DOI:
10.1039/C7RA06017C
(Paper)
RSC Adv., 2017,
7, 39502-39511
Highly efficient, one-pot synthesis of novel bis-spirooxindoles with skeletal diversity via sequential multi-component reaction in PEG-400 as a biodegradable solvent†
Received
29th May 2017
, Accepted 6th August 2017
First published on 11th August 2017
Abstract
A green and efficient one-pot, sequential, multi-component protocol has been developed for the synthesis of some novel symmetrical bis-spirooxindole derivatives from the reaction of isatins, dihalides, malono derivatives and C–H activated carbonyl compounds or ketene aminal derivatives in the presence of potassium carbonate (K2CO3) in polyethylene glycol 400 (PEG-400) as a biodegradable polymeric solvent at room temperature. In this research, a variety of bis-spiro-indoline-pyranopyranes, bis-spiro-indoline-chromenes, bis-spiro-indoline-pyrido-pyrimidines, bis-spiro-indoline-imidazo-pyridines and bis-spiro-indoline-pyridines were obtained with excellent yields within short reaction time and without chromatographic separation.
Introduction
Public concern over the effects of chemical release into the environment has grown steadily due to the potential threats to human health and indirect harm arising through disruptions of natural ecosystems.1 The introduction of green processes into chemistry has helped to reduce chemical related impact on human health and the environment.2 Since most organic reactions occur in the liquid phase, selection of solvent is one of the most active areas of research in green chemistry, because it makes up by far the greatest proportion of waste and a significant part of the hazard issues and energy intensity of a process.3,4 Green solvents should meet some basic requirements such as low toxicity, non-flammability, non-mutagenicity, non-volatility and widespread availability.5,6 Recently, polyethylene glycol (PEG) has been found to be a novel, powerful and interesting green solvent system.7,8 PEG covers many requirements of a green solvent such as thermal stability over a wide range of temperatures, recovery, non-explosiveness, commercial availability and non-toxicity.8,9 PEGs are readily soluble in polar solvents such as water, DMF and methanol but are insoluble in non-polar solvents such as diethyl ether and n-hexane; this unequalled property permits the recovery of PEGs by precipitation and filtration, which is extremely significant in soluble polymer-supported chemistry. Furthermore, PEG is a biological polymer that has been used widely in bio-conjugates as tools for diagnostics and in drug delivery.10,11
Development of efficient chemical reaction sequences in green media that provide a molecular diversity and structural complexity with a minimum number of synthetic steps could be major challenge.12 As a solution for these challenges, multi-component reactions (MCRs) are considered to be one of the most efficient strategies in modern organic synthesis and employing green solvents may influence the course of reaction, yields, selectivity and the reaction rates.13 MCRs are well known for their simplicity of operation, reduction of isolation and purification steps, and minimization of energy, time, costs, and waste production.14–17 The efforts to combine the advantages of MCRs and eco-friendly solvents through conducting MCRs in such solvents has been comprehensively reviewed.18 Conducting MCRs in water,19 ionic liquids,20 polyethylene glycol (PEG),21 super critical carbon dioxide (scCO2),22 bio-derived solvents,23 perfluorinated solvents24 and neat systems25 has attracted more attention in recent decade.
Isatin and bis-isatin spiro derivatives (including bis-spirooxindoles) have been synthesized using different methodologies and have been utilized successfully in many biological processes as antimicrobial, anticancer and enzyme inhibitor agents.26–28 However, many of the synthetic routes toward bis-spirooxindoles include the use of toxic solvents and catalysts or they have been conducted in harsh conditions like refluxing for long times.29–31 Furthermore, biological studies demonstrate that the presence of an aliphatic chain in a molecule decreases the polarity and lead to increases in lipophilicity of the compound. Therefore, this property enables the compound to cross the biological membrane which cause increasing the biological activity of the compound. On the basis of the above hypothesis and according to the biological activity of spirooxindoles,32 we would like to report an efficient one-pot, multi-component synthesis of novel bis-spirooxindole derivatives in green medium (Scheme 1).
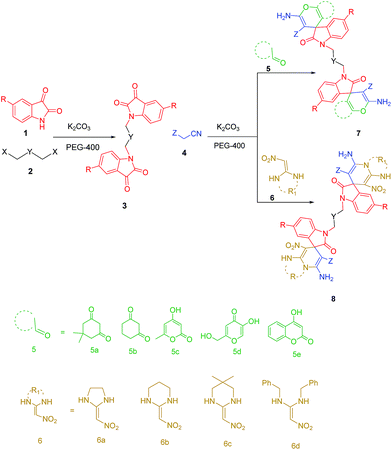 |
| Scheme 1 The one-pot, multi-component synthesis of bis-spirooxindoles in PEG-400/K2CO3 at room temperature. | |
Results and discussion
Initially, the sequential condensation of isatin 1a (2 mmol), 1,6-dibromohexane 2a (1 mmol), malononitrile 4a (2 mmol) and dimedone 5a (2 mmol) (Scheme 2) was investigated to find an appropriate reaction medium for the synthesis of bis-spirooxindoles. Different solvents, catalysts and reaction temperatures were explored in this investigation. Water, methanol, ethanol, acetonitrile, DMF, DMSO and PEG-400 were examined as solvent and the effects of temperature, the type and the amount of catalyst have been checked (Table 1). After this vast study, the results have depicted that the highest value for yield of desired bis-spirooxindole has been obtained in PEG-400 in the presence of 2 mmol of K2CO3 at room temperature. Initially, this reaction was carried out in PEG-400 without any catalyst at room temperature. It was observed that the reaction did not proceed even after 24 hours (Table 1, entry 1). Different catalysts including L-proline, DABCO, DBU, NaHCO3, Na2CO3, NaOH, CaCO3, Cs2CO3, KOH and K2CO3 in PEG-400 at room temperature were tested (Table 1, entries 2–11) and K2CO3 was the best catalyst for this transformation (Table 1, entry 11). Increasing the amount of K2CO3 did not improve the reaction results whereas; decreasing the amount of catalyst decreased the reaction yield (compare entries 11–13 Table 1). Increasing of the reaction temperature did not affect the reaction process (for comparison see Table 1, entries 11, 14 and 15). The model reaction was also examined in the presence of K2CO3 in other solvents including H2O, MeOH, EtOH, CH3CN, DMF and DMSO. In protic solvents (H2O, EtOH, and MeOH) the reaction did not proceed at all, whereas change of solvent from protic to aprotic (CH3CN, DMF, DMSO) resulted in the formation of the desired product. This observations could be related to the solubility of starting materials and activation of catalyst in aprotic solvents. The reaction was also tested in neat conditions in the presence of K2CO3 at different temperatures but did not proceed even after 24 h (Table 1, entries 22, 23). The reason behind this performance of PEG-400 could be related to its unique property in solving and gathering reactants and the catalyst together to react more efficiently in comparison to other solvents.33
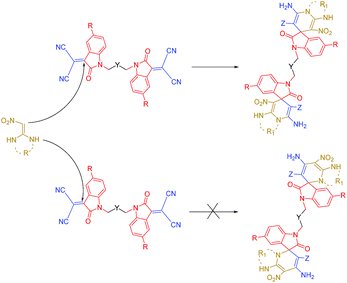 |
| Scheme 2 Regioselectivity in ketene aminal reactions with bis-isatin-malononitrile aggregate. | |
Table 1 Synthesis of bis-spiroindoline chromene 7a through one-pot sequential condensation reaction between isatin 1a (2 mmol), 1,6-dibromohexane 2a (1 mmol), malononitrile 4a (2 mmol) and dimedone 5a (2 mmol) using different amounts of basic catalysts in various solvents and temperatures
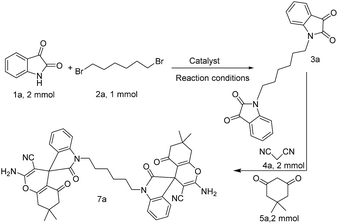
|
Entry |
Catalyst (mmol) |
Solvent |
Temperature (°C) |
Time (h) |
Yielda (%) |
Isolated yield. |
1 |
— |
PEG-400 |
r.t. |
24 |
— |
2 |
L-Proline (2) |
PEG-400 |
r.t. |
24 |
— |
3 |
DABCO (2) |
PEG-400 |
r.t. |
24 |
— |
4 |
DBU (2) |
PEG-400 |
r.t. |
24 |
— |
5 |
NaHCO3 (2) |
PEG-400 |
r.t. |
24 |
Trace |
6 |
Na2CO3 (2) |
PEG-400 |
r.t. |
24 |
42 |
7 |
NaOH (2) |
PEG-400 |
r.t. |
24 |
— |
8 |
CaCO3 (2) |
PEG-400 |
r.t. |
24 |
30 |
9 |
Cs2CO3 (2) |
PEG-400 |
r.t. |
24 |
73 |
10 |
KOH (2) |
PEG-400 |
r.t. |
24 |
— |
11 |
K2CO3 (2) |
PEG-400 |
r.t. |
9 |
91 |
12 |
K2CO3 (1.5) |
PEG-400 |
r.t. |
12 |
80 |
13 |
K2CO3 (3) |
PEG-400 |
r.t. |
9 |
91 |
14 |
K2CO3 (2) |
PEG-400 |
50 |
9 |
90 |
15 |
K2CO3 (2) |
PEG-400 |
80 |
9 |
91 |
16 |
K2CO3 (2) |
H2O |
r.t. |
24 |
— |
17 |
K2CO3 (2) |
MeOH |
r.t. |
24 |
— |
18 |
K2CO3 (2) |
EtOH |
r.t. |
24 |
— |
19 |
K2CO3 (2) |
CH3CN |
r.t. |
24 |
Trace |
20 |
K2CO3 (2) |
DMF |
r.t. |
18 |
80 |
21 |
K2CO3 (2) |
DMSO |
r.t. |
15 |
85 |
22 |
K2CO3 (2) |
— |
r.t. |
24 |
— |
23 |
K2CO3 (2) |
— |
100 |
24 |
— |
On the basis of biological studies, the existence of two or more different heterocyclic moieties in a single molecule often enhances the biological activity dramatically.34,35 A few synthetic methods are available for the synthesis of bis-spirooxindoles derivatives. However, survey of the literature data show that the majority of these methods suffer from one or more drawbacks, such as multistep sequences, toxic solvents like DMSO and DMF, application of hazardous and toxic agents like NaH and long reaction times.29–31,36 Considering the aforementioned drawbacks, producing of N-alkyl-linked bis-isatins and then obtaining the corresponding bis-spirooxindole compounds in our introduced reaction model have merits such as green solvent, non-toxic reagent, room temperature conditions, low cost, and easy work-up.
Having found the basic conditions for model reaction (PEG-400/K2CO3), we then directed our attention toward the reaction scope for the synthesis of bis-spirooxindoles and the results have been shown in Table 2.
Table 2 One-pot, multi-component synthesis of bis-spirooxindoles (7a–7u) in the presence of K2CO3 in PEG-400 at room temperature
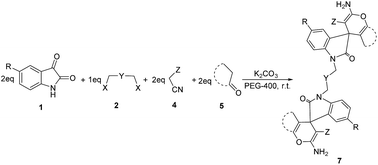
|
Entry |
R |
Y |
X |
Z |
Ketone |
Product |
Time (h) |
Yielda (%) |
Isolated yield. |
1 |
H |
(CH2)4 |
Br |
CN |
5a |
7a |
9 |
91 |
2 |
H |
(CH2)4 |
Br |
CO2Me |
5a |
7b |
10 |
90 |
3 |
H |
(CH2)4 |
Br |
CO2Et |
5a |
7c |
12 |
88 |
4 |
Me |
(CH2)4 |
Br |
CO2Me |
5d |
7d |
12 |
89 |
5 |
Me |
(CH2)4 |
Br |
CO2Et |
5c |
7e |
12 |
90 |
6 |
Br |
(CH2)4 |
Br |
CO2Et |
5b |
7f |
11 |
90 |
7 |
Br |
(CH2)4 |
Br |
CN |
5a |
7g |
9 |
93 |
8 |
Me |
(CH2)4 |
Br |
CO2Me |
5c |
7h |
11.5 |
89 |
9 |
F |
(CH2)4 |
Br |
CO2Me |
5c |
7i |
9 |
92 |
10 |
H |
(CH2)2 |
Br |
CO2Me |
5e |
7j |
10 |
90 |
11 |
Me |
(CH2)4 |
Br |
CN |
5a |
7k |
9 |
89 |
12 |
H |
(CH2)4 |
Br |
CO2Me |
5b |
7l |
10 |
90 |
13 |
H |
(CH2)4 |
Br |
CO2Et |
5d |
7m |
8 |
89 |
14 |
H |
(CH2)2 |
Br |
CO2Et |
5c |
7n |
9 |
88 |
15 |
H |
(CH2)2 |
Br |
CO2Me |
5b |
7o |
10 |
90 |
16 |
Br |
(p-C6H4) |
Cl |
CN |
5a |
7p |
8 |
95 |
17 |
Br |
(p-C6H4) |
Cl |
CO2Et |
5b |
7q |
8 |
90 |
18 |
Br |
(p-C6H4) |
Cl |
CN |
5b |
7r |
9 |
90 |
19 |
Me |
(p-C6H4) |
Cl |
CO2Et |
5a |
7s |
10 |
88 |
20 |
H |
(p-C6H4) |
Cl |
CO2Et |
5a |
7t |
10 |
90 |
21 |
H |
(p-C6H4) |
Cl |
CN |
5a |
7u |
9 |
91 |
As shown in Table 2, all reactions proceeded efficiently, affording the corresponding products in good to excellent yields. Considering different substitutions on starting isatins, malono-derivatives and enolizable ketones, the obtained compounds are representative for all possible 180 possible combinations.
It is well known that the presence of indole substructure in heterocyclic compounds such as spirooxindloes, makes them biologically active.37–39 Spirooxindoles are fascinating cores that can be found in many natural active compounds with antiproliferative, antibacterial, antifungal and antiviral activities.40 Therefore, as a future plan, it is worthy to evaluate the effect of the additional spiro core on the biological activity of the obtained bis-spiro compounds by comparing with mono-spiro activities.
Ketene aminals (KAs) are powerful and versatile intermediates in heterocyclic synthesis. Reactions of ketene aminals with a number of bis-electrophilic reagents have been successfully used to give five- and six-membered and fused heterocyclic compounds during the past years.41 Ketene aminals were synthesized according to literature.21,42 Briefly, 1,1-bis(methylthio)-2-nitroethylene (1 mmol) and diamines (1 mmol) or primary amines (2 mmol) have been reacted in EtOH at reflux for 2–4 h. The precipitate has been filtered and washed by cold ethanol (82–95% yield) (Table 3).
Table 3 Synthesis of ketene aminal derivatives
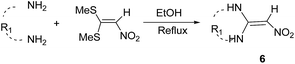
|
Entry |
R1 |
Product |
Time (h) |
Yielda (%) |
Isolated yield. |
1 |
–(CH2)2– |
6a |
2.5 |
82 |
2 |
–(CH2)3– |
6b |
4 |
93 |
3 |
–CH2C(CH3)2CH2– |
6c |
2.5 |
94 |
4 |
PhCH2– |
6d |
2 |
95 |
Subsequently, we expanded the scope of the performed condensation reaction by investigating the use of ketene aminals as alternatives for enolizable ketones. Under the same conditions for model reaction, the condensation reaction of isatins, dihalides, malono derivatives and ketene aminals in the presence of K2CO3 in PEG-400 at room temperature have been conducted, affording the corresponding products 8a–8n in good yields and in short reaction times (Table 4).
Table 4 One-pot, multi-component synthesis of bis-spirooxindole derivatives (8a–8n) in the presence of K2CO3 in PEG-400 at room temperature
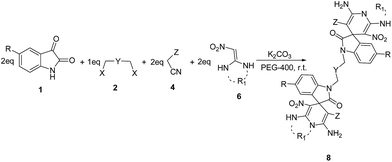
|
Entry |
R |
Y |
X |
Z |
Ketene aminal |
Product |
Time (h) |
Yielda (%) |
Isolated yield. |
1 |
H |
(CH2)2 |
Br |
CN |
6a |
8a |
11 |
73 |
2 |
H |
(CH2)4 |
Br |
CN |
6b |
8b |
12 |
74 |
3 |
H |
(p-C6H4) |
Cl |
CN |
6c |
8c |
11 |
69 |
4 |
NO2 |
(CH2)2 |
Br |
CN |
6b |
8d |
13 |
73 |
5 |
NO2 |
(CH2)4 |
Br |
CN |
6d |
8e |
10 |
75 |
6 |
NO2 |
(p-C6H4) |
Cl |
CN |
6a |
8f |
10 |
70 |
7 |
CH3 |
(CH2)4 |
Br |
CN |
6c |
8g |
9 |
72 |
8 |
CH3 |
(o-C6H4) |
Br |
CN |
6a |
8h |
11 |
69 |
9 |
CH3 |
(p-C6H4) |
Cl |
CN |
6b |
8i |
9 |
71 |
10 |
F |
(CH2)2 |
Br |
CN |
8b |
8j |
12 |
67 |
11 |
F |
(p-C6H4) |
Cl |
CN |
6d |
8k |
10 |
69 |
12 |
Br |
(CH2)4 |
Br |
CN |
6a |
8l |
12 |
72 |
13 |
Br |
(o-C6H4) |
Br |
CN |
6c |
8m |
13 |
71 |
14 |
Br |
(o-C6H4) |
Cl |
CN |
6d |
8n |
10 |
77 |
As shown in Table 4, different types of alkyl dihalides, which some of them have aromatic ring in their structure, have been effectively utilized as linker between two isatin molecules. Also isatins with electron withdrawing and electron donating substitutions on their aromatic ring have been successfully used for synthesis of these complex structures. It turned out that the electronic effects of substitution on aromatic ring of isatin has negligibly affected the time and yield of bis-spirooxindole. Various aliphatic cyclic and acyclic ketene aminals were successfully utilized to prepare the corresponding bis-spiro-indoline-pyrido-pyrimidines, bis-spiro-indoline-imidazo-pyridines and bis-spiro-indoline-pyridines derivatives in high yields. Noteworthy, as a result of the greater nucleophilicity of the α-carbon atom in comparison with nitrogen atom,43 attack of α-carbon leads to the reaction between ketene aminals (6a–6d) and bis-isatin-malononitrile aggregate to form the corresponding spiro-dihydropyridine ring in the target compounds (8a–8n) in moderate to good yields (67–75%) (upper pathway of Scheme 2). Recent studies on mono-spiro counterparts of bis-spirooxindoles that contain dihydropyridine rings have approved their ability in inhibition of acetyl cholinesterase and butyryl cholinesterase enzymes which are well known for they role in Alzheimer disease that it makes these bis-spiro molecules an attractive subjects of further biological investigation.21
It should be mentioned that the reaction failed when ethyl-2-cyanoacetate and methyl-2-cyanoacetate were used instead of malononitrile which could be related to lower tendency of ketene aminals to undergo condensation reactions when compared to enolizable ketones.
A plausible mechanism for the synthesis of bis-spirooxindole derivatives 7 and 8 in the presence of K2CO3 is shown on Scheme 3. The first step involves the formation of product 3 from condensation reaction between 2 eq. of isatin 1 with 1 eq. dihalide 2. In the PEG-400/K2CO3 system, because of the coordination of the cationic centre of K2CO3 with the oxygen of polyethylene glycol, the CO32− anion could be presented in solution44 and the reaction of CO32− anion with isatin derivatives was elevated by increasing the nucleophilicity of nitrogen atom to attack to dihalide. Then, fast Knoevenagel condensation occurs between 3 and acidic protons of compound 4 for the formation of olefin A. In the presence of K2CO3, ketene aminal 6 converts to its corresponding enolate, which easily reacts with olefin A to produce intermediate B, which followed by proton transfer and tautomerization to form the desired product 8.
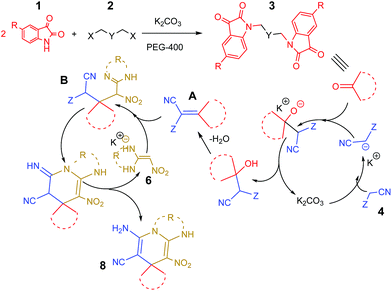 |
| Scheme 3 The proposed mechanism for the synthesis of bis-spirooxindole in the presence of K2CO3 in PEG-400. | |
In another study, reusability of PEG and K2CO3 were studied. For this propose, the reaction of isatin 1a (2 mmol) with 1,6-dibromohexane 2a (1 mmol), malononitrile 4a (2 mmol) and dimedone 5a (2 mmol) was performed in the presence of K2CO3 (2 mmol) in PEG-400 (3 mL) at room temperature. After completion of the reaction, as monitored by TLC, water (3 mL) was added to the reaction mixture to precipitate the product and separate it. After isolation of product, water was removed by direct distillation at 100 °C and the mixture of PEG-400 and K2CO3 was washed with diethyl ether (two times, every time with 2 mL). The regenerated PEG and K2CO3 were successfully used for the next run under identical reaction conditions. The reusability results are summarized in Chart 1. As Chart 1 indicates, PEG/K2CO3 system was successfully recycled and reused in four cycles with almost unchanged yields (Chart 1).
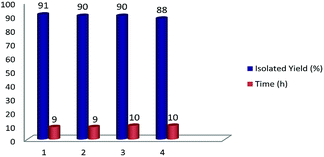 |
| Chart 1 The reaction of: isatin 1a (2 mmol) with 1,6-dibromohexane 2a (1 mmol), malononitrile 4a (2 mmol) and dimedone 5a (2 mmol) in the presence of recovered PEG-400/K2CO3 (2 mmol) at room temperature. | |
Experimental
All reagents were purchased from commercial suppliers and used without any further purification. All the reactions were carried out under air atmosphere. H and C NMR spectra were recorded on a Bruker Avance (400 and 100 MHz) NMR spectrometer in DMSO as solvent. The following abbreviations are used to indicate the multiplicity in NMR spectra: s—singlet; d—doublet; t—triplet; q—quartet; quint.—quintet; dd—double doublet; m—multiplet; br—broad signal and coupling constants (J values) in hertz (Hz). Melting points were recorded on a Büchi B-545 apparatus in open capillary tubes. Elemental analyses were performed by using a Leco Analyzer 932 (Leco Corp., St. Joseph, MI).
General procedure for the synthesis of ketene aminals
A solution of bis(methylthio)-2-nitroethylene (1 mmol) and diamine derivatives (1 mmol) or benzyl amine (2 mmol) were added in a 25 mL round-bottomed flask contained ethanol (4.0 mL), and the resulting mixture was reflux at 80 °C. After completion of the reaction confirmed by TLC, the reaction mixture was cooled to room temperature. Then, the precipitated product was filtered, and washed with cool ethanol (two times, every time with 4 mL) to give the pure product.
General procedure for the synthesis of bis-spirooxindole derivatives
A solution of isatin derivates (2 mmol) and K2CO3 (276 mg, 2 mmol) in PEG (3 mL) was stirred at room temperature. After 2 h, di-halide derivates (1 mmol) was added, and the mixture was stirred for 6 h at room temperature. After completion of the reaction confirmed by TLC, malono derivative (2 mmol) and enolizable carbonyl compound (5a–5d, 2 mmol) or ketene aminal derivative (6a–6d, 2 mmol) were added to the reaction mixture. After completion of the reaction, 3 mL of water was added to the reaction mixture; the precipitate was filtrated and washed with ethanol (two times, every time with 5 mL) to give the pure product. The recovered PEG-400 and K2CO3 were used for another time without loss of its activity.
1′,1′′′-(Hexane-1,6-diyl)bis(2-amino-7,7-dimethyl-2′,5-dioxo-5,6,7,8-tetrahydrospiro[chromene-4,3′-indoline]-3-carbonitrile) (7a). Cream solid; mp > 290 °C; 1H NMR (400 MHz, DMSO-d6): δ (ppm) = 1.02 (s, 6H), 1.04 (s, 6H), 1.41–1.44 (m, 4H), 1.59–1.63 (m, 4H), 2.09–2.19 (m, 4H), 3.04 (s, 4H), 3.59–3.67 (m, 4H), 6.86–6.88 (m, 4H), 7.02–7.04 (m, 2H), 7.25 (br, 4H, NH2); 13C NMR (100 MHz, DMSO-d6): δ = 26.5, 27.5, 28.1, 32.4, 45.2, 46.8, 50.4, 57.7, 108.8, 111.2, 117.7, 122.6, 123.4, 128.8, 134.2, 143.5, 159.3, 164.7, 176.8, 195.3.
Dimethyl-1′,1′′′-(hexane-1,6-diyl)bis(2-amino-7,7-dimethyl-2′,5-dioxo-5,6,7,8-tetrahydrospiro[chromene-4,3′-indoline]-3-carboxylate) (7b). Cream solid; mp > 290 °C; 1H NMR (400 MHz, DMSO-d6): δ (ppm) = 0.94 (s, 6H), 1.01 (s, 6H), 1.26 (s, 4H), 1.50–1.54 (m, 4H), 1.66–1.68 (m, 4H), 1.97–2.01 (m, 2H), 2.13–2.17 (m, 2H), 3.21 (s, 6H), 3.61–3.63 (m, 4H), 6.86–6.90 (m, 6H), 7.13 (s, 2H), 7.87 (br, 4H, NH2); 13C NMR (100 MHz, DMSO-d6): δ = 20.0, 27.5, 31.2, 37.4, 44.3, 47.0, 59.2, 76.2, 108.7, 109.9, 113.7, 125.7, 128.2, 130.3, 136.0, 138.3, 144.7, 150.2, 159.7, 165.8, 167.7, 178.7, 196.4.
Diethyl-1′,1′′′-(hexane-1,6-diyl)bis(2-amino-7,7-dimethyl-2′,5-dioxo-5,6,7,8-tetrahydrospiro[chromene-4,3′-indoline]-3-carboxylate) (7c). Cream solid; mp > 290 °C; 1H NMR (400 MHz, DMSO-d6): δ = 0.68–0.72 (m, 6H), 0.93–0.97 (s, 6H), 1.02 (s, 6H), 1.26 (s, 4H), 1.50–1.52 (m, 4H), 1.67–1.69 (m, 4H), 2.02–2.13 (m, 4H), 3.35–3.67 (m, 4H), 3.71–3.73 (m, 4H), 6.86–6.91 (m, 6H), 7.14 (s, 2H), 7.92 (br, 4H, NH2); 13C NMR (100 MHz, DMSO-d6): δ = 14.0, 19.6, 26.7, 27.2, 27.4, 31.2, 44.6, 46.5, 50.9, 53.4, 59.3, 75.9, 101.2, 107.7, 128.4, 134.3, 145.4, 158.4, 159.4, 159.5, 159.9, 163.7, 167.8, 177.5.
Dimethyl-1,1′′-(hexane-1,6-diyl)bis(2′-amino-6′-(hydroxymethyl)-5-methyl-2,8′-dioxo-8′H-spiro[indoline-3,4′-pyrano[3,2-b]pyran]-3′-carboxylate) (7d). Cream solid; mp > 290 °C; 1H NMR (400 MHz, DMSO-d6): δ = 1.44–1.48 (m, 4H), 1.64–1.66 (m, 4H), 2.23 (s, 6H), 3.25 (s 6H), 3.67–3.73 (m, 4H), 3.93–4.06 (m, 4H), 5.64 (br, 2H, OH), 6.33 (s, 2H), 6.96–7.01 (m, 4H), 7.07–7.09 (m, 2H), 8.11 (br, 4H, NH2); 13C NMR (100 MHz, DMSO-d6): δ = 26.2, 27.2, 31.2, 45.1, 51.1, 59.4, 73.9, 73.9, 108.7, 111.8, 124.4, 129.8, 131.9, 133.5, 136.8, 141.4, 148.3, 160.4, 167.5, 168.8, 169.8, 175.7.
Diethyl-1,1′′-(hexane-1,6-diyl)bis(2′-amino-5,7′-dimethyl-2,5′-dioxo-5′H-spiro[indoline-3,4′-pyrano[4,3-b]pyran]-3′-carboxylate) (7e). Cream solid; mp > 290 °C; 1H NMR (400 MHz, DMSO-d6): δ = 0.73 (t, J = 8 Hz, 6H), 1.46–1.47 (m, 4H), 1.63–1.65 (m, 4H), 2.20 (s, 6H), 2.21 (s, 6H) 3.45–3.52 (m, 2H), 3.63–3.68 (m, 2H), 3.70–3.75 (m, 4H), 6.31 (s, 2H), 6.78–6.82 (m, 4H), 6.98 (d, J = 8 Hz, 2H), 8.07 (br, 4H, NH2); 13C NMR (100 MHz, DMSO-d6): δ = 14.0, 19.6, 21.0, 26.8, 27.2, 44.6, 46.6, 59.3, 75.9, 98.1, 101.3, 107.4, 123.9, 128.5, 130.7, 134.5, 143.1, 159.4, 159.5, 159.9, 163.6, 167.7, 177.4.
Diethyl-1′,1′′′-(hexane-1,6-diyl)bis(2-amino-5′-bromo-2′,5-dioxo-5,6,7,8-tetrahydrospiro[chromene-4,3′-indoline]-3-carboxylate) (7f). Cream solid; mp > 290 °C; 1H NMR (400 MHz, DMSO-d6): δ = 0.71–0.74 (m, 6H), 1.47–1.49 (m, 4H), 1.64–1.66 (m, 4H), 1.88–1.89 (m, 4H), 2.17–2.22 (m, 4H), 2.64–2.67 (m, 4H), 3.47–3.50 (m, 2H), 3.62–3.65 (m, 2H), 3.67–3.77 (m, 4H), 6.84–6.87 (m, 2H), 7.12–7.13 (m, 2H), 7.29–7.33 (m, 2H), 7.99 (br, 4H, NH2); 13C NMR (100 MHz, DMSO-d6): δ = 14.1, 20.0, 26.7, 27.0, 27.4, 37.4, 44.6, 46.84, 59.2, 76.0, 109.3, 113.2, 113.9, 125.7, 130.4, 138.3, 144.8, 159.7, 165.4, 167.8, 178.1, 195.5.
1′,1′′′-(Hexane-1,6-diyl)bis(2-amino-5′-bromo-7,7-dimethyl-2′,5-dioxo-5,6,7,8-tetrahydrospiro[chromene-4,3′-indoline]-3-carbonitrile) (7g). White solid, mp > 290 °C; 1H NMR (400 MHz, DMSO-d6): δ = 1.02 (s, 6H), 1.07 (s, 6H), 1.40–1.42 (m, 4H), 1.58–1.60 (m, 4H), 2.13–1.16 (m, 4H), 2.59 (s, 4H), 2.64 (s, 4H), 3.57–3.71 (m, 4H), 6.97–6.99 (m, 2H), 7.29–7.30 (m, 2H), 7.39 (br, 4H, NH2); 13C NMR (100 MHz, DMSO-d6): δ = 26.3, 27.1, 27.7, 28.0, 32.5, 47.0, 50.0, 57.0, 61.5, 110.6, 110.8, 114.4, 118.9, 126.3, 131.5, 136.6, 142.9, 148.3, 155.2, 159.4, 165.2, 176.5, 195.6.
Dimethyl-1,1′′-(hexane-1,6-diyl)bis(2′-amino-5,7′-dimethyl-2,5′-dioxo-5′H-spiro[indoline-3,4′-pyrano[4,3-b]pyran]-3′-carboxylate) (7h). Light brown solid, mp > 290 °C; 1H NMR (400 MHz, DMSO-d6): δ = 1.47–1.49 (m, 4H), 1.61–1.64 (m, 4H), 2.20 (s, 6H), 2.21 (s, 6H), 3.24 (s, 6H), 3.60–3.72 (m, 4H), 6.31 (s, 2H), 6.79–6.81 (m, 4H), 6.96–98 (m, 2H), 8.02 (br, 4H, NH2); 13C NMR (100 MHz, DMSO-d6): δ = 19.6, 21.0, 26.7, 27.2, 27.4, 44.5, 46.6, 50.9, 59.3, 76.0, 101.3, 107.5, 123.9, 128.6, 130.7, 134.3, 142.9, 158.8, 159.4, 163.6, 167.859, 177.4.
Dimethyl-1,1′′-(hexane-1,6-diyl)bis(2′-amino-5-fluoro-7′-methyl-2,5′-dioxo-5′H-spiro[indoline-3,4′-pyrano[4,3-b]pyran]-3′-carboxylate) (7i). White solid, mp > 290 °C; 1H NMR (400 MHz, DMSO-d6): δ = 1.48–1.50 (m, 4H), 1.64–1.66 (m, 4H), 2.21 (s, 6H), 3.23 (s, 6H), 3.52–3.74 (m, 4H), 6.32 (s, 2H), 6.86–6.93 (m, 2H), 6.99–7.01 (m, 2H), 7.16–7.20 (m, 2H), 8.03 (br, 4H, NH2); 13C NMR (100 MHz, DMSO-d6): δ = 19.9, 27.0, 27.4, 27.5, 44.6, 46.4, 51.1, 59.1, 75.9, 101.4, 107.9, 124.1, 128.6, 130.7, 134.5, 143.1, 158.6, 159.4, 163.6, 167.9, 177.5.
Dimethyl-1,1′′-(butane-1,4-diyl)bis(2′-amino-2,5′-dioxo-5′H-spiro[indoline-3,4′-pyrano[3,2-c]chromene]-3′-carboxylate) (7j). Light brown solid, mp > 290 °C, 1H NMR (400 MHz, DMSO-d6): δ = 1.77–1.85 (m, 4H), 3.05 (s, 6H), 3.66–3.83 (m, 4H), 6.82–6.90 (m, 2H), 7.02–7.25 (m, 6H), 7.48 (d, J = 8 Hz, 2H), 7.54–7.57 (m, 2H), 7.75–7.84 (m, 2H), 8.07 (d, J = 8 Hz, 2H), 8.21 (br, 4H, NH2); 13C NMR (100 MHz, DMSO-d6): δ = 31.2, 49.0, 58.3, 113.0, 116.0, 117.3, 121.3, 123.4, 125.7, 126.9, 127.9, 128.2, 130.7, 134.5, 135.4, 137.1, 137.4, 147.4, 152.6, 155.4, 158.5, 159.1, 162.4.
1′,1′′′-(Hexane-1,6-diyl)bis(2-amino-5′,7,7-trimethyl-2′,5-dioxo-5,6,7,8-tetrahydrospiro[chromene-4,3′-indoline]-3-carbonitrile) (7k). Cream solid, mp > 290 °C; 1H NMR (400 MHz, DMSO-d6): δ = 1.02 (s, 6H), 1.04 (s, 6H), 1.41–1.44 (m, 4H), 1.59–1.63 (m, 4H), 2.09–2.19 (m, 4H), 2.25 (s, 6H), 3.04 (s, 4H), 3.59–3.67 (m, 4H), 6.86–6.88 (m, 4H), 7.02–7.05 (m, 2H), 7.45 (br, 4H, NH2); 13C NMR (100 MHz, DMSO-d6): δ = 21.1, 26.5, 27.2, 27.7, 27.9, 32.4, 44.5, 46.9, 50.4, 57.9, 108.5, 111.3, 117.7, 124.0, 129.0, 131.5, 134.3, 141.1, 159.2, 159.3, 164.6, 176.7, 195.3.
Dimethyl-1′,1′′′-(hexane-1,6-diyl)bis(2-amino-2′,5-dioxo-5,6,7,8-tetrahydrospiro[chromene-4,3′-indoline]-3-carboxylate) (7l). Cream solid, mp > 290 °C; 1H NMR (400 MHz, DMSO-d6): δ = 1.50–1.52 (m, 4H), 1.66–1.68 (m, 4H), 1.86–1.88 (m, 4H), 2.11 (s, 6H), 2.64–2.66 (m, 4H), 3.19–3.21 (m, 4H), 3.62–3.71 (m, 4H), 6.82–6.85 (m, 4H), 6.91–6.93 (d, J = 7 Hz, 2H), 7.11–7.14 (m, 2H), 7.86 (r, 4H, NH2); 13C NMR (100 MHz, DMSO-d6): δ = 14.1, 20.1, 26.8, 27.4, 31.2, 37.4, 46.7, 50.7, 76.6, 107.3, 114.6, 121.5, 122.8, 127.9, 135.6, 145.2, 159.5, 164.9, 168.1, 178.5, 195.3.
Diethyl-1,1′′-(hexane-1,6-diyl)bis(2′-amino-6′-(hydroxymethyl)-2,8′-dioxo-8′H-spiro[indoline-3,4′-pyrano[3,2-b]pyran]-3′-carboxylate) (7m). Light brown solid, mp > 290 °C; 1H NMR (400 MHz, DMSO-d6): δ = 0.68–0.72 (m, 6H), 1.43–1.45 (m, 4H), 1.64–1.66 (m, 4H), 3.57–3.70 (m, 4H), 3.71–3.85 (m, 4H), 3.97–3.98 (m, 4H), 5.63 (t, J = 6.4 Hz, 2H, OH), 6.35 (s, 2H), 7.08–7.10 (m, 2H), 7.49–7.50 (m, 4H), 8.20 (br, 4H, NH2); 13C NMR (100 MHz, DMSO-d6): δ = 14.0, 26.2, 27.2, 44.0, 51.2, 59.4, 73.3, 80.0, 110.0, 111.9, 114.7, 126.9, 132.2, 135.7, 137.1, 143.2, 147.2, 160.6, 167.2, 168.8, 169.9, 175.7.
Diethyl-1,1′′-(butane-1,4-diyl)bis(2′-amino-7′-methyl-2,5′-dioxo-5′H-spiro[indoline-3,4′-pyrano[4,3-b]pyran]-3′-carboxylate) (7n). Cream solid, mp > 290 °C; 1H NMR (400 MHz, DMSO-d6): δ = 0.63–0.68 (m, 6H), 1.80 (m, 4H), 2.23 (s, 6H), 3.59–3.66 (m, 4H) 3.73–3.80 (m, 4H), 6.34 (s, 2H), 6.86–6.90 (m, 2H), 6.98–7.02 (m, 4H), 7.14–7.20 (m, 2H), 8.07 (br, 4H, NH2); 13C NMR (100 MHz, DMSO-d6): δ = 14.0, 19.7, 25.2, 46.6, 59.2, 75.8, 98.2, 101.2, 107.9, 121.9, 123.2, 128.3, 134.6, 145.4, 158.8, 159.5, 160.0, 163.7, 167.7, 177.7.
Dimethyl-1′,1′′′-(butane-1,4-diyl)bis(2-amino-2′,5-dioxo-5,6,7,8-tetrahydrospiro[chromene-4,3′-indoline]-3-carboxylate) (7o). White solid, mp > 290 °C; 1H NMR (400 MHz, DMSO-d6): δ = 1.78–1.80 (m, 4H), 1.87–1.89 (m, 4H), 2.15–2.24 (m, 4H), 2.66–2.68 (m, 4H), 3.19 (s, 6H), 3.70–3.75 (m, 4H), 6.81–6.86 (m, 2H), 6.91–6.95 (m, 4H), 7.09–7.14 (m, 2H), 7.89 (br, 4H, NH2); 13C NMR (100 MHz, DMSO-d6): δ = 20.2, 26.1, 28.3, 38.0, 43.2, 45.3, 59.2, 75.8, 107.1, 113.1, 114.0, 124.9, 130.4, 138.3, 145.1, 159.7, 165.3, 168.2, 178.2, 194.9.
1′,1′′′-(1,4-Phenylenebis(methylene))bis(2-amino-5′-bromo-7,7-dimethyl-2′,5-dioxo-5,6,7,8-tetrahydrospiro[chromene-4,3′-indoline]-3-carbonitrile) (7p). Cream solid, mp > 290 °C; 1H NMR (400 MHz, DMSO-d6): δ = 1.04 (s, 6H), 1.06 (s, 6H), 2.21 (s, 4H), 2.60–2.68 (m, 4H), 4.91 (s, 4H), 6.67–6.71 (m, 2H), 7.34–7.36 (m, 4H), 7.43 (s, 4H), 7.46 (br, 4H, NH2); 13C NMR (100 MHz, DMSO-d6): δ = 27.5, 27.7, 28.0, 31.2, 32.6, 43.6, 47.2, 50.3, 56.9, 110.5, 111.4, 114.9, 117.8, 126.4, 127.6, 131.5, 135.2, 136.4, 142.4, 159.5, 165.5, 176.8, 195.8.
Diethyl-1′,1′′′-(1,4-phenylenebis(methylene))bis(2-amino-5′-bromo-2′,5-dioxo-5,6,7,8-tetrahydrospiro[chromene-4,3′-indoline]-3-carboxylate) (7q). Cream solid, mp > 290 °C; 1H NMR (400 MHz, DMSO-d6): δ = 0.54–0.62 (m, 6H), 1.91–1.94 (m, 4H), 2.21–2.35 (m, 4H), 2.68–2.70 (m, 4H), 3.42–4.49 (m, 2H), 3.77–3.83 (m, 2H), 4.70 (d, J = 16 Hz, 2H), 4.97 (d, J = 16 Hz, 2H), 6.63–6.65 (m, 2H), 7.17–7.18 (m, 2H), 7.25–7.28 (m, 2H), 7.56 (s, 4H), 8.03 (br, 4H, NH2); 13C NMR (100 MHz, DMSO-d6): δ = 13.9, 20.0, 28.9, 35.9, 42.1, 50.8, 51.1, 60.8, 81.1, 109.7, 11.7, 124.4, 129.9, 130.9, 133.5, 136.9, 141.4, 148.3, 160.4, 167.5, 168.9, 169.8, 175.9.
1′,1′′′-(1,4-Phenylenebis(methylene))bis(2-amino-5′-bromo-2′,5-dioxo-5,6,7,8-tetrahydrospiro[chromene-4,3′-indoline]-3-carbonitrile) (7r). Cream solid, mp > 290 °C; 1H NMR (400 MHz, DMSO-d6): δ = 1.95–1.98 (m, 4H), 2.25–2.29 (m, 4H), 2.70–2.85 (m, 4H), 4.90 (s, 4H), 6.69–6.71 (m, 2H), 6.97–6.99 (m, 2H), 7.11–7.14 (m, 4H), 7.32 (s, 2H), 7.45 (br, 4H, NH2); 13C NMR (100 MHz, DMSO-d6): δ = 20.1, 27.3, 36.7, 43.5, 44.6, 47.3, 57.0, 111.3, 111.5, 114.9, 117.8, 126.6, 127.6, 131.5, 135.2, 136.6, 142.3, 159.2, 159.3, 167.5, 176.9, 195.9.
Diethyl-1′,1′′′-(1,4-phenylenebis(methylene))bis(2-amino-5′,7,7-trimethyl-2′,5-dioxo-5,6,7,8-tetrahydrospiro[chromene-4,3′-indoline]-3-carboxylate) (7s). Cream solid, mp > 290 °C; 1H NMR (400 MHz, DMSO-d6): δ = 0.53–0.61 (m, 6H), 0.99 (s, 6H), 1.04 (s, 6H), 1.26 (s, 4H), 2.11 (s, 4H), 2.17 (s, 6H), 3.47–3.51 (m, 2H), 3.75–3.79 (m, 2H), 4.66 (d, J = 16 Hz, 2H), 4.97 (d, J = 16 Hz, 2H), 6.52–6.54 (m, 2H), 6.75 (s, 2H), 6.85–6.86 (m, 2H), 7.57 (s, 4H), 7.94 (br, 4H, NH2); 13C NMR (100 MHz, DMSO-d6): δ = 14.1, 24.8, 27.1, 27.3, 32.5, 42.8, 44.8, 50.9, 51.1, 79.1, 108.8, 113.3, 122.11, 122.7, 127.4, 127.9, 128.1, 134.9, 135.5, 145.3, 159.5, 159.6, 163.5, 168.1, 179.5.
Diethyl-1′,1′′′-(1,4-phenylenebis(methylene))bis(2-amino-7,7-dimethyl-2′,5-dioxo-5,6,7,8-tetrahydrospiro[chromene-4,3′-indoline]-3-carboxylate) (7t). Cream solid, mp > 290 °C; 1H NMR (400 MHz, DMSO-d6): δ = 0.53–0.55 (m, 6H), 0.98 (s, 6H), 1.05 (s, 6H), 1.26 (s, 4H), 2.11 (s, 4H), 3.40–3.45 (m, 2H), 3.77–3.79 (m, 2H), 4.71 (d, J = 16 Hz, 2H) 4.97 (d, J = 16 Hz, 2H), 6.67 (d, J = 8 Hz, 2H), 6.86 (d, J = 8 Hz, 2H), 6.94–6.95 (m, 2H), 7.06–7.08 (m, 2H), 7.59 (s, 4H), 7.96 (br, 4H, NH2); 13C NMR (100 MHz, DMSO-d6): δ = 14.1, 27.2, 27.3, 32.5, 41.9, 44.9, 51.9, 52.0, 80.1, 108.8, 113.2, 122.1, 122.6, 127.4, 127.9, 128.2, 134.9, 136.5, 145.5, 159.3, 159.5, 164.7, 168.1, 178.7.
1′,1′′′-(1,4-Phenylenebis(methylene))bis(2-amino-7,7-dimethyl-2′,5-dioxo-5,6,7,8-tetrahydrospiro[chromene-4,3′-indoline]-3-carbonitrile) (7u). Cream solid, mp > 290 °C; 1H NMR (400 MHz, DMSO-d6): δ = 1.04 (s, 6H), 1.07 (s, 6H), 2.12–2.26 (m, 4H), 2.57–2.63 (m, 4H), 4.90 (s, 4H), 6.69–6.71 (m, 2H), 6.98–7.00 (m, 2H), 7.01–7.15 (m, 4H), 7.34 (br, 4H, NH2); 13C NMR (100 MHz, DMSO-d6): δ = 27.5, 27.7, 28.0, 31.2, 32.5, 43.5, 47.2, 50.3, 56.9, 110.5, 111.4, 114.9, 117.8, 126.4, 127.6, 131.5, 135.2, 136.4, 142.4, 159.5, 165.5, 176.8, 195.8.
1′,1′′′-(Butane-1,4-diyl)bis(5-amino-8-nitro-2′-oxo-2,3-dihydro-1H-spiro[imidazo[1,2-a]pyridine-7,3′-indoline]-6-carbonitrile) (8a). Cream powder; mp > 290 °C; 1H NMR (400 MHz, CDCl3): δ = 1.75 (4H, m, CH2CH2N), 3.90 (4H, m, CH2N), 4.15 (4H, m, CH2NH), 4.65 (4H, m, CH2N), 6.99 (2H, d, Ar), 7.44 (4H, m, Ar), 7.87 (2H, d, Ar), 8.4–8.75 (br, NH2), 9.88 (2H, s, NH). 13C NMR (100 MHz, CDCl3): δ = 29.0, 47.3, 50.0, 51.6, 65.4, 112.7, 117.1, 120.5, 125.2, 127.3, 128.6, 132.5, 138.9, 146.8, 186.8. Anal. calcd for C34H30N12O6: C, 58.12; H, 4.03; N, 23.92%. Found: C, 58.11; H, 4.09; N, 23.93%.
1,1′′-(Hexane-1,6-diyl)bis(6′-amino-9′-nitro-2-oxo-1′,2′,3′,4′-tetrahydrospiro[indoline-3,8′-pyrido[1,2-a]pyrimidine]-7′-carbonitrile) (8b). White powder; mp > 290 °C; 1H NMR (400 MHz, CDCl3): δ = 1.89–2.35 (16H, m, aliph), 4.2–4.35 (8H, m, CH2N), 5.5–6.5 (br, NH2), 7.84–7.95 (2H, dd, Ar), 7.95–8.10 (2H, dd, Ar), 8.10–8.25 (2H, dd, Ar), 8.25–8.38 (2H, dd, Ar), 9.94 (2H, s, NH). 13C NMR (100 MHz, CDCl3): δ = 25.7, 31.2, 32.7, 43.2, 47.0, 48.6, 50.0, 66.9, 112.7, 120.5, 124.0, 127.3, 128.6, 131.1, 132.5, 138.9, 143.9, 146.8, 186.8. Anal. calcd for C38H38N12O6: C, 60.15; H, 5.05; N, 22.15%. Found: C, 60.22; H, 5.06; N, 22.19%.
1,1′′-(1,4-Phenylenebis(methylene))bis(6′-amino-3′,3′-dimethyl-9′-nitro-2-oxo-1′,2′,3′,4′-tetrahydrospiro[indoline-3,8′-pyrido[1,2-a]pyrimidine]-7′-carbonitrile) (8c). White powder; mp > 290 °C; 1H NMR (400 MHz, CDCl3): δ = 1.97 (12H, s, CH3), 3.80 (8H, t, CH2N), 6.82 (2H, d, CH2Ar), 7.35 (8H, m, Ar), 7.43 (4H, d, Ar), 9.70–10.10 (br, NH2), 10.16 (2H, s, NH). 13C NMR (100 MHz, CDCl3): δ = 23.9, 36.5, 42.3, 53.6, 55.2, 61.1, 112.3, 115.2, 120.3, 123.8, 127.4, 129.0, 129.6, 133.5, 136.3, 137.3, 143.0, 150.8, 154.1, 177.1. Anal. calcd for C44H42N12O6: C, 63.30; H, 5.07; N, 20.13%. Found: C, 63.30; H, 5.09; N, 22.19%.
1,1′′-(Butane-1,4-diyl)bis(6′-amino-5,9′-dinitro-2-oxo-1′,2′,3′,4′-tetrahydrospiro[indoline-3,8′-pyrido[1,2-a]pyrimidine]-7′-carbonitrile) (8d). Pale yellow powder; mp > 290 °C; 1H NMR (400 MHz, CDCl3): δ = 1.41–1.57 (4H, m, aliph), 1.80–2.02 (4H, m, aliph), 2.92–3.07 (4H, m, CH2N), 3.17–3.26 (4H, m, CH2N), 3.81–3.94 (4H, m, CH2N), 7.54–7.6 (4H, m, Ar), 7.94–8.01 (2H, d, Ar), 9.8 (2H, d, NH). 13C NMR (100 MHz, CDCl3): δ = 23.5, 25.5, 42.2, 49.5, 115.7, 116.1, 116.9, 129.2, 133.5, 137.1, 140.1, 143.8, 150.7, 154.5, 175.6. Anal. calcd for C36H32N14O10: C, 52.68; H, 3.93; N, 23.89%. Found: C, 52.72; H, 3.93; N, 23.94%.
1,1′′-(Hexane-1,6-diyl)bis(2′-amino-1′-benzyl-6′-(benzylamino)-5,5′-dinitro-2-oxo-1′H-spiro[indoline-3,4′-pyridine]-3′-carbonitrile) (8e). Yellow powder; mp > 290 °C; 1H NMR (400 MHz, CDCl3): δ = 0.70–0.82 (4H, m, aliph), 1.85–1.94 (4H, m, aliph), 4.04–4.12 (4H, m, CH2N), 6.26–6.33 (2H, t, CH2Ar), 7.45–7.51 (20H, m, Ar), 7.53–7.59 (2H, m, Ar), 8.5–9.5 (br, NH2), 9.98 (2H, d, NH). 13C NMR (100 MHz, CDCl3): δ = 26.7, 27.6, 42.4, 48.6, 49.4, 53.2, 115.2, 116.0, 117.0, 127.1, 127.6, 128.4, 128.9, 129.2, 133.7, 136.8, 137.2, 139.0, 140.2, 143.2, 150.6, 155.4, 174.8. Anal. calcd for C60H52N14O10: C, 63.82; H, 4.64; N, 17.37%. Found: C, 63.86; H, 4.61; N, 17.34%.
1′,1′′′-(1,4-Phenylenebis(methylene))bis(5-amino-5′,8-dinitro-2′-oxo-2,3-dihydro-1H-spiro[imidazo[1,2-a]pyridine-7,3′-indoline]-6-carbonitrile) (8f). Cream powder; mp > 290 °C; 1H NMR (400 MHz, CDCl3): δ = 3.89–4.00 (4H, dt, CH2N), 4.08–4.21 (4H, dt, CH2NH), 6.34 (4H, dd, CH2Ar), 6.69 (2H, d, Ar), 7.16 (2H, d, Ar), 7.29 (4H, m, Ar), 7.40 (2H, t, Ar), 8.91–9.52 (br, NH2), 9.98 (2H, d, NH). 13C NMR (100 MHz, CDCl3): δ = 42.4, 42.9, 48.7, 53.1, 115.8, 116.3, 117.0, 127.7, 128.2, 133.8, 136.4, 137.1, 140.7, 143.2, 150.3, 154.4, 175.9. Anal. calcd for C38H28N14O10: C, 54.29; H, 3.36; N, 23.32%. Found: C, 54.35; H, 3.31; N, 23.38%.
1,1′′-(Hexane-1,6-diyl)bis(6′-amino-3′,3′,5-trimethyl-9′-nitro-2-oxo-1′,2′,3′,4′-tetrahydrospiro[indoline-3,8′-pyrido[1,2-a]pyrimidine]-7′-carbonitrile) (8g). White powder; mp > 290 °C; 1H NMR (400 MHz, CDCl3): δ = 0.81 (4 H, m, CH2), 0.91 (6H, s, Me), 1.04 (6H, s, Me), 1.19 (4H, m, aliph), 2.93 (6H, s, MeAr), 4.04 (4H, d, NCH2), 4.26 (4H, d, NHCH2), 4.55 (4H, m, CH2N), 7.08 (2 H, d, Ar), 7.20 (2H, t, Ar), 7.31 (2H, t, Ar), 10.22 (2H, d, NH). 13C NMR (100 MHz, CDCl3): δ = 20.1, 23.1, 26.4, 27.0, 36.9, 42.1, 49.0, 55.8, 61.2, 115.0, 115.6, 126.3, 127.1, 128.1, 129.2, 133.8, 134.7, 137.6, 143.8, 150.1, 154.5, 174.8. Anal. calcd for C44H50N12O6: C, 62.69; H, 5.98; N, 19.94%. Found: C, 62.72; H, 6.04; N, 20.00%.
1′,1′′′-(1,2-Phenylenebis(methylene))bis(5-amino-5′-methyl-8-nitro-2′-oxo-2,3-dihydro-1H-spiro[imidazo[1,2-a]pyridine-7,3′-indoline]-6-carbonitrile) (8h). Cream powder; mp > 290 °C; 1H NMR (400 MHz, CDCl3): δ = 3.03 (6H, s, Me), 3.77 (8H, m, CH2N), 6.78 (4H, d, CH2Ar), 7.10 (2H, t, Ar), 7.17 (2H, d, Ar), 7.29 (2H, t, Ar), 7.40 (2H, t, Ar), 8.70–9.30 (br, NH2), 9.78 (2H, d, NH). 13C NMR (100 MHz, CDCl3): δ = 21.6, 42.1, 42.7, 50.2, 53.0, 115.3, 117.2, 126.8, 127.1, 127.6, 128.7, 129.3, 131.6, 133.0, 134.1, 137.4, 143.3, 150.3, 155.2, 174.4. Anal. calcd for C40H34N12O6: C, 61.69; H, 4.40; N, 21.58%. Found: C, 61.61; H, 4.35; N, 21.58%.
1,1′′-(1,4-Phenylenebis(methylene))bis(6′-amino-5-methyl-9′-nitro-2-oxo-1′,2′,3′,4′-tetrahydrospiro[indoline-3,8′-pyrido[1,2-a]pyrimidine]-7′-carbonitrile) (8i). Yellow powder; mp > 290 °C; 1H NMR (400 MHz, CDCl3): δ = 1.65 (4H, m, aliph), 3.59 (4H, t, CH2N), 3.73 (4H, t, CH2NH), 3.85 (6H, s, CH3), 6.47 (2 H, d, CH2Ar), 7.05 (2H, dt, Ar), 7.15 (2H, m, Ar), 7.35 (2H, dt, Ar), 7.45 (2H, t, Ar), 7.54 (2H, dt, Ar), 10.03 (2H, s, NH). 13C NMR (100 MHz, CDCl3): δ = 18.1, 20.1, 23.8, 42.6, 48.3, 49.0, 53.2, 115.8, 117.1, 127.7, 127.9, 128.8, 129.0, 133.7, 134.4, 136.1, 137.2, 143.7, 150.3, 153.3, 154.0, 175.6. Anal. calcd for C42H38N12O6: C, 62.65; H, 4.75; N, 20.83%. Found: C, 62.61; H, 4.72; N, 20.79%.
1,1′′-(Butane-1,4-diyl)bis(6′-amino-5-fluoro-7′-isocyano-9′-nitro-1′,2′,3′,4′-tetrahydrospiro[indoline-3,8′-pyrido[1,2-a]pyrimidin]-2-one) (8j). Cream powder; mp > 290 °C; 1H NMR (400 MHz, CDCl3): δ = 0.87 (4H, m, aliph), 1.01 (4H, m, aliph), 4.23 (8H, m, CH2N), 4.50 (4H, t, NCH2), 7.25 (2 H, d, Ar), 7.58 (2H, d, Ar), 7.70 (2H, t, Ar), 10.03 (2H, s, NH). 13C NMR (100 MHz, CDCl3): δ = 23.7, 25.5, 38.0, 42.3, 49.8, 114.2, 114.7, 117.2, 133.5, 136.8, 137.2, 143.9, 150.6, 161.1, 161.4, 176.3. Anal. calcd for C36H32F2N12O6: C, 56.39; H, 4.21; N, 21.92%. Found: C, 56.49; H, 4.28; N, 21.86%.
1,1′′-(1,4-Phenylenebis(methylene))bis(2′-amino-1′-benzyl-6′-(benzylamino)-5-fluoro-5′-nitro-2-oxo-1′H-spiro[indoline-3,4′-pyridine]-3′-carbonitrile) (8k). White powder; mp > 290 °C; 1H NMR (400 MHz, CDCl3): δ = 7.76 (8H, dd, CH2Ar), 6.28 (4H, t, CH2Ar), 7.21 (2H, d, Ar), 7.34 (2H, d, Ar), 7.43–7.60 (20H, m, Ar), 7.57 (2H, t, Ar), 7.92 (4H, m, Ar), 8.60–9.10 (br, NH2), 10.00 (2H, d, NH). 13C NMR (100 MHz, CDCl3): δ = 19.0, 42.0, 49.3, 49.5, 53.2, 114.2, 114.6, 115.6, 117.6, 127.4, 127.7, 128.1, 128.5, 129.2, 133.8, 136.3, 136.9, 139.5, 143.2, 150.8, 153.6, 154.0, 161.2, 173.9. Anal. calcd for C62H48F2N12O6: C, 68.00; H, 4.42; N, 15.35%. Found: C, 68.03; H, 4.36; N, 15.41%.
1′,1′′′-(Butane-1,4-diyl)bis(5-amino-5′-bromo-6-isocyano-8-nitro-2,3-dihydro-1H-spiro[imidazo[1,2-a]pyridine-7,3′-indolin]-2′-one) (8l). White powder; mp > 290 °C; 1H NMR (400 MHz, CDCl3): δ = 1.15 (4H, m, aliph), 3.94 (4H, m, CH2N), 4.13 (4H, m, CH2NH), 4.40 (4H, m, CH2N), 7.2–7.6 (br, NH2), 7.70–7.85 (4H, m, Ar), 7.93–7.99 (2H, m, Ar), 9.77 (1 H, s, NH). 13C NMR (100 MHz, CDCl3): δ = 25.5, 42.2, 42.7, 48.8, 49.8, 117.2, 117.7, 126.2, 133.0, 134.6, 136.4, 137.1, 143.0, 151.6, 160.2, 175.5. Anal. calcd for C34H28Br2N12O6: C, 47.46; H, 3.28; N, 19.53%. Found: C, 47.40; H, 3.25; N, 19.46%.
1,1′′-(1,2-Phenylenebis(methylene))bis(6′-amino-5-bromo-3′,3′-dimethyl-9′-nitro-2-oxo-1′,2′,3′,4′-tetrahydrospiro[indoline-3,8′-pyrido[1,2-a]pyrimidine]-7′-carbonitrile) (8m). Yellow powder; mp > 290 °C; 1H NMR (400 MHz, CDCl3): δ = 1.80 (6H, s, CH3), 1.85 (6H, s, CH3), 3.45 (4H, m, CH2N), 3.83 (4H, m, CH2NH), 4.70–5.04 (4H, dd, CH2Ar), 7.29 (2H, t, Ar), 7.47 (2H, d, Ar), 7.76 (2H, t, Ar), 8.06 (2H, d, Ar), 8.22 (2H, d, Ar), 9.90–10.20 (br, NH2), 10.26 (2H, s, NH). 13C NMR (100 MHz, CDCl3): δ = 23.8, 36.6, 42.8, 53.2, 55.1, 61.3, 115.1, 117.1, 117.5, 126.2, 127.6, 129.5, 131.1, 133.4, 134.5, 137.5, 143.1, 151.2, 151.5, 175.5. Anal. calcd for C44H40Br2N12O6: C, 53.24; H, 4.06; N, 16.93%. Found: C, 53.16; H, 4.11; N, 16.91%.
1,1′′-(1,4-Phenylenebis(methylene))bis(2′-amino-1′-benzyl-6′-(benzylamino)-5-bromo-5′-nitro-2-oxo-1′H-spiro[indoline-3,4′-pyridine]-3′-carbonitrile) (8n). Cream powder; mp > 290 °C; 1H NMR (400 MHz, CDCl3): δ = 6.00 (8H, dd, CH2Ar), 6.28 (4H, dt, CH2Ar), 7.15 (12H, m, Ar), 7.42 (4H, m, Ar), 7.63 (2H, t, Ar), 7.88 (2H, t, Ar), 8.02 (2H, d, Ar), 8.21 (4H, m, Ar), 8.31 (2H, d, Ar), 10.11 (2H, d, NH). 13C NMR (100 MHz, CDCl3): δ = 20.6, 43.0, 48.4, 48.7, 53.4, 115.4, 117.1, 117.6, 126.3, 127.2, 127.7, 128.5, 128.9, 129.5, 133.1, 134.4, 136.4, 139.5, 143.7, 150.3, 153.6, 153.9, 174.4. Anal. calcd for C62H48Br2N12O6: C, 61.19; H, 3.98; N, 13.81%. Found: C, 61.16; H, 4.03; N, 13.86%.
Conclusions
In summary, a highly efficient and green method for one-pot multi-component synthesis of bis-spirooxindoles has been reported. The described procedure takes advantage of PEG-400 as an inexpensive, biodegradable, commercially available and reusable solvent. Non-toxic and environmentally friendly reagent, K2CO3, was used to promote the reaction toward completion. The generality of the method has been investigated using ketene aminals as well as enolizable ketones to form a library of complex structure of bis-spiro compounds.
Conflicts of interest
There are no conflicts to declare.
Acknowledgements
The authors thank Persian Gulf University Research Councils for the financial support of this work.
References
- R. L. Swann and A. Eschenroeder, Fate of chemicals in the environment, ACS Symposium sries, Washington DC, 1983, vol. 225 Search PubMed.
- A. Sarkar, S. Santra, S. K. Kundu, A. Hajra, G. V. Zyryanov, O. N. Chupakhin, V. N. Charushin and A. Majee, Green Chem., 2016, 18, 4475–4525 RSC.
-
(a) D. J. C. Constable, A. D. Curzons and V. L. Cunningham, Green Chem., 2002, 4, 521–527 RSC;
(b) A. D. Curzons, D. J. C. Constable, D. N. Mortimer and V. L. Cunningham, Green Chem., 2001, 3, 1–6 RSC.
- H. R. Safaei, M. Shekouhy, S. Rahmanpur and A. Shirinfeshan, Green Chem., 2012, 14, 1696 RSC.
- J. Song and B. Han, Natl. Sci. Rev., 2015, 1–2 Search PubMed.
- C. Capello, U. Fisher and K. Kungerbühler, Green Chem., 2007, 9, 927–934 RSC.
- A. Chanda and V. V. Fokin, Chem. Rev., 2009, 109, 725–748 CrossRef CAS PubMed.
-
(a) J. Chen, S. K. Spear, J. G. Huddleston and R. D. Rogers, Green Chem., 2005, 7, 64–82 RSC;
(b) J. Bi, Z. Zhang, Q. Liu and G. Zhang, Green Chem., 2012, 14, 1159 RSC;
(c) J. M. Khurana, A. Chaudhary, A. Lumb and B. Nand, Green Chem., 2012, 14, 2321 RSC;
(d) Y. Zou, Q. Huang, T. K. Huang, Q. C. Ni, E. S. Zhang, T. L. Xu, M. Yuand and J. Lie, Org. Biomol. Chem., 2013, 11, 6967 RSC;
(e) G. D. Yadav and B. G. Motirale, Org. Process Res. Dev., 2009, 13, 341 CrossRef CAS;
(f) M. Vafaeezadeh and M. Mahmoodi Hashemi, J. Mol. Liq., 2015, 207, 73–79 CrossRef CAS.
- C. C. A. Cariou, G. J. Clarkson and M. Shipman, J. Org. Chem., 2008, 73, 9762–9764 CrossRef CAS PubMed.
- L. Zhou, D. S. Bohle, H. F. Jiang and C. J. Li, Synlett, 2009, 937–940 CAS.
- G. Ren, J. Zhang, Z. Duan, M. Cui and Y. Wu, Aust. J. Chem., 2009, 62, 75–81 CrossRef CAS.
- S. L. Schreiber, Science, 2000, 287, 1964–1969 CrossRef CAS PubMed.
- R. C. Cioc, E. Ruijter and R. V. A. Orru, Green Chem., 2014, 16, 2958–2975 RSC.
- M. Zolfigol, A. Khazaei, A. Zare, M. Mokhlesi, T. Hekmat-Zadeh, A. Hasaninejad, F. Derakhshan-Panah, A. Moosavi-Zare, H. Keypour, A. Deghani-Firouzabadi and M. Merajoddin, J. Chem. Sci., 2012, 124, 501–508 CrossRef CAS.
- A. Zare, R. Khanivar, M. Hatami, M. Mokhlesi, M. Zolfigol, A. Moosavi-Zare, A. Hasaninejad, A. Khazaei and V. Khakyzadeh, J. Mex. Chem. Soc., 2012, 4, 56 Search PubMed.
- A. Hasaninejad, S. Firoozi and F. Mandegani, Tetrahedron Lett., 2013, 54, 2791–2794 CrossRef CAS.
-
(a) P. Helene, Adv. Synth. Catal., 2012, 354, 237–294 CrossRef;
(b) J. G. Hernandez and E. Juaristi, Chem. Commun., 2012, 48, 5396–5409 RSC;
(c) D. Bonne, T. Constantieux, Y. Coquerel and J. Rodriguez, Org. Biomol. Chem., 2012, 10, 3969–3973 RSC.
- Y. Gu, Green Chem., 2012, 14, 2091–2128 RSC.
- G. R. Khabibullin, E. S. Fedotov, V. R. Akhmetov, E. S. Mesheryakov, L. M. Khalilov and A. G. bragimov, Mol. Diversity, 2016, 2, 557–565 CrossRef PubMed.
- S. K. Panja and S. Saha, RSC Adv., 2013, 3, 14495–14500 RSC.
- A. Maryamabadi, A. Hasaninejad, N. Nowrouzi, G. H. Mohebbi and B. Asghari, Bioorg. Med. Chem., 2016, 6, 1408–1417 CrossRef PubMed.
- P. Licence, J. Ke, M. Sokolova, S. K. Rossb and M. Poliakoff, Green Chem., 2003, 5, 99–104 RSC.
- A. Dandia, A. K. Jain and A. K. Laxkar, Tetrahedron Lett., 2013, 30, 3929–3932 CrossRef.
- R. A. Sheldon, Green Chem., 2005, 7, 267–287 RSC.
- A. Hasaninejad, A. Zare, M. Shekouhya and J. Ameri-Rada, Green Chem., 2011, 13, 958–964 RSC.
- R. Sakhuja, S. S. Panda, L. Khanna, S. Khurana and S. C. Jain, Bioorg. Med. Chem., 2011, 21, 5465–5469 CrossRef CAS PubMed.
- K. Parthasarathy, C. Praveen, C. Balachandran, p. S. Kumar, S. Ignacimuthu and P. T. Perumal, Bioorg. Med. Chem., 2013, 23, 2708–2713 CrossRef CAS PubMed.
- A. Maryamabadi, A. Hasaninejad, N. Nowrouzi and G. H. Mohebbi, Bioorg. Med. Chem., 2017, 25, 2057–2064 CrossRef CAS PubMed.
- P. Khanna, A. Saxena, L. Khanna, S. Bhagat and S. C. Jain, ARKIVOC, 2009, 5, 119–125 Search PubMed.
- M. Jain, R. Sakhuja, P. j. Khanna, S. Bhagat and S. C. Jaina, ARKIVOC, 2008, 15, 54–64 Search PubMed.
- C. M. Clay, H. M. Abdallah, C. Jordan, K. Knisley and D. M. Ketcha, ARKIVOC, 2012, 6, 317–325 Search PubMed.
- M. Kidwai, A. Jain, V. Nemaysh, R. Kumar and P. Luthra, Med. Chem. Res., 2013, 22, 2717–2723 CrossRef CAS.
-
(a) J. Chen, S. K. Spear, J. G. Huddleston and R. D. Rogers, Green Chem., 2005, 7, 64–82 RSC;
(b) D. Q. Xu, S. P. Luo, Y. F. Wang, A. B. Xia, H. D. Yue, L. P. Wang and Z. Y. Xu, Chem. Commun., 2007, 42, 4393–4395 RSC;
(c) Y. N. Li, J. L. Wang and L. N. He, Tetrahedron Lett., 2011, 52, 3485–3488 CrossRef CAS.
- R. Ballini, G. Bosica, M. L. Conforti, R. Magg, A. Mazzacani, P. Righi and G. Sartori, Tetrahedron, 2001, 7, 1395–1398 CrossRef.
- K. Hiramoto, A. Nasuhara, K. Michikoshi, T. Kato and K. Kikugawa, Mutat. Res., Genet. Toxicol. Environ. Mutagen., 1997, 395, 47–56 CrossRef CAS.
- G. Khanna, K. Aggarwal and J. M. Khurana, Synth. Commun., 2016, 23, 1880–1886 CrossRef.
- J. Ma and S. M. Hecht, Chem. Commun., 2004, 1190–1191 RSC.
- C. B. Cui, H. Kakeya and H. Osada, Tetrahedron, 1996, 52, 12651–12666 CrossRef CAS.
- T. Usui, M. Kondoh, C. B. Cui, T. Mayumi and H. Osada, Biochem. J., 1998, 333, 543–548 CrossRef CAS PubMed.
- J. F. M. Silva and A. C. Pinto, J. Braz. Chem. Soc., 2001, 12, 273–324 CrossRef.
- L. R. Wen, C. L. Mingli and L. J. Wang, J. Org. Chem., 2010, 75, 7605–7614 CrossRef CAS PubMed.
- D. P. Sangi, J. L. Monteiro, K. L. Vanzolini, Q. B. Cass, M. W. Paixao and A. G. Corrêa, J. Braz. Chem. Soc., 2014, 25, 887–889 CAS.
- K. M. Wang and S. J. Yan, Eur. J. Org. Chem., 2014, 6, 1129–1145 CrossRef.
- X. Wang, Z. Quan and Z. Zhang, Tetrahedron, 2007, 63, 8227–8233 CrossRef CAS.
Footnote |
† Electronic supplementary information (ESI) available. See DOI: 10.1039/c7ra06017c |
|
This journal is © The Royal Society of Chemistry 2017 |