DOI:
10.1039/C7RA06457H
(Paper)
RSC Adv., 2017,
7, 34448-34460
Synthesis and biological evaluation of novel neoflavonoid derivatives as potential antidiabetic agents†
Received
9th June 2017
, Accepted 3rd July 2017
First published on 7th July 2017
Abstract
Various substituted neoflavonoid derivatives were synthesized using sulfated montmorillonite K-10 as a catalyst. This method is environmental friendly, sustainable and economical, convenient in isolation and purification processes, with little byproducts, using earth-abundant catalysts and has relatively high yield. Those neoflavonoid derivatives were screened for antioxidant, a-glucosidase inhibitory, aldose reductase 2 (ALR2) inhibitory and advanced glycation end-product formation inhibitory effects. Most compounds exhibited significant antioxidant and advanced glycation end-product (AGE) formation inhibitory activities. It was interesting to note that out of thirty compounds, 8k and 8l were found to have greater ALR2 inhibitory activity than the standard drug quercetin. The pharmacological studies suggested neoflavonoid with adjacent 7,8-dihydroxy groups were more effective in inhibiting ALR2. Antidiabetic activity studies had shown that compounds 8l and 8m were equipotent to the standard drug glibenclamide in vivo. In summary, the target compound 8l provided a potential drug design concept for the development of therapeutic or prophylactic agents of diabetes and diabetes complications.
1. Introduction
Derivatives of neoflavonoids, also named 4-arylcoumarins, are widespread in nature and have possessed a broad spectrum of biological activities1 including antioxidant,2–4 antidiabetic,5 cytotoxic,6–8 antimicrobial,9–11 anti-inflammatory,12 antiprotozoal13–15 and estrogenic16 activities. Korec and co-workers17 reported that oral administration of an extract prepared from a commercial mixture of the stem barks of H. latiflora and E. caribaeum (CM) of uncertain origin decreased glucose levels in streptozotocin (STZ)-diabetic mice during short term experiments in 2000. Guerrero-Analco and co-workers18 found that the extract of H. latiflora and several 4-phenylcoumarins isolated from Rubiaceae were very effective in regulating blood glucose levels in hyperglycemia in 2007. The structure of the most effective compound (1,5-O-β-D-glucopyranosyl-7,3′,4′-trihydroxy-4-phenylcoumarin) was displayed in Fig. 1.
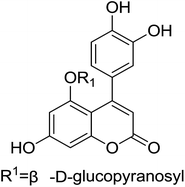 |
| Fig. 1 Structure of compound 1. | |
Owing to the potential biological activity and structural diversity of neoflavonoid, they have attracted an appreciable amount of attention in the synthetic community in recent decades.19 There are numerous reports of their synthesis in literature, but most of them have some disadvantages such as using expensive raw materials, delivering low regioselectivities and not economical or environmental friendly.20 Consequently, researchers are still looking for more environmental benign and economical synthetic processes. Lee and coworkers21 disclosed a new approach to the synthesis of neoflavonoid, based on a high yielding montmorillonite K-10 catalyzed lactone ring forming cyclization process (Fig. 2). In this article, we simplified and improved Lee's method by employing sulfated montmorillonite K-10 as catalyst (Fig. 2). In contrast to Lee's method, our substituted phenylpropiolic acids were not converted to acylate and the final product could be purified by recrystallization rather than chromatography on silica gel. In addition, we tried to use acetic acid as the solvent for this reaction, which was in accord with the concept of modern green chemistry.
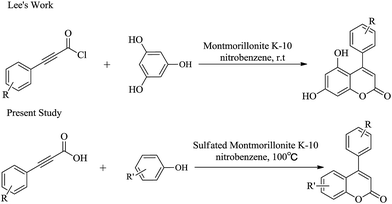 |
| Fig. 2 Methods to synthesize neoflavonoid. | |
2. Results and discussion
2.1. Chemistry
The common synthetic strategies for the target compounds 4a–4p and 8a–8n are summarized in Scheme 1. The synthetic pathway was started from Knoevenagel condensation reaction of commercially available material 1a–1e and malonic acid to afford corresponding 2a–2e.22 Then under the catalysis of sulfated montmorillonite K-10, 4a–4p can be prepared by the esterification–cyclization reaction of 2a–2e and different phenols 3a–3e. In order to confirm the optimal reaction conditions, 4-methoxyphenylacrylic acid 2a with resorcinol 3a were chosen as model substrates. This paper screened different solvents such as nitrobenzene, chlorobenzene and acetic acid. It was found that this reaction had the highest yield of 79% in the nitrobenzene and a lower yield of 66% in the acetic acid (Table 1). The further studies found that the optimal H2SO4 concentration was 30% to treat montmorillonite K-10. This paper also examined the reuse of the catalyst on the tandem esterification alkylation of substituted cinnamic acids and resorcinol to get dihydrocoumarin 4a. The results showed that montmorillonite K-10 could be reused without extra treatment to catalyze the same reaction, and the yield of 4a didn't have significant decrease (Table 2). Montmorillonite K-10 also had such advantages as low cost, non-toxicity, and commercial availability, rendering the synthetic process more environmental friendly and economical.
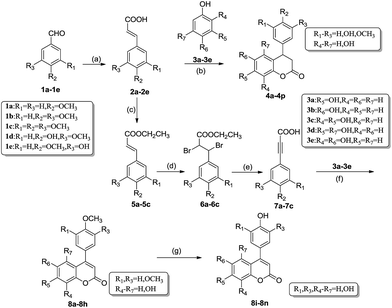 |
| Scheme 1 General synthetic route to neoflavonoid derivatives 4a–4p and 8a–8n. Reagents and conditions: (a) malonic acid, piperidine, pyridine, 90 °C; (b) nitrobenzene or acetic acid, montmorillonite K-10, 100 °C; (c) MeOH, SOCl2, reflux, 4 h; (d) Br2, CH2Cl2, 0 °C, 20 min; (e) KOH, EtOH, reflux, 6 h; (f) nitrobenzene, montmorillonite K-10, 100 °C; (g) I2, Al, acetonitrile, reflux, 5 h. | |
Table 1 The reaction conditions and yield of compound 4a. Reaction reagents and conditions: 2a (5 mmol), 3a (5.5 mmol), solvents (10 mL)
No. |
Solvents |
Concentration of H2SO4 (%) |
Montmorillonite K-10 (g) |
Reaction time (h) |
Yield (%) |
1 |
Nitrobenzene |
0 |
2 |
27 |
5 |
2 |
Nitrobenzene |
5 |
2 |
20 |
23 |
3 |
Nitrobenzene |
10 |
2 |
16 |
41 |
4 |
Nitrobenzene |
20 |
2 |
8 |
61 |
5 |
Nitrobenzene |
30 |
2 |
3 |
79 |
6 |
Nitrobenzene |
30 |
1 |
6 |
65 |
7 |
Nitrobenzene |
30 |
4 |
3 |
79 |
8 |
Nitrobenzene |
30 |
6 |
3 |
71 |
9 |
Chlorobenzene |
30 |
2 |
5 |
45 |
10 |
Acetic acid |
30 |
2 |
10 |
66 |
Table 2 Recovery of montmorillonite K-10 for synthesis of compound 4a and its corresponding yield. Reaction reagents and conditions: 2a (5 mmol), 3a (5.5 mmol), solvents (10 mL), montmorillonite K-10 (2 g)
Recycling times |
Reaction time (h) |
Yield (%) |
0 |
3 |
79 |
1 |
4.5 |
78 |
2 |
4.5 |
74 |
3 |
4.5 |
74 |
4 |
6 |
72 |
5 |
4 |
71 |
8a–8h were synthesized from different substituted cinnamic acids 2a–2c through four steps as outlined in Scheme 1. The key intermediates 4-methoxyphenylpropiolic acid (7a), 3,4-dimethoxyphenylpropiolic acid (7b) and 3,4,5-trimethoxyphenylpropiolic acid (7c) were prepared according to the routine reported previously by Sun's group.20 This procedure mainly included esterification, addition, elimination and acidification reactions. Then the neoflavonoids 8a–8h were successfully obtained via the reaction between key intermediates 7a–7c and different phenols 3a–3e in the presence of sulfated montmorillonite K-10 and nitrobenzene at 100 °C for 3–10 hours. The crude products were purified by recrystallization in pretty good yields (64–75%, Table 3). These neoflavonoids 8a–8h with 1, 2 or 3 methoxy group at the aryl in C4 could be easily converted to neoflavonoids 8i–8n with 1, 2 or 3 hydroxyl group at the aryl in C4 under the catalysis of I2 and Al in refluxing acetonitrile. Notably, the yield of this demethylating reaction was more than 90% without complex purification.
Table 3 Compounds 4a–4p and 8a–8n
Product |
R1 |
R2 |
R3 |
R4–7 |
Yield (%) |
4a |
H |
OCH3 |
H |
7-Hydroxy |
79 |
4b |
OCH3 |
OCH3 |
H |
|
53 |
4c |
OCH3 |
OH |
H |
|
55 |
4d |
H |
OCH3 |
H |
6-Hydroxy |
52 |
4e |
OCH3 |
OCH3 |
H |
|
53 |
4f |
H |
OCH3 |
H |
7,8-Dihydroxy |
79 |
4g |
OCH3 |
OCH3 |
H |
|
83 |
4h |
OCH3 |
OH |
H |
|
53 |
4i |
OH |
OCH3 |
H |
|
66 |
4j |
H |
OCH3 |
H |
5,7-Dihydroxy |
72 |
4k |
OCH3 |
OCH3 |
H |
|
62 |
4l |
OCH3 |
OH |
H |
|
53 |
4m |
OH |
OCH3 |
H |
|
64 |
4n |
H |
OH |
H |
|
62 |
4o |
OH |
OH |
H |
|
60 |
4p |
OCH3 |
OCH3 |
H |
6,8-Dihydroxy |
70 |
8a |
H |
OCH3 |
H |
7-Hydroxy |
75 |
8b |
OCH3 |
OCH3 |
H |
|
64 |
8c |
H |
OCH3 |
H |
7,8-Dihydroxy |
67 |
8d |
OCH3 |
OCH3 |
H |
|
73 |
8e |
OCH3 |
OCH3 |
OCH3 |
|
70 |
8f |
H |
OCH3 |
H |
5,7-Dihydroxy |
65 |
8g |
OCH3 |
OCH3 |
H |
|
70 |
8h |
OCH3 |
OCH3 |
H |
6,8-Dihydroxy |
72 |
8i |
OH |
OH |
H |
7-Hydroxy |
93 |
8j |
H |
OH |
H |
7,8-Dihydroxy |
93 |
8k |
OH |
OH |
H |
|
97 |
8l |
OH |
OH |
OH |
|
90 |
8m |
OH |
OH |
H |
5,7-Dihydroxy |
93 |
8n |
OH |
OH |
H |
6,8-Dihydroxy |
95 |
2.2. Biological evaluation
2.2.1. In vitro antioxidant activity. All the synthesized compounds were evaluated for their antioxidant activities in the way of scavenging 2,2-diphenyl-1-picrylhydrazyl (DPPH)23 and hydroxyl radical.24 Vitamin C was used as a reference compound in this assay. As shown in Table 4, most compounds of 4a–4p and 8a–8n demonstrated moderate to great scavenging activity, among which 8c, 8d and 8h–8n with several hydroxyl groups showed excellent activity in the both two aspects.
Table 4 Biological evaluation in vitro
Product |
DPPH |
OH |
a-Glucosidase inhibitory activity |
AGEs inhibitory activity |
ALR2 inhibitory activity |
IC50 value (μg mL−1) |
4a |
>1000 |
>2000 |
>1000 |
>1000 |
N/D |
4b |
>1000 |
>2000 |
>1000 |
51.472 ± 1.364 |
N/D |
4c |
25.146 ± 3.160 |
>2000 |
>1000 |
193.967 ± 15.232 |
N/D |
4d |
>1000 |
>2000 |
>1000 |
>1000 |
N/D |
4e |
>1000 |
>2000 |
>1000 |
>1000 |
N/D |
4f |
9.655 ± 0.151 |
996 ± 39 |
>1000 |
74.140 ± 0.420 |
N/D |
4g |
7.828 ± 0.041 |
1061 ± 29 |
>1000 |
6.730 ± 0.269 |
N/D |
4h |
6.929 ± 0.016 |
908 ± 32 |
>1000 |
121.279 ± 7.062 |
N/D |
4i |
6.396 ± 0.067 |
982 ± 27 |
>1000 |
29.706 ± 1.808 |
N/D |
4j |
568.079 ± 4.537 |
>2000 |
>1000 |
>1000 |
N/D |
4k |
459.341 ± 21.791 |
>2000 |
>1000 |
7.717 ± 0.241 |
N/D |
4l |
55.906 ± 1.589 |
>2000 |
>1000 |
5.572 ± 0.264 |
N/D |
4m |
24.462 ± 1.160 |
>2000 |
>1000 |
>1000 |
N/D |
4n |
250.661 ± 3.690 |
>2000 |
>1000 |
>1000 |
N/D |
4o |
3.673 ± 0.029 |
721 ± 20 |
>1000 |
4.712 ± 0.383 |
N/D |
4p |
15.732 ± 0.465 |
>2000 |
>1000 |
6.454 ± 0.090 |
N/D |
8a |
>1000 |
>2000 |
>1000 |
>1000 |
N/D |
8b |
>1000 |
>2000 |
>1000 |
1.605 ± 0.038 |
N/D |
8c |
9.544 ± 0.139 |
599 ± 16 |
>1000 |
2.257 ± 0.619 |
N/D |
8d |
6.961 ± 0.175 |
573 ± 8 |
>1000 |
4.356 ± 0.288 |
N/D |
8e |
24.544 ± 0.275 |
938 ± 18 |
>1000 |
6.329 ± 1.713 |
N/D |
8f |
543.121 ± 23.144 |
>2000 |
2.574 ± 0.496 |
>1000 |
N/D |
8g |
95.245 ± 17.881 |
>2000 |
>1000 |
5.467 ± 0.463 |
N/D |
8h |
10.059 ± 0.204 |
410 ± 22 |
0.499 ± 0.164 |
33.418 ± 0.160 |
N/D |
8i |
5.623 ± 0.024 |
486 ± 20 |
>1000 |
>1000 |
N/D |
8j |
11.450 ± 0.185 |
619 ± 6 |
>1000 |
24.200 ± 1.268 |
N/D |
8k |
3.390 ± 0.006 |
341 ± 5 |
1.020 ± 0.062 |
0.617 ± 0.017 |
0.357 ± 0.015 |
8l |
3.583 ± 0.126 |
289 ± 0 |
0.250 ± 0.042 |
1.148 ± 0.125 |
0.203 ± 0.001 |
8m |
4.014 ± 0.032 |
338 ± 10 |
0.469 ± 0.042 |
2.493 ± 0.079 |
4.97 ± 0.052 |
8n |
3.891 ± 0.002 |
417 ± 21 |
0.400 ± 0.036 |
0.468 ± 0.028 |
4.815 ± 0.189 |
Vitamin C |
6.509 ± 0.153 |
837 ± 24 |
|
|
|
Acarbose |
|
|
0.032 ± 0.002 |
|
|
AG |
|
|
|
31.265 ± 0.942 |
|
Quercetin |
|
|
|
|
1.119 ± 0.088 |
DPPH is a widely used method to evaluate antioxidant capacities of natural and synthetic products. Although the main skeleton for all compounds is same, the slight difference in their inhibitory potential might be due to the different substitution patterns on benzene ring. As shown in Table 4, the number and position of hydroxy groups were relatively correlated with DPPH radical scavenging activity. Generally, the increase of hydroxy groups and the decrease of methoxy groups were positive to this activity, while 3,4-dihydrogen structure was adverse to radical scavenging capacity. Moreover, compounds with adjacent 7,8-dihydroxy groups or 3′,4′-dihydroxy groups had great capacity to scavenge DPPH radical, especially compounds 4i, 4o, 8i, 8k, 8l, 8m and 8n were better than positive reference substance vitamin C (IC50 = 6.509 ± 0.153 μg mL−1). These conclusions were similar to ref. 25.
In addition, it can be found from the data that almost all compounds with two adjacent hydroxy groups exhibit great hydroxyl radical scavenging activity. Especially, the IC50 values of compounds 8h, 8k, 8l, 8m and 8n were from 289 ± 0 to 417 ± 21 μg mL−1, which were 2 to 3 folds of the value of vitamin C (IC50 = 837 ± 24 μg mL−1). The IC50 values, 4f > 8c and 4g > 8d, indicated that neoflavonoid with 3,4-dihydrogen had weaker hydroxyl radical scavenging activity.
2.2.2. In vitro a-glucosidase inhibitory activity26. a-Glucosidases, enzymes anchored in the brush border of the small intestine, are responsible for catalyzing the hydrolysis of carbohydrates.27 Their inhibitors were useful for the treatment of type II diabetes mellitus.28 Though all the compounds were tested a-glucosidase inhibitory activity in vitro, as shown in Table 4, just six compounds (8f, 8h, 8k, 8l, 8m and 8n) presented moderate to excellent inhibitory activity for a-glucosidase. Notably, 8l (IC50 = 0.250 ± 0.042 μg mL−1) had relatively strong activity, which displayed little weaker capacity than acarbose (IC50 = 0.032 ± 0.002 μg mL−1). None of the neoflavonoid with 3,4-dihydrogen showed good inhibitory activity, indicating that double bond between C3 and C4 was necessary for inhibiting a-glucosidase. The results showed that neoflavonoid with adjacent 7,8-dihydroxy groups was weaker than that with 5,7-dihydroxy groups and 6,8-dihydroxy groups in meta-position. After compound 8h was converted to 8n via demethylation, its IC50 value decreased from 0.499 ± 0.164 μg mL−1 to 0.400 ± 0.036 μg mL−1. According to in vitro α-glucosidase inhibition test results, we decided to study in vivo hypoglycemic activity of compounds 8l, 8m and 8n.
2.2.3. In vitro inhibitory activity of AGEs (advanced glycation end products) formation29. Advanced glycation end products are a group of complex and heterogeneous compounds, which are implicated in a number of biochemical abnormalities associated with diabetes.30 Therefore, the discovery of AGEs inhibitors would be beneficial to the prevention and treatment of diabetic or other pathogenic complications. The results listed in Table 4 displayed that most of the target compounds presented strong inhibitory activity to AGEs, even better than amino guanidine (AG) (IC50 = 31.265 ± 0.942 μg mL−1). The IC50 value, 8n < 8k < 8l < 8b < 8c < 8m < 3.0 μg mL−1, which were over 10 folds compared with positive reference substance. When the neoflavonoids both have the 3′,4′-dihydroxy groups, those compounds with 6,8-dihydroxy groups were better than those with 7,8-dihydroxy groups and which were stronger than those with 5,7-dihydroxy groups. The AGEs inhibitory capacities of 8b > 4b, 8c > 4f, 8d > 4g, 8g > 4k and 8m > 4o, indicating that absence of 3,4-dihydrogen groups in neoflavonoids enhanced their inhibitory activity to the formation of AGEs. The series of IC50 values, 8k < 8l < 8c < 8d < 8e showed that neoflavonoids with 7,8-dihydroxy groups had a strong inhibitory activity of AGEs formation.
2.2.4. In vitro ALR2 inhibitory activity31. The enzyme aldose reductase (ALR2) is a member of the aldo–keto reductase superfamily. Diabetic complications including cataracts, retinopathy, accelerated atherosclerosis, and increased cardiovascular risk, which are life threatening risks for diabetic patients. The development and progression of chronic diabetic complications are confirmed to be quite related to the activation and/or over expression of ALR2 (ref. 32). Therefore, ALR2 inhibitors may play a critical role in preventing or treating these complications. The results listed in Table 4 displayed that the target compounds (8k–8n) resulted in high inhibitory activities on ALR2 (IC50 = 0.203 ± 0.001 to 4.97 ± 0.052 μg mL−1). The potency of ALR2 inhibition was 0.2–5.5 times of the positive reference compound quercetin. The target compound 8k and 8l presented strong inhibitory activity to ALR2, showing better inhibitory activity than quercetin (IC50 = 1.119 ± 0.088 μg mL−1). Target compounds 8k and 8l exerted higher ALR2 inhibition activities than the other serial compounds, indicating that neoflavonoid with adjacent 7,8-dihydroxy groups was more effective in inhibiting ALR2. The neoflavonoids with 3′,4′-dihydroxy groups showed weak effect on enhancing their inhibitory activity to ALR2.
2.2.5. Oral toxicity to mice. With reference to Lorke's method,33 Kunming mice were used as targets to estimate oral toxicity of each compound to mice. We select compounds 8l, 8m and 8n at concentrations of 10, 100, 1000 mg kg−1 to test their oral toxicity in the first phase, and 1600, 2900 and 5000 mg kg−1 at the second phase. Results showed that none of the tested compounds significantly affected mice viability. No death and no appetite-suppressant effect were detected in the tested mice in 14 days. Since no death or damage was observed throughout the experiment, the LD50 was higher than 5000 mg kg−1 for the three compounds assayed, indicating their innocuousness for mice.
2.2.6. Acute hypoglycemic assay. The antidiabetic activity was determined by using a standard method.26 As shown in Table 5, the target compounds 8l, 8m and 8n (10 mg kg−1, 30 mg kg−1 and 100 mg kg−1 of bw) caused decreases in blood glucose levels in STZ-diabetic mice compared with normal mice (Table 6). Especially, 8l (30 mg kg−1 of bw) caused significant decreases in blood glucose levels when compared with vehicle-treated groups (p < 0.05). In STZ-diabetic animals, the hypoglycemic effect of 8l (30 mg kg−1 of bw) was larger than 50% and persisted throughout the experiment (Table 5). The highest antihyperglycemic effect was observed at doses of 30 and 100 mg kg−1 at 7 h (∼77.14 and ∼70.56%, respectively) (Table 5). In diabetic animals, the highest antihyperglycemic effect of 8m and 8n was observed at doses of 30 mg kg−1 at 9 h (∼64.20 and ∼62.77%, respectively) (Table 5). The target compounds 8m and 8n showed similar antidiabetic activity throughout the experiment. Glibenclamide (10 mg kg−1 of bw), used as a positive control, showed maximum hypoglycemic effect at 9 h in STZ-induced diabetic mice (Table 5). However, the administration of the target compounds 8l, 8m and 8n (10 mg kg−1, 30 mg kg−1 and 100 mg kg−1 of bw) did not show significant decreases of blood glucose levels in normoglycemic mice (Table 6).
Table 5 Acute effect of compounds 8l, 8m and 8n on blood glucose levels in STZ-diabetics mice. Each value is the mean ± SEM for six mice in each groupa
Test samples |
Blood glucose concentration (mM) |
Dose (per os) mg kg−1 of bw |
0 h |
1.5 h |
3 h |
5 h |
7 h |
9 h |
*p < 0.05 significantly different ANOVA followed by Dunnett's t-test for comparison with respect to initial levels in each group. % variation of glycemia are in parentheses. |
Control (vehicle) |
— |
14.7 ± 4.3 |
14.8 ± 4.8 (0.68) |
12.3 ± 6.5 (−15.65) |
11.6 ± 8.4 (−21.09) |
9.2 ± 6.0 (−31.41) |
9.1 ± 4.8 (−31.41) |
Glibenclamide |
10 |
16.1 ± 4.1 |
7.2 ± 1.2* (−55.28) |
6.9 ± 1.9 (−57.14) |
7.1 ± 1.6 (−55.90) |
6.6 ± 1.4 (−59.63) |
4.7 ± 1.1* (−70.81) |
8l |
10 |
18.7 ± 7.3 |
11.1 ± 4.3 (−40.64) |
9.9 ± 5.6 (−47.06) |
8.9 ± 4.4 (−52.94) |
7.9 ± 3.7 (−57.75) |
7.7 ± 3.3 (−59.36) |
8l |
30 |
17.5 ± 7.5 |
8.3 ± 2.6* (−51.43) |
5.7 ± 1.1* (−66.86) |
6.1 ± 0.9 (−65.14) |
4.0 ± 1.4* (−77.14) |
5.4 ± 1.9 (−69.14) |
8l |
100 |
18.0 ± 5.3 |
9.9 ± 3.2 (−45.00) |
8.7 ± 2.9 (−51.67) |
6.2 ± 1.6 (−65.56) |
5.2 ± 1.5 (−70.56) |
6.5 ± 1.3 (−63.89) |
8m |
10 |
14.4 ± 5.5 |
9.6 ± 2.7 (−33.30) |
8.8 ± 3.1 (−38.90) |
8.1 ± 2.2 (−43.05) |
6.5 ± 1.8 (−54.16) |
5.2 ± 1.2* (−63.19) |
8m |
30 |
15.0 ± 2.1 |
9.1 ± 1.6 (−39.33) |
8.3 ± 3.0 (−44.53) |
8.5 ± 2.5 (−43.33) |
6.7 ± 2.1 (−55.20) |
5.4 ± 1.3 (−64.20) |
8m |
100 |
13.6 ± 3.1 |
9.0 ± 1.9 (−33.82) |
8.3 ± 1.4 (−38.97) |
7.7 ± 1.2 (−45.59) |
6.1 ± 0.7 (−55.15) |
4.9 ± 0.6* (−63.97) |
8n |
10 |
17.6 ± 5.1 |
11.7 ± 5.0 (−33.52) |
11.2 ± 3.3 (−36.36) |
10.0 ± 3.0 (−43.75) |
9.4 ± 2.8 (−46.59) |
7.4 ± 2.1 (−57.39) |
8n |
30 |
18.8 ± 7.4 |
11.6 ± 4.0 (−38.30) |
10.5 ± 3.7 (−44.15) |
9.1 ± 2.9 (−51.60) |
8.7 ± 4.3 (−53.72) |
7.0 ± 2.9 (−62.77) |
8n |
100 |
17.5 ± 5.7 |
11.1 ± 5.9 (−36.57) |
9.9 ± 6.0 (−43.43) |
8.3 ± 1.7 (−52.57) |
8.1 ± 1.8 (−53.71) |
6.9 ± 1.4 (−60.57) |
Table 6 Acute effect of compounds 8l, 8m and 8n on blood glucose levels in normal mice. Each value is the mean ± SEM for six mice in each groupa
Test samples |
Blood glucose concentration (mM) |
Dose (per os) mg kg−1 of bw |
0 h |
1.5 h |
3 h |
5 h |
7 h |
9 h |
*p < 0.05 significantly different ANOVA followed by Dunnett's t-test for comparison with respect to initial levels in each group. % variation of glycemia are in parentheses. |
Control (vehicle) |
— |
10.2 ± 1.0 |
8.1 ± 0.9 (−20.59) |
8.2 ± 1.0 (−18.82) |
8.4 ± 1.5 (−17.65) |
7.1 ± 1.1 (−29.41) |
6.4 ± 0.9 (−36.27) |
Glibenclamide |
10 |
9.0 ± 1.9 |
6.0 ± 1.0* (−33.33) |
5.5 ± 0.8* (−38.89) |
6.0 ± 1.1* (−33.33) |
5.4 ± 1.1 (−40.00) |
5.5 ± 1.0 (−38.89) |
8l |
10 |
10.3 ± 0.6 |
8.1 ± 1.0 (−21.36) |
6.5 ± 0.7* (−36.89) |
7.3 ± 1.2 (−29.13) |
6.2 ± 0.9 (−39.81) |
6.6 ± 0.8 (−35.92) |
8l |
30 |
10.5 ± 1.3 |
6.6 ± 0.8 (−37.14) |
7.3 ± 0.7 (−30.48) |
6.5 ± 1.6 (−38.10) |
5.8 ± 1.4 (−44.76) |
7.1 ± 1.5 (−32.38) |
8l |
100 |
10.4 ± 0.8 |
8.0 ± 0.9 (−24.04) |
7.0 ± 0.8 (−32.69) |
7.2 ± 0.8 (−30.77) |
6.4 ± 0.7 (−39.42) |
7.2 ± 0.7 (−31.73) |
8m |
10 |
10.7 ± 1.1 |
9.4 ± 1.2 (−12.15) |
8.7 ± 1.3 (−18.69) |
9.3 ± 1.0 (−13.08) |
8.6 ± 1.4 (−20.56) |
7.8 ± 1.2 (−27.10) |
8m |
30 |
10.8 ± 1.3 |
9.0 ± 1.4 (−16.67) |
8.7 ± 1.3 (−19.44) |
9.3 ± 1.0 (−13.89) |
8.6 ± 1.5 (−20.37) |
7.9 ± 1.2 (−27.78) |
8m |
100 |
10.6 ± 1.1 |
9.2 ± 1.1 (−13.40) |
8.3 ± 1.0 (−21.70) |
8.1 ± 1.7 (−22.64) |
7.7 ± 1.4 (−27.36) |
7.8 ± 1.2 (−26.42) |
8n |
10 |
10.7 ± 1.1 |
9.6 ± 0.7 (−10.28) |
9.3 ± 0.9 (−13.08) |
8.8 ± 0.7 (−17.76) |
8.8 ± 0.9 (−17.76) |
8.2 ± 1.2 (−23.36) |
8n |
30 |
10.7 ± 1.0 |
9.7 ± 1.1 (−9.35) |
8.9 ± 1.0 (−16.82) |
8.9 ± 0.9 (−16.82) |
8.7 ± 1.1 (−18.69) |
7.9 ± 0.9 (−26.17) |
8n |
100 |
10.6 ± 1.1 |
9.5 ± 1.0 (−10.38) |
8.7 ± 0.9 (−17.92) |
8.4 ± 1.1 (−20.75) |
8.6 ± 0.9 (−18.87) |
8.1 ± 1.0 (−24.53) |
2.2.7. Effects of daily treatment with compounds 8l and 8m in STZ-induced diabetic mice. The long term antihyperglycemic effect of the target compounds 8l and 8m was performed by using a classical chronic experiment with STZ-induced diabetic mice.34 The results demonstrated that daily oral administration of the target compound 8l (30 mg kg−1 of bw each time), once a day, for 16 days, induced pronounced antihyperglycemic effect in the STZ-diabetic mice (Fig. 3). The target compound 8m was less efficient in decreasing blood glucose levels in diabetic mice (Fig. 3). Compounds 8l (30 mg kg−1 of bw each time) restored blood glucose levels to near normal values at the end of the experiment [Fig. 3 (16 days)] and the effect was equipotent to that of the glibenclamide (10 mg kg−1 of bw each time) which was used as a positive control.18 The treatments with the target compounds 8l (30 mg kg−1 of bw each time) and 8m (30 mg kg−1 of bw each time) also prevented body weight loss in hyperglycemic mice (Fig. 4) and the effect was equipotent to that of glibenclamide (10 mg kg−1 of bw each time). However, 8l was more efficient than 8m in preventing body weight loss in hyperglycemic mice (Fig. 4).
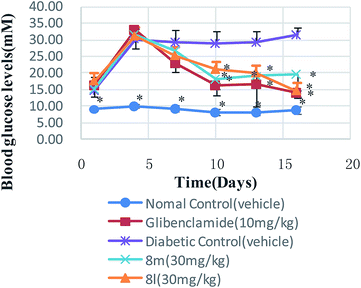 |
| Fig. 3 Long-term effect of the compound 8l and 8m on blood glucose levels in STZ-diabetic mice. Each value is the mean ± SEM for six mice in each group. *p < 0.05 significantly different ANOVA followed by Dunnett's t-test for comparison with the diabetic control group at same time. | |
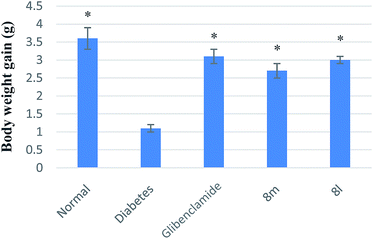 |
| Fig. 4 Body weight changes of daily treatment with compounds 8l and 8m in STZ-induced diabetic mice. Each value is the mean ± SEM for six mice in each group. *p < 0.05 significantly different ANOVA followed by Dunnett's t-test vs. the diabetic control group at same time. | |
2.2.8. Oral glucose tolerance test of compounds 8m and 8l on in STZ-induced diabetic mice. The oral glucose tolerance test was determined by using a standard method.35 Compared with vehicle treated group (p < 0.05) (Fig. 5), the target compounds 8l and 8m (30 mg kg−1 of bw) caused a significant decrease in the postprandial glycemia peak in both normal (data not shown) and STZ-diabetic mice. In all cases, the effect of the target compounds 8l and 8m (30 mg kg−1 of bw) were comparable to that of the glibenclamide (10 mg kg−1 of bw) used as a positive control. Compounds 8l and 8m decreased blood glucose levels to below initial values at the end of the experiment (Fig. 5).
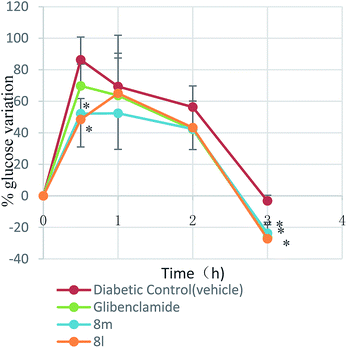 |
| Fig. 5 Oral glucose tolerance test of compounds 8m and 8l on in STZ-induced diabetic mice. Each value is the mean ± SEM for six mice in each group. * p < 0.05 significantly different ANOVA followed by Dunnett's t-test vs. the diabetic control group at same time. | |
3. Conclusions
Thirty neoflavonoid derivatives were synthesized by using sulfated montmorillonite K-10 as catalyst. Compared with traditional methods, this method is more environmental friendly, more sustainable and economical owning to the earth-abundant catalysts used, more convenient in isolation and purification processes due to less byproducts, and the yield is relatively higher than traditional methods. We then studied the pharmacological activity of the synthesized compounds. In the respect to its antioxidant activity, compounds 8k, 8l, 8m and 8n had strong hydroxyl radical scavenging activity. Compounds 8k and 8l showed significant effect on treatment of diabetic complications. Notably, 8l had relatively strong activity, which displayed little weaker capacity than acarbose. Oral toxicity tests indicated their innocuousness for mice. We selected compounds 8l, 8m and 8n to investigate their antidiabetic activity in vivo. The results showed the effect of the target compounds 8l and 8m were equipotent to that of the glibenclamide used as a positive control in vivo, in all cases. In conclusion, the target compound 8l offered a potential drug design concept for the development of therapeutic or preventive agents for diabetes and complications of diabetes.
4. Experimental
4.1. Synthesis
4.1.1. Materials and methods. Melting points were determined using a Thiele tube and were uncorrected. The FT-IR spectra were recorded using a Thermo-Nicolet Nexus 670 spectrometer with KBr pellets. The 1H NMR and 13C NMR spectra were recorded with a Bruker AM-600 spectrometer with TMS as the internal standard. Chemical shifts were reported at room temperature on a scale (ppm) with DMSO-d6 as the solvents and J values are given in hertz.Mass spectra were obtained with an Agilent Trap VL LC/MS spectrometer. The absorbance was recorded by a Hitachi U-3000 UV spectrophotometer. Column chromatography was performed on silica gel (200–300 mesh). Unless otherwise noted, all solvents and reagents were commercially available and used without further purification.
4.1.2. The treatment of montmorillonite K-10. The montmorillonite K-10 used in this study was supplied by Aladdin. This treatment method referred to literature36 with slight modification. To a 250 mL oven-dried three-neck round-bottom flask, 13 g montmorillonite K-10 was mixed with 130 mL 30% H2SO4 solution. This suspension was heated to 95 °C and keep for 4 hours under magnetic stirring. Then the acid treated clays were washed thoroughly with distilled water until the pH of the washings approached 7. The samples were dried in a vacuum oven at 40 °C to get 13.95 g sulfated montmorillonite K-10.
4.1.3. General procedures for the synthesis of 4-aryl-3,4-dihydrocoumarins 4a–4p. A mixture of 4-methoxyphenylacrylic acid 2a (5 mmol) and resorcinol 3a (5 mmol) in nitrobenzene (10 mL) was heated to 100 °C. 2 g of sulfated montmorillonite K-10 was added to the mixture after all reagents were dissolved and the mixture stirred at 100 °C for 3–12 h. The reaction process was monitored by TLC (CH2Cl2–CH3OH, 10
:
1). The suspension was directly filtered and a suitable amount of petroleum ether was added to the filtrate. Then cooled the mixture and stewing or stirred under low temperature for a certain length of time to promote crystallization of the product. The crude product was recrystallized from EtOAc–PE to afford 7-hydroxy-4-(4-methoxyphenyl)-3,4-dihydrocoumarin 4a. Compounds 4b–4p were obtained using the same procedures.
4.1.3.1. 7-Hydroxy-4-(4-methoxyphenyl)-3,4-dihydrocoumarin (4a). White solid, yield: 79%, mp 177.2–178.3 °C, IR (KBr, ν, cm−1): 3332 (OH); 1736 (C
O); 3040, 1614, 1583, 1507, 1450, 1105, 1028, 984, 832 (Ar); 1236, 1146 (C–O); 2800 (–OCH3). 1H NMR (600 MHz, DMSO-d6) δ (ppm): 3.03 (m, 2H), 3.73 (s, 3H), 4.32 (t, J = 6.0 Hz, 1H), 6.53 (dd, J = 2.4 and 8.4 Hz, 1H), 6.55 (d, J = 2.4 Hz, 1H), 6.84 (d, J = 8.4 Hz, 1H), 6.90 and 7.06 (d, J = 1.8 Hz, 4H), 9.74 (s, 1H). 13C NMR (151 MHz, DMSO-d6) δ (ppm): 36.76, 37.80, 54.95, 103.17, 111.51, 114.03, 116.47, 128.23, 128.81, 133.53, 151.81, 157.43, 158.10 and 167.73. MS: m/z (%): 207.7 [M + 1]+, 162.6.
4.1.3.2. 7-Hydroxy-4-(3,4-dimethoxyphenyl)-3,4-dihydrocoumarin (4b). White solid, yield 53%, mp 144.8–146.1 °C. IR (KBr, ν, cm−1): 3433 (OH); 1767 (C
O); 3030, 1628, 1597, 1514, 1447, 1101, 1025, 848, 810 (Ar); 1267, 1142 (C–O); 2790 (–OCH3). 1H NMR (600 MHz, DMSO-d6) δ (ppm): 3.05 (m, 2H), 3.70 and 3.71 (s, 6H), 4.29 (t, J = 6.0 Hz, 1H), 6.52 (s, 1H), 6.53–6.56 (m, 2H), 6.83–6.89 (m, 3H), 9.74 (s, 1H). 13C NMR (151 MHz, DMSO-d6) δ (ppm): 37.27, 38.81, 55.93, 55.95, 103.72, 111.76, 112.09, 112.34, 119.54, 129.40, 149.37, 158.00 and 168.37. MS: m/z (%): 300.8 [M + 1]+, 258.7, 190.6, 162.7.
4.1.3.3. 7-Hydroxy-4-(3-methoxy-4-hydroxyphenyl)-3,4-dihydrocoumarin (4c). White solid, yield 55%, mp 194.3–196.3 °C. IR (KBr, ν, cm−1): 3415 (OH); 1724 (C
O); 2994, 1625, 1602, 1518, 1454, 1112, 1028, 841, 809 (Ar); 1259, 1152 (C–O); 2929 (–OCH3). 1H NMR (600 MHz, DMSO-d6) δ (ppm): 3.03 (m, 2H), 3.71 (s, 3H), 4.24 (t, J = 6.0 Hz, 1H), 6.44 (d, J = 7.8 Hz, 1H), 6.51 (s, 1H), 6.54 (d, J = 8.4 Hz, 1H), 6.70 (d, J = 7.8 Hz, 1H), 6.78 (s, 1H), 6.84 (d, J = 7.8 Hz, 1H), 8.93 and 9.72 (s, 2H). 13C NMR (151 MHz, DMSO-d6) δ (ppm): 37.35, 38.81, 56.05, 103.69, 112.05, 115.93, 117.23, 119.84, 129.42, 132.94, 146.01, 148.20, 152.37, 157.95 and 168.45. MS: m/z (%): 287.1 [M + 1]+, 162.8.
4.1.3.4. 6-Hydroxy-4-(4-methoxyphenyl)-3,4-dihydrocoumarin (4d). White solid, yield 52%, mp 167.7–169.7 °C. IR (KBr, ν, cm−1): 3332, (OH); 1728 (C
O); 3000, 1613, 1600, 1502, 1450, 1105, 1028, 831, 807 (Ar); 1236, 1146 (C–O), 2829 (–OCH3). 1H NMR (600 MHz, DMSO-d6) δ (ppm): 3.01 (m, 2H), 3.73 (s, 3H), 4.34 (t, J = 6.3 Hz, 1H), 6.37 (s, 1H), 6.68 (d, J = 8.6 Hz, 1H), 6.92 (d, J = 8.0 Hz, 2H), 6.96 (d, J = 8.6 Hz, 1H), 7.1 (d, J = 8.6 Hz, 2H), 9.34 (s, 1H). 13C NMR (151 MHz, DMSO-d6) δ (ppm): 36.8, 39.99, 55.55, 114.68, 115.23, 117.76, 128.05, 129.05, 133.34, 144.43, 154.37, 158.81 and 168.53. MS: m/z (%): 207.7 [M + 1]+, 162.6.
4.1.3.5. 6-Hydroxy-4-(3,4-dimethoxyphenyl)-3,4-dihydrocoumarin (4e). White solid, yield 53%, mp 166.7–168.5 °C, IR (KBr, ν, cm−1): 3259 (OH); 1709 (C
O); 3071, 1593, 1522, 1487, 1451, 1106, 1022, 856, 817 (Ar); 1267, 1142 (C–O); 2790 (–OCH3). 1H NMR (600 MHz, DMSO-d6) δ (ppm): 3.04 (m, 2H), 3.72 and 3.73 (s, 6H), 4.33 (t, J = 6.3 Hz, 1H), 6.37 (d, J = 2.6 Hz, 1H), 6.62 (dd, J = 1.6 and 8.2 Hz, 1H), 6.68 (dd, J = 2.7 and 8.7 Hz, 1H), 6.88 (d, J = 1.5 Hz, 1H), 6.91 (d, J = 8.3 Hz, 1H), 6.96 (d, J = 8.7 Hz, 1H), 9.32 (s, 1H). 13C NMR (151 MHz, DMSO-d6) δ (ppm): 36.70, 39.99, 55.90, 111.99, 112.43, 114.67, 115.22, 117.70, 119.88, 128.06, 133.72, 144.42, 148.42, 149.41, 154.35 and 168.59. MS: m/z (%): 301.0 [M + 1]+, 259.0, 162.9.
4.1.3.6. 7,8-Dihydroxy-4-(4-methoxyphenyl)-3,4-dihydrocoumarin (4f). White solid, yield 79%, mp 168.9–171.1 °C. IR (KBr, ν, cm−1): 3330 (OH); 1738 (C
O); 3050, 1634, 1612, 1512, 1458, 1109, 1030, 1002, 830, 802 (Ar); 1236, 1154 (C–O); 2805 (–OCH3). 1H NMR (600 MHz, DMSO-d6) δ (ppm): 3.01 (m, 2H), 3.72 (s, 3H), 4.28 (t, J = 6.8 Hz, 1H), 6.28 (d, J = 8.0 Hz, 1H), 6.53 (d, J = 8.0 Hz, 1H), 6.88 and 7.06 (d, J = 8.0 Hz, 4H), 8.91 and 9.29 (s, 2H). 13C NMR (151 MHz, DMSO-d6) δ (ppm): 37.35, 38.93, 55.53, 111.7, 114.9, 118.5, 119.1, 128.83, 133.74, 134.11, 140.99, 146.31, 158.66 and 168.25. MS: m/z (%): 286.8 [M + 1]+, 244.6, 178.6, 160.6.
4.1.3.7. 7,8-Dihydroxy-4-(3,4-dimethoxyphenyl)-3,4-dihydrocoumarin (4g). White solid, yield 83%, mp 173.7–174.6 °C. IR (KBr, ν, cm−1): 3423 (OH); 1754 (C
O); 2935, 1610, 1515, 1470, 1413, 1025, 807 (Ar); 1240, 1137 (C–O); 2829 (–OCH3). 1H NMR (600 MHz, DMSO-d6) δ (ppm): 3.04 (m, 2H), 3.70 and 3.71 (s, 6H), 4.27 (t, J = 6.3 Hz, 1H), 6.28, 6.53, 6.56 and 6.87 (d, J = 8.2 Hz, 4H), 6.84 (s, 1H), 8.90 and 9.28 (s, 2H). 13C NMR (151 MHz, DMSO-d6) δ (ppm): 37.25, 39.98, 55.96, 111.48, 111.87, 112.32, 117.61, 118.26, 119.62, 133.68, 134.56, 140.99, 146.28, 148.26, 149.32 and 168.34. MS: m/z (%): 316.8 [M + 1]+, 178.5.
4.1.3.8. 7,8-Dihydroxy-4-(3-methoxy-4-hydroxyphenyl)-3,4-dihydrocoumarin (4h). White solid, yield 53%, mp 171.7–173.1 °C. IR (KBr, ν, cm−1): 3373 (OH); 1743 (C
O); 3018, 1612, 1517, 1464, 1033, 812, 693 (Ar); 1274, 1067 (C–O); 2810 (–OCH3). 1H NMR (600 MHz, DMSO-d6) δ (ppm): 3.01 (m, 2H), 3.71 (s, 3H), 4.22 (t, J = 6.2 Hz, 1H), 6.29 (d, J = 8.3 Hz, 1H), 6.45, 6.52 and 6.69 (d, J = 8.1 Hz, 3H), 6.78 (d, J = 1.5 Hz, 1H), 8.88, 8.90 and 9.26 (s, 3H). 13C NMR (151 MHz, DMSO-d6) δ (ppm): 37.34, 39.98, 56.08, 111.45, 112.19, 115.88, 117.63, 118.45, 119.90, 132.95, 133.65, 140.96, 145.97, 146.21, 148.14 and 168.41. MS: m/z (%): 303.1 [M + 1]+, 283.7, 260.7, 178.7, 150.6.
4.1.3.9. 7,8-Dihydroxy-4-(3-hydroxy-4-methoxyphenyl)-3,4-dihydrocoumarin (4i). White solid, yield 66%, mp 185.0–187.1 °C. IR (KBr, ν, cm−1): 3499, 3420 (OH); 1752 (C
O); 3010, 1610, 1591, 1518, 1469, 1030, 786, 698 (Ar); 1277, 1068 (C–O); 2810 (–OCH3). 1H NMR (600 MHz, DMSO-d6) δ (ppm): 2.97 (m, 2H), 3.72 (s, 3H), 4.19 (t, J = 5.4 Hz, 1H), 6.32 (d, J = 7.8 Hz, 1H), 6.51, 6.53 and 6.54 (d, J = 6.0 Hz, 3H), 6.84 (d, J = 7.8 Hz, 1H), 8.90, 8.95 and 9.29 (s, 3H). 13C NMR (151 MHz, DMSO-d6) δ (ppm): 37.44, 39.11, 56.10, 111.47, 112.85, 114.92, 117.73, 118.25, 133.68, 134.92, 140.96, 146.23, 147.03 and 168.26. MS: m/z (%): 302.9 [M + 1]+, 283.7, 260.7, 178.6, 150.6.
4.1.3.10. 5,7-Dihydroxy-4-(4-methoxyphenyl)-3,4-dihydrocoumarin (4j). White solid, yield 72%, mp 148.4–149.9 °C. IR (KBr, ν, cm−1): 3510 (OH); 1759 (C
O); 3050, 1635, 1611, 1513, 1457, 1134, 1061, 1032, 826 (Ar); 1231, 1179 (C–O); 2805 (–OCH3). 1H NMR (600 MHz, DMSO-d6) δ (ppm): 2.97 (m, 2H), 3.69 (s, 3H), 4.38 (d, J = 6.5 Hz, 1H), 6.02 (d, J = 2.1, 1H), 6.17 (d, J = 2.2 Hz, 1H), 6.83 and 6.98 (d, J = 8.6 Hz, 4H), 9.55 and 9.73 (s, 1H). 13C NMR (151 MHz, DMSO-d6) δ (ppm): 33.40, 37.75, 61.07, 94.51, 95.15, 99.18, 103.88, 114.44, 128.13, 134.74, 153.38, 155.79, 158.28 and 168.41. MS: m/z (%): 286.9 [M + 1]+, 178.7, 110.8.
4.1.3.11. 5,7-Dihydroxy-4-(3,4-dimethoxyphenyl)-3,4-dihydrocoumarin (4k). White solid, yield 62%, mp 185.8–187.7 °C. IR (KBr, ν, cm−1): 3402, (OH); 1778 (C
O); 1632, 1610, 1500, 1460, 1012, 825 (Ar); 1237, 1148 (C–O). 1H NMR (600 MHz, DMSO-d6) δ (ppm): 3.02 (m, 2H), 3.68 and 3.70 (s, 6H), 4.37 (d, J = 6.6 Hz, 1H), 6.01 (s, 1H), 6.17 (s, 1H), 6.40 (d, J = 8.4 Hz, 1H), 6.81 (d, J = 9.6 Hz, 2H), 9.56 and 9.74 (s, 2H). 13C NMR (151 MHz, DMSO-d6) δ (ppm): 33.76, 37.74, 55.87, 55.95, 95.11, 99.17, 103.74, 111.49, 112.22, 118.39, 135.29, 148.08, 153.45, 155.81, 158.27 and 168.47. MS: m/z (%): 316.9 [M + 1]+, 178.7, 164.7, 110.8.
4.1.3.12. 5,7-Dihydroxy-4-(3-methoxy-4-hydroxyphenyl)-3,4-dihydrocoumarin (4l). White solid, yield 53%, mp 173.0–174.0 °C. IR (KBr, ν, cm−1): 3404, (OH); 1767 (C
O); 3030, 1628, 1597, 1514, 1447, 1101, 1025, 848, 810 (Ar); 1267, 1142 (C–O), 2790 (–OCH3). 1H NMR (600 MHz, DMSO-d6) δ (ppm): 3.01 (m, 2H), 3.70 (s, 3H), 4.33 (d, J = 6.6 Hz, 1H), 6.00 (s, 1H), 6.16 (s, 1H), 6.30 (d, J = 7.8 Hz, 1H), 6.62 (d, J = 8.4 Hz, 1H), 6.75 (s, 1H), 8.91, 9.54 and 9.72 (s, 3H). 13C NMR (151 MHz, DMSO-d6) δ (ppm): 33.73, 37.86, 56.00, 95.09, 99.16, 103.95, 111.78, 115.72, 118.78, 133.68, 145.78, 148.05, 153.42, 155.78, 158.19 and 168.55. MS: m/z (%): 303.1 [M + 1]+, 261.1, 179.1, 151.1, 111.2.
4.1.3.13. 5,7-Dihydroxy-4-(3-hydroxy-4-methoxyphenyl)-3,4-dihydrocoumarin (4m). White solid, yield 64%, mp 228.3–230.3 °C. IR (KBr, ν, cm−1): 3332 (OH); 1751 (C
O); 3065, 1638, 1589, 1514, 1463, 1137, 1026, 841, 797 (Ar); 1285, 1166 (C–O), 2840 (–OCH3). 1H NMR (600 MHz, DMSO-d6) δ (ppm): 2.98 (m, 2H), 3.70 (s, 3H), 4.29 (d, J = 6.4 Hz, 1H), 6.01 (d, J = 2.2 Hz, 1H), 6.16 (d, J = 2.2 Hz, 1H), 6.55–6.39 (m, 2H), 6.80 (d, J = 8.3 Hz, 1H), 8.93 (s, 1H), 9.57 (s, 1H), 9.74 (s, 1H). 13C NMR (151 MHz, DMSO-d6) δ (ppm): 33.55, 37.84, 56.07, 95.08, 99.11, 103.92, 112.82, 114.49, 117.61, 135.46, 146.82, 146.85, 153.37, 155.80, 158.20 and 168.47. MS: m/z (%): 303.0 [M + 1]+, 260.8, 178.7, 150.7, 110.8.
4.1.3.14. 5,7-Dihydroxy-4-(4-hydroxyphenyl)-3,4-dihydrocoumarin (4n). White solid, yield 62%, mp 269.8–270.6 °C. IR (KBr, ν, cm−1): 3334 (OH); 1724 (C
O); 1616, 1513, 1464, 1413, 1132, 1062, 829 (Ar); 1288, 1190 (C–O). 1H NMR (600 MHz, DMSO-d6) δ (ppm): 2.76 (dd, J = 15.8, 1.6 Hz, 1H), 3.13 (dd, J = 15.8, 6.9 Hz, 1H),4.32 (m, 1H), 6.01 (d, J = 2.2 Hz, 1H), 6.16 (d, J = 2.3 Hz, 1H), 6.65 (m, 2H), 6.85 (d, J = 8.5 Hz, 2H), 9.30 (s, 1H), 9.57 (s, 1H), 9.75 (s, 1H). 13C NMR (151 MHz, DMSO-d6) δ (ppm): 33.37, 37.87, 95.09, 98.63, 104.03, 116.19, 122.46, 128.15, 132.95, 153.36, 155.75, 156.51, 158.18, 159.37, and 168.53. MS: m/z (%): 272.7 [M + 1]+, 178.5.
4.1.3.15. 5,7-Dihydroxy-4-(3,4-dihydroxyphenyl)-3,4-dihydrocoumarin (4o). White solid, yield 60%, mp 214.7–216.4 °C. IR (KBr, ν, cm−1): 3272 (OH); 1751 (C
O); 1620, 1521, 1477, 1420, 1190, 1055, 799 (Ar); 1294, 1163 (C–O). 1H NMR (600 MHz, DMSO-d6) δ (ppm): 2.72 (dd, J = 15.7, 1.6 Hz, 1H), 3.12 (m, 1H), 4.25 (m, 1H), 6.00 (d, J = 2.2 Hz, 1H), 6.16 (d, J = 2.3 Hz, 1H), 6.34 (dd, J = 8.2, 2.1 Hz, 1H), 6.42 (d, J = 2.1 Hz, 1H), 6.61 (d, J = 8.1 Hz, 1H), 8.75 (s, 1H), 8.84 (s, 1H), 9.55 (s, 1H), 9.72 (s, 1H). 13C NMR (151 MHz, DMSO-d6) δ (ppm): 33.56, 39.48, 94.53, 99.17, 104.17, 116.04, 117.85, 133.75, 144.42, 145.54, 153.36, 155.79, 158.13, 159.37, and 168.50. MS: m/z (%): 288.9 [M + 1]+, 178.7.
4.1.3.16. 6,8-Dihydroxy-4-(3,4-dimethoxyphenyl)-3,4-dihydrocoumarin (4p). White solid, yield 70%, mp 192.8–193.8 °C. IR (KBr, ν, cm−1): 3385 (OH); 1743 (C
O); 3058, 1638, 1608, 1518, 1450, 1311, 1106, 1024, 867 (Ar); 1267, 1171 (C–O), 2821 (–OCH3). 1H NMR (500 MHz, DMSO-d6) δ (ppm): 2.99 (m, 2H), 3.72 (d, J = 4.9 Hz, 6H), 4.21 (t, J = 6.7 Hz, 1H), 6.34 (s, 1H), 6.52 (s, 1H), 6.59 (dd, J = 8.2, 1.9 Hz, 1H), 6.84 (d, J = 1.9 Hz, 1H), 6.89 (d, J = 8.3 Hz, 1H), 8.86 (s, 1H), 9.19 (s, 1H). 13C NMR (126 MHz, DMSO-d6) δ (ppm): 37.32, 39.07, 55.98, 55.99, 104.42, 111.92, 112.42, 114.65, 116.68, 119.79, 134.53, 142.44, 144.15, 145.57, 148.32, 149.38 and 168.69. MS: m/z (%): 317.0 [M + 1]+, 178.8.
4.1.4. General procedures for the synthesis of 4-arylcoumarins 8a–8h. A mixture of 4-methoxybenzaldehyde 1a (200 mmol), malonic acid (240 mmol) and piperidine (2 mL) in pyridine (50 mL) was heated to reflux for 8 h at 90 °C. After the reaction is completed, hydrochloric acid solution (150 mL, 3 mol L−1) was added. Filtrate to obtain a white crude product 24 h later. The crude product was recrystallized from absolute ethanol to afford 4-methoxyphenylacrylic acid 2a.SOCl2 (60 mmol) was added to a solution of 2a (40 mmol) in anhydrous methanol (100 mL). The solution was then mechanically stirred and refluxed for 2 h. Stop the reaction and cool for 10 minutes to concentrate, then ice water (50 mL) was added and followed by removal of the solvent in vacuo to obtain the crude product 4-methoxyphenyl ethyl acrylate 5a.
To a solution of 4-methoxyphenyl ethyl acrylate 5a in CH2Cl2 (80 mL) was introduced Br2 (2.1 mL, 41 mmol) dropwise into the solution at 0 °C for 20 min until the reaction solution does not fade. Continue stirring for one hour, the reaction solution was washed with 10% aqueous solution of sodium bisulfite to remove excess bromine and washed again with water. The organic layer was concentrated in vacuo and afforded the crude product 2,3-dibromo-4-methoxyphenylpropionate 6a. A mixture of 6a and KOH (6.8 g, 121 mmol) in ethyl alcohol (100 mL) was heated to reflux for 10 h. After the reaction stops, add 20 mL water to dissolve the salt, with concentrated hydrochloric acid to adjust the pH to strong acid, with dichloromethane to extract the reaction solution, then concentrating in vacuo to obtain the crude product. The crude product was subjected to column chromatography (silica, EtOAc–PE, 1
:
2) to obtain purified compound 4-methoxybenzene propionic acid 7a (4.6 g, in 65% yield).
To a solution of 4-methoxyphenylpropionic acid 7a (1.76 g, 10 mmol) and catechol (1.2 g, 11 mmol), in nitrobenzene (20 mL, anhyd) and the solution was stirred and heated to 100 °C. Then, 4 g acidified montmorillonite K-10 was added and the reaction was monitored by TLC (CH2Cl2
:
CH3OH, 10
:
1) and the reaction was completed after 5 hours. Hot filter, add 30 mL of petroleum ether in the filtrate and place the natural crystallization overnight. The solvent was removed by vacuum suction filtration, washed with 20 mL of petroleum ether and dried to obtain pure product 4-(4′-methoxyphenyl)-7-hydroxycoumarin 8a (2.0 g, in 75% yield). Compounds 8b–8h were obtained by using the same procedures.
4.1.4.1. 7-Hydroxy-4-(4-methoxyphenyl)-coumarin (8a). Pink solid, yield: 75%, mp 265.1–266.3 °C, IR (KBr, ν, cm−1): 3206 (OH); 1702 (C
O); 3006, 1617, 1608, 1511, 1443, 1122, 1036, 1002, 828 (Ar); 1251, 1184 (C–O); 2841 (–OCH3). 1H NMR (600 MHz, DMSO-d6) δ (ppm): 3.84 (s, 3H), 6.11 (s, 1H), 6.79 (d, J = 8.7 Hz, 2H), 7.11 (d, J = 7.6 Hz, 2H), 7.36 (d, J = 8.1 Hz, 1H), 7.48 (d, J = 7.6 Hz, 2H), 10.65 (s, 1H). 13C NMR (151 MHz, DMSO-d6) δ (ppm): 39.57, 55.81, 60.23, 103.16, 110.26, 111.25, 113.58, 114.75, 127.78, 128.66, 130.47, 155.57, 156.07, 160.71, 160.82 and 161.78. MS: m/z (%): 268.9 [M + 1]+, 162.7.
4.1.4.2. 7-Hydroxy-4-(3,4-dimethoxyphenyl)-coumarin (8b). Pink solid, yield: 64%, mp 239.4–240.5 °C, IR (KBr, ν, cm−1): 3355 (OH); 1732 (C
O); 3069, 1622, 1558, 1519, 1450, 1139, 1014, 860, 820 (Ar); 1253, 1178 (C–O); 2843 (–OCH3). 1H NMR (600 MHz, DMSO-d6) δ (ppm): 3.83 (d, J = 12.1 Hz, 6H), 6.16 (s, 1H), 6.79 (dd, J = 6.3, 2.3 Hz, 2H), 7.09 (ddd, J = 11.2, 10.1, 5.1 Hz, 3H), 7.41–7.47 (m, 1H), 10.62 (s, 1H). 13C NMR (151 MHz, DMSO-d6) δ (ppm): 39.57, 49.07, 56.09, 56.09, 103.12, 110.32, 111.26, 112.18, 112.56, 113.60, 121.62, 127.94, 128.82, 149.28, 150.39, 155.75, 156.08, 160.75 and 161.75. MS: m/z (%): 299.0 [M + 1]+, 282.9, 256.8, 164.7.
4.1.4.3. 7,8-Dihydroxy-4-(4-methoxyphenyl)-coumarin (8c). Red solid, yield: 67%, mp 108–110 °C, IR (KBr, ν, cm−1): 3480 (OH); 1685 (C
O); 3165, 1601, 1560, 1508, 1442, 1177, 1017, 856, 819 (Ar); 1297, 1177 (C–O); 2841 (–OCH3). 1H NMR (600 MHz, DMSO-d6) δ (ppm): 3.84 (s, 3H), 6.09 (s, 1H), 6.82 (dd, J = 29.0, 8.7 Hz, 2H), 7.10 (d, J = 8.7 Hz, 2H), 7.47 (d, J = 8.6 Hz, 2H), 9.42 (s, 1H), 10.16 (s, 1H). 13C NMR (151 MHz, DMSO-d6) δ (ppm): 39.56, 39.70, 55.79, 110.16, 112.06, 112.72, 114.65, 117.83, 128.07, 130.48, 133.08, 144.42, 150.00, 156.11, 160.67 and 161.73. MS: m/z (%): 284.8 [M + 1]+, 256.7, 152.6.
4.1.4.4. 7,8-Dihydroxy-4-(3,4-dimethoxyphenyl)-coumarin (8d). Pink solid, yield: 73%, mp 126.1–127.3 °C, IR (KBr, ν, cm−1): 3322 (OH); 1700 (C
O); 2954, 1604, 1519, 1505, 1440, 1020, 817 (Ar); 1257, 1142 (C–O); 2834 (–OCH3). 1H NMR (600 MHz, DMSO-d6) δ (ppm): 3.82 (s, 3H), 3.84 (s, 3H), 6.15 (s, 1H), 6.80 (d, J = 8.7 Hz, 1H), 6.93 (d, J = 8.7 Hz, 1H), 7.06 (d, J = 8.2 Hz, 1H), 7.10 (dd, J = 8.8, 4.8 Hz, 2H), 9.38 (s, 1H),10.17 (s, 1H). 13C NMR (151 MHz, DMSO-d6) δ (ppm): 39.56, 56.08, 110.23, 112.05, 112.11, 112.61, 112.73, 117.97, 121.64, 128.23, 133.06, 144.45, 149.20, 149.97, 150.31, 156.27 and 160.72. MS: m/z (%): 314.9 [M + 1]+, 299.8, 164.6.
4.1.4.5. 7,8-Dihydroxy-4-(3,4,5-trimethoxyphenyl)-coumarin (8e). Pink solid, yield: 70%, mp 281.3–281.5 °C, IR (KBr, ν, cm−1): 3266 (OH); 1702 (C
O); 2944, 1624, 1519, 1504, 1446, 1037, 867 (Ar); 1255, 1171 (C–O); 2847 (–OCH3). 1H NMR (600 MHz, DMSO-d6) δ (ppm): 3.74 (s, 3H), 3.83 (s, 6H), 6.21 (s, 1H), 6.81 (d, J = 10.3 Hz, 3H), 6.95 (d, J = 8.7 Hz, 1H), 9.43 (s, 1H), 10.24 (s, 1H). 13C NMR (151 MHz, DMSO-d6) δ (ppm): 39.49, 49.07, 56.49, 60.54, 106.42, 110.54, 111.91, 112.83, 118.02, 131.35, 133.03, 138.60, 144.34, 150.03, 153.39, 156.41 and 160.68. MS: m/z (%): 345.0 [M + 1]+, 318.9, 299.0.
4.1.4.6. 5,7-Dihydroxy-4-(4-methoxyphenyl)-coumarin (8f). Red solid, yield: 65%, mp 256.6–258.2 °C, IR (KBr, ν, cm−1): 3208 (OH); 1696 (C
O); 1635, 1514, 1468, 1023, 825 (Ar); 1267, 1182 (C–O); 2868 (–OCH3). 1H NMR (600 MHz, DMSO-d6) δ (ppm): 3.79 (s, 3H), 5.74 (s, 1H), 6.22 (dd, J = 48.3, 2.3 Hz, 2H), 6.93 (m, 2H), 7.29 (m, 2H), 10.15 (s, 1H), 10.42 (s, 1H). 13C NMR (151 MHz, DMSO-d6) δ (ppm): 49.07, 55.57, 95.10, 99.61, 101.11, 110.45, 111.64, 113.10, 129.52, 130.96, 140.06, 156.29, 157.60, 159.64, 160.46 and 162.03. MS: m/z (%): 285.0 [M + 1]+, 252.8, 152.6.
4.1.4.7. 5,7-Dihydroxy-4-(3,4-methoxyphenyl)-coumarin (8g). Red solid, yield: 70%, mp 183.6–184.8 °C, IR (KBr, ν, cm−1): 3468 (OH); 1675 (C
O); 3051, 1595, 1516, 1458, 1079, 840 (Ar); 1246, 1171 (C–O); 2841 (–OCH3). 1H NMR (600 MHz, DMSO-d6) δ (ppm): 3.75 (s, 3H), 3.79 (s, 3H), 5.79 (s, 1H),6.19 (d, J = 2.3 Hz, 1H), 6.26 (d, J = 2.3 Hz, 1H), 6.88 (dd, J = 8.2, 2.0 Hz, 1H), 6.95 (dd, J = 5.1, 3.1 Hz, 2H), 10.14 (s, 1H), 10.42 (s, 1H). 13C NMR (151 MHz, DMSO-d6) δ (ppm): 39.5, 55.95, 95.09, 99.69, 101.15, 110.49, 111.01, 112.40, 120.45, 132.32, 147.94, 149.18, 156.36, 157.30, 157.61, 160.49 and 162.02. MS: m/z (%): 315.0 [M + 1]+, 164.7.
4.1.4.8. 6,8-Dihydroxy-4-(3,4-dimethoxyphenyl)-coumarin (8h). Red solid, yield: 72%, mp 311.6–313.7 °C. IR (KBr, ν, cm−1): 3230 (OH); 1669 (C
O); 3003, 1598, 1516, 1464, 1023, 847 (Ar); 1262, 1170 (C–O); 2831 (–OCH3). 1H NMR (600 MHz, DMSO-d6) δ (ppm): 3.84 (d, J = 7.3 Hz, 6H), 6.14 (s, 1H), 6.82 (s, 1H), 6.97 (s, 1H), 7.10 (m, 3H), 9.48 (s, 1H), 10.25 (s, 1H). 13C NMR (151 MHz, DMSO-d6) δ (ppm): 39.50, 56.09, 103.64, 110.41, 110.68, 111.38, 112.11, 112.45, 121.47, 128.23, 143.27, 148.98, 149.16, 150.24, 150.84, 155.57 and 162.02. MS: m/z (%): 315.0 [M + 1]+, 164.7.
4.1.5. General procedures for the synthesis of 4-arylcoumarins 8i–8n. To a solution of iodine (9.6 g, 37.6 mmol) and aluminum (1.0 g, 37 mmol) in acetonitrile (150 mL) and the solution was stirred and heated to reflux for 3 h. Then, cooled to room temperature and compound 8a (0.54 g, 2 mmol) was added. Continue to reflux and the reaction was monitored by TLC (CH2Cl2
:
CH3OH, 6
:
1) until the starting material disappeared completely. The reaction solution was concentrated, and 5% sodium bisulfite was added to remove excess iodine. After the hydrochloric acid was acidified, the mixture was extracted with ethyl acetate. The organic layer was concentrated to obtain 4-(4′-hydroxyphenyl)-7-hydroxycoumarin 8i (0.46 g, in 92% yield). Compounds 8j–8n were obtained by using the same procedures.
4.1.5.1. 7-Hydroxy-4-(3,4-dihydroxyphenyl)-coumarin (8i). Yellow solid, yield: 92%, mp 265.5–267.9 °C, IR (KBr, ν, cm−1): 3430 (OH); 1667 (C
O); 1627, 1542, 1513, 1444, 1116, 1007, 839 (Ar); 1263, 1194 (C–O). 1H NMR (600 MHz, DMSO-d6) δ (ppm): 6.04 (s, 1H), 6.81 (dt, J = 10.3, 1.9 Hz, 3H), 6.90 (dd, J = 5.1, 2.9 Hz, 2H), 7.46 (d, J = 8.5 Hz, 1H), 9.37 (s, 1H), 9.52 (s, 1H), 10.66 (s, 1H). 13C NMR (151 MHz, DMSO-d6) δ (ppm): 49.07, 103.10, 109.61, 111.24, 113.47, 116.31, 120.45, 126.55, 128.87, 145.89, 147.55, 156.06, 160.82 and 161.67. MS: m/z (%): 270.9 [M + 1]+, 242.2.
4.1.5.2. 7,8-Dihydroxy-4-(4-hydroxyphenyl)-coumarin (8j). Yellow solid, yield: 93%, mp 272.0–273.1 °C, IR (KBr, ν, cm−1): 3352 (OH); 1685 (C
O); 2959, 1610, 1586, 1514, 1454, 1111, 1042, 838 (Ar); 1229, 1066 (C–O). 1H NMR (600 MHz, DMSO-d6) δ (ppm): 6.06 (s, 1H), 6.80 (d, J = 8.8 Hz, 1H), 6.90 (dd, J = 18.2, 8.6 Hz, 3H), 7.36 (d, J = 8.5 Hz, 2H), 9.93 (s, 1H), 9.37 (s, 1H), 10.14 (s, 1H). 13C NMR (151 MHz, DMSO-d6) δ (ppm): 39.56, 60.23, 109.76, 112.09, 112.66, 115.98, 117.93, 126.43, 130.54, 133.04, 144.45, 149.91, 156.46, 159.25 and 160.74. MS: m/z (%): 270.8 [M + 1]+, 242.7, 152.5.
4.1.5.3. 7,8-Dihydroxy-4-(3,4-dihydroxyphenyl)-coumarin (8k). Yellow solid, yield: 97%, mp 182.0–183.1 °C, IR (KBr, ν, cm−1): 3265 (OH); 1666 (C
O); 2918, 1597, 1531, 1449, 1343, 1119, 1043, 847 (Ar); 1243, 1043 (C–O). 1H NMR (600 MHz, DMSO-d6) δ (ppm): 6.02 (s, 1H), 6.80 (s, 1H), 6.81 (d, J = 2.0 Hz, 1H), 6.89 (m, 2H), 6.95 (d, J = 8.8 Hz, 1H), 9.35 (s, 1H), 9.41 (s, 1H), 9.48 (s, 1H), 10.20 (s, 1H). 13C NMR (151 MHz, DMSO-d6) δ (ppm): 49.06, 109.54, 112.60, 116.31, 118.02, 120.45, 126.88, 133.01, 144.44, 145.79, 147.43, 149.87, 156.62, 160.77 and 172.51. MS: m/z (%): 286.9 [M + 1]+, 240.8, 152.6.
4.1.5.4. 7,8-Dihydroxy-4-(3,4,5-trihydroxyphenyl)-coumarin (8l). Light yellow solid, yield: 90%, mp 312.5–313.5 °C, IR (KBr, ν, cm−1): 3386 (OH); 1704 (C
O); 2851, 1600, 1539, 1452, 1317, 1038, 833 (Ar); 1226, 1077 (C–O). 1H NMR (600 MHz, DMSO-d6) δ (ppm): 5.98 (s, 1H), 6.42 (s, 2H), 6.80 (d, J = 8.8 Hz, 1H), 6.99 (d, J = 8.8 Hz, 1H), 8.60 (s, 1H), 9.22 (s, 2H), 9.36 (s, 1H), 10.13 (s, 1H). 13C NMR (151 MHz, DMSO-d6) δ (ppm): 56.54, 60.23, 107.99, 109.35, 112.06, 112.55, 118.11, 125.98, 132.98, 135.19, 144.43, 146.60, 149.86, 156.95 and 160.76. MS: m/z (%): 302.9 [M + 1]+, 256.8, 152.7.
4.1.5.5. 5,7-Dihydroxy-4-(3,4-dihydroxyphenyl)-coumarin (8m). Light yellow solid, yield: 93%, mp 261.5–262.5 °C, IR (KBr, ν, cm−1): 3305 (OH); 1698 (C
O); 1597, 1522, 1442, 1349, 1033, 845 (Ar); 1265, 1083 (C–O). 1H NMR (600 MHz, DMSO-d6) δ (ppm): 5.69 (s, 1H), 6.18 (d, J = 2.4 Hz, 1H), 6.25 (d, J = 2.3 Hz, 1H), 6.62 (dd, J = 8.1, 2.1 Hz, 1H), 6.72 (m, 2H), 9.01 (s, 1H), 9.11 (s, 1H), 10.11 (s, 1H), 10.40 (s, 1H). 13C NMR (151 MHz, DMSO-d6) δ (ppm): 49.07, 60.26, 95.02, 99.61, 101.19, 110.04, 115.94, 119.26, 130.93, 144.50, 146.03, 156.85, 157.65, 160.55 and 161.90. MS: m/z (%): 286.9 [M + 1]+, 152.6.
4.1.5.6. 6,8-Dihydroxy-4-(3,4-dihydroxyphenyl)-coumarin (8n). Light yellow solid, yield: 95%, mp 301.5–303.5 °C, IR (KBr, ν, cm−1): 3365 (OH); 1683 (C
O); 1607, 1520, 1446, 1395, 861 (Ar); 1276 (C–O). 1H NMR (600 MHz, DMSO-d6) δ (ppm): 5.99 (s, 1H), 6.80 (dd, J = 7.9, 2.3 Hz, 2H), 6.88 (d, J = 2.1 Hz, 1H), 6.90 (d, J = 8.1 Hz, 1H), 6.98 (s, 1H), 9.31 (s, 1H), 9.40 (s, 1H), 9.44 (s, 1H), 10.20 (s, 1H). 13C NMR (151 MHz, DMSO-d6) δ (ppm): 56.19, 103.61, 109.82, 110.78, 111.58, 116.30, 120.27, 126.95, 143.27, 145.84, 147.39, 148.97, 150.85, 155.98 and 161.15. MS: m/z (%): 287.1 [M + 1]+, 152.8.
4.2. Biological activity
4.2.1. Animals. Normoglycemic Kunming mice, weight 18–22 g, were obtained from Jinan PengYue Experimental Animal Co., Ltd. (License number: SCXK (Lu) 2014–0007). The animals were housed under standard laboratory conditions and maintained on a standard pellet diet and water ad libitum. All experiments involving living animals and their care were performed in strict accordance with the National Care and Use of Laboratory Animals by the National Animal Research Authority (China) and guidelines of Animal Care and Use issued by University of Jinan Institutional Animal Care and Use Committee. The experiments were approved by the Institutional Animal Care and Use Committee of the School of Medicine and Life Sciences, University of Jinan. All efforts were made to minimize animal's suffering and to reduce the number of animals used.
4.2.2. In vitro antioxidant activity. The ability of the target compounds and vitamin C to scavenge the hydroxyl radical was evaluated with method described by Ismaili and coworkers24 with slight modifications. In test tube 1 mL H2O2 solution (0.03%), 1 mL FeSO4 solution (2 mmol L−1), 1 mL salicylic acid solution (10 mmol L−1) and 1 mL of 4-arylcoumarins and standard water solution with different concentration (0.4–2.0 mg mL−1) were mixed. Finally adds 1 mL H2O2 solution (0.03%) to this mixture to start the reaction. Solutions were kept at 37 °C. After 30 minutes, the absorbance was measured at 510 nm against the reagent blank, where the distilled water was substituted for the H2O2 solution. The percentage of ·OH scavenging by target compounds and vitamin C was calculated using the following equation:
·OH scavenging effect (%) = [A0 − (A1 − A2)]/A0 × 100% |
where A0 is the absorbance of 1.0 mL distilled water + 1.0 mL H2O2 + 1.0 mL FeSO4 + 1.0 mL salicylic acid + 1.0 mL H2O2; A1 is the absorbance of 1.0 mL 4-arylcoumarins or standard + 1.0 mL H2O2 + 1.0 mL FeSO4 + 1.0 mL salicylic acid + 1.0 mL H2O2; A2 is the absorbance of 1.0 mL 4-arylcoumarins or standard + 1.0 mL H2O2 + 1.0 mL FeSO4 + 1.0 mL absolute ethanol + 1.0 mL H2O2.The effect of target compounds and vitamin C to scavenge the DPPH free radical was evaluated with method described by Villaño and coworkers37 with slight modifications.
2.0 mL of different concentrations of the sample solution was added to 2.0 mL of DPPH solution (0.04 mg mL−1), shaken and allowed to react at room temperature for 30 min. The absorbance value was measured at 517 nm with an ultraviolet-visible spectrophotometer. The blank reference cuvette contained absolute ethanol. All measurements were performed in triplicate. IC50 values were calculated. The percentage DPPH free radical scavenging rate was determined as
DPPH free radical scavenging effect (%) = [A0 − (A1 − A2)]/A0 × 100% |
where
A0 is the absorbance of 2.0 mL DPPH solution + 2.0 mL absolute ethanol;
A1 is the absorbance of 2.0 mL DPPH solution + 2.0 mL 4-arylcoumarins solution or standard;
A2 is the absorbance of 2.0 mL 4-arylcoumarins solution or standard + 2.0 mL absolute ethanol.
4.2.3. In vitro a-glucosidase inhibitory activity. α-Glucosidase (G0660-750UN, Sigma Aldrich) and 4-nitrophenyl α-D-glucopyranoside (PNPG, Macklin) were dissolved in phosphate buffer (pH 6.8, 100 mM), and the test compounds were dissolved in DMSO solution. The experiment was divided into blank group, control group, sample blank group and sample group. The reagents were loaded in 96-well plates at the dose of the Table 7. The solution was bathed in 37 °C water for 10 min, after the end, enzyme solution was added. After reaction at 37 °C for 20 min, 70 μL Na2CO3 solution (0.2 mM) was added to stop the reaction. All experiments were run in triplicate. Acarbose (Sigma Aldrich) was used as a standard inhibitor. Since PNPG can produce glucose and p-nitrophenol (PNP) under the action of α-glucosidase, PNP has the greatest absorption at 405 nm. The absorbance was determined by the microplate reader, and the inhibition rate of α-glucosidase and the IC50 value of each sample were calculated according to the formula.
Inhibition rate% = {[(AC − AB) − (AS − ASB)]/(AC − AB)} × 100% |
where AC is the absorbance of control group; AB is the absorbance of blank group; AS is the absorbance of sample group; ASB is the absorbance of sample blank group.
Table 7 The amount and order of each reactant of α-glucosidase inhibition test
Reagents |
Volume (μL) |
Blank group |
Control group |
Sample blank group |
Sample group |
PBS |
20 |
10 |
20 |
10 |
Compounds/inhibitors |
0 |
0 |
10 |
10 |
PNPG |
20 |
20 |
20 |
20 |
Water |
10 |
10 |
0 |
0 |
Mix well and incubate at 37 °C for 10 minutes |
α-Glucosidase |
0 |
10 |
0 |
10 |
Mix well and react at 37 °C for 20 minutes |
Na2CO3 |
70 |
70 |
70 |
70 |
4.2.4. In vitro inhibitory activity of AGEs (advanced glycation end products) formation. To prepare the AGE reaction solution, 10 mg mL−1 of bovine serum albumin in 50 mM PBS (pH 7.4) was added to 0.2 M glucose, and 0.02% sodium azide was added to prevent bacterial growth. The reaction mixture (3 mL) was then mixed with various concentrations (0.5–1000 μg mL−1) of the target compounds (1 mL) dissolved in DMSO. After incubating at 37 °C for 14 d, the fluorescence intensity of AGE was determined by a fluorospectrophotometer (PE, USA) with excitation and emission wavelengths at 350 nm and 420 nm, respectively. All experiments were run in triplicate. Aminoguanidine hydrochloride was used as a reference compound. The inhibition rate of AGEs formation and the IC50 value of each sample were calculated according to the formula.
Inhibition rate% = {[(AC − AB) − (AS − ASB)]/(AC − AB)} × 100% |
where AC is the absorbance of control group (1.0 mL glucose + 1.0 mL bovine serum albumin + 1.0 mL sodium azide + 1.0 mL DMSO); AB is the absorbance of blank group (1.0 mL PBS + 1.0 mL bovine serum albumin + 1.0 mL sodium azide + 1.0 mL DMSO); AS is the absorbance of sample group (1.0 mL glucose + 1.0 mL bovine serum albumin + 1.0 mL sodium azide + 1.0 mL target compound solution or aminoguanidine hydrochloride solution); ASB is the absorbance of sample blank group (1.0 mL PBS + 1.0 mL bovine serum albumin + 1.0 mL sodium azide + 1.0 mL target compound solution or aminoguanidine hydrochloride solution).
4.2.5. In vitro ALR2 inhibitory activity. After homogenization and centrifugation, the crude ALR2 from mice was obtained.38 The inhibitory activity of the compounds on ALR2 was carried out using crude enzyme and different concentrations of the compounds (1–1000 μg mL−1) in 200 mM PBS (pH 6.2) containing 0.10 mM NADPH. The reaction was initiated by addition of glyceraldehyde and the decrease in the optical density of NADPH at 340 nm was recorded for 3 min. All experiments were run in triplicate. IC50 of the compounds was calculated. The flavonoid quercetin was used as a reference in the ALR2 assay.
4.2.6. Oral toxicity to mice. Experiments were performed on Kunming mice (male and female half, body weight range, 25–30 g). Mice were housed in a climate and light controlled room with a 12 h light/dark cycle. Twelve hours before experiments, food was withheld, but animals had free access to drinking water. The compounds were suspended in vehicle (Tween-80, 0.2% in saline). The concentrations were adjusted to orally administrate 0.2 mL/10 g of bw (body weight). Mice were treated in two phases. In the first, intragastric doses of 10, 100 and 1000 mg kg−1 of bw of compounds were administered. On the second, the doses were adjusted to 1600, 2900 and 5000 mg kg−1 of bw of compounds. In both phases, mice were observed daily in a period of 14 days for mortality, toxic effects and/or changes in behavioral pattern. At the end of the experiments the mice were sacrificed in a CO2 chamber.
4.2.7. Acute hypoglycemic assay. Type II diabetes mellitus was induced in mice by a single intraperitoneal injection of freshly prepared STZ (Sigma Aldrich) dissolved in 0.1 M citrate buffer, pH 4.5, in a volume of 110 mg kg−1 of bw. After 7 days of STZ administration, blood glucose levels of each mouse were determined. Mice with blood glucose levels higher than 11 mM were considered diabetic and were included in the study.STZ-Induced diabetic mice and normal mice were placed in single cages with wire-net floors and deprived of food for 12 h before experimentation but allowed free access to tap water throughout. The compounds (at the doses of 10, 30 and 100 mg kg−1 of bw) were suspended in 0.05% Tween-80 in saline solution. Glibenclamide (10 mg kg−1 of bw) was suspended in the same vehicle. The target compounds were freshly prepared immediately before experimentation and administered by the intragastrical route at the doses of 10 mL kg−1 of bw. Control mice received only the vehicle (0.05% Tween-80 in saline solution) by the same route. Blood glucose levels were measured at 0, 1.5, 3, 5, 7, 9 h after drugs administration.
4.2.8. Effects of daily treatment with compounds 8l and 8m in STZ-induced diabetic mice. Type II diabetes mellitus was induced in 24 mice by the same method showed in the section of the induction of experimental diabetes. Thereafter, the target compounds 8l, 8m and glibenclamide were administered once (9 a.m.) on a daily basis, for a period of 16 days, to STZ-induced diabetic mice which were divided into four groups (A–D). A five group of normal mice (group E) was used as a control. Groups D (diabetic control) and E received vehicle (0.05% Tween-80 in saline solution) per os during 16 days. Group A received glibenclamide (10 mg kg−1 of bw daily). Groups B and groups C received different treatments: (B) compound 8l (30 mg kg−1 of bw daily); (C) compound 8m (30 mg kg−1 of bw daily). All tested materials were suspended in the same vehicle and administered per os daily for 16 days. The animals were housed under standard laboratory conditions and maintained with free access to water and food during all the experiment. Blood glucose concentration on animals of diabetic (groups A–D) and control group (group E) was estimated at days 1, 4, 7, 10, 13 and 16. The body weights of the animals were measured concomitantly to the blood glucose analyses. All experiments were carried out using six animals per group.
4.2.9. Oral glucose tolerance test of compounds 8m and 8l in STZ-induced diabetic mice. The target compounds 8l and 8m (30 mg kg−1 of bw) and control drugs (glibenclamide 10 mg kg−1 of bw) were dissolved in the vehicle (Tween-80, NaCl 0.9%). Glucose (2 g kg−1 of bw) was used for the glucose tolerance tests. The control mice group received only the vehicle. All cases were treated orally. Blood samples were collected from caudal vein by means of a small incision in the end of the tail. Blood glucose levels were estimated by a commercial glucometer (Bayer Contour TS). For the antihyperglycemic tests, blood samples were obtained 0.5, 1, 1.5, 2, and 3 hour after the glucose load. Percentage variation of glycemia for each group was calculated with respect to initial (0 h) level according to:
% variation of glycemia = [(Gi − Gt)/Gi] × 100% |
where Gi is initial glycemia values and Gt is the glycemia value after samples administration. For the antihyperglycemic tests data were represented as % variation of glycemia versus time. Studies were performed in normoglycemic and STZ-induced diabetic mice.
4.2.10. Statistical analysis. Data were shown as mean ± S.D. differences between individual groups were analyzed by using ANOVA followed by Dunnett's test. A difference with a P value of <0.05 was considered to be significant.
Conflict of interest
There are no conflicts of interest to declare.
Acknowledgements
The authors are grateful to support from the Project of Shandong Province Higher Educational Science and Technology Program (J14M02), the Science and Technology Research Program of Shandong Academy of Medical Sciences (2014-4), Shandong Provincial Natural Science Foundation (ZR2015YL041) and the Innovation Project of Shandong Academy of Medical Sciences.
References
- M. M. Garazd, Y. L. Garazd and V. P. Khilya, Chem. Nat. Compd., 2003, 39, 54–121 CrossRef CAS.
- M. H. Lin, Y. S. Chou, Y. J. Tsai and D. S. Chou, J. Exp. Clin. Med., 2011, 3, 126–131 CrossRef CAS.
- K. Zhang, W. Ding, J. Sun, B. Zhang, F. Lu, R. Lai, Y. Zou and G. Yedid, Chem. Nat. Compd., 2012, 107, 203–210 Search PubMed.
- F. Pérez-Cruz, F. A. Villamena, G. Zapata-Torres, A. Das, C. A. Headley, E. Quezada, C. Lopez-Alarcon and C. Olea-Azar, J. Phys. Org. Chem., 2014, 26, 773–783 CrossRef.
- R. Korec, M. Korecová, K. H. Sensch and T. Zoukas, Diabetes Res. Clin. Pract., 2000, 50, 42 CrossRef.
- E. Rizzi, S. Dallavalle, L. Merlini, G. L. Beretta, G. Pratesi and F. Zunino, Bioorg. Med. Chem. Lett., 2005, 15, 4313 CrossRef CAS PubMed.
- C. Billard, F. Menasria, C. Quiney, A. M. Faussat, J. P. Finet, S. Combes and J. P. Kolb, Exp. Hematol., 2008, 36, 1625 CrossRef CAS PubMed.
- F. Menasria, A. G. B. Azebaze, C. Billard, A. M. Faussat, A. E. Nkengfack, M. Meyer and J. P. Kolb, Leuk. Res., 2008, 32, 1914–1926 CrossRef CAS PubMed.
- Y. Kong, Y. J. Fu, Y. G. Zu, F. R. Chang, Y. H. Chen, X. L. Liu, J. Stelten and H. M. Schiebel, Food Chem., 2010, 121, 1150–1155 CrossRef CAS.
- S. K. Roy, N. Kumari, S. Pahwa, U. C. Agrahari, K. K. Bhutani, S. M. Jachak and H. Nandanwar, Fitoterapia, 2013, 90, 140 CrossRef CAS PubMed.
- C. Canning, S. Sun, X. Ji, S. Gupta and K. Zhou, J. Ethnopharmacol., 2013, 147, 259–262 CrossRef CAS PubMed.
- T. Taechowisan, C. Lu, Y. Shen and S. Lumyong, Microbiology, 2005, 151, 1691 CrossRef CAS PubMed.
- R. Argotteramos, G. Ramírezavila, M. C. Rodríguezgutiérrez, M. Ovillamuñoz, H. Lanzmendoza, M. H. Rodríguez, M. Gonzalezcortazar and L. Alvarez, J. Nat. Prod., 2006, 69, 1442–1444 CrossRef CAS PubMed.
- J. T. Pierson, A. Dumètre, S. Hutter, F. Delmas, M. Laget, J. P. Finet, N. Azas and S. Combes, Eur. J. Med. Chem., 2010, 45, 864–869 CrossRef CAS PubMed.
- J. V. Richard, K. A. Werbovetz, M. Gelb and A. Whitty, Curr. Opin. Chem. Biol., 2010, 14, 447 CrossRef CAS PubMed.
- S. Nishimura, M. Taki, S. Takaishi, Y. Iijima and T. Akiyama, ChemInform, 2000, 31, 505–508 Search PubMed.
- R. Korec, S. K. Heinz and T. Zoukas, Arzneim. Forsch., 2000, 50, 122–128 CAS.
- J. Guerrero-Analco, O. Medina-Campos, F. Brindis, R. Bye, J. Pedraza-Chaverri, A. Navarrete and R. Mata, Phytochemistry, 2007, 68, 2087–2095 CrossRef CAS PubMed.
- M. M. Garazd, Y. L. Garazd and V. P. Khilya, Chem. Nat. Compd., 2005, 41, 245–271 CrossRef CAS.
- J. Sun, X. W. Ding, K. Y. Zhang and Y. Zou, Chin. Chem. Lett., 2011, 22, 667–670 CrossRef CAS.
- L. Jian-Ming, T. Tsui-Hwa and L. Yean-Jang, Synthesis, 2001, 2001, 2247–2254 CrossRef.
- J. Sun, X. W. Ding, X. P. Hong and K. Y. Zhang, Chem. Nat. Compd., 2012, 48, 16–22 CrossRef CAS.
- J. B. Veselinović, A. M. Veselinović and Ž. J. Vitnik, et al., Antioxidant properties of selected 4-phenyl hydroxycoumarins: integrated in vitro and computational studies, Chem.–Biol. Interact., 2014, 214(1), 49 CrossRef PubMed.
- L. Ismaili, A. Nadaradjane, L. Nicod, C. Guyon, A. Xicluna, J. F. Robert and B. Refouvelet, Eur. J. Med. Chem., 2008, 43, 1270–1275 CrossRef CAS PubMed.
- K. Y. Zhang, W. X. Ding, J. Sun, B. Zhang, F. J. Lu, R. Lai, Y. Zou and G. Yedid, Biochimie, 2014, 48(1), 16–22 Search PubMed.
- R. Mata, S. Cristians, S. Escandónrivera, K. Juárezreyes and I. Riverocruz, J. Nat. Prod., 2013, 76, 468–483 CrossRef CAS PubMed.
- J. Riverachávez, M. Figureroa, M. C. González, A. E. Glenn and R. Mata, J. Nat. Prod., 2015, 78, 730 CrossRef PubMed.
- D. Liu, W. He, Z. Wang, L. Liu, C. Wang, C. Zhang, C. Wang, Y. Wang, G. Tanabe and O. Muraoka, Eur. J. Med. Chem., 2016, 110, 224 CrossRef CAS PubMed.
- Z. Ni, Z. Zhuge, W. Li, H. Xu, Z. Zhang and H. Dai, Biol. Pharm. Bull., 2012, 35, 2050–2053 CAS.
- X. Q. Zou, S. C. Peng, L. F. Tan, Q. Yuan, H. W. Deng and Y. J. Li, Bioorg. Med. Chem., 2010, 18, 3020–3025 CrossRef CAS PubMed.
- X. Qin, X. Hao, H. Han, S. Zhu, Y. Yang, B. Wu, S. Hussain, S. Parveen, C. Jing and B. Ma, J. Med. Chem., 2015, 58, 1254–1267 CrossRef CAS PubMed.
- Y. C. Hwang, M. Kaneko, S. Bakr, H. Liao, Y. Lu, E. R. Lewis, S. Yan, S. Ii, M. Itakura and L. Rui, FASEB J., 2004, 18, 1192–1199 CrossRef CAS PubMed.
- D. Lorke, Arch. Toxicol., 1983, 54(4), 275–287 CrossRef CAS PubMed.
- S. Cristians, R. Bye, A. Navarrete and R. Mata, J. Ethnopharmacol., 2013, 145, 530–535 CrossRef CAS PubMed.
- S. Cristians, R. Mata and R. Bye, J. Ethnopharmacol., 2014, 152, 308–313 CrossRef CAS PubMed.
- C. N. Rhodes and D. R. Brown, Catal. Lett., 1994, 24, 285–291 CrossRef CAS.
- D. Villaño, M. S. Fernándezpachón, M. L. Moyá, A. M. Troncoso and M. C. Garcíaparrilla, Talanta, 2007, 71, 230 CrossRef PubMed.
- S. Chethan, S. M. Dharmesh and N. G. Malleshi, Bioorg. Med. Chem., 2008, 16, 10085–10090 CrossRef CAS PubMed.
Footnotes |
† Electronic supplementary information (ESI) available. See DOI: 10.1039/c7ra06457h |
‡ These authors contributed equally. |
|
This journal is © The Royal Society of Chemistry 2017 |