DOI:
10.1039/C7RA06109A
(Paper)
RSC Adv., 2017,
7, 45252-45259
Fruit bromelain ameliorates rat constipation induced by loperamide†
Received
1st June 2017
, Accepted 29th August 2017
First published on 22nd September 2017
Abstract
Unsweetened pineapple juice is beneficial for routine constipation. The objective of this study was to determine the effect of bromelain (EC 3.4.22.33) isolated and purified from pineapple fruit on constipation. A rat constipation model was established by gavage administration of loperamide hydrochloride. The effects of bromelain on constipation in rats were evaluated in vitro and in vivo. In the ex vivo study, the isometric force of jejunal strips and changes in intracellular Ca2+ ([Ca2+]i) were measured simultaneously. The effect of bromelain on myosin light chain kinase (MLCK)-related intestinal contraction was also studied. Bromelain significantly relieved the high visceral sensitivity and infrequent stools in constipated rats. Bromelain also reversed the slow gastric emptying and slow intestinal transit in constipated rats. Bromelain stimulated intestinal contraction, and the stimulatory effect was inhibited by hemicholinium-3 and atropine, which block the synthesis of endogenous acetylcholine (ACh). The amount of ([Ca2+]i) was significantly increased by bromelain. Bromelain significantly reversed the decreased phosphorylation of the 20 kDa regulatory light chain of myosin (p-MLC20) and the expression of MLCK in constipated rats. These results indicated that bromelain triggered the release of endogenous ACh, increased [Ca2+]i, and enhanced p-MLC20 via activation of MLCK expression, resulting in the increase of gut motility. The beneficial effect of pineapple on constipation is partly dependent on fruit bromelain although the fibers and fluid in pineapple juice are also beneficial.
Introduction
Bromelain is a protease found in pineapple plants. The proteinases found in pineapple include fruit bromelain (EC 3.4.22.33), stem bromelain (EC 3.4.22.32), and ananain (EC 3.4.22.31).1,2 Bromelain was reported to have a wide range of therapeutic benefits, including antiedematous, anti-inflammatory, antithrombotic, fibrinolytic, and anticancer effects.3,4 Bromelain is a phytotherapeutic agent with high efficacy and safety, with no undesired side effects after oral administration, and has earned growing acceptance and compliance among patients. Borrelli and colleagues reported that stem bromelain could inhibit the contractions induced by different spasmogenic substances in the mouse ileum.5 The results of our previous experiments indicated that fruit bromelain could increase gut motility in mice and rats. The objective of this study was to characterize the effects of fruit bromelain on constipated rats and determine the mechanisms underlying these effects. Constipation is a common disorder that affects approximately 20% of the population and significantly impacts an individual's quality of life, and the current treatment remains unsatisfactory.6,7 Agents with low toxicity and high efficacy possess novel mechanisms in fighting against constipation and can be well tolerated by the affected patients.
Fruit bromelain, a 34 kDa cysteine peptidase, was purified and identified by our research group as fruit bromelain (EC 3.4.22.33) using high-speed counter-current chromatography.8 The stimulatory effects of bromelain on gastrointestinal motility disorder was evaluated by establishing a constipation rat model via gavage administration of loperamide hydrochloride.9 The effects of gavage administration of bromelain on constipation were studied because bromelain can be absorbed in the intestine without being degraded and without losing its pharmacological activity.10 Chronic constipation is the main symptom of constipation in rats with low gastrointestinal motility compared with normal controls.7,11 As intestinal contractility is positively correlated with the phosphorylation of the 20 kDa regulatory light chain of myosin (p-MLC20),12 the effects of fruit bromelain on the extent of p-MLC20 and levels of myosin light chain kinase (MLCK) protein were measured using western blotting. The expression of MLCK mRNA was determined using quantitative reverse transcription polymerase chain reaction (qRT-PCR). Isolated jejunal segments of rats were selected for the measurement of contractility using a bath recording system because the intestine is modulated by the enteric nervous system and fulfills a pivotal role even when isolated from the body.13
Materials and methods
Preparation of bromelain
The purified bromelain used in this study was purified from the crude protein extract of pineapple fruit by high-speed counter-current chromatography, as previously described.14 Purified bromelain was analyzed by SDS-PAGE, compared with the standard protein, and identified by TOF/TOF-MS.
Ethical statement
This study was performed in strict accordance with the NIH guidelines for the care and use of laboratory animals (NIH Publication no. 85-23 Rev. 1985) and was approved by the Animal Care and Ethics Committee of Dalian Medical University (Dalian, China).
Animals and reagents
The animals were obtained from the Experimental Animal Center of Dalian Medical University (Certificate of Conformity no. SCXK (Liao) 2008-0002). Eighty four Sprague-Dawley rats (50% male and 50% female) weighing 180–220 g were randomly divided into seven groups, including normal controls, constipated rats, constipated rats treated with 5, 10 and 20 mg kg−1 of bromelain, and constipated rats treated with 5 and 20 mg kg−1 of crude bromelain. The animals were acclimatized to laboratory conditions (23 °C, 12 h/12 h light/dark cycles, 50% humidity, and access to food and water ad libitum) for 2 weeks before the experiments. One rat was housed per cage. The animal protocol was designed to minimize pain or discomfort to the animals.
The purified fruit bromelain used in this study was isolated and purified as detailed in our previous study.8 Unless otherwise indicated, chemicals were obtained from Sigma-Aldrich (St. Louis, MO, USA).
Experimental model of constipation
A rat constipation model was established by gavage administration of 3 mg kg−1 of loperamide hydrochloride daily for 6 days.9,15 The control rats were prepared by daily gavage administration of DDH2O at room temperature. On the second day after loperamide hydrochloride administration, the rats in the bromelain-treated constipation group were gavaged with bromelain for 7 days. The moisture content and number of fecal pellets from all rat groups were measured daily at 09:30 h throughout the study period. The water content was calculated as the difference between the wet and dry weights of the pellets.
Evaluation of visceral sensitivity
Five abdominal withdrawal reflex (AWR) scores (0 to 4) were used to measure the visceral sensitivity of the rats during colorectal distension (CRD).16 The CRD balloon covered with lubricant was completely inserted into the descending colon and rectum under ether anesthesia. The AWR was scored as follows: AWR0, no remarkable changes in the behavior of the rats; AWR1, immobility of the rat body or occasional clinches of the head; AWR2, mild abdominal muscle contraction; AWR3, lifting the abdomen off the platform or flatting of the abdomen; AWR4, body arching or lifting the pelvic structures off the platform. The threshold was defined as the minimal pressure (kPa) inside the balloon when the rat showed flattening of the abdomen (AWR3) during CRD.
Evaluation of gastric emptying and intestinal transit
Gastric emptying and intestinal transit were measured according to previously described methods with some modifications.17,18 Briefly, 2 mL of distilled water containing 1.5% methylcellulose and anthracotic marker (MAM) was given intragastrically and the rats were killed by CO2 inhalation after 30 min. Gastric emptying was calculated using the formula (gavaged MAM – MAM left in gastric)/gavaged MAM. Intestinal transit was calculated as the length traveled by the marker from the intestine to the pylorus divided by the distance from the pylorus to the anus.
Tissue preparation and determination of jejunal contractility
Segments of the intact tubular jejunum were prepared according to a method described previously.19 Jejunal segments were cut into a length of approximately 2 cm (tubes) and then allowed to equilibrate in aerated Krebs buffer for 50 min; the bath solution was replaced every 10 min. The resting tension was set optimally at 1.0 g. The contractile amplitude of each jejunal segment was measured from the baseline to the peak and was expressed as a percentage of the normal contractile amplitude. The contractile amplitude was recorded, and identical time intervals in each assay with the same start and stop time were chosen to compare the amplitude before and after drug treatment in different assay conditions. The mean amplitude was calculated from six independent assays.
Western blot analysis
The phosphorylation of the 20 kDa regulatory light chain subunit of myosin (MLC20) and the MLCK protein content in jejunal samples were examined using western blot analysis as described previously.20 The jejunal segments were immediately isolated from rats and stored in liquid nitrogen. The samples were homogenized and incubated in ice-cold homogenization buffer for 30 min. The blots were transferred to a nitrocellulose filter membrane and probed with phosphor-myosin light chain 2 (Ser 19) antibody (1
:
1000) (Product no. 3671, Cell Signaling Technology, Inc. (CST) USA) and myosin light chain 2 (total myosin light chain) antibody (1
:
1000) (Product no. 3672, CST, USA) at 4 °C overnight with gentle shaking. For MLCK analysis, the blots were transferred to a nitrocellulose filter membrane and probed with MLCK antibody (1
:
1000) (Product no. ab76092, Abcam [Hong Kong] Ltd., UK). After that, the blots were incubated with anti-rabbit IgG secondary antibody (1
:
250) for 90 min at room temperature and the bands were detected and quantified using a MultiSpectral Imaging System (UVP, Cambridge, UK).
Simultaneous measurement of [Ca2+]i and isometric force of intestinal strips in situ
The isolated jejunal segments were cut into strips (length of 1 to 4 mm) without endothelium and adventitia. These strips were mounted vertically on an organ bath filled with physiological salt solution, gassed with 5% CO2/95% O2, and maintained at 37 °C. The isometric force of jejunal strips and changes in intracellular Ca2+ ([Ca2+]i) were measured simultaneously.21 The jejunal strips were incubated with 12.5 μM of fura-2/AM. Changes in [Ca2+]i were recorded continuously with a spectrofluorometer (CAM-230, Japan Spectroscopic) equipped with a randomized optical fiber system.22
RT-PCR analysis of MLCK expression
Total RNA was extracted from jejunal segments using TRIzol® reagent (Life Technologies, Rockville, MD, USA). For cDNA synthesis, oligo (dT) primers were used to prime the reverse transcription reactions. The primers (Takara Biotechnology (Dalian) CO., LTD) were designed using published sequences to amplify the MLCK transcripts. The reference gene GAPDH served as an internal control. The primer sequences for PCR were MLCK: 5′-AATGGTGTTGCTGGAGATCGAGGT-3′ (forward) and 5′-GCTGGATCAAATTGCGGTGGTTCA-3′ (reverse), and GAPDH: 5′-GGCAAGTTCAATGGCACAGT-3′ (forward) and 5′-TGGTGAAGACGCCAGTAGACTC-3′ (reverse). RT-PCR was performed using the SYBR green method for fluorescence detection on an ABI 7500 Fast Real-Time PCR System (Applied Biosystems). PCR conditions were as follows: initial denaturation at 95 °C for 30 s followed by 40 thermal cycles at 95 °C for 5 s, 60 °C for 34 s, and 72 °C for 30 s. The amplification of MLCK was normalized to the amplification of GAPDH mRNA in the same sample (ΔCt, the difference between CtMLCK and CtGAPDH). ΔCt was then transformed to absolute values (2−ΔCt) and expressed as percentage of the expression in control samples.
Statistical analysis
One-way ANOVA was used for the comparison of three or more groups of data. Student t-test was used to compare two groups of data. Data were expressed as the mean ± SD. The data followed a normal distribution and the variance of each group was equal. The data were further evaluated by using the Kruskal–Wallis rank sum test. All experiments were repeated at least six times and a P-value of less than 0.05 was considered statistically significant.
Results
Effect of bromelain on constipation in vivo
Food intake, water intake, number of fecal pellets, water content of fecal pellets, and weight of fecal pellets in constipated rats were significantly decreased compared with those of normal control rats, which suggests the successful establishment of the constipation model. These changes were reversed in the constipation group by gavage administration of bromelain (Table 1).
Table 1 Effect of bromelain on food intake, water intake, and characteristics of fecal pellets of constipated ratsa
|
Normal control |
Constipation |
Bromelain (5 mg kg−1) treatment |
Bromelain (10 mg kg−1) treatment |
Bromelain (20 mg kg−1) treatment |
Data in normal control group are set to a relative value of 100% and expressed as the mean ± SD. Other data are the relative values compared with normal control. P < 0.01 compared with the NC. P < 0.01 compared with the constipation group, n = 6 rats. |
Food intake |
(100.0 ± 9.1)% |
(96.3 ± 8.2)% |
(106.7 ± 11.6)% |
(109.4 ± 13.8)% |
(104.3 ± 11.2)% |
Water intake |
(100.0 ± 10.3)% |
(58.3 ± 6.9)b% |
(79.2 ± 6.3)c% |
(89.5 ± 9.2)c% |
(93.5 ± 8.7)c% |
Number of fecal pellet |
(100.0 ± 12.6)% |
(31.2 ± 2.4)b% |
(51.3 ± 5.2)c% |
(79.6 ± 7.4)c% |
(94.2 ± 10.5)c% |
Water content of fecal pellet |
(100.0 ± 13.1)% |
(53.7 ± 6.2)b% |
(73.6 ± 8.1)c% |
(88.3 ± 7.8)c% |
(96.4 ± 8.7)c% |
Weight of fecal pellet |
(100.0 ± 10.8)% |
(61.2 ± 7.8)b% |
(80.3 ± 9.1)c% |
(93.0 ± 10.1)c% |
(101.6 ± 12.4)c% |
Bromelain (Fig. 1A) at the doses of 5.0 and 20.0 mg kg−1 significantly increased intestinal transit and gastric emptying in constipated rats. Bromelain (Fig. 1A) at the doses of 5.0, 10 and 20.0 mg kg−1 significantly increased intestinal transit and gastric emptying in normal mice (ESI Fig. 1†). The AWR score was used to assess rat visceral hypersensitivity. The AWR score was significantly higher in the constipated group than that in the normal control, suggesting that visceral hypersensitivity in constipated rats was higher than that in normal control rats. These changes were reversed in the constipated group by gavage administration of bromelain (Fig. 1B).
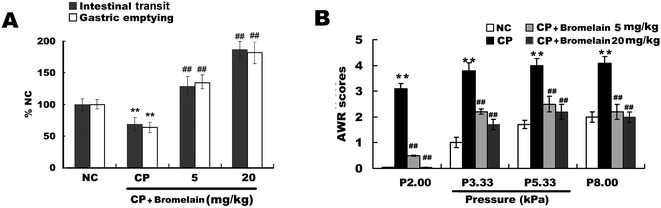 |
| Fig. 1 In vivo analysis of bromelain-exerted effects on the gut function in rats with constipation (CP). (A) Effect of bromelain (5.0, 20.0 mg kg−1) on gastric emptying and intestinal transit in CP rats. (B) Effects of bromelain (5.0, 20.0 mg kg−1) on visceral sensitivity in CP rats. Five abdominal withdrawal reflex (AWR) scores (0 to 4) were used to measure the visceral sensitivity of the rats during colorectal distension. In panel A, data in the normal control group are set to a relative value of 100% (normal control, NC) and expressed as the mean ± SD. The remaining data represented the relative values compared with the NC. **P < 0.01 compared with the NC; ##P < 0.01 compared with the CP group, n = 6 rats. | |
Effects of bromelain on intestinal contraction and [Ca2+]i
Isolated rat jejunal segments were used to further characterize the bromelain-induced modulation of intestinal smooth muscle contractility. At the concentration of 2.5–80.0 μmol L−1, bromelain increased the contractile amplitude of jejunal segments (Fig. 2A). At the concentration of 5.0–20.0 μmol L−1, bromelain reversed the reduction of the contractility of jejunal segments incubated in Krebs buffer containing a low Ca2+ concentration (2.5 mmol L−1) or in the presence of 10.0 μmol L−1 norepinephrine (NE). Moreover, bromelain reversed the decrease of the contractility of jejunal segments isolated from constipated rats (Fig. 2B).
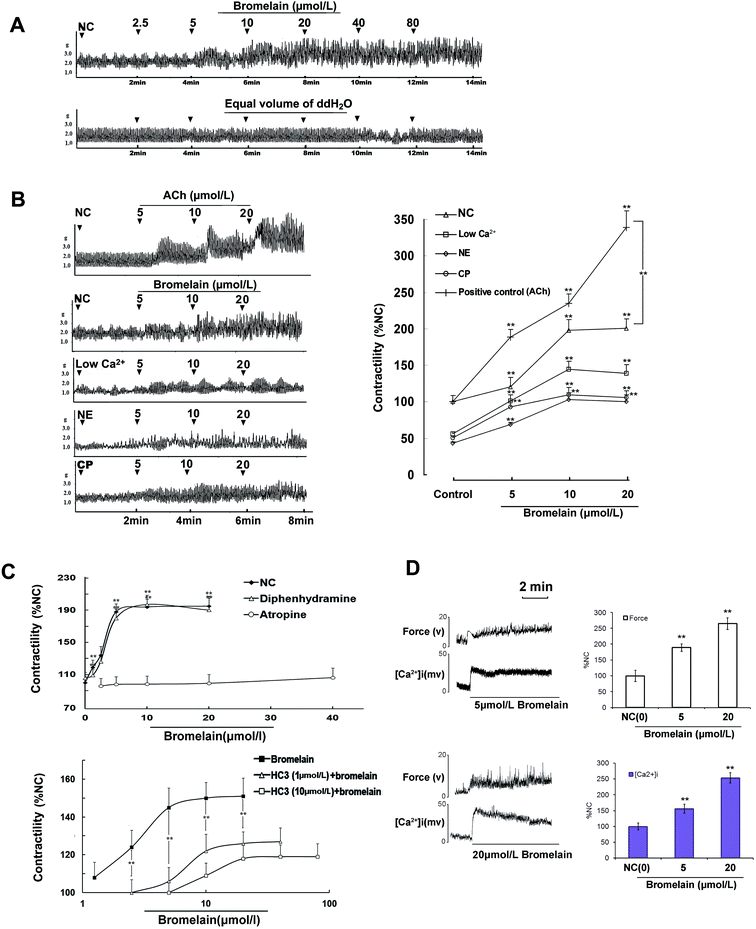 |
| Fig. 2 Mechanisms underlying bromelain-induced jejunal contraction (A) effects of bromelain on jejunal contraction. (B) effects of bromelain on jejunal contraction in different contractile states. (C) Effects of bromelain on jejunal contraction in the presence of atropine and hemicholinium-3 (HC-3), respectively. (D) Effects of bromelain on intracellular free Ca2+ concentration ([Ca2+]i). Data are expressed as mean ± SD. Data in the group not treated with bromelain were set to 100% (NC), and the remaining data corresponded to the relative value compared with the NC. (% NC, n = 6). **P < 0.01 compared with the NC group, n = 6 tissues. | |
Histamine H1 receptor blocker diphenhydramine did not significantly change the effect of bromelain on intestinal contraction (Fig. 2C, upper panel). Muscarinic receptor blocker atropine blocked bromelain-induced contraction (Fig. 2C, upper panel). In the presence of hemicholinium-3 (0.1 μmol L−1, 10 μmol L−1), an endogenous blocker of ACh synthesis, the maximum effect exerted by bromelain was significantly decreased and the dose–response curve was shifted to the right (Fig. 2C; lower panel).
The incubation of jejunal smooth muscle strips with 5.0 μmol L−1 and 20.0 μmol L−1 bromelain for 5 minutes increased the contractile force and significantly increased [Ca2+]i from 100.0 ± 12.7% to 156.2 ± 12.1% and from 100.0 ± 13.9% to 253.5 ± 18.3%, respectively.
Effect of bromelain on p-MLC20
The extent of myosin phosphorylation is positively correlated with smooth muscle contraction (20). The targeted deletion of MLCK in gastrointestinal smooth muscle resulted in severe gastrointestinal dysmotility, characterized by weak peristalsis and dilation of the digestive tract, suggesting that MLCK is central to smooth muscle contraction and is required for gastrointestinal motility (21, 22). In the present study, the effects of bromelain on p-MLC20, MLCK mRNA expression, and MLCK protein levels were investigated. The extent of p-MLC20 in constipated rats was significantly decreased compared with that in the normal control group (from 100.0 ± 9.1% to 37.2 ± 1.6%). After gavage with bromelain (5.0, 10.0, or 20.0 mg kg−1 once daily for 3 consecutive days), the extent of p-MLC20 was significantly increased in constipated rats treated with 20.0 mg kg−1 of bromelain (from 37.2 ± 1.6% to 88.3 ± 6.3%) (Fig. 3A). However, there was no significant difference in the extent of p-MLC20 between the bromelain-treated groups and the normal control group. MLCK mRNA expression in constipated rats was significantly decreased compared with that in the normal control group (from 100.0 ± 6.2% to 41.2 ± 2.4%). MLCK mRNA expression in constipated rats treated with 20.0 mg kg−1 of bromelain was significantly increased (from 41.2 ± 2.4% to 113.2 ± 8.6%) (Fig. 3B). The effect of bromelain on MLCK protein levels agreed with the results on MLCK mRNA expression (Fig. 3C). These results suggest that abnormal gastrointestinal hypomotility in constipated rats is closely related to the decrease in p-MLC20, which leads to the relaxation of the gastrointestinal tract, and bromelain restores gastrointestinal motility in constipated rats by increasing p-MLC20 via upregulation of MLCK expression.
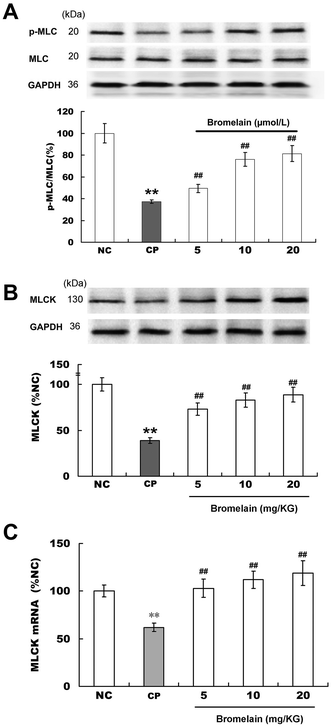 |
| Fig. 3 Effect of bromelain on myosin phosphorylation in rat jejunal smooth muscle. Rats from the normal control (NC), constipation (CP) group, and CP rats treated with bromelain were killed on day 14. Jejunal segments from each group were isolated to determine the effects of bromelain on the phosphorylation of the 20 kDa regulatory light chain subunit of myosin (p-MLC20), myosin light chain kinase (MLCK) mRNA expression, and MLCK protein content. (A) Representative traces and statistical analysis of bromelain on the p-MLC20. (B) Representative traces and statistical analysis of bromelain on the protein content of MLCK. (C) Effects of bromelain on the expression of MLCK mRNA. The data obtained from the NC group were set to a relative value of 100%. The remaining data corresponded to the relative values compared with the NC. Data are expressed as the mean ± SD. **P < 0.01 compared with the NC group, ##P < 0.01 compared with the CP group, n = 6 experiments. | |
Comparative study with crude bromelain
As purified bromelain is the main bioactive constituent in crude bromelain. The effects of purified bromelain on gastrointestinal motility were also studied compared with crude bromelain. Crude bromelain (20 mg kg−1) also reversed the decreased gastric emptying and intestinal transit, but the effect is lower than that exerted by purified bromelain (Fig. 4A and B).
 |
| Fig. 4 Effect of bromelain on constipated (CP) rats compared with crude bromelain. (A) Effects of bromelain and crude bromelain on gastric emptying in CP; (B) effects of bromelain and crude bromelain on intestinal transit in CP. The data obtained from the NC group were set to a relative value of 100%. The remaining data corresponded to the relative values compared with the NC. Data are expressed as the mean ± SD. **P < 0.01 compared with the NC group, ##P < 0.01 compared with the CP group, &&P < 0.01 compared with 300 μg mL−1 bromelain treated group, n = 6 rats. | |
In the ex vivo study, 300 μg mL−1 crude also stimulated the contractility of isolated intestinal segment, but the stimulatory effect is lower than that exerted by purified bromelain (Fig. 5A and B).
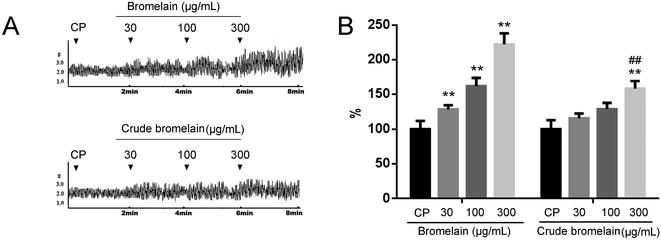 |
| Fig. 5 Effect of bromelain on the contractility of constipated rat (CP) intestinal preparation compared with crude bromelain. The data obtained from the CP group were set to a relative value of 100%. The remaining data corresponded to the relative values compared with the CP. Data are expressed as the mean ± SD. **P < 0.01 compared with the NC group, ##P < 0.01 compared with 300 μg mL−1 bromelain treated group, n = 6 rats. | |
Discussion
In the present study, the effects of purified fruit bromelain on constipation were investigated in vivo and ex vivo. Our results indicated that bromelain significantly alleviated the loperamide-induced constipation symptoms by releasing endogenous ACh and phosphorylating Ca2+-dependent myosin.
The anti-inflammatory effects of bromelain seem to be related to the protease activity. However, other effects such as inhibition of cell growth and metastasis are associated with other nonproteinolytic activity.23 According to the results in this study, we assumed that the proteolytic activity of the bromelain may be not the main mechanism underlying bromelain induced stimulation on gastrointestinal motility. Loperamide is a commonly used as antidiarrheal agent, and loperamide is used as an agent to set constipation model by inhibiting the intestinal smooth muscle contraction and reducing bowel movements.24 The smooth muscle contraction related mechanisms were mainly studied to uncover the mechanisms underlying bromelain induced stimulation on gut motility. The activation of muscarinic receptors or histamine H1 receptor may be mediated by the increase in gastrointestinal motility.25 In this study, bromelain-induced intestinal contraction was not affected by histamine H1 receptor blocker diphenhydramine, suggesting that histamine H1 receptor was not involved in the bromelain-induced stimulation of intestinal contraction. The bromelain-induced stimulatory effects were blocked by atropine and hemicholinium-3. Considering that bromelain-induced contraction was significantly weaker than ACh-induced contraction and the core structures of bromelain and ACh are different, the stimulatory effect of bromelain on jejunal contractility may be correlated with the stimulation of the release of endogenous ACh. Ca2+ is a major signaling and regulatory molecule in interstitial cells of Cajal and smooth muscle cells.26,27 Electrical excitability in smooth muscle is associated with a robust increase in [Ca2+]i.28 In this study, [Ca2+]i was significantly increased by bromelain treatment, and the increased [Ca2+]i was accompanied by smooth muscle contraction. We hypothesize that the increase in [Ca2+]i by bromelain involves at least two mechanisms: 1 bromelain induces the release of endogenous ACh and then promotes the increase in [Ca2+]i, and bromelain directly promotes the increase in [Ca2+]i, however, these hypotheses need to be confirmed by future studies.
The targeted deletion of MLCK in gastrointestinal smooth muscle resulted in severe gastrointestinal dysmotility, characterized by weak peristalsis and dilation of the digestive tract, suggesting that MLCK is central to smooth muscle contraction and is required for gastrointestinal motility.29 In the present study, the expression of p-MLC20 and MLCK was significantly increased by bromelain treatment. These results suggest that abnormal gastrointestinal hypomotility in constipated rats is closely related to the decrease in p-MLC20, which leads to relaxation of the gastrointestinal tract, and bromelain restores gastrointestinal motility in constipated rats by increasing p-MLC20 via upregulation of the MLCK expression.
It is known that pineapple juice is beneficial for alleviating routine constipation, including infrequent or difficult-to-pass stools. Carbohydrates, organic acids, fatty acids, and some types of salt are also present in pineapple fruit. However, the most well-known constituent with potent pharmacological effects is bromelain.30 Compared with crude bromelain, the purified bromelain exerted stronger stimulatory effects on gastrointestinal motility at the same dose/concentration. This study suggests that the ameliorative effect of pineapple fruit on constipation is partly dependent on fruit bromelain. Natural bioactive constituents may be used in drug discovery because of the low toxicity of these constituents.31,32 The results of the present study provide new insights into constipation therapy by bromelain.
Conflicts of interest
The authors declare that there are no conflicts of interest associated with this study.
Acknowledgements
This research was supported by the National Natural Science Foundation of China under Protocol No. 81600440 and 30772601. The authors are grateful to Zhi Lin and Fan Yuan for their comments.
References
- A. D. Rowan and D. J. Buttle, Methods Enzymol., 1994, 244, 555–568 CAS.
- L. P. Hale, Int. Immunopharmacol., 2004, 4, 255–264 CrossRef CAS PubMed.
- H. R. Maurer, Cell. Mol. Life Sci., 2001, 58, 1234–1245 CrossRef CAS PubMed.
- S. Wen, T. H. Huang, G. Q. Li, J. Yamahara, B. D. Roufogalis and Y. Li, Life Sci., 2006, 78, 995 CrossRef CAS PubMed.
- F. Borrelli, R. Capasso, B. Severino, F. Fiorino, G. Aviello, R. G. De, M. Mazzella, B. Romano, F. Capasso and I. Fasolino, Neurogastroenterol. Motil., 2011, 23, 745 CrossRef CAS PubMed.
- E. Rey, A. Balboa and F. Mearin, Am. J. Gastroenterol., 2014, 109, 876–884 CrossRef PubMed.
- A. Emmanuel, M. Cools, L. Vandeplassche and R. Kerstens, Am. J. Gastroenterol., 2014, 109, 887–894 CrossRef CAS PubMed.
- L. Yina, C. K. Sunb, H. Xu, L. Xua, Y. Xua, Q. Yan and J. Penga, Food Chem., 2011, 129, 925–932 CrossRef PubMed.
- J. Choi, J. W. Kim, H. Cho, K. Kim, J. Lee, J. H. Sohn and K. U. Saekwang, Exp. Ther. Med., 2014, 8, 1847–1854 CrossRef PubMed.
- J. V. Castell, G. Friedrich, C. S. Kuhn and G. E. Poppe, Am. J. Physiol., 1997, 273, 139–146 Search PubMed.
- F. F. Liu, D. P. Chen, Y. J. Xiong, B. C. Lv and Y. Lin, Korean J. Physiol. Pharmacol., 2014, 18, 47–53 CrossRef CAS PubMed.
- Z. Y. Tang, Z. N. Liu, L. Fu, D. P. Chen, Q. D. Ai and Y. Lin, Physiol. Res., 2010, 59, 919–926 CAS.
- M. Schemann, J. Pediatr. Gastroenterol. Nutr., 2005, 41(suppl 1), S4–S6 CrossRef PubMed.
- L. Yin, C. Sun, X. Han, L. Xu, Y. Xu, Y. Qi and J. Peng, Food Chem., 2011, 129, 925–932 CrossRef CAS PubMed.
- E. K. Ji, S. H. Park, M. H. Kwak, J. Go, E. K. Koh, S. H. Song, E. S. Ji, H. S. Lee, T. H. Jin and D. Y. Hwang, PLoS One, 2015, 10, e0129664 Search PubMed.
- V. P. Zagorodnyuk, M. Kyloh, S. Nicholas, H. Peiris, S. J. Brookes, B. N. Chen and N. J. Spencer, J. Physiol., 2011, 589, 1691–1706 CrossRef CAS PubMed.
- Y. J. Xiong, H. W. Chu, Y. Lin, F. Han, Y. C. Li, A. G. Wang, F. J. Wang, D. P. Chen and J. Y. Wang, Acta Pharmacol. Sin., 2016, 37, 1091–1100 CrossRef CAS PubMed.
- L. Trudel, C. Tomasetto, M. C. Rio, M. Bouin, V. Plourde, P. Eberling and P. Poitras, Am. J. Physiol.: Gastrointest. Liver Physiol., 2002, 282, G948 CrossRef CAS PubMed.
- R. Mathison and E. Shaffer, BMC Gastroenterol., 2006, 6, 8 CrossRef PubMed.
- D. Chen, Y. Xiong, Y. Lin, Z. Tang, J. Wang, L. Wang and J. Yao, Mol. Nutr. Food Res., 2015, 59, 1482–1490 CAS.
- F. Nakao, S. Kobayashi, K. Mogami, Y. Mizukami, S. Shirao, S. Miwa, N. Todorokiikeda, M. Ito and M. Matsuzaki, Circ. Res., 2002, 91, 953–960 CrossRef CAS PubMed.
- N. Morikage, H. Kishi, M. Sato, F. Guo, S. Shirao, T. Yano, M. Soma, K. Hamano, K. Esato and S. Kobayashi, Circ. Res., 2006, 99, 299–306 CrossRef CAS PubMed.
- K. Bhui, S. Prasad, J. George and Y. Shukla, Cancer Lett., 2009, 282, 167–176 CrossRef CAS PubMed.
- D. Wu, X. Wang, J. Zhou, J. Yuan, B. Cui, R. An and Z. Hu, J. Ethnopharmacol., 2010, 130, 347–353 CrossRef PubMed.
- M. Li, C. P. Johnson, M. B. Adams and S. K. Sarna, Am. J. Physiol.: Gastrointest. Liver Physiol., 2002, 283, G544 CrossRef CAS PubMed.
- J. Shao, W. Sun, Y. Yao, R. X. Wang, X. Cao and D. O. Ophthalmology, Recent Adv. Ophthalmol., 2014, 38, 301–312 Search PubMed.
- S. Torihashi, T. Fujimoto, C. Trost and S. Nakayama, J. Biol. Chem., 2002, 277, 19191–19197 CrossRef CAS PubMed.
- J. Tack and T. K. Smith, Neurogastroenterol. Motil., 2004, 16(suppl 1), 86–95 CrossRef PubMed.
- W. He, Y. Peng, W. C. Zhang, N. Lv, J. Tang, C. Chen, C. Zhang, S. Gao, H. Chen, G. Zhi and R. Feil, Gastroenterology, 2008, 135, 610–620 CrossRef CAS PubMed.
- H. Maurer, Cell. Mol. Life Sci., 2001, 58, 1234–1245 CrossRef CAS PubMed.
- S. Kasapis and S. S. Sablani, Pure Appl. Chem., 2006, 77, 1923–1942 Search PubMed.
- G. Appendino, A. Minassi and O. Taglialatelascafati, Nat. Prod. Rep., 2014, 31, 880–904 RSC.
Footnotes |
† Electronic supplementary information (ESI) available. See DOI: 10.1039/c7ra06109a |
‡ These authors contributes equally to this work. |
|
This journal is © The Royal Society of Chemistry 2017 |
Click here to see how this site uses Cookies. View our privacy policy here.