. Zhang, H.-J. Zhang, T. Han, W. Ruan and T.-B. Wen, J. Org. Chem., 2015, 80, 620 CrossRef CAS PubMed;
(h) B. Chen and S. Ma, Org. Lett., 2013, 15, 3884 CrossRef CAS PubMed;
(i) X.-D. Fei, Z.-Y. Ge, T. Tang, Y.-M. Zhu and S.-J. Ji, J. Org. Chem., 2012, 77, 10321 CrossRef CAS PubMed;
(j) P.-Y. Chen, K.-S. Huang, C.-C. Tsai, T.-P. Wang and E.-C. Wang, Org. Lett., 2012, 14, 4930 CrossRef CAS PubMed;
(k) S. Cai, F. Wang and C. Xi, J. Org. Chem., 2012, 77, 2331 CrossRef CAS PubMed.
- For selected references on multicomponent reactions, see:
(a) B. B. Toure and D. G. Hall, Chem. Rev., 2009, 109, 4439 CrossRef CAS PubMed;
(b) A. Dömling, W. Wang and K. Wang, Chem. Rev., 2012, 112, 3083 CrossRef PubMed;
(c) Y. Liu, H. Wang and J.-P. Wan, Asian J. Org. Chem., 2013, 2, 374 CrossRef CAS;
(d) F. Shi, Z.-L. Tao, S.-W. Luo, S.-J. Tu and L.-Z. Gong, Chem.–Eur. J., 2012, 18, 6885 CrossRef CAS PubMed;
(e) F. Shi, G.-J. Xing, R.-Y. Zhu, W. Tan and S. Tu, Org. Lett., 2013, 15, 128 CrossRef CAS PubMed;
(f) W. Dai, X.-L. Jiang, J.-Y. Tao and F. Shi, J. Org. Chem., 2016, 81, 185 CrossRef CAS PubMed;
(g) J.-P. Wan, Y. Lin, Q. Huang and Y. Liu, J. Org. Chem., 2014, 79, 7232 CrossRef CAS PubMed.
- For selected references on cascade reactions, see:
(a) K. C. Nicolaou and J. S. Chen, Chem. Soc. Rev., 2009, 38, 2993 RSC;
(b) C. Grondal, M. Jeanty and D. Enders, Nat. Chem., 2010, 2, 167 CrossRef CAS PubMed;
(c) J.-P. Wan and Y. Gao, Chem. Rec., 2016, 16, 1164 CrossRef CAS PubMed;
(d) Y.-C. Zhang, J.-J. Zhao, F. Jiang, S.-B. Sun and F. Shi, Angew. Chem., Int. Ed., 2014, 53, 13912 CrossRef CAS PubMed;
(e) F. Shi, H.-H. Zhang, X.-X. Sun, J. Liang, T. Fan and S.-J. Tu, Chem.–Eur. J., 2015, 21, 3465 CrossRef CAS PubMed;
(f) F. Jiang, Y.-C. Zhang, X. Yang, Q.-N. Zhu and F. Shi, Synlett, 2016, 27, 575 CAS;
(g) J.-P. Wan, Y. Jing, C. Hu and S. Sheng, J. Org. Chem., 2016, 81, 6826 CrossRef CAS PubMed;
(h) Y. Li, J.-P. Wan and C. Wen, Tetrahedron, 2017, 73, 2323 CrossRef CAS.
-
(a) X. Chen, W. Hao and Y. Liu, Org. Biomol. Chem., 2017, 15, 3423 RSC;
(b) Y. Liu, M. Huang and L. Wei, Asian J. Org. Chem., 2017, 6, 41 CrossRef CAS;
(c) Y. Liu, H. Wang, J. Zhang, J.-P. Wan and C. Wen, RSC Adv., 2014, 4, 19472 RSC;
(d) Y. Liu, B. Huang, X. Cao, D. Wu and J.-P. Wan, RSC Adv., 2014, 4, 37733 RSC;
(e) Y. Liu, H. Wang, X. Cao, Z. Fang and J.-P. Wan, Synthesis, 2013, 45, 2977 CrossRef CAS.
Footnote |
† Electronic supplementary information (ESI) available: General experimental information, full characterization data and 1H/13C NMR spectra. See DOI: 10.1039/c7ra06707k |
|
This journal is © The Royal Society of Chemistry 2017 |
Click here to see how this site uses Cookies. View our privacy policy here.