DOI:
10.1039/C7RA05601J
(Paper)
RSC Adv., 2017,
7, 33231-33240
Synthesis, structure and properties of imidazolium-based energetic ionic liquids†
Received
18th May 2017
, Accepted 24th June 2017
First published on 30th June 2017
Abstract
A series of imidazolium energetic ionic liquids (EILs) 2a, 3a–f, and 4a–f with a nitrooxyethyl or hydroxyethyl side chain in their cations were easily synthesized starting from N-methylimidazole via quaternization, nitration, and metathesis reactions. The EILs and intermediates were fully characterized by FT-IR, UV/Vis, ESI-MS, 1H NMR, 13C NMR, or elemental analysis. Compounds 3a, 3b, and 4b were further studied through single crystal X-ray diffraction. Solubility experiment shows that all the EILs have good solubilities in most polar solvents. The thermal properties of the EILs were investigated via differential scanning calorimetry (DSC) and thermo-gravimetric analysis (TGA). All the EILs have a very wide liquid temperature range over 100 °C. DSC data show that all the energetic compounds are typical ionic liquids with glass transition temperatures from −77 °C to −12 °C and melting temperatures below 100 °C. TGA data indicate that the new ionic liquids possess good thermal stabilities with decomposition temperatures above 165 °C, except for compounds 3d, 4c, and 4d. Generally, the introduction of a nitrooxy group lowered the melting points and decomposition temperatures, but increased the glass transition temperatures of the EILs. The energetic properties of the EILs were further estimated by Kamlet–Jacobs formula. The detonation velocities of ionic liquids 2a and 4a–f are 6.84–7.63 km s−1, which are between those of TNT and RDX. The detonation pressures of ionic liquids 2a, 3e, 4a, 4b, 4d, and 4e are better than that of TNT. Besides, energetic data show that the introduction of a nitrooxy group improved the energetic properties of the new ionic liquids.
Introduction
Ionic liquids, defined as low-melting salts that melt at or below 100 °C, are composed of an organic cation and an inorganic or organic anion.1 They are of great interest because of their unique chemical and physical properties such as low vapor pressure, non-flammability, high thermal stability, good ionic conductivity, and excellent fluidity and solubility.2 Initially, ionic liquids were introduced as alternative green reaction media,3–5 but today they have gradually shown their significant roles in various fields including organic synthesis,6,7 catalysis,8–10 analysis,11 electrochemistry,12,13 material chemistry,14,15 energy technology,16,17 as well as many others.
In the past dozen years, many ionic liquids have been reported to have potentials as green energetic materials.18–21 Compared with traditional molecular energetic compounds, such as TNT, RDX, and HMX, energetic ionic liquids (EILs) have some intrinsic properties such as low vapour pressures, high thermal stabilities, and low melting points,22–24 which make them ideal candidates for minimizing or even eliminating hazardous conditions associated with handling, processing and transporting explosive materials.25 A large number of EILs have been reported based on imidazolium, triazolium, tetrazolium and so on with different energetic anions.25 However, compared with energetic anions, energetic cations are relatively limited due to the absence of some energetic groups such as –NO2, –NO, –N3, –ONO2 etc.18,26 There has been an interest in exploring new EILs, especially for those containing energetic anions with a wider range and cations with desired energetic groups.26
In this study, a series of new imidazolium-based EILs, consisting of different energetic anions and imidazolium cations with a hydroxyethyl or nitrooxyethyl side chain, were designed and easily synthesized. The EILs were fully characterized by FT-IR, UV/Vis, ESI-MS, 1H NMR, 13C NMR, elemental analysis, and/or single crystal diffraction. The influences of the introduction of nitrooxy group on the thermal and energetic properties of the EILs were also investigated.
Results and discussion
Synthesis
A series of energetic alkali metal salts such as potassium 3,5-dinitro-1,2,4-triazolate (KDNT),27,28 potassium picrate (KP),29 potassium 3-nitro-5-oxo-1,2,4-triazolate monohydrate (KNTO·H2O),30 potassium dinitromethanide (KDNM),31 potassium dinitramide (KDN),32 and sodium 5-nitrotetrazolate dihydrate (NaNT·2H2O)33,34 were synthesized according to literature procedures. These energetic alkali metal salts will be used in subsequent metathesis reactions in order to introduce desired energetic anions.
N-Hydroxyethylimidazolium chloride (1) was synthesized via a quaternization reaction of N-methylimidazole with 2-chloroethanol under reflux without solvents (Scheme 1). Subsequent direct metathesis reactions of compound 1 with KDNT, KP, KNTO, KDNM, KDN, and NaNT in acetone successfully gave N-hydroxyethylimidazolium EILs 3a–f.35 Nitration of compound 1 with fuming nitric acid at a low temperature gave N-nitrooxyethylimidazolium nitrate (2a) with an energetic nitrooxy group in the side chain. At the same time, the chloride ion in compound 1 was replaced by a nitrate ion. However, direct metathesis reactions of compound 2a with the alkali metal energetic salts in acetone were unsuccessful. It is probably because that sodium nitrate or potassium nitrate is slightly soluble in acetone, which leads to the presence of few NO3− anions remaining in products. Comparatively, sodium chloride or potassium chloride is insoluble in acetone, indicating that Cl− anion could be a suitable choice for the metathesis reaction. Ion exchange of compound 2a with Cl− type ion exchange resin successfully gave N-nitrooxyethylimidazolium chloride (2b). Compounds 4a–f was then readily obtained by metathesis reactions of compound 2b with KDNT, KP, KNTO, KDNM, KDN, or NaNT in acetone (Scheme 1). The potassium chloride or sodium chloride precipitated was removed by vacuum filtration.
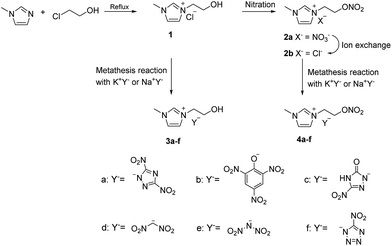 |
| Scheme 1 Synthesis of energetic ionic liquids. | |
Spectroscopy
EILs 1, 2a–b, 3a–f, and 4a–f were characterized by UV-Vis, FT-IR, ESI-MS, 1H NMR, 13C NMR, and elemental analysis, of which the data are listed in Experimental section.
The FT-IR spectra were determined in KBr pellets from 4000 to 450 cm−1 (see ESI†). All the compounds have the aromatic C–H stretching vibration absorption peak at 3200–3000 cm−1 of imidazolium ring, and the aliphatic C–H stretching vibration peak at 3000–2700 cm−1 of side chains. Besides, the characteristic IR absorption peaks of 3,5-dinitro-1,2,4-triazolate, picrate, 3-nitro-5-oxo-1,2,4-triazolate, dinitromethanide, dinitramide, and 5-nitrotetrazolate anions are also clearly observed for compounds 2a, 3a–f, and 4a–f.23,36–39
The UV-Vis spectra of EILs 1, 2a–b, 3a–f, and 4a–f were recorded in water by an UV-1800PC ultraviolet and visible spectrophotometer (see ESI†). The maximum UV absorptions of imidazolium cations are obtained at 213–230 nm, and those of anions are found at 285 nm (3,5-dinitro-1,2,4-triazolate), 354 nm (picrate), 344 nm (3-nitro-5-oxo-1,2,4-triazolate), 361 nm (dinitromethanide), 283 nm (dinitramide), and 255–256 nm (5-nitrotetrazolate), respectively. UV-Vis data show that all the compounds have both desired ions.
The ESI-MS spectra of EILs 1, 2a–b, 3a–f, and 4a–f were also recorded (see ESI†). In ESI-MS positive ion mode, the cationic peaks of compounds 1 and 3a–f are observed at about 126.9 (1-hydroxyethyl-3-methylimidazolium cation), and those of compounds 2a–b and 4a–f are at about 172.0 (1-methyl-3-nitrooxyethylimidazolium cation), indicating the existence of desired imidazolium cations. In ESI-MS negative ion mode, the anionic peaks of compounds 2a, 3a–f, and 4a–f were also detected at 61.8 (nitrate anion), 157.8 (3,5-dinitro-1,2,4-triazolate anion), 227.8 (picrate anion), 128.7 (3-nitro-5-oxo-1,2,4-triazolate anion), 104.6 (dinitromethanide anion), 105.6 (dinitramide anion), and 113.7 (5-nitrotetrazolate anion), respectively. ESI-MS data show that the new energetic salts have both desired cations and anions.
The structures of EILs 1, 2a–b, 3a–f, and 4a–f were further confirmed by 1H NMR and 13C NMR spectra (see ESI†). 1H NMR data show that the aromatic hydrogen signals of C2–
on imidazolium rings were at 8.69–9.27 ppm in DMSO-d6 and disappeared in D2O. This indicated that the C2–
on imidazolium rings is acidic, which underwent a H/D exchange reaction in D2O. Besides, the aromatic hydrogen signals of C4–
and C5–
on imidazolium rings were obtained at 7.34–7.83 ppm. The side chain hydrogen signals of imidazolium cations in EILs 2a–b and 4a–f are at 4.78–4.94 ppm (–C
2–ONO2), 4.51–4.65 ppm (N–C
2–), and 3.78–3.89 ppm (N–C
3), while those of imidazolium cations in compounds 1 and 3a–f are at 5.18–5.23 ppm (–CH2–O
), 4.22–4.38 ppm (N–C
2–), 3.80–3.95 ppm (N–C
3), and 3.73–3.96 ppm (–C
2–OH). Among the anions of the compounds, only picrate and dinitromethanide anions have hydrogen signals at 8.59 ppm and 8.16 ppm respectively. Besides, 13C NMR spectra display the aromatic carbon signals of imidazolium cation at about 137 ppm (C2), 124 ppm (C5), and 123 ppm (C4). The side chain carbon signals of imidazolium cations in compounds 2a–b and 4a–f are at about 71 ppm (–
H2–ONO2), 47 ppm (N–
H2–), and 36 ppm (N–
H3), while those of 1 and 3a–f are at about 60 ppm (–
H2–OH), 52 ppm (N–
H2–), and 36 ppm (N–
H3). The anions of compounds 2a–b, 3e and 4e without carbon have no carbon signals. The 3,5-dinitro-1,2,4-triazolate anion of 3a and 4a has a carbon signal at 163 ppm. The picrate anions of compound 3b and 4b have carbon signals at 161–162, 141–142, 126–127, and 125–127 ppm. The 3-nitro-5-oxo-1,2,4-triazolate anions of 3c and 4c exhibit carbon signals at about 165 and 160 ppm. The dinitromethanide anions of compounds 3d and 4d have a carbon signal at about 123 ppm. The tetrazolate anions of compounds 3f and 4f exhibit carbon signals at 167–169 ppm.
Thermal properties
The thermal stabilities of the new energetic compounds were determined by thermogravimetric analysis (TGA) (Fig. 1). The decomposition temperatures obtained from TG data are listed in Table 1. Comparatively, the decomposition temperatures of EILs 3a–f with a N-hydroxyethyl side chain are much higher than those of compounds 4a–f with a N-nitrooxyethyl side chain, when their anions are the same. This indicates that the introduction of a nitrooxy group into the side chains lowered the decomposition temperatures, rendering the corresponding EILs a poorer thermo-stability. Generally, energetic salts 2a, 3a–f, 4a–b, and 4e–f possess good thermal stabilities, of which the decomposition temperatures are higher than 150 °C. However, EILs 3d and 4d with a dinitromethanide anion have the lowest decomposition temperatures at 151 °C and 138 °C. When heated up to 650 °C, a nearly 100% weight loss was observed for compounds 3d–e and 4e–f, while compounds 3b and 4b with picrate anions had a very high weight residue above 20% (Fig. 1). It is probably because the oxygen-rich dinitromethanide, dinitramide, or 5-nitrotetrazolate anions provide compounds 3d–e and 4e–f a better oxygen balance than picrate anion does.
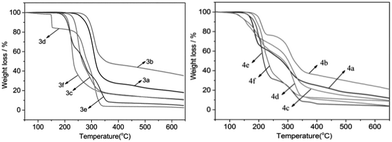 |
| Fig. 1 TG curves of compounds 3a–f (left) and 4a–f (right) in argon atmosphere. | |
Table 1 Physicochemical properties of compounds 2a, 3a–f and 4a–f
Compound |
Appearancea |
Tgb (°C) |
Tmc (°C) |
Tdd (°C) |
s: solid at r.t., l: liquid at r.t. Glass transition temperature. Melting point. Decomposition temperature. Ref. 28, KDNT: potassium 3,5-dinitro-1,2,4-triazolate. Ref. 40, KPic: potassium picrate. Ref. 41, KNTO: potassium 3-nitro-1,2,4-triazolate-one. Ref. 31, KDNM: potassium dinitromethanide. Ref. 42, KDN: potassium dinitramide. Ref. 34, NaNT: sodium 5-nitrotetrazolate. |
2a |
l |
−51 |
— |
165 |
3a |
s |
— |
94 |
263 |
3b |
s |
−22 |
89 |
283 |
3c |
s |
−24 |
100 |
231 |
3d |
l |
−73 |
— |
151 |
3e |
l |
−77 |
— |
200 |
3f |
s |
−50 |
79 |
207 |
4a |
s |
−21 |
55 |
169 |
4b |
s |
−58 |
81 |
198 |
4c |
l |
−12 |
— |
139 |
4d |
l |
−51 |
— |
138 |
4e |
l |
−64 |
— |
171 |
4f |
s |
−36 |
45 |
185 |
KDNTe |
s |
— |
— |
265 |
KPicf |
s |
— |
— |
359 |
KNTO·H2Og |
s |
— |
— |
230 |
KDNMh |
s |
— |
— |
220 |
KDNi |
s |
— |
— |
228 |
NaNT·2H2Oj |
s |
— |
— |
200 |
DTG curves of the energetic compounds show that compounds 3a, 3b, and 3f have one weight loss process, compounds 2a, 3c, 3d and 4a–d generally have two weight loss processes, and compounds 3e and 4e–f have three weight loss processes (see ESI†). Generally, the first weight loss processes of compounds 4a–f are surely due to the decomposition of their N-nitrooxyethyl side chains, as indicated by the structure and thermo-stability differences between compounds 3a–f and compounds 4a–f.
The thermal properties of compounds 2a, 3a–f and 4a–f were further determined by differential scanning calorimetry (DSC) (see ESI†). The melting points and glass transition temperatures obtained from DSC data are also listed in Table 1. DSC data show that the energetic salts are typical ionic liquids with glass transition temperatures from −77 °C to −12 °C and melting points below 100 °C. Importantly, all the EILs have a very wide liquid temperature range over 100 °C (Table 1). Besides, EILs 3a–c, 3f, and 4b have a melting point from 79 °C to 100 °C and a much higher decomposition temperature from 198 °C to 283 °C, which may be potential choices for melt-cast explosives. Generally, when the anions are the same, the melting temperatures of 3a–f with a hydroxyethyl side chain are higher than those of 4a–f with a nitrooxyethyl side chain, while their glass transition temperatures are just the opposite (Table 1). It shows that the introduction of nitrooxy group lowered the melting points, but increased the glass transition temperatures of the ionic liquids.
Compounds 2a, 3d, 3e, 4c, 4d and 4e are liquid at room temperature, of which the secondary heating DSC curves are shown in ESI.† During the secondary heating process, only glass transitions were observed without melting or crystallization. Compounds 3b, 3c, 4a, and 4f are solid at room temperature, of which the first heating DSC curves and secondary heating DSC curves are quite different (see ESI†). During the first heat process, only melting peaks were observed without glass transition and crystallization peaks. Comparatively, there are no melting peaks in the secondary heating DSC curves, whereas obvious glass transition processes are observed. It is probably because that compounds 3b, 3c, 4a, and 4f have very slow crystallization rates, of which crystal states could not be formed during the cooling and heating processes. Compounds 3f and 4b are solid at room temperature, of which the first and secondary heating DSC curves are different clearly (see ESI†). During the first heating process, only melting peaks were observed, where the glass states of compounds 3f and 4b turned into a liquid state. During the secondary heating process, there are glass transition processes at −50 °C and −12 °C, where compounds 3f and 4b turned into supercooled liquids. When further heated, compounds 3f and 4b crystallized and then melted into a normal liquid.
Solubility
Solubility test shows that all of the salts were soluble in most polar solvents such as acetonitrile, acetone, ethanol, methanol, dimethyl sulfoxide, and water, but insoluble in diethyl ether, petroleum ether, ethyl acetate, and dichloromethane.
Crystal structures
Slow recrystallization of compounds 3a, 3b, and 4b from acetone at room temperature gave crystals suitable for X-ray diffraction analysis. The crystallographic data are summarized in Table 2. Selected bond lengths, bond angles, and some further information about crystal-structure determinations are given in the ESI.† The molecular structures and the packing diagrams of compounds 3a, 3b, and 4b are shown in Fig. 2–4, respectively.
Table 2 Crystal data and structure refinement parameters of compounds 3a, 3b and 4b
Compound |
3a |
3b |
4b |
CCDC number |
1550836 |
1550837 |
1550835 |
Empirical formula |
C8H11N7O5 |
C12H13N5O8 |
C12H12N6O10 |
Formula weight |
285.24 |
355.27 |
400.28 |
Temperature [K] |
293.15 |
293.15 |
293.15 |
Crystal system |
Triclinic |
Monoclinic |
Monoclinic |
Space group |
P![[1 with combining macron]](https://www.rsc.org/images/entities/char_0031_0304.gif) |
P21/n |
P21/n |
a [Å] |
6.7821(4) |
14.1861(4) |
11.0072(13) |
b [Å] |
9.5516(6) |
7.38696(18) |
8.8756(7) |
c [Å] |
10.3880(5) |
15.4711(4) |
17.3685(17) |
α [°] |
94.882(4) |
90.00 |
90.00 |
β [°] |
96.973(4) |
110.333(3) |
99.588(10) |
γ [°] |
110.449(5) |
90.00 |
90.00 |
Volume [Å3] |
619.95(6) |
1520.22(7) |
1673.1(3) |
Z |
2 |
4 |
4 |
ρcalc [g cm−3] |
1.528 |
1.552 |
1.589 |
μ [mm−1] |
1.115 |
1.154 |
1.235 |
F(000) |
296.0 |
736.0 |
824.0 |
Crystal size [mm3] |
0.52 × 0.48 × 0.44 |
0.48 × 0.46 × 0.42 |
0.46 × 0.38 × 0.32 |
2θ range [°] |
12.29–133.18 |
10.46–133.18 |
8.88–133.18 |
Reflections collected |
6297 |
8196 |
10 675 |
Independent reflections/Rint |
2179/0.0224 |
2679/0.0273 |
2913/0.0355 |
Data/restraints/parameters |
2179/0/184 |
2679/0/229 |
2913/0/255 |
Goodness-of-fit on F2 |
1.040 |
1.069 |
1.112 |
R1/wR2 [I ≥ 2σ (I)] |
0.0408/0.1130 |
0.0479/0.1292 |
0.0702/0.2069 |
R1/wR2 [all data] |
0.0429/0.1152 |
0.0525/0.1334 |
0.0813/0.2307 |
Largest diff. peak/hole [e Å−3] |
0.20/−0.22 |
0.54/−0.34 |
0.42/−0.33 |
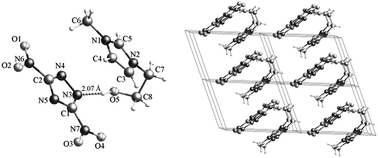 |
| Fig. 2 Molecular structure and packing diagram of compound 3a. | |
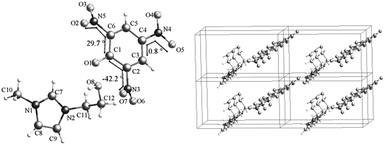 |
| Fig. 3 Molecular structure and packing diagram of compound 3b. | |
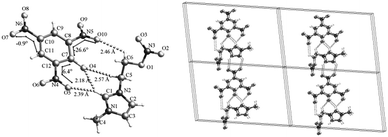 |
| Fig. 4 Molecular structure and packing diagram of compound 4b. | |
Compound 3a crystallizes in a triclinic space group P
with a density of 1.528 g cm−3 and a unit cell volume of 619.95 Å3 (Table 2). The molecular unit of compound 3a consists of one 2,5-dinitrotriazolate anion and one 1-hydroxyethyl-3-methylimidazolium cation without coordinated water (Fig. 2). All the atoms of 2,5-dinitrotriazolate anion are almost coplanar. The C–N bonds in the 2,5-dinitrotriazolate ring have nearly same bond lengths of 1.33 Å, showing a conjugation system existed. The bond lengths of N1–C5, N2–C5, N1–C4, and N2–C3 in the imidazolium ring of compound 4b are 1.32, 1.32, 1.38, and 1.38 Å, respectively. These C–N bonds are much shorter than a classical C–N single bond (1.47 Å), whereas longer than a C
N double bond (1.31 Å), indicating a conjugation existed in the imidazolium ring. Comparatively, the bond lengths of N1–C5 and N2–C5 are shorter than those of N1–C4 and N2–C3, which is consistent with 1,3-disubstituted imidazoliums.43 There is a strong intramolecular O5–H⋯N3 hydrogen bond with a bond length of 2.07 Å. The crystal structure is formed by intramolecular and intermolecular O–H⋯N hydrogen bonds, π-stack as well as the charge attraction between imidazolium cation and 2,5-dinitrotriazolate anion. The O5–H⋯N3 strong hydrogen bond and the charge attraction between the cation and the anion make compound 3a have a U-shape layout in the crystal, as shown in Fig. 2.
Compound 3b crystallizes in a monoclinic space group P21/n with a density of 1.552 g cm−3 and a unit cell volume of 1520.22 Å3 (Table 2). The molecular unit of compound 3b consists of one picrate anion and one 1-hydroxyethyl-3-methylimidazolium cation (Fig. 3). Interestingly, there is no intramolecular hydrogen bond observed although there is a hydroxyl group in compound 3b. Actually, the three nitro groups of picrate anion are not coplanar. The C2–NO2 has a very big O7–N3–C2–C1 torsion angle of −42.2° due to the steric hindrances of both O1 and the hydroxyethyl side chain (Fig. 3). The O8–C12 bond length of 1-hydroxyethyl-3-methylimidazolium cation is 1.408 Å, which is much longer than that of O1–C1 (1.241 Å) in picrate anion. The difference originates from the delocalization of the negative charge on the benzene ring. In addition, the π–π conjugation between the nitro group and the aromatic ring results in the C–N bond lengths in picrate anion range from 1.443 to 1.451 Å, which are a little shorter than those of C–N bonds in the imidazolium cation (1.465 and 1.474 Å).
Compound 4b crystallizes in a monoclinic space group P21/n with a density of 1.589 g cm−3 and a unit cell volume of 1673.1(3) Å3 (Table 2). Fig. 4 shows that the molecular unit of compound 4b consists of one picrate anion and one 1-methyl-3-nitrooxyimidazolium cation without coordinated water. Interestingly, there are no common hydrogen bonds but several intra- and intermolecular C–H⋯O hydrogen bonds formed in the crystal system (Fig. 4). The C1–H on the imidazolium is acidic, which interact with O4 and O5 forming two hydrogen bonds via a six-membered ring. The resulting C1–H⋯O4 and C1–H⋯O5 have a hydrogen bond length of 2.18 Å and 2.39 Å, which is significantly shorter than the sum of their van der Waals radii (d0, 2.72 A°). The bond length of C1–H⋯O4 is a little shorter than that of C1–H⋯O5 probably because that O4 is deprotonated and negatively charged. It is the C1–H⋯O4 and C1–H⋯O5 hydrogen bonds that make the torsion angle of O5–N4–C12–C7 (+6.4°) remarkably smaller than that of O10–N5–C8–C7 (−26.6°). Besides, C5–H and C6–H activated respectively by a quaternary ammonium nitrogen (N2) and a nitrooxy group are reasonable hydrogen bond donors.44–46 The C5–H⋯O4 (d = 2.57 Å) and C6–H⋯O10 (d = 2.46 Å) hydrogen bonds render imidazolium ring and the nitrooxy group a gauche form conformation, as shown in Fig. 4. Furthermore, the C2–H and C3–H on imidazolium ring formed two intermolecular C–H⋯O hydrogen bonds with the two nitro oxygens of picrate in another molecule (Fig. 4). The 2D layer structure is formed by intra- and intermolecular C–H⋯O hydrogen bonds as well as the charge attraction between imidazolium cation and picrate anion, and the 2D layer stacks are parallel to the crystallographic c axis.
Energetic properties
As one of the most important properties of the EILs, density can be calculated by Gaussian software. The molar volume can be calculated with Monte-Carlo method. Furthermore, the density can be readily extracted. Heat of formation is one of the most essential features of the compounds. On the basis of a Born–Haber energy cycle (Scheme 2), the heats of formation of all the EILs can be simplified by the expression shown in eqn (1). |
ΔH0298 (ionic salts, 298 K) = ∑ΔH0298 (cation, 298 K) + ∑ΔH0298 (anion, 298 K) − ΔHL
| (1) |
where ΔHL is the lattice energy of the ionic liquids, which can be predicted by eqn (2) suggested by Jenkins et al. |
ΔHL = UPOT + [p(nM/2 − 2) + q(nX/2 − 2)]RT
| (2) |
where nM and nX depend on the nature of the ions Mp+ and Xq−, respectively, and are equal to 3 for monatomic ions, 5 for linear polyatomic ions, and 6 for nonlinear polyatomic ions. The equation for lattice potential energy UPOT has the form shown in eqn (3).where ρ is the density (g cm−1), M is the chemical formula mass of the ionic liquid (g mol−1). The coefficients γ and δ are obtained from literatures.47 For MX (1
:
1) salts, γ is 1981.2 kJ mol−1 and δ is 103.8 kJ mol−1.
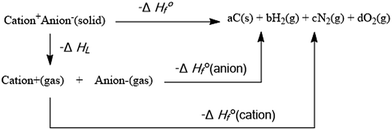 |
| Scheme 2 Born–Haber cycle for the formation of energetic ionic liquids. | |
The molar enthalpies of formation of energetic anions are obtained from literatures.14,23,48 The molar enthalpy of formation of the cation is computed by Gaussian software based on the designed isodesmic reaction (Scheme 3). The enthalpy of reaction (ΔH0r 298) is obtained by combining the B3LYP/6-31++G** energy differences for the reaction, the scaled zero-point energies, and other thermal factors.
 |
| Scheme 3 Isodesmic reactions of imidazolium cations. | |
The detonation velocity (D) and detonation pressure (P) of energetic compounds could be predicted by eqn (4) and (5).49,50
|
D = 1.01(N 1/2Q1/2)1/2(1 + 1.30ρ)
| (4) |
|
P = 1.558ρ2N 1/2Q1/2
| (5) |
The calculated densities, lattice energies, molar enthalpies of formation and detonation parameters of the EILs are listed in Table 3.
Table 3 Calculated densities, lattice energies, molar enthalpies of formation and detonation parameters of compounds 2a, 3a–f and 4a–f (298 K)
Compound |
ρ1 (ρ2)a (g cm−3) |
ΔfHocb (kJ mol−1) |
ΔfHoac (kJ mol−1) |
ΔHLd (kJ mol−1) |
ΔfHome (kJ mol−1) |
Qf (cal g−1) |
Dg (km s−1) |
Ph (GPa) |
ρ1: calculated density, ρ2: pycnometric density. Calculated molar enthalpy of formation of cations. Molar enthalpy of formation of anions. Molar lattice energy. Calculated molar enthalpy of formation of ionic liquids. Detonation heat. Detonation velocity. Detonation pressure. Single crystal density. |
2a |
1.53 (1.51) |
528.61 |
−307.90 |
478.79 |
−258.08 |
1697.72 |
7.53 |
22.65 |
3a |
1.53 (1.53)i |
481.22 |
−41.20 |
455.86 |
−15.84 |
1026.08 |
6.51 |
16.98 |
3b |
1.59 (1.55)i |
481.22 |
−108.50 |
435.54 |
−62.82 |
1297.88 |
6.73 |
18.60 |
3c |
1.49 (1.47) |
481.22 |
63.90 |
465.15 |
79.97 |
976.51 |
6.39 |
16.04 |
3d |
1.48 (1.48) |
481.22 |
−221.70 |
476.02 |
−216.50 |
1021.32 |
6.42 |
16.14 |
3e |
1.51 (1.50) |
481.22 |
−162.00 |
478.33 |
−159.11 |
1401.85 |
7.20 |
20.57 |
3f |
1.47 (1.47) |
481.22 |
112.80 |
470.39 |
123.63 |
841.00 |
6.24 |
15.15 |
4a |
1.59 (1.55) |
528.61 |
−41.20 |
443.53 |
43.88 |
1191.37 |
7.06 |
20.50 |
4b |
1.64 (1.59)i |
528.61 |
−108.50 |
425.74 |
−5.63 |
1339.40 |
7.12 |
21.25 |
4c |
1.56 (1.53) |
528.61 |
63.90 |
451.52 |
140.99 |
1244.79 |
6.93 |
19.48 |
4d |
1.55 (1.52) |
528.61 |
−221.70 |
460.75 |
−153.84 |
1268.27 |
7.09 |
20.34 |
4e |
1.59 (1.54) |
528.61 |
−162.00 |
462.70 |
−96.09 |
1459.08 |
7.63 |
23.84 |
4f |
1.54 (1.52) |
528.61 |
112.80 |
455.98 |
185.43 |
1164.08 |
6.84 |
18.80 |
TNT |
1.60 |
— |
— |
— |
−74.50 |
— |
6.84 |
20.29 |
RDX |
1.81 |
— |
— |
— |
61.55 |
— |
8.75 |
34.70 |
As shown in Table 3, the calculated densities of compounds 2a, 3a–f, and 4a–f are from 1.47 to 1.64 g cm−3, which are generally close to their crystal or pycnometric densities. Compounds 2a, 3a, 3b, 3d, 3e, 4b, 4d and 4e possess negative heats of formation, which mainly result from the large negative heats of formation of their anions. It is noteworthy that the 5-nitrotetrazolate anion possesses a positive heat of formation of 112.80 kJ mol−1 and its salts 3f and 4f have a much higher enthalpy of formation than those of other salts. Generally, with the same anions, EILs 4a–f with an energetic nitrooxy group in their side chains have a higher enthalpy of formation than EILs 3a–f without a nitrooxy group (Table 3).
When the anions are the same, the detonation velocities and detonation pressures of ionic liquids 4a–f with a nitrooxyethyl side chain are much higher than those of ionic liquids 3a–f with a hydroxyethyl side chain (Table 3). It indicates that the introduction of nitrooxy energetic group can improve their energetic properties. The detonation velocities of ionic liquids 2a and 4a–f are 6.84–7.63 km s−1, which are between those of TNT and RDX. The detonation pressures of ionic liquids 2a, 3e, 4a, 4b, 4d, and 4e are better than that of TNT. Generally, some of the EILs have good energetic properties which have potentials as new energetic materials.
Experimental section
Caution
We did not experience any problems in handling these compounds, though some of them were sensitive to shock, heat, or friction. We strongly suggested that they should be synthesized in a very small scale and handled with extreme care using all of the standard safety precautions because of their high nitrogen content and rather high heats of formation.
Materials and methods
All chemical reagents of analytically pure grade were obtained from commercial sources and used as received without additional purification. UV-Vis spectra were recorded by an UV-1800PC ultraviolet and visible spectrophotometer. IR spectra were recorded by using KBr plates in the range 4000–450 cm−1 by a Perkin Elmer Spectrum 100 FT-IR spectrometer. 1H NMR and 13C NMR spectra were recorded on a Bruker Avance III 600 nuclear magnetic resonance spectrometer operating at 600 MHz and 151 MHz, respectively. Meanwhile, a part of 1H NMR spectra were recorded on a Bruker Advance 400 MHz instrument with deuterium oxide (D2O) as the solvent. All 1H and 13C chemical shifts were reported in ppm relative to tetramethylsilane (TMS). Mass spectra for salts were determined using an electrospray ion source (ESI) in Varian 1200 L high-performance liquid chromatography tandem mass spectrometry (LC-MS). M+ and M− were the mass of the cation and anion, respectively. Elemental analyses were performed using a Netsch STA 429 simultaneous thermal analyser. The melting and decomposition points of all the salts were recorded on a Q2000 differential scanning calorimeter (DSC) from TA Instruments at a scan rate of 10 °C min−1. Thermal decomposition of the salts up to 650 °C in argon (flow rate 30 mL min−1) were performed by a Perkin Elmer Pyris 1 thermal gravimetric analysis (TGA) at a heating rate of 10 °C min−1.
Synthesis of ionic liquids
1-(2-Hydroxyethyl)-3-methylimidazolium chloride (1). A mixture of 1-methylimidazole (5.2 g, 63.5 mmol) and 2-chloroethanol (5.2 g, 64.0 mmol) was added into a 100 mL two-necked round-bottomed flask that was fitted with a reflux condenser. The mixture was stirred at 80 °C for 20 h, to which 10 mL anhydrous ethanol was then added. The resulting mixture was poured into 100 mL cold ethyl acetate and cooled to room temperature. After decanting the ethyl acetate layer, the crude product was washed with diethyl ether (3 × 10 mL) and dried in vacuum overnight to give compound 1 as fairly hygroscopic white crystals (9.1 g, 55.9 mmol, 88% yield). UV-Vis (H2O) λmax: 227 nm; 1H NMR (600 MHz, D2O), δ 7.59 (d, J = 2.4 Hz, 1H, C5–H), 7.54 (d, J = 2.4 Hz, 1H, C4–H), 4.38 (t, J = 4.8 Hz, 2H, N–CH2–), 3.96 (t, J = 4.8 Hz, 2H, –CH2–OH), 3.95 (s, 3H, N–CH3); 13C NMR (151 MHz, D2O), δ 136.25 (C2), 123.70 (C5), 122.59 (C4), 59.96 (–CH2–OH), 51.72 (N–CH2–), 36.02 (N–CH3); FT-IR (KBr) v: 3411.3, 3156.4, 3111.2, 2958.9, 2886.2, 1635.5, 1574.8, 1450.2, 1357.6, 1167.8, 1068.8, 944.9, 870.8, 841.3, 753.7, 651.2 cm−1; ESI-MS m/z (%): 126.9 (M+, 100).
1-Methyl-3-(2-nitrooxyethyl)imidazolium nitrate (2a). Compound 1 (2.4 g, 15.0 mmol) was added in small portions to a two-necked round-bottomed flask containing fuming nitric acid (10 mL, 240.0 mmol) and cooled by a water-ice bath to maintain the temperature below 10 °C. After addition, the reaction mixture was stirred for 1 h at 10–15 °C and then for 6 h at room temperature. The mixture was poured into 150 mL cold diethyl ether. After stirring for 2 h, the diethyl ether layer was decanted. The crude product was washed with diethyl ether (3 × 10 mL) and dried in vacuum overnight to obtain 2a as a colorless liquid (3.0 g, 12.8 mmol, 85% yield). UV-Vis (H2O) λmax: 226 nm; 1H NMR (600 MHz, D2O), δ 8.69 (s, 1H, C2–
), 7.42 (t, J = 1.8 Hz, 1H, C5–
), 7.34 (t, J = 1.8 Hz, 1H, C4–
), 4.78 (t, J = 4.2 Hz, 2H, –C
2–ONO2), 4.51 (t, J = 4.2 Hz, 2H, N–C
2–), 3.78 (s, 3H, N–C
3); 13C NMR (151 MHz, D2O) δ 136.68 (C2), 124.65 (C5), 122.54 (C4), 70.85 (–
H2–ONO2), 46.51 (N–
H2–), 35.75 (N–
H3); FT-IR (KBr) v: 3153.9, 3110.2, 2968.7, 2853.0, 1642.4, 1577.9, 1565.6, 1377.5, 1282.6, 1171.6, 1036.7, 1005.4, 895.9, 848.0, 826.3, 754.9, 707.8, 623.4 cm−1; ESI-MS m/z (%): 172.0 (M+, 100), 61.8 (M−, 100). Anal. calcd for C6H10N4O6: C 30.78, H 4.30, N 23.93; found C 30.74, H 4.31, N 23.91.
Pretreatment of Amberlite 717
Amberlite 717 was immersed in distilled water for 24 h and filtered. Then the resin was immersed in brine for another 24 h in order that all OH− was replaced by Cl−. At last, the resin was washed by deionized water several times until there were no precipitations formed in the filtrate by addition of a silver nitrate solution.
1-Methyl-3-(2-nitrooxyethyl)imidazolium chloride (2b). Anion exchange resin (40 g) was added into a two-necked round-bottomed flask containing compound 2a (10.3 g, 44.0 mmol) in 10 mL water and 40 mL methanol. Then the mixture was stirred at room temperature for 12 h. The resin was removed by vacuum filtration, giving a colorless filtrate. The solvent was removed by a rotary evaporator to yield 2b as a slight yellow solid (9.0 g, 43.4 mmol, 99%). 1H NMR (600 MHz, DMSO-d6) δ 9.19 (s, 1H, C2–
), 7.81 (t, J = 1.8 Hz, 1H, C5–
), 7.74 (t, J = 1.8 Hz, 1H, C4–
), 4.94 (t, J = 4.8 Hz, 2H, –C
2–ONO2), 4.62 (t, J = 4.8 Hz, 2H, N–C
2–), 3.88 (s, 3H, N–C
3); 13C NMR (151 MHz, D2O) δ 136.91 (C2), 123.85 (C5), 122.53 (C4), 71.22 (–
H2–ONO2), 46.51 (N–
H2–), 35.79 (N–
H3).
General procedures for the preparation of energetic ionic liquids 3a–f and 4a–f
Compounds 1 or 2b was dissolved in acetone, and then one equivalent of KDNT, KP, KNTO·H2O, KDNM, KDN, or NaNT·2H2O was added. The precipitation of white chloride salts was observed intermediately and the resulting slurry was stirred at room temperature for 24 h. After removal of the precipitation by vacuum filtration, the solvent was evaporated via a rotary evaporator. The resulting crude product was dissolved in acetone to remove the residual chloride salts by vacuum filtration, the filtrate was evaporated, and then the residue was washed twice with Et2O.
1-(2-Hydroxyethyl)-3-methylimidazolium 3,5-dinitro-1,2,4-triazolate (3a). Slightly yellow solid, yield: 93.5%. UV-Vis (H2O) λmax: 214, 285 nm; 1H NMR (600 MHz, DMSO-d6) δ 9.08 (s, 1H, C2–H), 7.72 (t, J = 1.8 Hz, 1H, C5–H), 7.69 (t, J = 1.8 Hz, 1H, C4–H), 5.18 (t, J = 4.8 Hz, 1H, –CH2–O
), 4.22 (t, 2H, J = 4.8 Hz, N–C
2–), 3.87 (s, 3H, N–C
3), 3.73 (q, J = 4.8 Hz, 2H, –C
2–OH); 13C NMR (151 MHz, DMSO-d6) δ 163.32 (–
–NO2), 137.26 (C2), 123.80 (C5), 123.11 (C4), 59.79 (N–
H2–), 52.09 (–
H2–OH), 36.12 (N–
H3); FT-IR (KBr) v: 3395.3, 3149.2, 2854.6, 2755.1, 1555.6, 1538.6, 1503.4, 1413.3, 1390.0, 1385.6, 1300.5, 1100.4, 1049.6, 850.8, 836.5, 771.2, 651.4, 605.6 cm−1; ESI-MS m/z (%): 126.9 (M+, 100), 157.7 (M−, 100), 316.8 (32); anal. calcd for C8H11N7O5: C 33.69, H 3.89, N 34.38; found C 33.65, H 3.90, N 34.37.
1-(2-Hydroxyethyl)-3-methylimidazolium picrate (3b). Yellow solid, yield: 97.9%. UV-Vis (H2O) λmax: 221, 354 nm; 1H NMR (600 MHz, DMSO-d6) δ 9.10 (s, 1H, C2–
), 8.61 (s, 2H, picrate), 7.73 (t, J = 1.8 Hz, 1H, C5–
), 7.70 (t, J = 1.8 Hz, 1H, C4–
), 4.23 (t, J = 4.8 Hz, 2H, N–C
2–), 3.88 (s, 3H, N–C
3), 3.74 (t, J = 4.8 Hz, 2H, –C
2–OH); 13C NMR (151 MHz, D2O) δ 162.13 (picrate C1′), 140.79 (picrate C2′ C6′), 136.37 (C2), 127.09 (picrate C4′), 126.65 (picrate C3′ C5′), 123.61 (C5), 122.48 (C4), 59.84 (–
H2–OH), 51.55 (N–
H2–), 35.74 (N–
H3); FT-IR (KBr) v: 3417.7, 3155.8, 3086.3, 2959.6, 2894.5, 2824.6, 1630.7, 1611.8, 1563.3, 1496.8, 1483.1, 1438.6, 1369.5, 1334.6, 1277.7, 1163.7, 1081.1, 937.5, 907.9, 795.9, 746.0, 704.0, 651.4, 621.8 cm−1; ESI-MS m/z (%): 126.8 (M+, 100), 227.7 (M−, 100); anal. calcd for C12H13N5O8: C 40.57, H 3.69, N 19.71; found C 40.62, H 3.58, N 19.65.
1-(2-Hydroxyethyl)-3-methylimidazolium 3-nitro-5-oxo-1,2,4-triazolate (3c). Orange solid, yield: 94.1%. UV-Vis (H2O) λmax: 230, 344 nm; 1H NMR (400 MHz, D2O) δ 7.49 (d, J = 2.0 Hz, 1H, C5–
), 7.43 (d, J = 2.0 Hz, 1H, C4–
), 4.30 (t, J = 4.8 Hz, 2H, N–C
2–), 3.91 (t, J = 4.8 Hz, 2H, –C
2–OH), 3.89 (s, 3H, N–C
3); 13C NMR (151 MHz, DMSO-d6) δ 165.31 (![[C with combining low line]](https://www.rsc.org/images/entities/char_0043_0332.gif)
O), 160.56 (
–NO2), 137.42 (C2), 123.74 (C5), 123.13 (C4), 59.80 (–
H2–OH), 52.06 (N–
H2–), 36.10 (N–
H3); FT-IR (KBr) v: 3362.5, 3251.5, 3153.7, 3110.2, 2966.7, 2841.5, 2791.4, 1699.3, 1656.1, 1592.2, 1576.5, 1544.2, 1508.7, 1424.7, 1383.3, 1312.7, 1167.9, 1117.6, 1050.0, 1015.0, 848.8, 812.0, 776.8, 746.8, 619.6 cm−1; ESI-MS m/z (%): 126.9 (M+, 100), 128.7 (M−, 100), 258.8 (38); anal. calcd for C8H12N6O4: C 37.50, H 4.72, N 32.80; found C 37.34, H 4.86, N 32.65.
1-(2-Hydroxyethyl)-3-methylimidazolium dinitromethanide (3d). Yellow viscous liquid, yield: 91.5%. UV-Vis (H2O) λmax: 214, 361 nm; 1H NMR (400 MHz, D2O) δ 7.42 (d, J = 1.2 Hz, 1H, C5–
), 7.36 (d, J = 1.2 Hz, 1H, C4–
), 4.23 (t, J = 4.8 Hz, 2H, N–C
2–), 3.84 (t, J = 4.8 Hz, 2H, –C
2–OH), 3.80 (s, 3H, N–C
3); 13C NMR (151 MHz, DMSO-d6) δ 137.32 (C2), 123.73 (C5), 123.11 (C4), 122.58 (
H(NO2)2), 59.75 (N–
H2–), 52.01 (–
H2–OH), 36.11 (N–
H3); FT-IR (KBr) v: 3372.3, 3148.9, 3110.8, 2957.9, 2884.9, 1638.1, 1570.6, 1462.9, 1413.9, 1365.3, 1300.4, 1170.5, 1079.8, 1003.1, 946.5, 842.3, 784.5, 747.2, 692.2, 650.5 cm−1; ESI-MS m/z (%): 126.9 (M+, 100), 104.6 (M−, 100); anal. calcd for C7H12N4O5: C 36.21, H 5.21, N 24.13; found C 36.18, H 5.23, N 24.11.
1-(2-Hydroxyethyl)-3-methylimidazolium dinitramide (3e). Slightly yellow liquid, yield: 98.8%. UV-Vis (H2O) λmax: 221, 283 nm; 1H NMR (400 MHz, D2O) δ 8.75 (s, 1H, C2–
), 7.51 (d, J = 1.6 Hz, 1H, C5–
), 7.45 (d, J = 1.6 Hz, 1H, C4–
), 4.33 (t, J = 4.0 Hz, 2H, N–C
2–), 3.92 (t, J = 4.0 Hz, 2H, –C
2–OH), 3.91 (s, 3H, N–C
3); 13C NMR (151 MHz, DMSO-d6) δ 137.26 (C2), 123.79 (C5), 123.11 (C4), 59.79 (–
H2–OH), 52.09 (N–
H2–), 36.11 (N–
H3); FT-IR (KBr) v: 3429.4, 3156.3, 3116.5, 2960.5, 2888.1, 1633.1, 1573.5, 1565.7, 1519.9, 1434.8, 1358.7, 1337.8, 1186.6, 1068.6, 1013.0, 828.4, 759.2, 651.7, 622.8 cm−1; ESI-MS m/z (%): 126.9 (M+, 100), 105.6 (M−, 100); anal. calcd for C6H11N5O5: C 30.91, H 4.76, N 30.03; found C 30.99, H 4.62, N 30.17.
1-(2-Hydroxyethyl)-3-methylimidazolium 5-nitrotetrazolate (3f). Slightly yellow solid, yield: 93.2%. UV-Vis (H2O) λmax: 221, 256 nm; 1H NMR (600 MHz, DMSO-d6) δ 9.11 (s, 1H, C2–
), 7.74 (t, J = 1.8 Hz, 1H, C5–
), 7.70 (t, J = 1.8 Hz, 1H, C4–
), 5.23 (t, J = 4.8 Hz, 1H, –CH2–O
), 4.24 (t, J = 4.8 Hz, 2H, N–C
2–), 3.89 (s, 3H, N–C
3), 3.74 (q, J = 4.8 Hz, 2H, –C
2–OH); 13C NMR (151 MHz, D2O) δ 167.54 (–
–NO2), 136.29 (C2), 123.54 (C5), 122.40 (C4), 59.74 (–
H2–OH), 51.52 (N–
H2–), 35.68 (N–
H3); FT-IR (KBr) v: 3410.6, 3153.6, 3114.0, 2960.1, 2881.4, 1631.7, 1574.2, 1564.5, 1542.1, 1437.5, 1416.0, 1384.7, 1355.5, 1341.1, 1169.2, 1072.9, 868.1, 838.0, 763.1, 671.8, 652.4, 623.7 cm−1; ESI-MS m/z (%): 126.8 (M+, 100), 113.8 (M−, 100), 228.7 (43); anal. calcd for C7H11N7O3: C 34.86, H 4.60, N 40.65; found C 34.75, H 4.56, N 40.55.
1-Methyl-3-(2-nitrooxyethyl)imidazolium 3,5-dinitro-1,2,4-triazolate (4a). Slightly yellow solid, yield: 96%. UV-Vis (H2O) λmax: 225, 285 nm; 1H NMR (600 MHz, DMSO-d6) δ 9.17 (s, 1H, C2–
), 7.81 (t, J = 1.8 Hz, 1H, C5–
), 7.74 (t, J = 1.8 Hz, 1H, C4–
), 4.94 (t, J = 4.8 Hz, 2H, –C
2–ONO2), 4.63 (t, J = 4.8 Hz, 2H, N–C
2–), 3.89 (s, 3H, N–C
3); 13C NMR (151 MHz, DMSO-d6) δ 163.32 (–
–NO2), 137.64 (C2), 124.19 (C5), 123.16 (C4), 71.61 (–
H2–ONO2), 46.70 (N–
H2–), 36.33 (N–
H3); FT-IR (KBr) v: 3156.2, 3096.6, 2904.4, 1644.3, 1539.1, 1487.8, 1378.4, 1346.8, 1282.5, 1204.7, 1170.2, 1113.8, 1026.0, 894.5, 856.9, 843.1, 769.0, 756.1, 711.0, 652.5, 627.4 cm−1; ESI-MS m/z (%): 172.0 (M+, 100), 157.8 (M−, 100). Anal. calcd for C8H10N8O7: C 29.10, H 3.05, N 33.93; found C 29.04, H 3.09, N 33.87.
1-Methyl-3-(2-nitrooxyethyl)imidazolium picrate (4b). Yellow solid, yield: 97%. UV-Vis (H2O) λmax: 216, 354 nm; 1H NMR (600 MHz, DMSO-d6) δ 9.16 (s, 1H, C2–
), 8.59 (s, 2H, picrate), 7.79 (t, J = 1.8 Hz, 1H, C5–
), 7.72 (t, J = 1.8 Hz, 1H, C4–
), 4.92 (t, J = 4.8 Hz, 2H, –C
2–ONO2), 4.61 (t, J = 4.8 Hz, 2H, N–C
2–), 3.88 (s, 3H, N–C
3); 13C NMR (151 MHz, DMSO-d6) δ 161.32 (picrate C1′), 142.29 (picrate C2′ C6′), 137.64 (C2), 125.71 (picrate C4′), 124.69 (picrate C3′ C5′), 124.19 (C5), 123.16 (C4), 71.61, (–
H2–ONO2), 46.69 (N–
H2–), 36.33 (N–
H3); FT-IR (KBr) v: 3165.2, 3140.5, 3099.0, 3047.6, 2990.2, 2894.2, 1633.0, 1559.1, 1492.1, 1434.1, 1384.0, 1364.6, 1335.0, 1308.0, 1277.4, 1160.4, 1076.3, 1037.2, 890.4, 856.4, 788.6, 766.0, 710.0, 652.6 cm−1; ESI-MS m/z (%): 172.0 (M+, 100), 227.8 (M−, 100). Anal. calcd for C12H12N6O10: C 36.01, H 3.02, N 21.00; found C 35.95, H 3.06, N 20.95.
1-Methyl-3-(2-nitrooxyethyl)imidazolium 3-nitro-5-oxo-1,2,4-triazolate (4c). Orange liquid, yield: 86%. UV-Vis (H2O) λmax: 225, 344 nm; 1H NMR (600 MHz, DMSO-d6) δ 9.27 (s, 1H, C2–
), 7.83 (t, J = 1.8 Hz, 1H, C5–
), 7.75 (t, J = 1.8 Hz, 1H, C4–
), 4.94 (t, J = 4.8 Hz, 2H, –C
2–ONO2), 4.65 (t, J = 4.8 Hz, 2H, N–C
2–), 3.89 (s, 3H, N–C
3); 13C NMR (151 MHz, DMSO-d6) δ 164.82 (![[C with combining low line]](https://www.rsc.org/images/entities/char_0043_0332.gif)
O), 159.88 (
–NO2), 137.81 (C2), 124.17 (C5), 123.17 (C4), 71.69 (–
H2–ONO2), 46.65 (N–
H2–), 36.29 (N–
H3); FT-IR (KBr) v: 3361.8, 3251.7, 3148.4, 3101.8, 2842.2, 2791.3, 1698.5, 1648.3, 1578.2, 1543.6, 1509.9, 1423.4, 1384.1, 1132.0, 1282.9, 1171.7, 1117.6, 1049.4, 1014.7, 896.6, 849.2, 777.0, 747.2, 709.0, 621.9 cm−1; ESI-MS m/z (%): 172.0 (M+, 100), 128.7 (M−, 100). Anal. calcd for C8H11N7O6: C 31.90, H 3.68, N 32.55; found C 31.83, H 3.73, N 31.52.
1-Methyl-3-(2-nitrooxyethyl)imidazolium dinitromethanide (4d). Brown liquid, yield: 91%. UV-Vis (H2O) λmax: 213, 361 nm; 1H NMR (600 MHz, DMSO-d6) δ 9.17 (s, 1H, C2–
), 8.16 (s, 1H, C
(NO2)2), 7.80 (t, J = 1.8 Hz, 1H, C5–
), 7.73 (t, J = 1.8 Hz, 1H, C4–
), 4.93 (t, J = 4.8 Hz, 2H, –C
2–ONO2), 4.62 (t, J = 4.8 Hz, 2H, N–C
2–), 3.88 (s, 3H, N–C
3); 13C NMR (151 MHz, DMSO-d6) δ 137.68 (C2), 124.18 (C5), 123.79 (
H(NO2)2), 123.16 (C4), 71.63 (–
H2–ONO2), 46.67 (N–
H2–), 36.30 (N–
H3); FT-IR (KBr) v: 3148.8, 3100.7, 3061.7, 2959.7, 2899.3, 1632.7, 1591.5, 1541.4, 1487.4, 1467.1, 1440.2, 1412.4, 1384.0, 1305.4, 1280.7, 1220.2, 1123.2, 1086.7, 1020.1, 1004.9, 893.7, 861.8, 787.5, 750.6, 707.6, 692.3, 666.5 cm−1; ESI-MS m/z (%): 172.0 (M+, 100), 104.6 (M−, 100). Anal. calcd for C7H11N5O7: C 30.33, H 4.00, N 25.27; found C 30.24, H 4.06, N 25.20.
1-Methyl-3-(2-nitrooxyethyl)imidazolium dinitramide (4e). Yellow liquid, yield: 96%. UV-Vis (H2O) λmax: 223, 283 nm; 1H NMR (600 MHz, DMSO-d6) δ 9.15 (s, 1H, C2–
), 7.79 (t, J = 1.8 Hz, 1H, C5–
), 7.72 (t, J = 1.8 Hz, 1H, C4–
), 4.93 (t, J = 4.8 Hz, 2H, –C
2–ONO2), 4.62 (t, J = 4.8 Hz, 2H, N–C
2–), 3.88 (s, 3H, N–C
3); 13C NMR (151 MHz, DMSO-d6) δ 137.61 (C2), 124.14 (C5), 123.13 (C4), 71.56 (–
H2–ONO2), 46.68 (N–
H2–), 36.29 (N–
H3); FT-IR (KBr) v: 3156.1, 3117.5, 2967.8, 2916.8, 1643.0, 1577.5, 1564.1, 1517.5, 1434.0, 1338.3, 1282.9, 1184.4, 1009.4, 895.6, 847.0, 757.0, 708.9, 623.1 cm−1; ESI-MS m/z (%): 172.0 (M+, 100), 105.6 (M−, 100). Anal. calcd for C6H10N6O7: C 25.91, H 3.62, N 30.21; found C 25.81, H 3.69, N 30.15.
1-Methyl-3-(2-nitrooxyethyl)imidazolium 5-nitrotetrazolate (4f). Brown solid, yield: 97%. UV-Vis (H2O) λmax: 217, 255 nm; 1H NMR (600 MHz, D2O) δ 8.73 (s, 1H, C2–
), 7.45 (t, J = 1.8 Hz, 1H, C5–
), 7.37 (t, J = 1.8 Hz, 1H, C4–
), 4.81 (t, J = 4.8 Hz, 2H, –C
2–ONO2), 4.54 (t, J = 4.8 Hz, 2H, N–C
2–), 3.81 (s, 3H, N–C
3); 13C NMR (151 MHz, DMSO-d6) δ 169.2 (–
–NO2), 137.64 (C2), 124.17 (C5), 123.16 (C4), 71.60 (–
H2–ONO2), 46.70 (N–
H2–), 36.34 (N–
H3); FT-IR (KBr) v: 3156.2, 3114.9, 2968.6, 2912.2, 2853.0, 1643.2, 1564.9, 1536.5, 1435.3, 1412.0, 1384.6, 1313.8, 1282.9, 1171.4, 1024.7, 896.0, 837.5, 755.0, 707.3, 672.5, 623.6 cm−1; ESI-MS m/z (%): 172.0 (M+, 100), 113.7 (M−, 100). Anal. calcd for C7H10N8O5: C 29.38, H 3.52, N 39.15; found C 29.34, H 3.56, N 39.12.
Conclusions
A series of imidazolium-based energetic salts with a hydroxyethyl or nitrooxyethyl side chain were easily synthesized and characterized through NMR, IR, MS, UV/Vis, elemental analysis (EA), DSC, TGA, and/or single crystal X-ray diffraction. DSC analysis shows that the energetic salts are all typical ionic liquids with a wide liquid temperature over 100 °C. TGA data show that the EILs possess good thermal stabilities with decomposition temperatures above 165 °C, except for compounds 3d, 4c, and 4d. Besides, compounds 3a–c, 3f, and 4b have a melting temperature from 79 °C to 100 °C and a much higher decomposition temperature from 198 °C to 283 °C, which may be suitable choices for melt-cast explosives. The calculated detonation velocities of ionic liquids 2a and 4a–f are 6.84–7.63 km s−1, which are between those of TNT and RDX. The calculated detonation pressures of ionic liquids 2a, 3e, 4a, 4b, 4d, and 4e are better than that of TNT. Data show that the introduction of the nitrooxy group lowered the melting points and decomposition temperatures, while increased the glass transition temperatures, and improved the energetic properties of the EILs. Solubility experiment shows that all the EILs have a good solubility in most polar solvents. Generally, these EILs have good stabilities and energetic properties, which could be applied as potential energetic materials.
Acknowledgements
The authors are grateful to China Academy of Engineering Physics for financial support (No. 15zh0083) and Dr Liangchun Li for his kindly support in the energetic property calculations.
Notes and references
- J. S. Wilkes, Green Chem., 2002, 4, 73–80 RSC.
- J. Levillain, G. Dubant, I. Abrunhosa, M. Gulea and A. C. Gaumont, Chem. Commun., 2003, 2914–2915 RSC.
- M. Smiglak, A. Meltin and R. D. Rogers, Acc. Chem. Res., 2007, 40, 1182–1192 CrossRef CAS PubMed.
- J. P. Hallett and T. Welton, Chem. Rev., 2011, 111, 3508–3576 CrossRef CAS PubMed.
- T. Welton, Chem. Rev., 1999, 99, 2071–2084 CrossRef CAS PubMed.
- M. A. P. Martins, C. P. Frizzo, D. N. Moreira, N. Zanatta and H. G. Bonacorso, Chem. Rev., 2008, 108, 2015–2050 CrossRef CAS PubMed.
- R. Skoda-Földes, Molecules, 2014, 19, 8840–8884 CrossRef PubMed.
- F. Jutz, J. M. Andanson and A. Baiker, Chem. Rev., 2011, 111, 322–353 CrossRef CAS PubMed.
- V. M. Muzalevskiy, A. V. Shastin, N. G. Shikhaliev, A. M. Magerramov, A. N. Teymurova and V. G. Nenajdenko, Tetrahedron, 2016, 72(45), 7159–7163 CrossRef CAS.
- A. Singh and H. K. Chopra, Curr. Org. Synth., 2017, 14(4), 488–510 CrossRef CAS.
- S. Pandey, Anal. Chim. Acta, 2006, 556, 38–45 CrossRef CAS PubMed.
- D. A. Walsh, K. R. J. Lovelock and P. Licence, Chem. Soc. Rev., 2010, 39, 4185–4194 RSC.
- D. M. Cabral, P. C. Howlett and D. R. MacFarlane, Electrochim. Acta, 2016, 220(1), 347–353 CrossRef CAS.
- R. H. Wang, H. X. Gao, C. F. Ye and J. M. Shreeve, Chem. Mater., 2007, 19, 144–152 CrossRef CAS.
- J. Dupont, G. S. Fonseca, A. P. Umpierre, P. F. P. Fichtner and S. R. Teixeira, J. Am. Chem. Soc., 2002, 124, 4228–4229 CrossRef CAS PubMed.
- E. Mourad, L. Coustan, P. Lannelongue, D. Zigah, A. Mehdi, A. Vioux, S. A. Freunberger, F. Favier and O. Fontaine, Nat. Mater., 2017, 16, 446–453 CrossRef CAS PubMed.
- D. R. MacFarlane, M. Forsyth, P. C. Howlett, M. Kar, S. Passerini, J. M. Pringle, H. Ohno, M. Watanabe, F. Yan, W. Zheng, S. Zhang and J. Zhang, Nat. Rev. Mater., 2016, 1, 1–15 Search PubMed.
- Q. H. Zhang and J. M. Shreeve, Chem. Rev., 2014, 114, 10527–10574 CrossRef CAS PubMed.
- R. H. Wang, H. Y. Xu, Y. Guo, R. J. Sa and J. M. Shreeve, J. Am. Chem. Soc., 2010, 132, 11904–11905 CrossRef CAS PubMed.
- R. Fareghi-Alamdari, F. Ghorbani-Zamani and N. Zekri, RSC Adv., 2016, 6, 26386–26391 RSC.
- E. Thomas, K. P. Vijayalakshmi and B. K. George, RSC Adv., 2015, 5, 71896–71902 RSC.
- G. H. Tao, Y. Guo, Y. Joo, B. Twamley and J. M. Shreeve, J. Mater. Chem., 2008, 18, 5524–5530 RSC.
- L. He, G. H. Tao, D. A. Parrish and J. M. Shreeve, Inorg. Chem., 2011, 50, 679–685 CrossRef CAS PubMed.
- S. Schneider, T. Hawkins, M. Rosander, J. Mills, A. Brand, L. Hudgens, G. Warmoth and A. Vij, Inorg. Chem., 2008, 47, 3617–3624 CrossRef CAS PubMed.
- E. Sebastiao, C. Cook, A. Hu and M. Murugesu, J. Mater. Chem. A, 2014, 2, 8153–8173 CAS.
- W. Liu, W. L. Liu and S. P. Pang, RSC Adv., 2017, 7, 3617–3627 RSC.
- R. L. Simpson, P. F. Pagoria, A. R. Mitchell and C. L. Coon, Propellants, Explos., Pyrotech., 1994, 19, 174–179 CrossRef CAS.
- R. Haiges, G. Belanger-Chabot, S. M. Kaplan and K. O. Christe, Dalton Trans., 2015, 44, 2978–2988 RSC.
- P. M. Marcos, J. R. Ascenso, M. A. P. Segurado, R. J. Bernardino and P. J. Cragg, Tetrahedron, 2009, 65, 496–503 CrossRef CAS.
- T. Mukundan, G. N. Purandare and J. K. Nair, Def. Sci. J., 2002, 52, 127–133 CrossRef CAS.
- A. Langlet, N. V. Latypov, U. Wellmar, U. Bemm and P. Goede, J. Org. Chem., 2002, 67, 7833–7838 CrossRef CAS PubMed.
- G. H. Nazeri, R. Mastour and M. Fayaznia, Iran. J. Chem. Chem. Eng., 2008, 27, 85–89 CAS.
- M. Murotani, H. Mura, M. Takeda and H. Shibafuchi, EP 669325, 1994.
- T. M. Klapotke, C. M. Sabate and J. M. Welch, Dalton Trans., 2008, 6372–6380 RSC.
- Y. Liu, X. Zhang, H. Ning and H. Yang, Chin. J. Org. Chem., 2016, 36(5), 1133–1142 CrossRef CAS.
- H. Xue, B. Twamley and J. M. Shreeve, J. Mater. Chem., 2005, 15(34), 3459–3465 RSC.
- J. Yi, F. Zhao, H. Gao, S. Xu, M. Wang and R. Hu, J. Hazard. Mater., 2008, 153(1–2), 261–268 CrossRef CAS PubMed.
- J. Cui, J. Han, J. Wang and R. Huang, J. Chem. Eng. Data, 2010, 55(9), 3229–3234 CrossRef CAS.
- T. M. Klapötke and C. Miró Sabaté, Cent. Eur. J. Energ. Mater., 2010, 7(2), 161–173 Search PubMed.
- D. L. Sheng and E. F. Ma, Energ. Mater., 2004, 12, 93–96 CAS.
- G. Singh and S. P. Felix, J. Hazard. Mater., 2002, 90(1), 1–17 CrossRef CAS PubMed.
- M. Lei, Z.-Z. Zhang, Y.-H. Kong, Z.-R. Liu, C.-H. Zhu, Y.-H. Shao and P. Zhang, Thermochim. Acta, 1999, 335, 105–112 CrossRef CAS.
- P. C. Hillesheim and K. A. Scipione, Acta Crystallogr., Sect. E: Struct. Rep. Online, 2014, 70, o1248–o1249 CAS.
- C. E. Cannizzaro and K. N. Houk, J. Am. Chem. Soc., 2002, 124, 7163–7169 CrossRef CAS PubMed.
- T. Steiner, Cryst. Rev., 1996, 6(1), 1–57 CrossRef CAS.
- G. R. Desiraju, Acc. Chem. Res., 1996, 29, 441–449 CrossRef CAS PubMed.
- H. D. B. Jenkins, D. Tudela and L. Glasser, Inorg. Chem., 2002, 41, 2364–2367 CrossRef CAS PubMed.
- H. Xue, H.-X. Gao, B. Twamley and J. M. Shreeve, Chem. Mater., 2007, 19, 1731–1739 CrossRef CAS.
- L. Qiu, H. M. Xiao, X. D. Gong, X. H. Ju and W. H. Zhu, J. Phys. Chem. A, 2006, 110, 3797–3807 CrossRef CAS PubMed.
- M. J. Kamlet and S. J. Jacobs, J. Chem. Phys., 1968, 48, 23–35 CrossRef CAS.
Footnote |
† Electronic supplementary information (ESI) available: Data of single crystal diffraction, DSC, and TG-DTG, as well as spectra of UV-Vis, FT-IR, ESI-MS, 1H NMR, and 13C NMR. CCDC 1550835–1550837. For ESI and crystallographic data in CIF or other electronic format see DOI: 10.1039/c7ra05601j |
|
This journal is © The Royal Society of Chemistry 2017 |
Click here to see how this site uses Cookies. View our privacy policy here.