DOI:
10.1039/C7RA06006H
(Paper)
RSC Adv., 2017,
7, 37830-37838
2-Hydroxyeupatolide attenuates inflammatory responses via the inhibiting of NF-κB signaling pathways
Received
29th May 2017
, Accepted 27th July 2017
First published on 1st August 2017
Abstract
2-Hydroxyeupatolide (2-HE), a sesquiterpene lactone, was found in the genus Eupatorium that has been used for a long time in traditional Chinese medicine for the treatment of several diseases. In this study, the anti-inflammatory activity of 2-HE and the underlying mechanism of this action were explored. We demonstrated that 2-HE ameliorated hyperemia, edema, alveolar wall thickening, and inflammatory cell infiltration in the lung tissues, and inhibited the serum inflammatory cytokines, interleukin-1β (IL-1β), interleukin-6 (IL-6), and tumor necrosis factor-α (TNF-α) of lipopolysaccharide (LPS) challenged ICR mice effectively. Besides, 2-HE inhibited the mRNA expression levels of IL-6 in lung, spleen, and hypothalamus in the mice. Moreover, 2-HE was found to suppress the production of nitric oxide (NO) and the mRNA and protein levels of TNF-α, IL-1β, and IL-6 in LPS-treated RAW 264.7 macrophage cells in a concentration-dependent manner. The mechanistic study showed that 2-HE inhibited the nuclear factor-kappa B (NF-κB) transactivity and nucleic translocation of NF-κB p65 and p-NF-κB p65 in RAW 264.7 cells. Taken together, our study suggested that 2-HE may suppress the inflammatory response through inhibiting the activation of NF-κB signaling pathways, and make a contribution to further understanding the pharmaceutical activity of Eupatorium plants.
1. Introduction
Inflammation is a common fundamental pathological process for biological organisms. A suitable inflammatory response is beneficial for resisting organ damage, clearing the infection and promoting wound healing. However, an excessive inflammatory response can lead to negative effects on tissue function or even result in overt tissue damage.1 Further, numerous reports have demonstrated that inflammation can even be detrimental, as it is associated with many diseases such as atherosclerosis, cancer, asthma, rheumatoid arthritis, and diabetes mellitus.2–5 Nonsteroidal anti-inflammatory drugs (NSAIDs) are often the first line of anti-inflammatory agents worldwide. It is still urgent to develop more effective and less toxic agents to combat inflammatory diseases.
Pro-inflammatory cytokines such as TNF-α, IL-1β, IL-6, and key pro-inflammatory mediators such as NO can be released by activated macrophages, which play a crucial role in a lot of inflammatory processes.6–8 These inflammatory molecules can be produced by LPS, a primary component of endotoxin of the outer membrane of Gram-negative bacteria induced macrophages.6,9,10 Besides, in vivo investigations showed peripheral immune stimuli by LPS induced acute inflammatory injury via secreting pro-inflammatory factors including IL-1β, TNF-α, and IL-6 in various tissues, for example lung and spleen as well as specific brain structure hypothalamic.11–13 Previous literatures had reported that suppressing the production of these pro-inflammatory factors was a potential way to inhibit inflammation.14–18 Therefore, the drugs which can suppress the production of pro-inflammatory factors could be potential for treatment of inflammatory diseases and endotoxin shock.
Accumulating study has highlighted the crucial role of nuclear factor-kappa B (NF-κB) signaling pathway in the production of pro-inflammatory cytokines,16,19–22 and increasing attention has been concentrated on the development of anti-inflammatory drugs targeting NF-κB.23 In the unstimulated state, NF-κB resides in the cytoplasm as heterodimers p50 (also known as NF-κB1) and p65 (also known as RelA and NF-κB3), and binds to its inhibitory IκBs, which mask the nuclear localization signal. Stimuli (such as LPS and TNF-α) that activate NF-κB initiates a cascade of signaling events including phosphorylation, ubiquination, and subsequent proteasomal degradation of IκB.24 Consequently, liberated NF-κB translocates to the nucleus and initiates transcription of many genes. The NF-κB protein p65 can be inducibly phosphorylated by the same stimuli that cause degradation of IκB. Thus, pathways that regulate the phosphorylation of NF-κB p65 and the nuclear translocation of p-NF-κB p65 constitute another potential mechanism for controlling the transcriptional activity of NF-κB.
2-HE is a natural compound occurring in genus Eupatorium chinense L. (Compositae) that has been used in traditional Chinese medicine for the treatment of several diseases.25–27 It belongs to sesquiterpene lactones that have diverse biological activities, especially cytotoxic.25,28,29 So far, few reports have been issued on the anti-inflammatory activity of 2-HE and the underlying mechanisms involved. In this study, we aim to explore the anti-inflammatory property of 2-HE in LPS-induced acute inflammation in ICR mice in vivo and RAW 264.7 macrophages in vitro, and enlarge our knowledge of pharmacological activities of plant Eupatorium.
2. Materials and methods
2.1. Chemicals and reagents
2-HE of 98% purity was purchased from Shanghai R&D Center for Standardization of TCM (Shanghai, China), and the chemical structure was shown in Fig. 1. LPS, dimethyl sulfoxide (DMSO), dexamethasone (DXM), and MTT were purchased from the Sigma Chemical Co. (St. Louis, MO, USA).
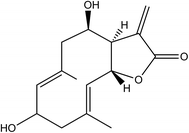 |
| Fig. 1 The chemical structure of 2-HE. | |
2.2. Animals and treatments
The protocols for the animal study were approved by the Institute Animal Care and Use Committee of Shanghai University of Traditional Chinese Medicine (Approved Number: 2013014). Seven-week old ICR mice were purchased from the SLAC Laboratory (Shanghai, China), and were housed at a controlled temperature (22–23 °C) on a 12 h light/dark cycle with food and water ad libitum. To investigate the effects of 2-HE in vivo, mice were housed one week to adapt to the environment, and randomly assigned to five groups: a control group (saline) (control), a LPS-treated group (10 mg kg−1) (LPS), a LPS- and DXM (3 mg kg−1)-treated group (LPS + DXM), two LPS- and 2-HE (30 and 100 mg per kg body weight per day, respectively)-treated groups (2-HE 30 + LPS and 2-HE 100 + LPS). DXM (3 mg kg−1), compound 2-HE (30, and 100 mg kg−1), and saline were administered by intraperitoneal injection (i.p.) for two days. The next day, the mice were treated with DXM and 2-HE before injection with LPS. One hour later, the treated mice received LPS (10 mg kg−1) by i.p. All mice were sacrificed 6 h after LPS treatment, anesthetized using 20% urethane (Sigma, St. Louis, MO, USA), and then were dissected. The cardiac blood samples were collected, then the lung, hypothalamus, and spleen were separated and snap-frozen using liquid nitrogen and stored at −80 °C.
2.3. Enzyme-linked immunosorbent assay (ELISA)
The effect of 2-HE on the production of TNF-α, IL-1β, and IL-6 in vivo and in vitro were measured by ELISA. For detecting the levels of TNF-α, IL-1β, and IL-6 in serum, the cardiac blood samples were collected and centrifuged at 800 g for 15 min, and then the serum was collected and stored at −80 °C. In vitro, RAW 264.7 cells (ATCC, Rockville, MD, USA) were seeded into 48-well plates (2 × 106 cells per well) and cultured at 37 °C, 5% CO2, and 95% humidity overnight. Cells were pre-incubated with 2-HE (0, 1, 2.5, 5 10, or 25 μM) for 1 h, then stimulated with 1 μg mL−1 LPS for another 24 h. Subsequently, the cell supernatant was collected. Both the serum and cell supernatant TNF-α, IL-1β, and IL-6 levels were measured with TNF-α (Biolegend, USA), IL-1β, and IL-6 ELISA Kit (eBioscience, USA), according to the instructions of the manufacturers, respectively. The production of pro-inflammatory cytokines was calculated according to the standard curve in each ELISA kits. All experiments above were performed in triplicate.
2.4. Histological examination of lung
For histological examinations staining, the lung tissue was fixed in 4% formaldehyde, embedded in paraffin and cut into 10 μm sections which were stained with hematoxylin and eosin (H&E) according to a standard protocol. Finally, the histopathologic changes of the lung tissues were examined using a Zeiss Axio Imager microscope (Zeiss, Germany).
2.5. RNA extraction and quantitative PCR
Total RNA from mice hypothalamus, lung, and spleen or RAW 264.7 cells were extracted using the Trizol reagent (TaKaRa, Tokyo, Japan). The RNA samples were subjected to DNase I (Invitrogen) digestion to remove possible contamination of genomic DNA. The first-strand cDNA was synthesized using a cDNA synthesis kit (Fermentas, Madison, WI, USA). Quantitative real-time polymerase chain reaction (RT-PCR) was carried out using SYBR green PCR Mastermix. The results were analyzed on an ABI StepOnePlus real-time PCR system (Applied Biosystems, Carlsbad, CA, USA). Values were normalized to beta-actin (β-actin) and all the results were obtained from at least three experiments independently. The sequences of primers (Generay Biotech Co., Ltd, Shanghai, China) used in this study are listed in Table 1.
Table 1 Sequences of the mouse primers used in RT-PCR
Gene |
Primer sequences |
TNF-α |
Forward |
5′-ATGGATCTCAAAGACAACCAACTAG-3′ |
Reverse |
5′-ACGGCAGAGAGGAGGTTGACTT-3′ |
IL-1β |
Forward |
5′-TCGTGCTGTCGGACCCATAT-3′ |
Reverse |
5′-GGTTCTCCTTGTACAAAGCTCATG-3′ |
IL-6 |
Forward |
5′-AACCACGGCCTTCCCTACTT-3′ |
Reverse |
5′-TCTGTTGGGAGTGGTATCCTCTGT-3′ |
β-Actin |
Forward |
5′-TGTCCACCTTCCAGCAGATGT-3′ |
Reverse |
5′-AGCTCAGTAACAGTCCGCCTAGA-3′ |
2.6. Cell culture and treatment
The macrophage RAW 264.7 cell line was cultured in DMEM (Hyclone, Logan, UT) containing 10% fetal bovine serum (FBS, HyClone, Logan, UT, USA), 100 μg mL−1 streptomycin, and 100 U/mL penicillin at 37 °C in an atmosphere containing 5% CO2 and 95% humidity. The cells were pretreated with the indicated concentrations of 2-HE for 1 h in the presence or absence of LPS (1 μg mL−1).
2.7. Cell viability assay
Cell viability was evaluated by 3-(4,5-dimethylthiazol-2-yl)-2,5-diphenyltetrazolium bromide (MTT, sigma, USA) assay. RAW 264.7 cells were seeded into 96-well plates and cultured for 24 h. Then the cells were treated with various concentrations (0, 2.5, 5, 10, 20, 50, and 100 μM) of 2-HE for another 24 h. Subsequently, the plates were incubated with 5 mg mL−1 of MTT for 4 h at 37 °C. After being treated with DMSO (100 μL per well), the cells were determined by a multi-detection microplate reader (Biotek, Synergy HT, USA).
2.8. Nitric oxide assay
Nitric oxide (NO) concentration in the culture medium was measured by the Griess reaction. In brief, RAW 264.7 cells were seeded into 48-well plates (2 × 106 cells per well) and cultured at 37 °C, 5% CO2, and 95% humidity overnight. Subsequently, the plates were supplied with fresh medium and treated with 2-HE (10, 25, and 50 μM) for 1 h before treat with LPS (1 μg mL−1) for 24 h. The cell supernatants were collected at the speed of 1000 rpm min−1 and the content of NO in cell supernatants was assayed according to the NO reagent kit (Beyotime, Beijing, China).
2.9. Transient transfection of cultured cells and reporter assays
The reporter assay was performed using the Dual-Luciferase Reporter Assay system (Promega, Madison, WI, USA). Briefly, HEK 293T cells were seeded in 48-well plates (5 × 105 cells per well) 24 h prior to transfection. Transfections were performed with pcDNA 3.1 NF-κB, pNF-κB-Luc, and pREP7-Renilla-Luc plasmids using FuGENE-HD (Roche, Mannheim, Germany) according to the manufacturer's protocol. The plates were cultured overnight and then supplied with fresh medium and treated with 2-HE (10, 25, and 50 μM) for 1 h before stimulation with TNF-α (100 ng mL−1) for 24 h. Subsequently, luciferase activities were measured by Dual Luciferase Reporter reagents according to manufacturer's instruction using a luminometer (FB 12 Luminometer, Berthold Detection Systems GmbH, Pforzheim, Germany). All samples were performed in triplicate.
2.10. Immunofluorescence staining and microscopic imaging
RAW 264.7 cells were seeded onto U10 mm cover slides in 24-well plates. 2-HE (2, 5, 10, and 25 μM) and TNF-α (100 ng mL−1) were added to the plate after the cells adherent. Then the cells were cultured at 37 °C, 5% CO2 for 24 h. Then the cells were washed with PBS, and the coverslips were fixed with 4% paraformaldehyde for 30 min at room temperature, subsequently wash with PBS three times. Cells were incubated with anti-NF-κB p65 and anti-p-NF-κB p65 (Ser536) antibodies (Cell Signaling Technology, USA) for 30 min at 37 °C. After being washed with PBS, the cells were incubated with corresponding secondary antibodies for 30 min at 37 °C followed by PBS washing twice. The coverslips were counterstained for nuclei with Hoechst for 5 min, and then washed with PBS. Coverslips were mounted, and images were taken with a Zeiss Axio Imager microscope.
2.11. Statistical analysis
All results were expressed as mean ± SEM. Data was analyzed by one-way analysis of variance (ANOVA) using the SPSS package (Version 18.0, SPSS, Chicago, IL, USA) for Windows statistical program. Differences were considered as significant when p < 0.05. All experiments were confirmed by replication in at least three independent repeats.
3. Results
3.1. Effects of 2-HE on serum TNF-α, IL-1β, and IL-6 levels in LPS-treated ICR mice
To determine the anti-inflammatory effects of 2-HE in vivo, the pro-inflammatory cytokine levels in the serum of LPS-challenged ICR mice were determined by ELISA. As shown in Fig. 2A–C, compared with the control group, the concentrations of TNF-α, IL-1β, and IL-6 in serum were significantly increased at 6 h after LPS stimulation. DXM (3 mg kg−1) and 2-HE (30 and 100 mg kg−1) pretreatment efficiently reduced the production of TNF-α, IL-1β, and IL-6.
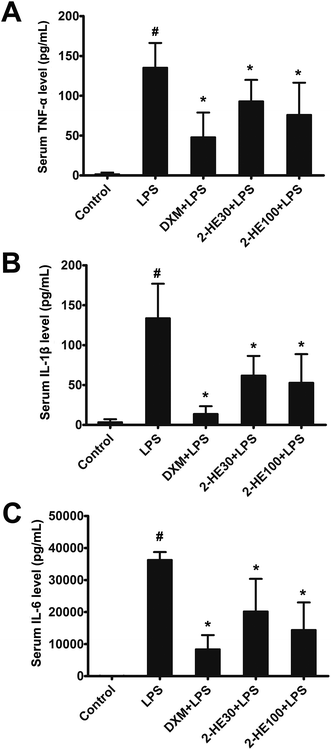 |
| Fig. 2 Effects of 2-HE on serum levels of TNF-α, IL-1β, and IL-6 levels of ICR mice. (A) Serum TNF-α; (B) serum IL-1β; (C) serum IL-6. 2-HE (30 or 100 mg kg−1) and DXM (3 mg kg−1) were administrated (i.p.) 1 h prior to LPS challenge (10 mg kg−1, i.p.) the next day, mice were sacrificed 6 h after LPS treatment, and then serum was collected for ELISA. Values are presented as mean ± SEM for six mice per group. #p < 0.05 compared with the control group; *p < 0.05 compared with the LPS-stimulated group. | |
3.2. Effects of 2-HE on the lung tissues in LPS-treated ICR mice
Lung is easy to be impaired by intraperitoneal injection LPS. To evaluate the histological changes with 2-HE treatment in LPS-induced ICR mice, the lung tissues, collected at 6 h after stimulation of LPS, were subjected to H&E staining. As shown in Fig. 3, the lung tissues of the control group showed a normal structure without histopathologic changes. In the LPS-induced group, the lung tissues exhibited significant pathologic changes, characterized by thickening of the alveolar wall, infiltration of inflammatory cells, interstitial hemorrhage, and edema. Interestingly, these serious pathological changes stimulated by LPS were effectively attenuated by 2-HE (30 and 100 mg kg−1) and DXM (3 mg kg−1) treatment.
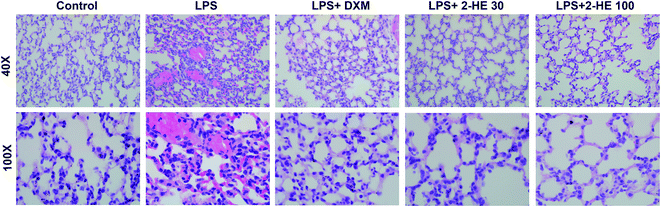 |
| Fig. 3 Effects of 2-HE on lung pathology of ICR mice induced by LPS. 2-HE (30 or 100 mg kg−1) and DXM (3 mg kg−1) were administrated (i.p.) 1 h before LPS challenge (10 mg kg−1, i.p.) the next day, mice were sacrificed 6 h after LPS treatment, and then lung samples were collected for H&E. Each group has six mice. | |
3.3. Effects of 2-HE on transcriptional levels of TNF-α, IL-1β, and IL-6 in lung, hypothalamus, and spleen of LPS-treated ICR mice
According to previous study, peripheral immune stimuli (such as i.p.) by LPS induced acute inflammatory injury in various tissues such as lung, hypothalamic as well as spleen by secreting inflammatory response including IL-1β, TNF-α, and IL-6. Therefore, we investigated the effects of 2-HE on transcriptional levels of TNF-α, IL-1β, and IL-6 of the lung, spleen, and hypothalamus by RT-PCR. Our results indicated that treatment with 2-HE obviously reduced the mRNA expression of the pro-inflammatory cytokine IL-6 in lung, hypothalamic, and spleen when compared with LPS-induced groups (Fig. 4A–C). Besides, 100 mg kg−1 of 2-HE notably decreased the expression level of IL-1β in hypothalamus (Fig. 4B). Also, the TNF-α mRNA level was also slightly downregulated in hypothalamus (Fig. 4B).
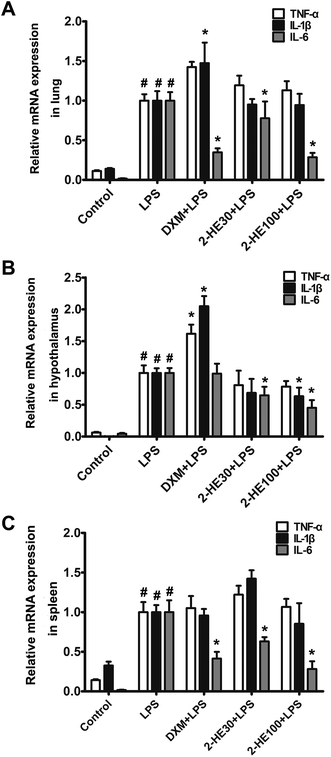 |
| Fig. 4 Effects of 2-HE on mRNA expression of TNF-α, IL-1β, and IL-6 in the tissues of ICR mice induced by LPS. (A) The mRNA expression in the lung; (B) the mRNA expression in the hypothalamus; (C) the mRNA expression in the spleen. Values are presented as mean ± SEM for six mice per group. #p < 0.05 compared with the control group; *p < 0.05 compared with the LPS-stimulated group. | |
3.4. Effects of 2-HE on the viability of RAW 264.7 cells
Results in vivo revealed that 2-HE is a potential inhibitor of LPS-stimulated inflammatory response. Next, the effects of 2-HE on RAW 264.7 macrophages were tested. To select an optimal concentration in cell experiments, we investigated the effects of 2-HE on cell viability by a MTT assay. As seen in Fig. 5, 2-HE (2.5–25 μM) did not influence the viability of RAW 264.7 macrophages. Therefore, we chose the concentration of 2-HE ranging from 0 to 25 μM for the subsequent experiment on RAW 264.7 cells.
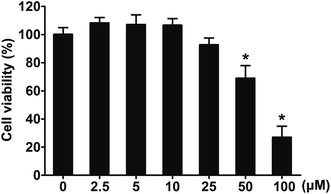 |
| Fig. 5 Effects of 2-HE on RAW 264.7 cell viability. RAW 264.7 macrophage cells were treated with LPS alone or with 2-HE (2.5, 5, 10, 25, 50, 100 μM) for 24 h, and cells viability was evaluated by a MTT assay. The values were presented as mean ± SEM for three samples per group. *p < 0.05 compared with the control group. | |
3.5. Inhibitory effects of 2-HE on NO production in RAW 264.7 cells
To investigate whether 2-HE could affect the production of NO which plays an important role in inflammatory response. NO in the culture medium of LPS-activated RAW 264.7 cells was measured by the Griess reaction. As seen in Fig. 6A, compared with the LPS group, 2-HE reduced LPS (1 μg mL−1)-stimulated NO production of macrophage RAW 264.7 cells even at concentration of 1 μM, and the inhibitory effect of 2-HE on NO production was in a concentration-dependent manner.
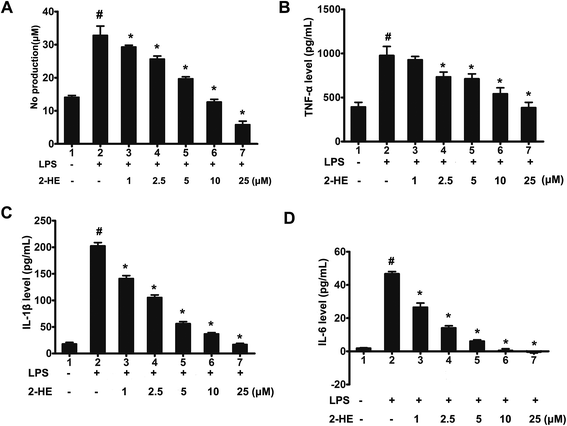 |
| Fig. 6 Effects of 2-HE on LPS-induced NO, TNF-α, IL-1β, and IL-6 production in RAW 264.7 macrophages. (A) NO; (B) TNF-α; (C) IL-1β, and (D) IL-6. Cells were treated with the indicated concentrations of 2-HE (1, 2.5, 5, 10, and 25 μM) for 1 h, and then incubated with LPS (1 μg mL−1) for 24 h. The NO, TNF-α, IL-1β and IL-6 concentrations were determined. The values were presented as mean ± SEM for three samples per group. #p < 0.05 compared with the control group; *p < 0.05 compared with the LPS-stimulated group. | |
3.6. Effects of 2-HE on pro-inflammatory cytokines production in LPS-stimulated RAW 264.7 cells
Considering that TNF-α, IL-6, and IL-1β have been reported to play a vital role in inflammatory response, we explored the secretion of these pro-inflammatory cytokines in RAW 264.7 macrophage culture medium by ELISA. The results showed that 2-HE decreased secretion of TNF-α, IL-1β, and IL-6 in a concentration-dependent manner (Fig. 6B–D). Moreover, at the concentration of 25 μM, the production of IL-6 stimulated by LPS was completely suppressed by 2-HE (Fig. 6D).
3.7. Effects of 2-HE on transcriptional levels of pro-inflammatory cytokines in LPS-stimulated RAW 264.7 cells
To further understand the anti-inflammatory effect of 2-HE on LPS-induced RAW 264.7 cells at transcriptional levels, the relative mRNA expression of pro-inflammatory cytokines in RAW 264.7 macrophage was measured. Cells were pretreated with 2-HE (1, 2.5, 5, 10 or 25 μM) for 1 h and treated with LPS (1 μg mL−1) for 24 h. The results indicated that 2-HE decreased the mRNA expression of TNF-α, IL-1β, and IL-6 in LPS-induced RAW 264.7 cells in a concentration-dependent manner (Fig. 7A–C). These results implied that the 2-HE inhibited the TNF-α, IL-1β, and IL-6 at the transcriptional level in LPS-stimulated RAW 264.7 cells.
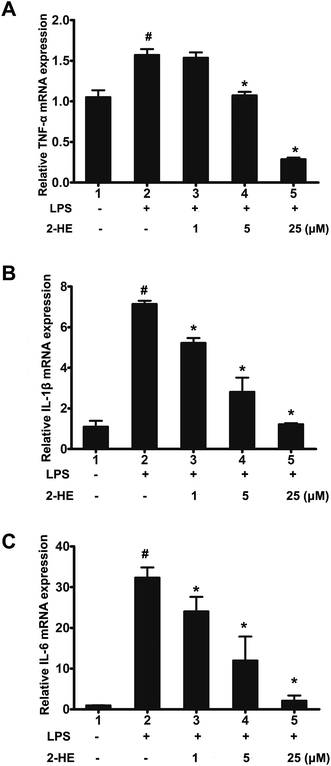 |
| Fig. 7 Effects of 2-HE on mRNA expression levels of cytokines in LPS-stimulated RAW 264.7 macrophages. (A) TNF-α; (B) IL-1β; (C) IL-6. Cells were pre-incubated with 2-HE (0, 1, 5, or 25 μM) for 1 h, and then incubated with LPS (1 μg mL−1) for 24 h. The total cell lysate was subjected to extract RNA and gene expression was performed by real time RT-PCR. The values were presented as mean ± SEM for three samples per group. #p < 0.05 compared with the control group; *p < 0.05 compared with the LPS-stimulated group. | |
3.8. 2-HE inhibited the translocation of NF-κB p65 in RAW 264.7 cells
NF-κB is an important transcription factor regulating pro-inflammatory factors such as TNF-α, IL-1β, and IL-6 in activated macrophages. Therefore, inhibiting the phosphorylation and nuclear translocation of NF-κB p65 subunit is a potential way to suppress the activation of NF-κB, and then attenuate the inflammation. As we can see in Fig. 8A, TNF-α significantly enhanced the activation of NF-κB, while, pretreatment 2-HE (10, 25, and 50 μM) inhibited this activation in a concentration-dependent manner. Further, we tested the effect of 2-HE on the nuclear translocation of NF-κB p65 subunit through the immunofluorescence assay. As shown in Fig. 8B, most NF-κB p65 resided in the cytoplasm in unstimulated cells. After TNF-stimulation, most of the cytoplasmic NF-κB p65 translocated to the nucleus. However, the nucleic localization of NF-κB p65 was effectively decreased by 2-HE (2, 5, and 10 μM). To evaluate the effect of 2-HE on the inhibition of phosphorylation of NF-κB p65 subunit and its nuclear translocation, we confirmed the nuclear level of p-NF-κB p65 in RAW 264.7 cells. Our results demonstrated that treatment with 2-HE (2, 5, and 10 μM) markedly suppressed the p-NF-κB p65 nuclear translocation which increased by TNF-α induction (Fig. 8C).
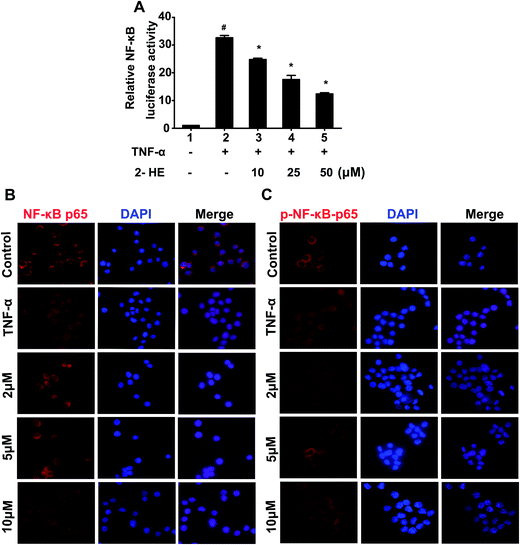 |
| Fig. 8 Effects of 2-HE on LPS-induced NF-κB transcriptional activity and nuclear translocation of the p65 subunit in RAW 264.7 cells. (A) NF-κB transcriptional activity. HEK293T cells were transiently co-transfected with pcDNA 3.1 NF-κB, pNF-κB-Luc, and pREP7-Renilla-Luc plasmids and incubated overnight, then pretreated treated with 2-HE (10, 25, 50 μM) for 1 h. TNF-α (100 ng mL−1) was then added and cells were further incubated for 24 h. The cells were harvested and then the luciferase activities were determined by using the dual luciferase report assay system. The values were presented as mean ± SEM for three samples per group. #p < 0.05 compared with control group; *p < 0.05 compared with the TNF-α-stimulated group. (B) NF-κB p65 and (C) p-NF-κB p65 were examined by immunofluorescence staining. 400×. | |
4. Discussion
The present work was aim to evaluate the anti-inflammatory activity of 2-HE, using established inflammatory models including a mouse model of LPS-induced acute inflammation in vivo and a LPS-induced RAW 264.7 macrophage cells in vitro. Our results showed that 2-HE significantly reduced the mice serum levels of TNF-α, IL-1β, and IL-6 and ameliorated hyperemia, edema, alveolar wall thickening, and inflammatory cell infiltration in lung tissues. Furthermore, 2-HE significantly inhibited the mRNA expression levels of IL-6 in lung, spleen, and hypothalamus in the acute inflammation mice. Treatment with 100 mg kg−1 of 2-HE also inhibited the expression level of IL-1β in hypothalamus. These results have been further confirmed by examining the level of these cytokines as well as NO in culture medium of LPS-induced macrophages. Besides, 2-HE suppressed the NF-κB transactivity and the translocation of NF-κB p65 and phosphorylation of NF-κB p65 to nucleus of RAW 264.7 cells, and reduced the mRNA expression of pro-inflammatory cytokines TNF-α, IL-1β, and IL-6 in the cells.
Given that pro-inflammatory cytokines such as TNF-α, IL-1β, and IL-6 mediate inflammation which impair various organ integrity,12,30 we explored the effect of 2-HE on the production of cytokines in LPS-induced acute inflammation in vivo. Our results showed that 2-HE reduced the amount of pro-inflammatory cytokines (TNF-α, IL-1β, and IL-6) in serum which were significantly increased by LPS (Fig. 2A–C). However, not all the mRNA expression of pro-inflammatory cytokines in lung, spleen, and hypothalamus were inhibited by 2-HE, the reason maybe was that we just choice one time point of 6 h in our in vivo experiment, since the mRNA expression pattern of TNF-α, IL-1β, and IL-6 were different with time changing after LPS injection.12 These results implied that 2-HE improved the inflammation of lung, hypothalamus, and spleen which maybe mainly targeted on suppressing the pro-inflammatory cytokine IL-6 at time point of 6 h. Whether 2-HE could inhibit the mRNA expression of other pro-inflammatory cytokines such as TNF-α and IL-1β and the exact mechanism involved need further evaluated. Besides, the TNF-α and IL-1β mRNA in DXM-treated group were higher when compared with the LPS-group. This is possibly associated with its side effect such as metabolic effects which had been reported.31 Taken together, our in vivo results suggest that 2-HE could be a potent anti-inflammatory drug.
Macrophages, a major inflammatory cell type in the immune system, play a vital role in numerous inflammatory processes by releasing pro-inflammatory cytokines.6–8 It has been reported that LPS can stimulate macrophages to produce inflammatory cytokines and mediator NO.17,32 In the preset work, we evaluated the anti-inflammatory properties of 2-HE in vitro using LPS-induced RAW 264.7 macrophage cells.
NO plays a various pro-inflammatory effects on many cell types such as macrophage cells.10 Less NO production by macrophages is important for removing harmful bacteria, however, too much NO production results in inflammatory responses.33 It has been reported that high levels of NO was toxic for many inflammatory-related diseases, such as rheumatoid arthritis, diabetes, and atherosclerosis.10 Therefore, whether a drug could reduce the production of NO is an important index for evaluating its anti-inflammatory effects. In our work, 2-HE effectively reduced NO production in LPS-stimulated RAW 264.7 cells in a concentration-dependent manner (Fig. 6A). These results support that 2-HE is a promising anti-inflammatory agent.
LPS, as well as TNF-α, is an effective activator of NF-κB.34 NF-κB plays an important role in the regulation of cell survival genes and to induce the expression of inflammatory cytokines, such as TNF-α, IL-1, and IL-6.35,36 Inhibiting the transcription activity of NF-κB in the macrophage nucleus can significantly suppress the expression of proinflammatory cytokines.37,38 Also, some reports have demonstrated that inhibited the nuclear translocation of NF-κB p65 subunit of NF-κB from the cytosol maybe is a potential way to suppress the NF-κB and attenuate the inflammation.39,40 For example, eupatolide, an analogue of 2-HE, had been reported to block the expression of COX-2 and iNOS by suppressing the LPS-induced expression of NF-κB and its transcription activity in RAW 264.7 macrophages.18 In our study, 2-HE inhibited the transactivity of NF-κB (Fig. 8A) and reduced nuclear levels of the NF-κB p65 and p-NF-κB p65 in LPS-induced RAW 264.7 macrophage cells (Fig. 8B and C). Thus, we inferred that 2-HE attenuates inflammation by suppressing NF-κB signal transduction of RAW 264.7 macrophage cells.
5. Conclusion
In conclusion, the present study demonstrated that 2-HE acts as an anti-inflammatory agent. We found that 2-HE significantly inhibited LPS-induced acute mouse inflammation damage in the lung, and 2-HE inhibited the production of pro-inflammatory mediator NO and pro-inflammatory cytokines such as TNF-α, IL-1β, and IL-6, suppressed the mRNA expression of TNF-α, IL-1β, and IL-6 in LPS-induced RAW 264.7 cells. The mechanistic study showed that 2-HE suppressed the activation of NF-κB and the nucleic translocation of NF-κB p65 as well as its phosphorylation form. Therefore, our findings demonstrate the potential anti-inflammatory property of 2-HE, and highlight the potential pharmaceutical use of genus Eupatorium.
Conflict of interest
There is no conflict of interest among the authors.
Abbreviations
2-HE | 2-Hydroxyeupatolide |
IL-1β | Interleukin-1β |
IL-6 | Interleukin-6 |
TNF-α | Tumor necrosis factor-α |
LPS | Lipopolysaccharide |
NO | Nitric oxide |
NF-κB | Nuclear factor-kappa B |
NSAIDs | Nonsteroidal anti-inflammatory drugs |
DXM | Dexamethasone |
DMSO | Dimethyl sulfoxide |
ELISA | Enzyme-linked immunosorbent assay |
H&E | Hematoxylin and eosin |
RT-PCR | Quantitative real-time polymerase chain reaction |
i.p. | Intraperitoneal injection |
MTT | 3-(4,5-Dimethylthiazol-2-yl)-2,5-diphenyltetrazolium bromide |
References
- R. Medzhitov, Cell, 2010, 140, 771–776 CrossRef CAS PubMed.
- I. Kriszbacher, M. Koppán and J. Bódis, N. Engl. J. Med., 2014, 353, 429–430 Search PubMed.
- B. Hoesel and J. A. Schmid, Mol. Cancer, 2013, 12, 86 CrossRef CAS PubMed.
- E. H. Choy and G. S. Panayi, N. Engl. J. Med., 2001, 344, 907–916 CrossRef CAS PubMed.
- J. Spranger, A. Kroke, M. Mohlig, K. Hoffmann, M. M. Bergmann, M. Ristow, H. Boeing and A. F. Pfeiffer, Diabetes, 2003, 52, 812–817 CrossRef CAS PubMed.
- L. Bosca, M. Zeini, P. G. Traves and S. Hortelano, Toxicology, 2005, 208, 249–258 CrossRef CAS PubMed.
- A. K. McNally and J. M. Anderson, Adv. Exp. Med. Biol., 2011, 713, 97–111 CrossRef CAS PubMed.
- M. Pohanka, S. Snopkova, K. Havlickova, P. Bostik, Z. Sinkorova, J. Fusek, K. Kuca and J. Pikula, Curr. Med. Chem., 2011, 18, 539–551 CrossRef CAS PubMed.
- C. Nathan, FASEB J., 1992, 6, 3051–3064 CAS.
- S. Moncada, J. R. Soc. Med., 1999, 92, 164–169 CrossRef CAS.
- S. Eklind, H. Hagberg, X. Wang, K. Sävman, A. L. Leverin, M. Hedtjärn and C. Mallard, Pediatr. Res., 2006, 60, 161–168 CrossRef CAS PubMed.
- S. Layé, P. Parnet, E. Goujon and R. Dantzer, Mol. Brain Res., 1994, 27, 157–162 CrossRef.
- Q. Zhang, Q. Li, B. L. Mao, G. S. Qian, J. C. Xu and Z. T. Chen, Chin. Crit. Care Med., 2004, 16, 585–588 CAS.
- S.-i. Kanno, A. Shouji, A. Tomizawa, T. Hiura, Y. Osanai, M. Ujibe, Y. Obara, N. Nakahata and M. Ishikawa, Life Sci., 2006, 78, 673–681 CrossRef CAS PubMed.
- X. Hou, Q. Tong, W. Wang, C. Shi, W. Xiong, J. Chen, X. Liu and J. Fang, J. Nat. Prod., 2015, 78, 1689–1696 CrossRef CAS PubMed.
- X. Ci, R. Ren, K. Xu, H. Li, Q. Yu, Y. Song, D. Wang, R. Li and X. Deng, Inflammation, 2010, 33, 126–136 CrossRef CAS PubMed.
- D. Feng, W. H. Ling and R. D. Duan, Inflammation Res., 2010, 59, 115–121 CrossRef CAS PubMed.
- J. Lee, N. Tae, J. J. Lee, T. Kim and J. H. Lee, Eur. J. Pharmacol., 2010, 636, 173–180 CrossRef CAS PubMed.
- L. B. Ivashkiv, Eur. J. Immunol., 2011, 41, 2477–2481 CrossRef CAS PubMed.
- K. R. Aupperle, B. L. Bennett, D. L. Boyle, P. P. Tak, A. M. Manning and G. S. Firestein, J. Immunol., 1999, 163, 427–433 CAS.
- K. Aupperle, B. Bennett, Z. Han, D. Boyle, A. Manning and G. Firestein, J. Immunol., 2001, 166, 2705–2711 CrossRef CAS.
- C. Monaco, E. Andreakos, S. Kiriakidis, C. Mauri, C. Bicknell, B. Foxwell, N. Cheshire, E. Paleolog and M. Feldmann, Proc. Natl. Acad. Sci. U. S. A., 2004, 101, 5634–5639 CrossRef CAS PubMed.
- M. Karin, Y. Yamamoto and Q. M. Wang, Nat. Rev. Drug Discovery, 2004, 3, 17–26 CrossRef CAS PubMed.
- M. J. May and S. Ghosh, Immunol. Today, 1998, 19, 80–88 CrossRef CAS PubMed.
- E. A. Sattar, A. M. Galal and G. S. Mossa, J. Nat. Prod., 1996, 59, 403–405 CrossRef CAS PubMed.
- A. Lexa, J. Fleurentin, P. R. Lehr, F. Mortier, M. Pruvost and J. M. Pelt, Planta Med., 1989, 55, 127–132 CrossRef CAS PubMed.
- S. P. Yang, J. Huo, Y. Wang, A. Liguang Lou and J. M. Yue, J. Nat. Prod., 2004, 67, 638–643 CrossRef CAS PubMed.
- D. K. Kim, N. I. Baek, S. U. Choi, C. O. Lee, K. R. Lee and O. P. Zee, J. Nat. Prod., 1997, 60, 1199–1202 CrossRef CAS PubMed.
- J. Huo, S. P. Yang, J. Ding and J. M. Yue, J. Nat. Prod., 2004, 67, 1470–1475 CrossRef CAS PubMed.
- G. Schett, D. Elewaut, I. B. Mcinnes, J. M. Dayer and M. F. Neurath, Nat. Med., 2013, 19, 822–824 CrossRef CAS PubMed.
- P. J. Barnes, Br. J. Pharmacol., 2006, 148, 245–254 CrossRef CAS PubMed.
- M. Rossol, H. Heine, U. Meusch, D. Quandt, C. Klein, M. J. Sweet and S. Hauschildt, Crit. Rev. Immunol., 2011, 31, 379–446 CrossRef CAS PubMed.
- J. Macmicking, Q. W. Xie and C. Nathan, Annu. Rev. Immunol., 1997, 15, 323–350 CrossRef CAS PubMed.
- T. Hanada and A. Yoshimura, Cytokine Growth Factor Rev., 2002, 13, 413–421 CrossRef CAS PubMed.
- A. Roshak, J. R. Jackson, M. Chabotfletcher and L. A. Marshall, J. Pharmacol. Exp. Ther., 1997, 283, 955–961 CAS.
- A. Yoshimura, Cancer Sci., 2006, 97, 439–447 CrossRef CAS PubMed.
- P. Rafiee, C. P. Johnson, M. S. Li, H. Ogawa, J. Heidemann, P. J. Fisher, T. H. Lamirand, M. F. Otterson, K. T. Wilson and D. G. Binion, J. Biol. Chem., 2002, 277, 35605–35615 CrossRef CAS PubMed.
- H. Yin, F. Zhang, M. Yu, H. Cheng, J. Lin, Y. Gao, B. Han and L. Zhu, Neuroendocrinology, 2005, 81, 10–18 CrossRef CAS PubMed.
- D. Wang and B. A. Baldwin Jr, J. Biol. Chem., 1998, 273, 29411–29416 CrossRef CAS PubMed.
- M. Naumann and C. Scheidereit, EMBO J., 1994, 13, 4597–4607 CAS.
Footnote |
† Zunli Ke, Mingxia Li and Xin Liu contributed equally to this work. |
|
This journal is © The Royal Society of Chemistry 2017 |
Click here to see how this site uses Cookies. View our privacy policy here.