DOI:
10.1039/C7RA05379G
(Paper)
RSC Adv., 2017,
7, 35038-35047
Selenoprotein SelK increases the secretion of insulin from MIN6 β cells
Received
12th May 2017
, Accepted 2nd July 2017
First published on 12th July 2017
Abstract
The trace element selenium has an insulin-like effect on humans and animals. In this study, the effect and mechanism of mouse selenoprotein K (mSelK) on the secretion of insulin from mouse MIN6 β cells were investigated. An adenovirus vector, Ad-mSelK, was used to over-express the mSelK gene in the MIN6 β cells. Likewise, a lentivirus vector, LV-mSelK-RNAi, was used to knockdown mSelK expression in the MIN6 β cells. It was shown that the over-expression/knockdown of mSelK could increase/decrease the insulin secretion from MIN6 β cells. Meanwhile, the cytosolic free Ca2+ level and inositol trisphosphate receptor type 3 (IP3R3) expression were also increased/decreased significantly as a consequence of the over-expression/knockdown of mSelK in MIN6 β cells. Over-expression/knockdown of mSelK did not affect the expression of glutathione peroxidase 1 (GPx1) in the MIN6 β cells. Further studies revealed that the mSelK expression and insulin release levels were increased significantly by treatment of MIN6 β cells with selenium supplement (sodium selenite, Na2SeO3). In addition, mSelK protein levels were also up-regulated significantly in MIN6 β cells by adding glucose. These results suggest that mSelK plays a vital role in the process of trace element selenium promoting the secretion of insulin from MIN6 β cells. The expression of mSelK may increase the secretion of insulin by improving the expression of IP3R3 on the endoplasmic reticulum (ER), which elevated the cytosolic free Ca2+ level by enhancing the release of Ca2+ from the ER.
1 Introduction
The trace element selenium (Se) has wide physiological effects: controlling the onset of human Kaschin–Beck disease and Keshan disease, boosting the innate immune system, maintaining thyroid function, preventing the occurrence of cardiovascular disease and Alzheimer's disease, increasing fertility, and preventing cancer etc.1,2 Most functions of selenium are implemented through selenoproteins. There are currently 25 known selenoproteins in the human proteome.3 Sixteen selenoproteins exist in the cytosol and exhibit antioxidative or other physiological effects.4 Nine mammalian selenoproteins are located in the endoplasmic reticulum (ER), including deiodinase 1, 2, and 3 (DIO1, DIO2, and DIO3), the 15 kDa selenoprotein (Sep15), and selenoproteins K, M, S, N, and T.2 They all have different physiological functions in cells.
The insulin-like effect and the antidiabetic effect of Se have attracted increasing attention in recent years, although some researches proved that high level of selenium in humans and animals could increase the risk of type 2 diabetes (T2D).5–11 The influences of selenoproteins, such as glutathione peroxidase 1 (GPx1) and selenoprotein P (SePP), on the occurrence and development of T2D have been studied by many researchers.12–17 Among these studies, the surveys, which were reported that supplement of selenium increased the risk of T2D, were mostly obtained from the United States and other areas of the world in which the soils contain high selenium.18 However, in most parts of the world, soils are deficient in selenium.1,2 Therefore, it is of great significance to moderately supply selenium for the prevention and treatment of T2D in these regions.
Studies have demonstrated that selenium could regulate insulin-mediated metabolism and exert insulin-like effect, and thus decrease the level of blood glucose.19–23 The mechanisms by which selenium reduces the risk of T2D were investigated from the relationship between selenoproteins and glucose metabolism. Selenoprotein M (SelM) was demonstrated to regulate the calcium signaling and prevent the oxidative stress in the islets cells.24 SelM deficiency in mice leads to adult-onset body to gain weight and increase adiposity, suggesting SelM has an important role in obesity.25 It has been shown that, in T2D patients, selenoprotein S (SelS) mRNA expression in subcutaneous adipose tissue is increased after insulin infusion, whereas no effect was seen in nondiabetic subjects.26 In addition, it was demonstrated that, in isolated human adipocytes, SelS expression is also up-regulated after insulin stimulation, which supports a role for SelS in the development of metabolic disease, especially in the context of insulin resistance.27 Selenoprotein T (SelT) was found to be up-regulated by pituitary adenylate cyclase-activating polypeptide (PACAP) in β-pancreatic cells, and it potentiated insulin secretion, which suggested that SelT may take part in glucose homeostasis.28 Conditional knockout of SelT in islet β cells leads to defective insulin secretion, suggesting SelT is vital to the function of β cells. However, the physiological functions of SelK in the secretion of insulin and the prevention of T2D are still unclear.
The increase of Ca2+ level in the cytoplasm can trigger insulin secretion from the β cells.29–33 Insulin release and glucose homeostasis are closely associated with the subtle changes in calcium dynamics.34 Although the changes in calcium homeostasis play an important role in insulin secretion and insulin resistance, little is known about the relationship between the secretion of insulin and selenoproteins which can affect the intracellular Ca2+ level. In our previous studies, it was shown that selenoprotein SelK in Drosophila S2 cells and human gastric cancer BGC 823 cells could increase the expression of inositol trisphosphate receptor (IP3R) on the ER, which increased the level of intracellular free calcium by promoting the release of Ca2+ from ER to cytosol.35,36 And this effect has been verified by others.37,38 Further studies revealed that the stable expression of IP3R needs the palmitoylation of the protein, which requires the catalysis by an enzyme complex composed of DHHC6 (letters represent the amino acids aspartic acid, histidine, and cysteine in the catalytic domain) and selenoprotein SelK. The DHHC6/Selk interactions in the ER membrane depended on Src-homology 3 (SH3)/SH3 binding domain interactions of the two proteins. Therefore, an original DHHC6/SelK enzyme complex is vital for the regulation of stable expression level of IP3R.39
Thus, we consider that SelK may play an important role in the process which trace element selenium promotes islet β cells to secrete insulin. In this paper, the influence of the over-expression/knockdown of mSelK on the insulin secretion from MIN6 β cells was studied. And the mechanism of the above influence was explored by analyzing the variation of cytosolic Ca2+ level and IP3R expression in the cells after the over-expression/knockdown of mSelK. Moreover, the effect of selenium supplement (sodium selenite, Na2SeO3) on the expression of mSelK and the release of insulin was also investigated.
2 Materials and methods
2.1 β cells culture
The mouse MIN6 β cell line was a kind gift from Dr Qiu-Yu Wang (Liaoning University, Liaoning, China). The cells were cultured in Dulbecco's modified Eagle's medium (DMEM, Hyclone, Logan, UT, USA) containing 10% fetal bovine serum (FBS, Gibco, GrandIsland, NY, USA), penicillin (100 U mL−1), streptomycin (100 μg mL−1), 0.5% β-mercaptoethanol, and 10 mM L-glutamine at 37 °C in a fully humidified incubator with 5% CO2.
2.2 Adenovirus vectors and adenovirus infection
An adenovirus vector, Ad-mSelK, was used to over-express mSelK gene in the MIN6 β cells. mSelK over-expression adenovirus vector (Ad-mSelK) and blank adenovirus vector (Ad) were constructed by HanBio Technology (Shanghai, China). MIN6 β cells were seeded at 2 × 104 cells per well in 96-well plates, or 1 × 106 cells per well in 6-well plates for 24 h, and then treated with the above adenovirus vectors for 24 h. The medium was then transferred to fresh medium without adenovirus vectors. After incubated for 24 h, the infected β cells were used for further experiments.
2.3 Lentivirus vectors and lentivirus infection
A lentivirus vector, LV-mSelK-RNAi, was used to knockdown the expression of mSelK gene in the MIN6 β cells. mSelK knockdown lentivirus vector (LV-mSelK-RNAi) and blank lentivirus vector (LV) were constructed by GeneChem Company (Shanghai, China). MIN6 β cells were seeded at 1 × 104 cells per well in 96-well plates, or 5 × 105 cells per well in 6-well plates for 24 h, and then treated with the above lentivirus vectors for 24 h. The medium was then transferred to fresh medium without lentivirus vectors. After incubated for 48 h, the infected β cells were used for further experiments.
2.4 Cell viability assay by MTT
The cell viability was determined using the 3-(4,5-dimethylthiazol-2-yl)-2,5-diphenyltetrazolium bromide (MTT, Gibco) assay. MIN6 β cells treated with Na2SeO3 (0.1–300 μM) for 24 h were used to detect the cell viability. MIN6 β cells were transfected with 2.5–20 multiplicity of infection (MOI) of the above vectors, and the cell viability was then evaluated by MTT assay. Briefly, the medium was removed and the cells were incubated with 0.25 mg mL−1 MTT for 4 h at 37 °C. The formazan crystals were dissolved in dimethyl sulfoxide (DMSO), and the absorbance was measured at 490 nm by a microplate reader (TECAN, Switzerland). Cell viability was expressed as the percentage values taking the non-infection control group (Con) as 100%.
2.5 Western blot analysis
MIN6 β cells transfected with the above vectors (15 MOI) were harvested to detect the expression of mSelK, GPx1, and IP3R3 proteins by western blot assay. MIN6 β cells treated with Na2SeO3 (1–100 μM) or glucose (2.5–25 mM) for 24 h were also used to test the expression of mSelK protein by western blot assay. Briefly, mouse MIN6 β cells were lysed in ice-cold radio immunoprecipitation assay (RIPA) lysis buffer (Beyotime, Shanghai, China) supplemented with protease inhibitors (1 mM phenylmethyl-sulfonylfluoride, 1 μg mL−1 pepstatin, 1 μg mL−1 leupeptin, and 1 μg mL−1 aprotinin) for 1 h at 4 °C. And total proteins in the supernatant fluids were collected. Then proteins were boiled in loading buffer and separated on SDS-PAGE gels, followed by being transferred onto polyvinylidene fluoride membrane (PVDF, Millipore, Bedford, MA). After blocking, blots were incubated with primary antibodies against mouse mSelK (Sigma-Aldrich, St. Louis, MO, USA), GPx1 (Abcam, Cambridge, MA), IP3R3 (Santa Cruz, CA, USA), or mouse β-actin (Abcam), followed by being incubated with anti-rabbit (Sigma) or anti-goat (Santa Cruz) secondary antibodies. The membranes were processed with the enhanced chemiluminescence (ECL) reagents (Tanon, Shanghai, China) and visualized with a Tanon 5200 Multi Chemiluminescent System (Tanon).
2.6 ELISA assay
The enzyme-linked immunosorbent assay (ELISA) kit for mouse insulin was from Lvye Biotechnology (Jiangshu, China). ELISA was performed according to manufacturer's instructions. After the preparation of reagents, the diluted supernatant fluids of infected β cells and non infected standard samples were added to the ELISA plate at 37 °C. After incubated for 30 min, the plate was washed and added standard enzyme reagent for 30 min at 37 °C. The plate was washed again and incubated in colored solution for 30 min. After the addition of the stop solution, the absorbance was tested by the microplate reader.
2.7 Measurement of cytosolic free Ca2+ level by flow cytometry
MIN6 β cells transfected with the above vectors (15 MOI) were harvested to detect the cytosolic Ca2+ level by loading the cells with Fluo-3/AM followed by flow cytometry analysis. The Fluo-3-Ca2+ complex were able to give an increased green fluorescence with the excitation. Specific method was as follows: treated cells were digested using 0.25% trypsin, harvested by centrifugation, and washed thrice with ice-cold phosphate-buffered saline (PBS). Then the cells were re-suspended with 300 μL DMEM medium without serum and antibiotic, subsequently, hatched with 5 μM Fluo-3/AM for 30 min at 37 °C. After that, they were washed twice with PBS, re-suspended with 300 μL of PBS, and immediately analyzed by a FACScan flow cytometer (Becton-Dickinson, San Jose, CA, USA).
2.8 Real-time polymerase chain reaction (PCR) assay
MIN6 β cells transfected with the above vectors (15 MOI) were harvested to detect the mRNA expression of IP3 receptor type 1, 2, or 3 (IP3R1, IP3R2, or IP3R3) by real-time PCR. MIN6 β cells treated with Na2SeO3 (10 μM) for 24 h were also used to detect the mRNA expression of mSelK by real-time PCR assay. Briefly, total RNA was extracted from MIN6 β cells in TRIzol reagent (Invitrogen, Carlsbad, CA, USA) according to manufacturer's instructions. Total RNA (1 μg) was reverse transcribed into complementary DNA (cDNA) in reaction volumes of 20 μL using a PrimeScript™ RT reagent Kit with gDNA Eraser (TaKaRa, Japan). The cDNA was then analyzed by real-time PCR. Real-time PCR amplification was performed on a 7500 Fast Real-Time PCR System (Applied Biosystems). Expression of genes was quantified by real-time PCR using a SYBR Premix Ex TaqTMII (TaKaRa) and 0.1 μg of cDNA in each reaction. The following primers were used for amplification: mSelK forward, 5′-AAA AGA AAG AGG CTA CGG G-3′; mSelK reverse, 5′-TTA CCT TCC TCA TCC ACC-3′; actin forward, 5′-TTC CTT CTT GGG TAT GGA AT-3′; actin reverse, 5′-GAG CAA TGA TCT TGA TCT TC-3′; IP3R1 forward, 5′-AAG CGG ATG GAC CTG GTG TTA GAA CTG-3′; IP3R1 reverse, 5′-AAT TTG TGC TGT GTG CTT CGC GTA GAA CT-3′; IP3R2 forward, 5′-CTG TTC TTC TTC ATC GTC ATC ATC ATC G-3′; IP3R2 reverse, 5′-GAA ACC AGT CCA AAT TCT TCT CCG TGA-3′; IP3R3 forward, 5′-CTT CTT TAT CGT CAT CAT CAT CGT GTT G-3′; IP3R3 reverse, 5′-AGG TTC TTG TTC TTG ATC ATC TGA GCC A-3′. For data analysis, the raw threshold cycle value of each sample was first normalized to that of actin. Thus, we calculated ΔCt for each sample and then the normalized ΔCt. Ratio = 2−ΔΔCt
2.9 Statistical analysis
SPSS 19.0 software was used to compare means by the Student's t-test between two groups or by one-way ANOVA among three or more groups. Data were represented as the means ± SEM of three experiments.
3 Results and discussion
3.1 Effect of mSelK on the insulin secretion from MIN6 β cells
Selenium has insulin-like effect.5–8 Most functions of selenium are implemented through selenoproteins. The influences of selenoproteins on the occurrence and development of T2D have been studied previously.22–28 However, the influence of selenoprotein SelK on T2D risk or insulin secretion has not been elucidated. In this study, the effect of SelK on insulin secretion in β cells line was reported for the first time.
In order to eliminate the influence of the transfection of different vectors on the cell viability, the effect of various vectors on the viability of MIN6 β cells was first detected by MTT method (Fig. 1A and B). After being transfected with above adenovirus vectors for 48 h or being transfected with above lentivirus vectors for 72 h in the concentration of 2.5–20 MOI, the viability of MIN6 β cells had no significant change compared to the blank control group (Con). This suggested that the over-expression or knockdown of mSelK did not affect the viability of the β cells.
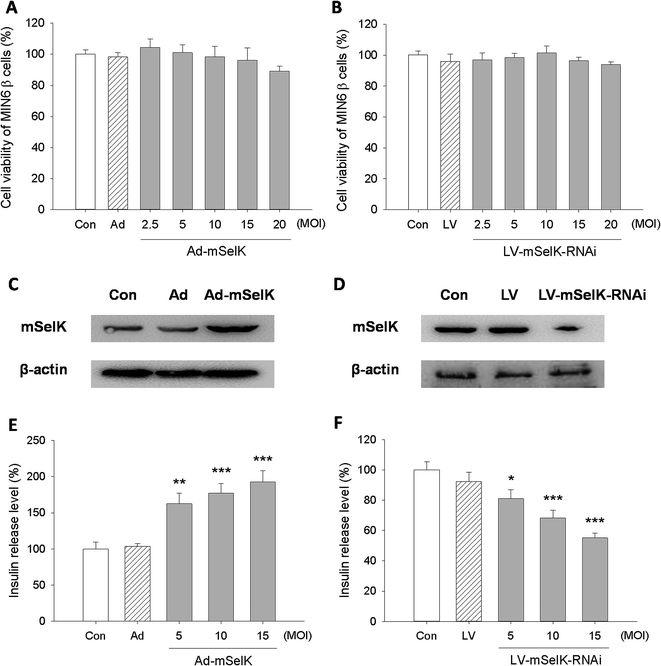 |
| Fig. 1 Effect of mSelK on the insulin secretion from MIN6 β cells. (A and B) Viability of β cells transfected with the blank adenovirus vector (Ad), mSelK over-expression adenovirus vector (Ad-mSelK), blank lentivirus vector (LV), mSelK knockdown lentivirus vector (LV-mSelK-RNAi), or non-transfected cells (Con) was tested by MTT assay. (C and D) The expression of mSelK and β-actin protein in MIN6 β cells transfected with Ad, Ad-mSelK, LV, LV-mSelK-RNAi, or non-transfected cells (Con) was analyzed by western blot. (E and F) Insulin release level of MIN6 β cells transfected with with Ad, Ad-mSelK, LV, LV-mSelK-RNAi, or non-transfected cells (Con) was analyzed by ELISA. *P < 0.05, **P < 0.01, ***P < 0.001 as compared with the Con group. | |
The influence of the transfection of Ad vector (blank adenovirus vector), Ad-mSelK vector (mSelK over-expression adenovirus vector), LV vector (blank lentivirus vector), and LV-mSelK-RNAi vector (mSelK knockdown lentivirus vector) on the expression of mSelK protein in the β cells was detected by western blot assay (Fig. 1C and D). The results indicated that the expression of mSelK protein was increased in the β cells transfected with Ad-mSelK vector (Fig. 1C), while the expression of mSelK was decreased by the transfection of LV-mSelK-RNAi vector compared to the blank control group (Fig. 1D). Moreover, the blank adenovirus vector (Ad) and blank lentivirus vector (LV) did not show significant influence on the mSelK protein (Fig. 1C and D). These results confirmed the efficiency of over-expression/knockdown of mSelK gene by transfecting cells with Ad-mSelK/LV-mSelK-RNAi vectors.
The influence of the over-expression/knockdown of mSelK on the release of insulin from the MIN6 β cells was then tested by ELISA experiment. And it was shown that the level of insulin released from MIN6 β cells transfected with Ad-mSelK vector was significantly elevated compared to the control group (Con) in a MOI-dependent manner (Fig. 1E). In contrast, insulin level released from the β cells transfected with LV-mSelK-RNAi was declined significantly, and also in a MOI-dependent manner (Fig. 1F). The Ad and LV groups did not show significant variation (Fig. 1E and F). These data strongly suggested that the release of insulin is closely related to the expression of mSelK in MIN6 β cells.
Since the increased insulin level in islet β cells was associated with the elevated level of selenoprotein GPx1, an antioxidant enzyme existed in cytosol,12,40–42 the influence of the over-expression/knockdown of mSelK on the expression of GPx1 in the MIN6 β cells was investigated. The result showed that the expression of GPx1 was not altered in the transfected cells, indicating that over-expression/knockdown of mSelK did not affect the GPx1 in the MIN6 β cells (data not shown).
3.2 Effect of mSelK on the intracellular free Ca2+ level in MIN6 β cells
Glucose and other nutrients promote islet β cells to release insulin by increasing intracellular ATP/ADP ratio, resulting in the closure of the KATP channel, cell membrane depolarization, and calcium influx. The increase of Ca2+ level in the cytoplasm can trigger insulin secretion.29–33 In our previous studies, it is shown that selenoprotein SelK in Drosophila S2 cells and human gastric cancer BGC 823 cells can increase the level of cytosolic free Ca2+ by promoting the release of Ca2+ from the ER to cytosol.35,36 In order to illustrate the inherent mechanism of the increased insulin secretion, the influence of the over-expression and knockdown of mSelK on the cytosolic free Ca2+ level in MIN6 β cells was also investigated. It was shown by flow cytometry that cytosolic free Ca2+ level was elevated by the over-expression of mSelK and declined by the knockdown of mSelK in MIN6 β cells compared to the control group (Con) (Fig. 2A and B), just like the results in Drosophila S2 cell line and human gastric cancer BGC 823 cell line by our group35,36 and in T cells, neutrophils, and macrophages by Verma et al.37 And it was shown that after the over-expression/knockdown of mSelK, the intracellular free Ca2+ level was increased/decreased to 149%/70% compared to the blank control group (taking blank control group as 100%), which suggested that the variation of free Ca2+ level in MIN6 β cells by the over-expression/knockdown of mSelK was similar to that of the release of insulin.
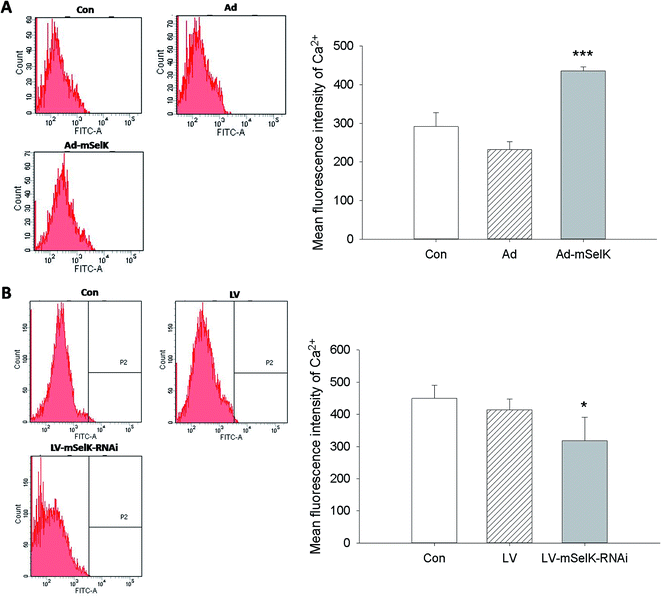 |
| Fig. 2 Effect of mSelK on the intracellular free Ca2+ level in MIN6 β cells. (A) The cytosolic free Ca2+ level in the β cells transfected with Ad, Ad-mSelK, or non-transfected cells (Con). (B) The cytosolic free Ca2+ level in the β cells transfected with LV, LV-mSelK-RNAi, or non-transfected cells (Con). The intracellular free Ca2+ levels were detected by flow cytometry after the cells were loaded with Fluo-3/AM. *P < 0.05, ***P < 0.001 as compared with the Con group. | |
3.3 Effect of mSelK on the IP3R expression level in MIN6 β cells
The basic molecular function of SelK is increasing the stable expression of IP3R by catalyzing the palmitoylation of IP3R.38 The increased expression of IP3R by the SelK up-regulation stimulates the release of Ca2+ from the ER calcium store to the cytosol of the cells.35,36 IP3R is a ligand-gated ion channel protein presented on ER and nuclear envelope, which was originally identified in mouse pancreas cells. IP3R is classified in five types, among which IP3R types 1, 2, and 3 (IP3R1, IP3R2, and IP3R3) are expressed in islet β cells and IP3R3 is the most abundant in this gland.33
In this study, the effect of mSelK on the mRNA transcription of IP3R1, IP3R2, or IP3R3 in MIN6 β cells was investigated by real-time PCR. As shown in Fig. 3A–C, in mSelK knockdown cells, the mRNA expression of IP3R1 and IP3R2 had no significant change, while the mRNA level of IP3R3 decreased significantly. On the other hand, as shown in Fig. 3D–F, the mRNA expression of IP3R1 and IP3R2 in mSelK over-expressed cells also had no significant change, while the IP3R3 mRNA level was increased significantly. Since only the transcript level of IP3R3 was significantly influenced by the over-expression/knockdown of mSelK, the expression level of IP3R3 protein was detected by western blot, and it was shown that the expression of IP3R3 protein was also significantly increased in mSelK over-expressed MIN6 β cells (Fig. 3G). SelK has been demonstrated to increase the expression of IP3R in macrophage, BGC823, and other mammalian cells.35,36,43 This is the first report for the potent enhancement effect of mSelK on the expression of IP3R3 in the β cells. These data strongly suggest that the expression of mSelK up-regulates IP3R3 mRNA transcription followed by increasing IP3R3 protein production in MIN6 β cells. Thus, our results presented here indicated that mSelK may acts to enhance the insulin secretion from MIN6 β cells by increasing the cytosolic free Ca2+ level resulted from the up-regulated IP3R3 expression. The stable expression of IP3R needs the palmitoylation of the protein, which requires the catalysis of an enzyme complex composed of DHHC6 and SelK on the ER membrane.39 Therefore, an original DHHC6/SelK enzyme complex is vital for the regulation of stable expression level of the IP3R. Further studies are required in order to understand whether mSelK acts to regulate the IP3R3 expression by the palmitoylation of the protein in MIN6 β cells.
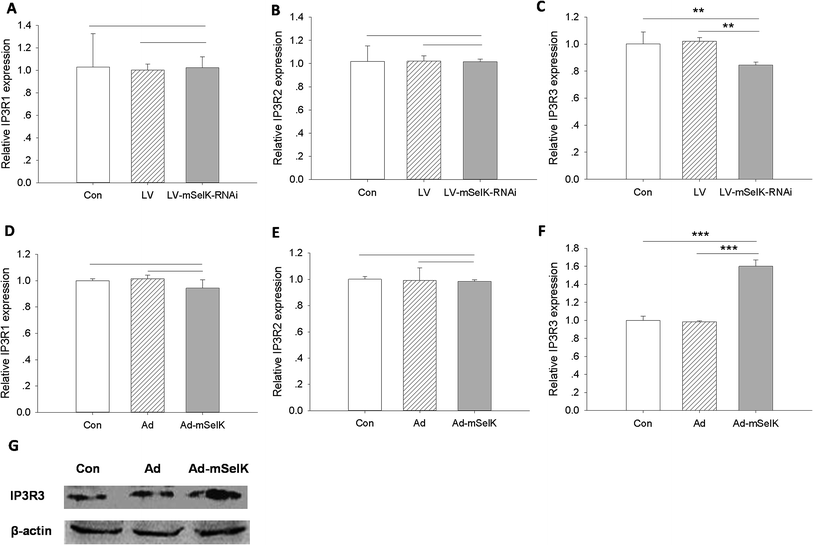 |
| Fig. 3 Effect of mSelK on the IP3R expression level in MIN6 β cells. Transcript levels of IP3R1 (A), IP3R2 (B), and IP3R3 (C) in MIN6 β cells transfected with LV, LV-mSelK-RNAi, or non-transfected cells (Con) were assessed by real-time PCR. Transcript levels of IP3R1 (D), IP3R2 (E), and IP3R3 (F) in MIN6 β cells transfected with Ad, Ad-mSelK, or non-transfected cells (Con) was assessed by real-time PCR. **P < 0.01, ***P < 0.001. (G) The expression of IP3R3 and β-actin protein in MIN6 β cells transfected with Ad, Ad-mSelK, or non-transfected cells (Con) was analyzed by western blot. | |
3.4 Effect of trace element selenium on the expression of mSelK and the release of insulin
Studies have demonstrated that trace element selenium has insulin-like effect, and moderate selenium supplements can reduce the T2D risk.5,19,20 Although the increased expression of SelK by selenium supplements has been studied in hepatocarcinoma cell,44 lung cancer cells, prostate cancer cells, colon cancer cells,45 and muscle cells,46 the influence of selenium supplements on the expression of SelK in islet β cells has not been studied. In this study, after treatment of mouse MIN6 β cells with different concentrations of Na2SeO3 in vitro for 24 h, the expression of mSelK was assessed by real-time PCR and western blot, and the release level of insulin was measured by ELISA.
According to the result of cytotoxicity test, treatment of MIN6 β cells with Na2SeO3 (0.1–10 μM) did not affect the cell viability. The toxicity was found when the cells were treated with Na2SeO3 at the concentration of 30–300 μM (Fig. 4A).
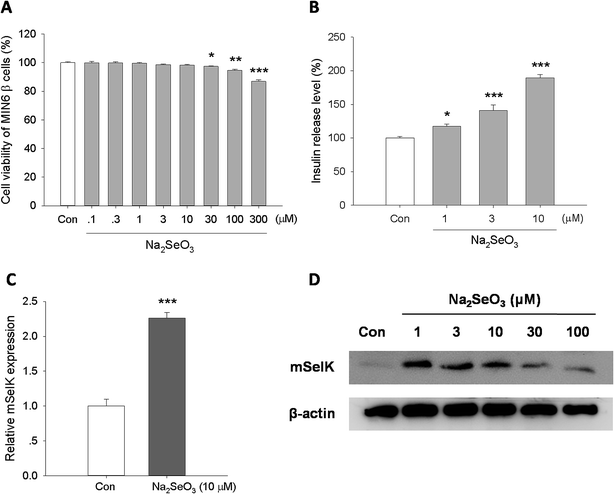 |
| Fig. 4 Effect of trace element selenium on the expression of mSelK and the release of insulin. (A) Viability of MIN6 β cells treated with Na2SeO3 (0.1–300 μM) for 24 h was tested by MTT assay. (B) Insulin release level of MIN6 β cells treated with Na2SeO3 (1–10 μM) for 24 h was analyzed by ELISA. (C) Transcript level of mSelK in MIN6 β cells treated with Na2SeO3 (10 μM) for 24 h was assessed by real-time PCR. (D) The expression of mSelK and β-actin protein in MIN6 β cells treated with Na2SeO3 (1–100 μM) for 24 h was assayed by western blot. *P < 0.05, **P < 0.01, ***P < 0.001 as compared with the Con group. | |
The result of real-time PCR analysis showed that the mSelK mRNA expression is increased in the presence of Na2SeO3 (10 μM). The relative mSelK mRNA expression efficiency of Na2SeO3 treated group was 226 ± 8% (Fig. 4C). Further studies by western blot showed that the levels of mSelK protein in MIN6 β cells was markedly up-regulated after Na2SeO3 (1–100 μM) treatment for 24 h. The effects of Na2SeO3 on the expression of SelK in human hepatoma cell lines (HepG2, Huh7 and HCC) had been assessed previously in vitro, and the results showed that the increase observed for the SelK expression is correlated with the increase of Na2SeO3.47,48 The results obtained here in MIN6 β cells are consistent with those reports.47,48 Moreover, when the cells were treated with excess amounts of Na2SeO3 (30 and 100 μM), the mSelK expression levels were decreased obviously compared to the Na2SeO3 (1–10 μM) groups (Fig. 4D). One of the reasons for this phenomenon could be that excessive Na2SeO3 (30 and 100 μM) might induce oxidative stress and lesions of ER membrane, resulting in declined mSelK expression on the ER of cells.
As shown in Fig. 4B, Na2SeO3 (1, 3, and 10 μM) could significantly increase the insulin release level in a concentration-dependent manner, which was consistent with the previous report.49
These data indicated that Na2SeO3 could increase the expression of mSelK and the release of insulin in MIN6 β cells. Our results revealed that mSelK may be an important selenoprotein for trace element selenium exerting their potential roles in the prevention and treatment of T2D by triggering the insulin secretion from islet β cells.
3.5 Effect of glucose on the expression of mSelK in MIN6 β cells
It is well known that the insulin secretion from islet β cells can be increased by glucose supply.50,51 Glucose administration revealed that SelT-knockout mice display impaired glucose tolerance, which showed that SelT is involved in the control of glucose homeostasis.28 SePP levels were decreased in isolated murine islets cultured in high-glucose medium, which might contribute to glucotoxicity in islets β cells.52 Moreover, serum SelS levels have been demonstrated to be negatively correlated with fasting plasma glucose.53 However, the effect of glucose on the expression of SelK is still unclear.
In order to test the effect of glucose on mSelK expression in the β cells, mouse MIN6 β cells were treated with low glucose (2.8 mM), normal glucose (5.6 mM, the glucose concentration in the DMEM medium applied for MIN6 cell culture), or high glucose (12.8 and 25 mM) for 24 h, and the expression of mSelK protein was assessed by western blot. The result showed that mSelK expression is obviously increased by glucose supply (Fig. 5). This suggested that, in MIN6 β cells, mSelK expression and insulin release might have a close association. mSelK may act as a regulator in the process of glucose regulating insulin secretion from MIN6 β cells. Further studies are required to clarify the role of mSelK in the prevention and/or treatment of diabetes.
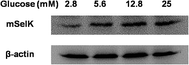 |
| Fig. 5 Effect of glucose on the expression of mSelK in MIN6 β cells. Expression of mSelK and β-actin protein in MIN6 β cells treated with glucose (2.5–25 mM) for 24 h was assayed by western blot. | |
4 Conclusion
In summary, our data indicate a novel role of SelK in insulin secretion of MIN6 β cells. It is demonstrated that mSelK acts to enhance the secretion of insulin from MIN6 β cells by increasing the cytosolic free Ca2+ level resulted from the up-regulated IP3R3 expression, and mSelK plays a vital role in the process of trace element selenium promoting the insulin secretion. These results suggest that mSelK may be an important selenoprotein for selenium exerting their functions in the prevention and/or treatment of T2D by enhancing the insulin secretion from islet β cells.
Acknowledgements
The authors are grateful to Dr Qiu-Yu Wang (Liaoning University, Liaoning, China) for providing mouse MIN6 β cells and Dr Hao Pan (Liaoning University, Liaoning, China) for the review and editing of the manuscript. This study was supported by the National Natural Science Foundation of China (No. 31371085 and No. 81503085), the Foundation of the Education Department of Liaoning Province of China (No. L2013010 and No. LT2015011), and the Foundation of the Department of Science and Technology of Liaoning Province of China (No. 2014020171).
References
- C. Chen, F. Huan, X. Meng and J. Lv, The effect of selenium on human health and diseases and the scientifically supplementation of selenium to human bodies, J. Liaoning Univ., Nat. Sci. Ed., 2016, 45, 155–168 Search PubMed.
- M. P. Rayman, Selenium and human health, Lancet, 2012, 379, 1256–1268 CrossRef CAS.
- G. V. Kryukov, S. Castellano, S. V. Novoselov, A. V. Lobanov, O. Zehtab, R. Guigó and V. N. Gladyshev, Characterization of mammalian selenoproteomes, Science, 2003, 300, 1439–1443 CrossRef CAS PubMed.
- R. Pillai, J. H. Uyehara-Lock and F. P. Bellinger, Selenium and selenoprotein function in brain disorders, IUBMB Life, 2014, 66, 229–239 CrossRef CAS PubMed.
- O. Bozkurt, S. Haman Bayari, M. Severcan, C. Krafft, J. Popp and F. Severcan, Structural alterations in rat liver proteins due to streptozotocin-induced diabetes and the recovery effect of selenium: Fourier transform infrared microspectroscopy and neural network study, J. Biomed. Opt., 2012, 17, 076023 CrossRef PubMed.
- X. Q. Sheng, K. X. Huang and H. B. Xu, New experimental observation on the relationship of selenium and diabetes mellitus, Biol. Trace Elem. Res., 2004, 99, 241–253 CrossRef CAS PubMed.
- S. Roy, S. K. Dontamalla, A. K. Mondru, S. Sannigrahi and P. R. Veerareddy, Downregulation of apoptosis and modulation of TGF-β1 by sodium selenate prevents streptozotocin-induced diabetic rat renal impairment, Biol. Trace Elem. Res., 2011, 139, 55–71 CrossRef CAS PubMed.
- J. Zhou, K. Huang and X. G. Lei, Selenium and diabetes–evidence from animal studies, Free Radical Biol. Med., 2013, 65, 1548–1556 CrossRef CAS PubMed.
- P. Faure, O. Ramon, A. Favier and S. Halimi, Selenium supplementation decreases nuclear factor-kappa B activity in peripheral blood mononuclear cells from type 2 diabetic patients, Eur. J. Clin. Invest., 2004, 34, 475–481 CrossRef CAS PubMed.
- S. S. Pillai, J. K. Sugathan and M. Indira, Selenium downregulates RAGE and NFκB expression in diabetic rats, Biol. Trace Elem. Res., 2012, 149, 71–77 CrossRef CAS PubMed.
- G. M. Barakat, M. E. Moustafa and A. B. Bikhazi, Effects of selenium and exendin-4 on glucagon-like peptide-1 receptor, IRS-1, and Raf-1 in the liver of diabetic rats, Biochem. Genet., 2012, 50, 922–935 CrossRef CAS PubMed.
- J. P. McClung, C. A. Roneker, W. Mu, D. J. Lisk, P. Langlais, F. Liu and X. G. Lei, Development of insulin resistance and obesity in mice overexpressing cellular glutathione peroxidase, Proc. Natl. Acad. Sci. U. S. A., 2004, 101, 8852–8857 CrossRef CAS PubMed.
- X. Chen, T. O. Scholl, M. J. Leskiw, M. R. Donaldson and T. P. Stein, Association of glutathione peroxidase activity with insulin resistance and dietary fat intake during normal pregnancy, J. Clin. Endocrinol. Metab., 2003, 88, 5963–5968 CrossRef CAS PubMed.
- H. Steinbrenner, B. Speckmann, A. Pinto and H. Sies, High selenium intake and increased diabetes risk: experimental evidence for interplay between selenium and carbohydrate metabolism, J. Clin. Biochem. Nutr., 2011, 48, 40–45 CrossRef CAS PubMed.
- L. Schomburg and J. Köhrle, On the importance of selenium and iodine metabolism for thyroid hormone biosynthesis and human health, Mol. Nutr. Food Res., 2008, 52, 1235–1246 CAS.
- H. Misu, T. Takamura, H. Takayama, H. Hayashi, N. Matsuzawa-Nagata, S. Kurita, K. Ishikura, H. Ando, Y. Takeshita, T. Ota, M. Sakurai, T. Yamashita, E. Mizukoshi, T. Yamashita, M. Honda, K. Miyamoto, T. Kubota, N. Kubota, T. Kadowaki, H. J. Kim, I. K. Lee, Y. Minokoshi, Y. Saito, K. Takahashi, Y. Yamada, N. Takakura and S. Kaneko, A liver-derived secretory protein, selenoprotein P, causes insulin resistance, Cell Metab., 2010, 12, 483–495 CrossRef CAS PubMed.
- S. J. Yang, S. Y. Hwang, H. Y. Choi, H. J. Yoo, J. A. Seo, S. G. Kim, N. H. Kim, S. H. Baik, D. S. Choi and K. M. Choi, Serum selenoprotein P levels in patients with type 2 diabetes and prediabetes: implications for insulin resistance, inflammation, and atherosclerosis, J. Clin. Endocrinol. Metab., 2011, 96, E1325–E1329 CrossRef CAS PubMed.
- S. Stranges, J. R. Marshall, R. Natarajan, R. P. Donahue, M. Trevisan, G. F. Combs, F. P. Cappuccio, A. Ceriello and M. E. Reid, Effects of long-term selenium supplementation on the incidence of type 2 diabetes: a randomized trial, Ann. Intern. Med., 2007, 147, 217–223 CrossRef PubMed.
- D. Hwang, S. Seo, Y. Kim, C. Kim, S. Shim, S. Jee, S. Lee, M. Jang, M. Kim, S. Yim, S. K. Lee, B. Kang, I. Jang and J. Cho, Selenium acts as an insulin-like molecule for the down-regulation of diabetic symptoms via endoplasmic reticulum stress and insulin signalling proteins in diabetes-induced non-obese diabetic mice, J. Biosci., 2007, 32, 723–735 CrossRef CAS PubMed.
- Y. J. Hei, S. Farahbakhshian, X. Chen, M. L. Battell and J. H. McNeill, Stimulation of MAP kinase and S6 kinase by vanadium and selenium in rat adipocytes, Mol. Cell. Biochem., 1998, 178, 367–375 CrossRef CAS PubMed.
- A. Pinto, B. Speckmann, M. Heisler, H. Sies and H. Steinbrenner, Delaying of insulin signal transduction in skeletal muscle cells by selenium compounds, J. Inorg. Biochem., 2011, 105, 812–820 CrossRef CAS PubMed.
- A. Pinto, D. T. Juniper, M. Sanil, L. Morgan, L. Clark, H. Sies, M. P. Rayman and H. Steinbrenner, Supranutritional selenium induces alterations in molecular targets related to energy metabolism in skeletal muscle and visceral adipose tissue of pigs, J. Inorg. Biochem., 2012, 114, 47–54 CrossRef CAS PubMed.
- S. S. Kim, J. H. Koo, I. S. Kwon, Y. S. Oh, S. J. Lee, E. J. Kim, W. K. Kim, J. Lee and J. Y. Cho, Exercise training and selenium or a combined treatment ameliorates aberrant expression of glucose and lactate metabolic proteins in skeletal muscle in a rodent model of diabetes, Nutr. Res. Pract., 2011, 5, 205–213 CrossRef CAS PubMed.
- L. Ozcan, A. S. Ergin, A. Lu, J. Chung, S. Sarkar, D. Nie, M. G. Myers Jr and U. Ozcan, Endoplasmic reticulum stress plays a central role in development of leptin resistance, Cell Metab., 2009, 9, 35–51 CrossRef CAS PubMed.
- M. A. Reeves, F. P. Bellinger and M. J. Berry, The neuroprotective functions of selenoprotein M and its role in cytosolic calcium regulation, Antioxid. Redox Signaling, 2010, 12, 809–818 CrossRef CAS PubMed.
- H. K. Karlsson, H. Tsuchida, S. Lake, H. A. Koistinen and A. Krook, Relationship between serum amyloid A level and Tanis/SelS mRNA expression in skeletal muscle and adipose tissue from healthy and type 2 diabetic subjects, Diabetes, 2004, 53, 1424–1428 CrossRef PubMed.
- M. Olsson, B. Olsson, P. Jacobson, D. S. Thelle, J. Björkegren, A. Walley, P. Froguel, L. M. Carlsson and K. Sjöholm, Expression of the selenoprotein S (SELS) gene in subcutaneous adipose tissue and SELS genotype are associated with metabolic risk factors, Metabolism, 2011, 60, 114–120 CrossRef CAS PubMed.
- G. Prevost, A. Arabo, L. Jian, E. Quelennec, D. Cartier, S. Hassan, A. Falluel-Morel, Y. Tanguy, S. Gargani, I. Lihrmann, J. Kerr-Conte, H. Lefebvre, F. Pattou and Y. Anouar, The PACAP-regulated gene selenoprotein T is abundantly expressed in mouse and human β-cells and its targeted inactivation impairs glucose tolerance, Endocrinology, 2013, 154, 3796–3806 CrossRef CAS PubMed.
- P. Thams, M. R. Anwar and K. Capito, Glucose triggers protein kinase A-dependent insulin secretion in mouse pancreatic islets through activation of the KATP+ channel-dependent pathway, Eur. J. Endocrinol., 2005, 152, 671–677 CrossRef CAS PubMed.
- H. Hatakeyama, T. Kishimoto, T. Nemoto, H. Kasai and N. Takahashi, Rapid glucose sensing by protein kinase A for insulin exocytosis in mouse pancreatic islets, J. Physiol., 2006, 570, 271–282 CrossRef CAS PubMed.
- S. G. Straub and G. W. Sharp, Glucose-stimulated signaling pathways in biphasic insulin secretion, Diabetes/Metab. Res. Rev., 2002, 18, 451–463 CrossRef CAS PubMed.
- X. Zhou, W. Yang and J. Li, Ca2+- and protein kinase C-dependent signaling pathway for nuclear factor-kappa B activation, inducible nitric-oxide synthase expression, and tumor necrosis factor-alpha production in lipopolysaccharide-stimulated rat peritoneal macrophages, J. Biol. Chem., 2006, 281, 31337–31347 CrossRef CAS PubMed.
- O. Blondel, J. Takeda, H. Janssen, S. Seino and G. I. Bell, Sequence and functional characterization of a third inositol trisphosphate receptor subtype, IP3R-3, expressed in pancreatic islets, kidney, gastrointestinal tract, and other tissues, J. Biol. Chem., 1993, 268, 11356–11363 CAS.
- L. Li, A. Trifunovic, M. Köhler, Y. Wang, J. Petrovic Berglund, C. Illies, L. Juntti-Berggren, N. G. Larsson and P. O. Berggren, Defects in β-cell Ca2+ dynamics in age-induced diabetes, Diabetes, 2014, 63, 4100–4114 CrossRef CAS PubMed.
- S. B. Ben, Q. Y. Wang, L. Xia, J. Z. Xia, J. Cui, J. Wang, F. Yang, H. Bai, M. S. Shim, B. J. Lee, L. G. Sun and C. L. Chen, Selenoprotein dSelK in Drosophila elevates release of Ca2+ from endoplasmic reticulum by upregulating expression of inositol 1,4,5-tris-phosphate receptor, Biochemistry, 2011, 76, 1030–1036 CAS.
- S. B. Ben, B. Peng, G. C. Wang, C. Li, H. F. Gu, H. Jiang, X. L. Meng, B. J. Lee and C. L. Chen, Overexpression of selenoprotein SelK in BGC-823 cells inhibits cell adhesion and migration, Biochemistry, 2015, 80, 1344–1353 CAS.
- S. Verma, F. W. Hoffmann, M. Kumar, Z. Huang, K. Roe, E. Nguyen-Wu, A. S. Hashimoto and P. R. Hoffmann, Selenoprotein K knockout mice exhibit deficient calcium flux in immune cells and impaired immune responses, J. Immunol., 2011, 186, 2127–2137 CrossRef CAS PubMed.
- Z. Huang, F. W. Hoffmann, R. L. Norton, A. C. Hashimoto and P. R. Hoffmann, Selenoprotein K is a novel target of m-calpain, and cleavage is regulated by Toll-like receptor-induced calpastatin in macrophages, J. Biol. Chem., 2011, 286, 34830–34838 CrossRef CAS PubMed.
- G. J. Fredericks, F. W. Hoffmann, A. H. Rose, H. J. Osterheld, F. M. Hess, F. Mercier and P. R. Hoffmann, Stable expression and function of the inositol 1,4,5-triphosphate receptor requires palmitoylation by a DHHC6/selenoprotein K complex, Proc. Natl. Acad. Sci. U. S. A., 2014, 111, 16478–16483 CrossRef CAS PubMed.
- X. D. Wang, M. Z. Vatamaniuk, S. K. Wang, C. A. Roneker, R. A. Simmons and X. G. Lei, Molecular mechanisms for hyperinsulinaemia induced by overproduction of selenium-dependent glutathione peroxidase-1 in mice, Diabetologia, 2008, 51, 1515–1524 CrossRef CAS PubMed.
- X. Yang, L. Feng, C. Li and Y. Li, Tranilast alleviates endothelial dysfunctions and insulin resistance via preserving glutathione peroxidase 1 in rats fed a high-fat emulsion, J. Pharmacol. Sci., 2014, 124, 18–30 CrossRef CAS.
- T. L. Merry, M. Tran, M. Stathopoulos, F. Wiede, B. C. Fam, G. T. Dodd, I. Clarke, M. J. Watt, S. Andrikopoulos and T. Tiganis, High-fat-fed obese glutathione peroxidase 1-deficient mice exhibit defective insulin secretion but protection from hepatic steatosis and liver damage, Antioxid. Redox Signaling, 2014, 20, 2114–2129 CrossRef CAS PubMed.
- V. A. Shchedrina, R. A. Everley, Y. Zhang, S. P. Gygi, D. L. Hatfield and V. N. Gladyshev, Selenoprotein K binds multiprotein complexes and is involved in the regulation of endoplasmic reticulum homeostasis, J. Biol. Chem., 2011, 286, 42937–42948 CrossRef CAS PubMed.
- F. Rusolo, B. Pucci, G. Colonna, F. Capone, E. Guerriero, M. R. Milone, M. Nazzaro, M. G. Volpe, G. Di Bernardo, G. Castello and S. Costantini, Evaluation of selenite effects on selenoproteins and cytokinome in human hepatoma cell lines, Molecules, 2013, 18, 2549–2562 CrossRef CAS PubMed.
- L. H. Sun, J. G. Li, H. Zhao, J. Shi, J. Q. Huang, K. N. Wang, X. J. Xia, L. Li and X. G. Lei, Porcine serum can be biofortified with selenium to inhibit proliferation of three types of human cancer cells, J. Nutr., 2013, 143, 1115–1122 CrossRef CAS PubMed.
- H. Yao, W. Zhao, X. Zhao, R. Fan, P. A. Khoso, Z. Zhang, W. Liu and S. Xu, Selenium deficiency mainly influences the gene expressions of antioxidative selenoproteins in chicken muscles, Biol. Trace Elem. Res., 2014, 161, 318–327 CrossRef CAS PubMed.
- F. Rusolo, B. Pucci, G. Colonna, F. Capone, E. Guerriero, M. R. Milone, M. Nazzaro, M. G. Volpe, G. Di Bernardo, G. Castello and S. Costantini, Evaluation of selenite effects on selenoproteins and cytokinome in human hepatoma cell lines, Molecules, 2013, 18, 2549–2562 CrossRef CAS PubMed.
- N. Potenza, F. Castiello, M. Panella, G. Colonna, G. Ciliberto, A. Russo and S. Costantini, Human MiR-544a Modulates SELK Expression in Hepatocarcinoma Cell Lines, PLoS One, 2016, 11, e0156908 Search PubMed.
- S. C. Campbell, A. Aldibbiat, C. E. Marriott, C. Landy, T. Ali, W. F. Ferris, C. S. Butler, J. A. Shaw and W. M. Macfarlane, Selenium stimulates pancreatic beta-cell gene expression and enhances islet function, FEBS Lett., 2008, 582, 2333–2337 CrossRef CAS PubMed.
- J. C. Henquin, Triggering and amplifying pathways of regulation of insulin secretion by glucose, Diabetes, 2000, 49, 1751–1760 CrossRef CAS PubMed.
- F. M. Ashcroft and P. Rorsman, Electrophysiology of the pancreatic beta-cell, Prog. Biophys. Mol. Biol., 1989, 54, 87–143 CrossRef CAS PubMed.
- H. Steinbrenner, A. L. Hotze, B. Speckmann, A. Pinto, H. Sies, M. Schott, M. Ehlers, W. A. Scherbaum and S. Schinner, Localization and regulation of pancreatic selenoprotein P, J. Mol. Endocrinol., 2012, 50, 31–42 CrossRef PubMed.
- S. S. Yu, L. L. Men, J. L. Wu, L. W. Huang, Q. Xing, J. J. Yao, Y. B. Wang, G. R. Song, H. S. Guo, G. H. Sun, Y. H. Zhang, H. Li and J. L. Du, The source of circulating selenoprotein S and its association with type 2 diabetes mellitus and atherosclerosis: a preliminary study, Cardiovasc. Diabetol., 2016, 15, 70 CrossRef PubMed.
|
This journal is © The Royal Society of Chemistry 2017 |