DOI:
10.1039/C7RA05750D
(Paper)
RSC Adv., 2017,
7, 34411-34414
A water-soluble pillar[5]arene-based chemosensor for highly selective and sensitive fluorescence detection of L-methionine†
Received
22nd May 2017
, Accepted 25th June 2017
First published on 10th July 2017
Abstract
L-Methionine (L-Met) is an essential amino acid for the human body. The detection of L-Met in water solution is very important. Herein, we report an efficient approach for the fluorescent detection of L-Met with high selectivity and sensitivity in water using a cationic pillar[5]arene-based chemosensor (AWP5). When various L-amino acids are added to the AWP5 water solution, only L-Met can induce fluorescence in AWP5 at λem = 520 nm, showing dramatic enhancement; thus, other amino acid do not interfere in the L-Met recognition process. AWP5 also shows high sensitivity for L-Met in water, with the lowest detection limit of AWP5 for L-Met being 5.46 × 10−7 mol L−1.
1. Introduction
L-Amino acids play a significant role in chemistry and biology. In particular, L-Met is one of the most essential amino acid for protein synthesis in the human body, which can promote the growth of hair and nails, enhance muscle activity etc. L-Methionine acts as a methyl group donor in many methyltransferase reactions including biosynthesis of phospholipids1 and bioelectrical activity.2 Loss of L-methionine in brain cells of aged animals is linked with the loss of dopaminergic β-adrenergic binding sites and changes in cell membrane composition.3 Therefore, the selective detection of L-Met is very important. To date, there are various useful strategies or methods such as artificial chemosensors, high-performance liquid chromatography, and capillary zone electrophoresis4–8 that have been applied for the detection of L-amino acids. However, these methods suffer from a number of problems. First, the instrument detection methods often employ expensive equipment. Second, owing to the low water solubility of organic groups, most synthesized chemosensors do not work well in water solution. However, most biological or environmental procedures are carried out in water systems. Therefore, it is still a big challenge and an important task to develop a water soluble chemosensor for the detection L-Met in water.
Recently, pillararene chemistry has undergone rapid development9–16 and pillararene derivatives have been widely used in the field of molecular recognition,17–23 drug delivery,24–30 molecular devices,31–37 and so on. Note that two kinds of important water-soluble pillararene derivatives have been developed to date: (1) cationic pillararene38 and (2) anionic pillararene.39,40 Due to their nice water solubility, inclusion and host–guest properties, these kinds of pillararene derivatives provide a great opportunity for the design of water-soluble chemosensors.
In view of this and based on our research interest in molecule recognition,41–48 herein, we report a novel and efficient way for the detection of L-Met in water via a cationic pillar[5]arene-based chemosensor (AWP5). As shown in Scheme 1, the chemosensor AWP5 possesses high water solubility and can enable fluorescence detection of L-Met in water with high selectivity and sensitivity through a host–guest mechanism.
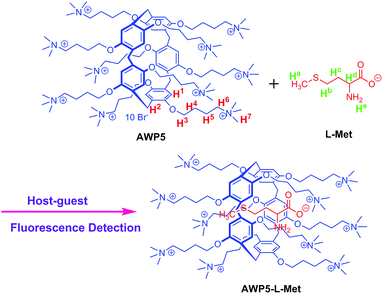 |
| Scheme 1 The chemical structure of AWP5 and possible selective detection mechanism of the sensor AWP5 for L-Met. | |
2. Results and discussion
Cationic pillar[5]arene (AWP5) was synthesized according to Scheme S1.† To investigate L-amino acid recognition abilities of the sensor AWP5 in water, we carried out a series of host–guest recognition experiments. The recognition profiles of the sensor AWP5 for various L-amino acids, including L-α-Ala, L-Arg, L-Cys, L-Gly, L-Lys, L-Pro, L-Met, L-Thr, L-Ser, L-His, L-Val, L-Tyr, L-Asn, L-Glu, L-Trp, L-Gln, L-Ile, L-Phe, L-Asp, and L-Leu, were primarily investigated using fluorescence spectroscopy in water.
As shown in Fig. 1, when 10 equiv. of L-Met was added to the water solution of sensor AWP5, a blue fluorescence emission appeared. Moreover, in the corresponding fluorescence spectrum, the maximum emission peak appeared at 617 nm. However, other L-amino acids such as L-α-Ala, L-Arg, L-Cys, L-Gly, L-Lys, L-Pro, L-Thr, L-Ser, L-His, L-Val, L-Tyr, L-Asn, L-Glu, L-Trp, L-Gln, L-Ile, L-Phe, L-Asp, and L-Leu did not induce similar fluorescence changes (Fig. 2 and S10†). Therefore, in water solution, AWP5 showed specific fluorescence selectivity for L-Met.
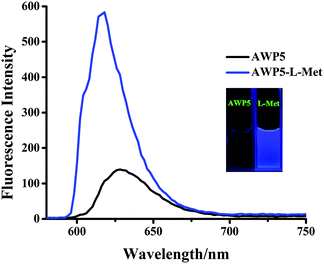 |
| Fig. 1 Fluorescence spectra of AWP5 (C = 2 × 10−5 mol L−1 in water) and the AWP5 water solution after the addition of 10 equiv. of L-Met (λex = 520 nm). | |
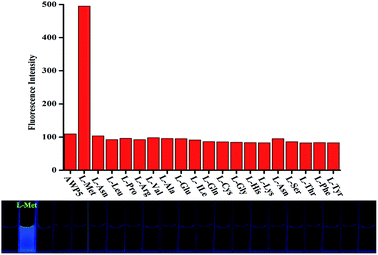 |
| Fig. 2 Fluorescence spectra of the sensor AWP5 in water solution (2 × 10−5 mol L−1) upon the addition of 10 equiv. of L-Ala, L-Arg, L-Asp, L-Cys, L-Gln, L-Glu, L-His, L-Ile, L-Gly, L-Asn, L-Leu, L-Lys, L-Met, L-Phe, L-Pro, L-Ser, L-Thr, L-Trp, L-Tyr, and L-Val (λex = 520 nm). | |
To further investigate the efficiency of the sensor AWP5 for L-Met detection, we carried out fluorescence titration experiments. As shown in Fig. 3, in the fluorescence spectrum, with an increasing amount of L-Met, emission peak at 594 nm gradually increased. Furthermore, the detection limit of the fluorescent spectrum changes calculated on the basis of 3s/m (ref. 49) is 5.46 × 10−7 M for L-Met (Fig. S11, ESI†). Moreover, we carried out similar experiments in the presence of 10 equiv. of L-his (Fig. S12, ESI†). The L-Met detection limit was 2.07 × 10−6 M in the presence of 10 equiv. of L-His, which indicated that AWP5 showed high sensitivity for L-Met in the presence of other amino acids.
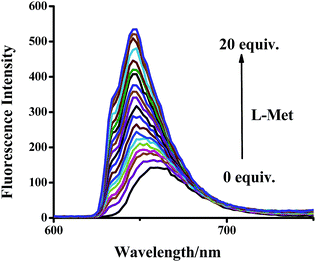 |
| Fig. 3 Fluorescence intensity changes of AWP5 water solution (C = 1 × 10−5 mol L−1) upon the addition of different amounts of L-Met (λex = 520 nm). | |
To further exploit the utility of AWP5 as a selective sensor for L-Met, competitive experiments were carried out in the presence of 10.0 equiv. of L-Met and 2.0 equiv. of various L-amino acids in water. The fluorescence emission spectrum of the sensor AWP5 with L-Met was not influenced by the subsequent addition of other competing L-amino acids, which indicated that AWP5 had specific selectivity for L-Met (Fig. 4).
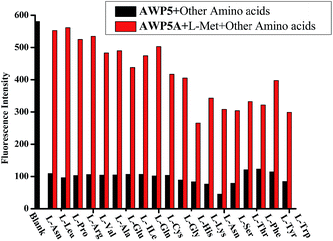 |
| Fig. 4 Fluorescence intensity changes of the sensor AWP5 water solution (1 × 10−5 mol L−1) ensemble in the presence of other amino acids (2.0 equiv.) (λex = 520 nm). | |
The recognition mechanism of AWP5 towards L-Met was investigated by 1H-NMR titrations, 2D NOESY-NMR, ESI-mass spectrometry, and job plots. As shown in Fig. 5, in 1H-NMR, upon the addition of L-Met to an AWP5 D2O solution, proton peaks of AWP5 and L-Met show obvious shifts. The triplet peaks of Ha, Hb, Hc, and Hd of L-Met show obvious upfield shifts (Δδ = −0.15, −0.23, −1.54, −0.10, −0.12 ppm), which indicates that L-Met is located in the cavity of AWP5. Simultaneously, the proton peaks of H1 and H2 of AWP5 shift upfield (Δδ = −0.25 and −0.40 ppm), which indicate electrostatic interactions between the carboxylic acid groups of L-Met and ammonium salts of AWP5. The electrostatic interactions directly lead to charge transfer from the carboxylate groups of L-Met to the AWP5 ammonium salt groups. By this way, these proton chemical shift changes indicated that the complexation took place between AWP5 and L-Met in solution.
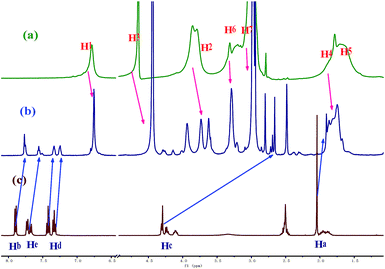 |
| Fig. 5 1H-NMR spectra (600 MHz, 298 K) of (a) AWP5 (1 × 10−3 mol L−1 in H2O); (b) equimolar mixture of AWP5 and L-Met (1 : 1); and (c) L-Met (1 × 10−3 mol L−1 in DMSO-d6). | |
Furthermore, a 2D NOESY NMR study of a mixture of AWP5 (10.0 mM) and L-Met (10.0 mM) in D2O was carried out to investigate the relative spatial positions of this host–guest complex. As shown in Fig. 6, clear correlation signals were observed between proton H1 of AWP5 and proton Ha of L-Met, indicating that L-Met penetrated through the cavity of AWP5.
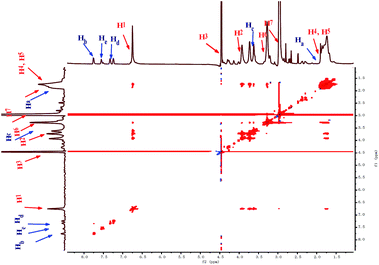 |
| Fig. 6 NOESY-NMR spectra of (600 MHz, D2O, 298 K) of AWP5 : L-Met = 1 : 1. | |
To confirm the binding stoichiometry between the sensor AWP5 and L-Met in H2O, a job plot was constructed. The results illustrate that L-Met forms a 1
:
1 complex with AWP5 (Fig. 7). Furthermore, mass spectrometry experiments also support the abovementioned proposed host–guest mechanism. In the ESI-MS of the sensor AWP5 and L-Met solution, (Fig. S13, ESI†) a peak at m/z = 444.0376 for [AWP5 + L-Met + 4Br]5+ proved the 1
:
1 complexation stoichiometry between AWP5 and L-Met.
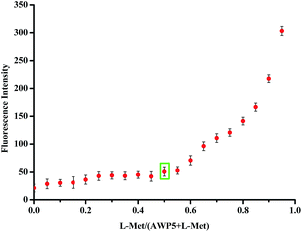 |
| Fig. 7 Job plot for complexation between AWP5 and L-Met in H2O. | |
Through the abovementioned phenomena and description, a possible mechanism was proposed (Scheme 1). On addition of L-Met, AWP5 forms a stable inclusion complex with L-Met in solution because of their multiple interactions. The methylene groups of L-Met can result in C–H⋯π interactions with the pillar[5]arene group, whereas the amino groups of L-Met can form hydrogen bonds with oxygen atoms of the pillar[5]arene group. In addition, electrostatic interactions occur between ammonium groups of AWP5 and carboxylic acid group of L-Met. These multiple interactions stabilize the resulting inclusion complex between AWP5 and L-Met.
3. Conclusions
In summary, we employed cationic pillar[5]arene (AWP5) as a water-soluble chemosensor for the recognition of amino acids. The chemosensor AWP5 could fluorescently detect L-Met in water with high selectivity and sensitivity by a host–guest mechanism. Moreover, the detection limit of the sensor AWP5 for L-Met was 5.46 × 10−7 mol L−1, which indicated that the sensor AWP5 could be useful as a highly selective and sensitive sensor for detecting L-Met. The chemosensor AWP5 can thus serve as a practical and convenient fluorescence test kit to detect L-Met.
Acknowledgements
This study was supported by the National Natural Science Foundation of China (NSFC) (No. 21662031; 21661028; 21574104; 21262032), the Natural Science Foundation of Gansu Province (1506RJZA273) and the Program for Changjiang Scholars and Innovative Research Team in University of Ministry of Education of China (IRT 15R56).
Notes and references
- G. L. Cantoni, J. Am. Chem. Soc., 1952, 74, 2942 CrossRef CAS.
- H. Borsook and J. W. Dubnoff, J. Biol. Chem., 1947, 171, 363 CAS.
- H. A. Friedel, K. L. Goa and P. Benfield, Drugs, 1989, 38, 389 CrossRef CAS PubMed.
- Y. k. Yue, F. Huo, P. Ning, Y. Zhang, J. Chao, X. Meng and C. Yin, J. Am. Chem. Soc., 2017, 139, 3181 CrossRef CAS PubMed.
- Y. Konya, M. Taniguchi and E. Fukusaki, J. Biosci. Bioeng., 2017, 123, 126 CrossRef CAS PubMed.
- E. Monteagudo, A. Virgili, T. Parella and P. T. Míriam, Anal. Chem., 2017, 89, 4939 CrossRef CAS PubMed.
- H. J. Issaq and K. C. Chan, Electrophoresis, 1995, 16, 467 CrossRef CAS PubMed.
- M. R. Silva, R. M. Olivas, O. F. X. Donard and M. Lamotte, Appl. Organomet. Chem., 1997, 11, 21 CrossRef.
- T. Ogoshi, S. Kanai, S. Fujinami, T. Yamagishi and Y. J. Nakamoto, J. Am. Chem. Soc., 2008, 130, 5022 CrossRef CAS PubMed.
- D. Cao, Y. Kou, J. Liang, Z. Chen, L. Wang and H. Meier, Angew. Chem., Int. Ed., 2009, 48, 9721 CrossRef CAS PubMed.
- P. J. Cragg and K. Sharma, Chem. Soc. Rev., 2012, 41, 597 RSC.
- M. Xue, Y. Yang, X. Chi, Z. Zhang and F. Huang, Acc. Chem. Res., 2012, 45, 1294 CrossRef CAS PubMed.
- G. Yu, D. Wu, Y. Li, Z. Zhang, L. Shao, J. Zhou, Q. Hu, G. Tang and F. Huang, Chem. Sci., 2016, 7, 3017 RSC.
- S. Dasgupta, A. Chowdhurya and P. S. Mukherjee, RSC Adv., 2015, 5, 85791 RSC.
- M. Bojtár, Z. Szakács, D. Hessz, M. Kubinyibc and I. Bitter, RSC Adv., 2015, 5, 26504 RSC.
- S. K. Wang, L. L. Tan, D. X. Chen, N. Song, G. Xi, S. X. A. Zhang, C. Li and Y. W. Yang, Org. Biomol. Chem., 2012, 10, 9405 Search PubMed.
- K. Jie, Y. Zhou, Y. Yao, B. Shi and F. Huang, J. Am. Chem. Soc., 2015, 137, 10472 CrossRef CAS PubMed.
- T. Ogoshi, S. Takashima and T. Yamagishi, J. Am. Chem. Soc., 2015, 137, 10962 CrossRef CAS PubMed.
- B. Shi, K. Jie, Y. Zhou, J. Zhou, D. Xia and F. Huang, J. Am. Chem. Soc., 2016, 138, 80 CrossRef CAS PubMed.
- B. Shi, D. Xia and Y. Yao, Chem. Commun., 2014, 50, 13932 RSC.
- L. L. Tan, H. Li, Y. Tao, S. X. A. Zhang, B. Wang and Y. W. Yang, Adv. Mater., 2014, 26, 7027 CrossRef CAS PubMed.
- T. Ogoshi, K. Yoshikoshi, R. Sueto, H. Nishihara and T. Yamagishi, Angew. Chem., Int. Ed., 2015, 54, 6566 CrossRef.
- T. Ogoshi, R. Sueto, K. Yoshikoshi, Y. Sakata, S. Akine and T. Yamagishi, Angew. Chem., 2015, 127, 9987 CrossRef.
- Y. Chang, C. Hou, J. Ren, X. Xin, Y. Pei, Y. Lu, S. Cao and Z. Pei, Chem. Commun., 2016, 52, 9578 RSC.
- X. Wu, Y. Li, C. Lin, X. Y. Hu and L. Wang, Chem. Commun., 2015, 51, 6832 RSC.
- Y. Chang, K. Yang, P. Wei, S. Huang, Y. Pei, W. Zhao and Z. Pei, Angew. Chem., Int. Ed., 2014, 53, 13126 CrossRef CAS PubMed.
- Y. Cao, X. Y. Hu, Y. Li, X. Zou, S. Xiong, C. Lin, Y. Z. Shen and L. Wang, J. Am. Chem. Soc., 2014, 136, 10762 CrossRef CAS PubMed.
- Q. Duan, Y. Cao, Y. Li, X. Hu, T. Xiao, C. Lin, Y. Pan and L. Wang, J. Am. Chem. Soc., 2013, 135, 10542 CrossRef CAS PubMed.
- L. Gao, B. Zheng, W. Chen and C. ASchalley, Chem. Commun., 2015, 51, 14901 RSC.
- Y. Wang, J. Du, Y. Wang, Q. Jin and J. Ji, Chem. Commun., 2015, 51, 2999 RSC.
- T. Ogoshi, K. Kida and T. Yamagishi, J. Am. Chem. Soc., 2012, 134, 20146 CrossRef CAS PubMed.
- M. Xue, Y. Yang, X. Chi, X. Yan and F. Huang, Chem. Rev., 2015, 115, 7398 CrossRef CAS PubMed.
- W. Zheng, L. J. Chen, G. Yang, B. Sun, X. Wang, B. Jiang, G. Q. Yin, L. Zhang, X. Li, M. Liu, G. Chen and H. B. Yang, J. Am. Chem. Soc., 2016, 138, 4927 CrossRef CAS PubMed.
- B. Jiang, J. Zhang, J. Q. Ma, W. Zheng, L. J. Chen, B. Sun, C. Li, B. W. Hu, H. Tan, X. Li and H. B. Yang, J. Am. Chem. Soc., 2016, 138, 738 CrossRef CAS PubMed.
- L. L. Tan, H. Li, Y. C. Qiu, D. X. Chen, X. Wang, R. Y. Pan, Y. Wang, S. X. A. Zhang, B. Wang and Y. W. Yang, Chem. Sci., 2015, 6, 1640 RSC.
- Y. Fang, X. Yuan, L. Wu, Z. Peng, W. Feng, N. Liu, D. Xu, S. Li, A. Sengupta, P. K. Mohapatra and L. Yuan, Chem. Commun., 2015, 51, 4263 RSC.
- J. Bi, X. Zeng, D. Tian and H. Li, Org. Lett., 2016, 18, 1092 CrossRef CAS PubMed.
- Y. Ma, X. Ji, F. Xiang, X. Chi, C. Han, J. He, Z. Abliz, W. Chen and F. Huang, Chem. Commun., 2011, 47, 12340 RSC.
- T. Ogoshi, M. Hashizume, T. Yamagishi and Y. Nakamoto, Chem. Commun., 2010, 46, 3708 RSC.
- X. Y. Hu, X. Liu, W. Zhang, S. Qin, C. Yao, Y. Li, D. Cao, L. Peng and L. Wang, Chem. Mater., 2016, 28, 3778 CrossRef CAS.
- Q. Lin, T. T. Lu, X. Zhu, T. B. Wei, H. Li and Y. M. Zhang, Chem. Sci., 2016, 75, 341 Search PubMed.
- Q. Lin, T. T. Lu, J. C. Lou, G. Y. Wu, T. B. Wei and Y. M. Zhang, Chem. Commun., 2015, 51, 12224 RSC.
- Q. Lin, T. T. Lu, X. Zhu, B. Sun, Q. P. Yang, T. B. Wei and Y. M. Zhang, Chem. Commun., 2015, 51, 1635 RSC.
- Q. Lin, B. Sun, Q. P. Yang, Y. P. Fu, X. Zhu, T. B. Wei and Y. M. Zhang, Chem.–Eur. J., 2014, 20, 11457 CrossRef CAS PubMed.
- Q. Lin, X. Liu, T. B. Wei and Y. M. Zhang, Chem.–Asian J., 2013, 8, 3015 CrossRef CAS PubMed.
- T. B. Wei, J. F. Chen, X. B. Cheng, H. Li, Q. Lin, H. Yao and Y. M. Zhang, RSC Adv., 2016, 6, 65898 RSC.
- G. Y. Wu, B. B. Shi, Q. Lin, H. Li, Y. M. Zhang, H. Yao and T. B. Wei, RSC Adv., 2015, 5, 4958 RSC.
- T. B. Wei, J. F. Chen, X. B. Cheng, H. Li, B. B. Han, Y. M. Zhang, H. Yao and Q. Lin, Org. Chem. Front., 2017, 4, 210 RSC.
- Analytical Methods Committee, Analyst, 1987, 112, 199 RSC.
Footnote |
† Electronic supplementary information (ESI) available: Experimental details, synthesis of AWP5, NMR spectra, and other materials. See DOI: 10.1039/c7ra05750d |
|
This journal is © The Royal Society of Chemistry 2017 |
Click here to see how this site uses Cookies. View our privacy policy here.