DOI:
10.1039/C7RA05071B
(Paper)
RSC Adv., 2017,
7, 33378-33384
Tumor homing and penetrating peptide-conjugated niosomes as multi-drug carriers for tumor-targeted drug delivery†
Received
5th May 2017
, Accepted 21st June 2017
First published on 3rd July 2017
Abstract
The development of nanoscale drug delivery systems, which can mediate efficient tumor targeting together with high cellular internalization, is crucial for glioma treatment. The combination of therapeutic agents in nanoparticles provides synergistic effects and allows further surface modifications with targeting ligands for specific glioma therapy. To achieve this goal, both doxorubicin and curcumin were encapsulated in polyethylene glycolated niosomes (PEGNIO). The surface of co-drug loaded PEGNIO was modified with tumor homing and penetrating peptide (tLyp-1). Physicochemical properties were determined via dynamic light scattering (DLS) and spectral analysis. Moreover, flow cytometry studies were performed to examine the specific cellular uptake of the tLyp-1 targeted niosomal formulation. In vitro cytotoxicity and inhibition of tumor-like spheroids growth were investigated on human glioblastoma (U87) and human mesenchymal stem cells (hMSC) cells. The results clearly indicated that the strategy by co-administration of doxorubicin and curcumin with tLyp-1 functionalized niosomes could significantly improve anti-glioma treatment.
Introduction
Glioblastoma is a malignant brain tumor and patients diagnosed with glioma have a median survival of less than 2 years. The current treatment for brain cancer is usually chemotherapy. However, the therapeutic efficacy of many anticancer drugs is limited by the existence of the blood–brain barrier (BBB), the blood–brain tumor barrier (BBTB), a relatively weak enhanced permeability and retention (EPR) effect and by their severe side effects on healthy cells.1
The combination of therapeutic agents has recently attracted great attention for glioblastoma chemotherapy.2 It provides synergistic effects and decreases adverse side effects associated with high doses of single anticancer drugs and helps to avoid drug resistance.3 Over the last decade, the advances in nanomedicine have enabled the development of novel nanocarriers for site-specific drug delivery and to gain access to brain tumors.4,5 By taking advantages of these nanocarriers, different combinations of various drugs were co-loaded on nanoparticles for glioma therapy.6,7 Dilnawaz et al. loaded both curcumin and temozolomide into magnetic nanoparticles (MNPs) and studied their in vitro cytotoxic effects on 3D glioma tumor spheroids. The dual drug loaded MNPs formulations demonstrated higher cytotoxic effects than the single drug loaded MNPs formulations as compared to their corresponding native drugs in 2D and 3D culture.6 In another study Xu et al. studied in vitro cytotoxicity effects of paclitaxel and temozolomide co-loaded in polymer nanocomposites and the results suggested that the composite gel possessed much higher growth-inhibiting effects as well as apoptosis-inducing rates in glioma cells than other formulations.8
Niosomes are drug carriers exhibiting a bilayer structure and are in most cases formed by self-association of non-ionic surfactants and cholesterol in aqueous phase. They can accommodate a large number of drugs with a wide range of solubilities.9,10 Hydrophilic drugs and lipophilic drugs can be entrapped into the aqueous core and membrane bilayer of niosome respectively.11,12 Therefore, niosomes are promising nanocarriers in multi-drug delivery applications. The efficiency of niosomal multi-drug delivery systems can be further improved by active targeting for tumor therapy by using a ligand coupled to the surface of niosomes. In this way, nanocarriers can be actively taken up, for example, via receptor-mediated endocytosis.13 Recently, Tavano et al. reported the dual encapsulation of hydrophobic curcumin or quercetin and hydrophilic doxorubicin in Pluronic-based niosomes for cancer multi-drug delivery.14 Besides, the surface of the niosomes was coupled with transferrin and/or folic acid for breast cancer targeting. Results showed the high potential of the dual drug loaded niosomes in breast tumor treatment.
Neuropilin-1 (NRP-1) is a transmembrane protein overexpressed on the surface of both glioma and endothelial cells of angiogenic blood vessels.15–17 CGNKRTR (tLyP-1) is a homing peptide which penetrates tumor cell through an NRP-1 mediated endocytosis via C-end Rule (CendR) internalization pathway.18,19 Therefore, tLyP-1 is a promising targeting ligand for the delivery of therapeutic agents to tumors. Xu et al. produced tLyp-1 targeted camptothecin-loaded mesoporous silica nanoparticles and it showed minimal adverse effects on human mesenchymal stem cells (hMSC), whereas significant induction of tumor cell death was observed.20 Moreover, Hu et al. conjugated the tLyp-1 peptide to the surface of paclitaxel (PTX) loaded PEG–PLA nanoparticles via a maleimide-thiol coupling reaction for anti-glioma drug delivery. They achieved the longest survival of the mice bearing intracranial C6 glioma treated with PTX-loaded tLyp-1-nanoparticles in comparison to PTX loaded nanoparticles.17
In this study, polyethylene glycolated niosomes (PEGNIO) were prepared from span60, cholesterol and 1,2-distearoyl-sn-glycero-3-phosphoethanolamine-N-[maleimide (polyethylene glycol)-2000] (DSPE-PEG (2000) Maleimide) for multi-drug delivery. Curcumin (C) and doxorubicin (D) were encapsulated into the PEGNIO. The tumor-homing peptide tLyp-1 was conjugated to C and D encapsulated PEGNIO (PEGNIO/D–C) via the formation of a thioether linkage. The effect of the PEGNIO/D–C/tLyp-1 on human glioblastoma cells (U87) and hMSC was investigated in detail. Our results suggest that this targeted and co-loaded drug delivery platform could improve the efficacy of doxorubicin on glioma therapy.
Materials and methods
Materials
DSPE-PEG(2000) Maleimide was provided by Avanti (Alabama, USA). Sorbitan monostearate (Span60), cholesterol, 3-(4,5-dimethylthiazol-2-yl)-2,5-diphenyl tetrazoliumbromide (MTT), Dulbecco's Modified Eagle Medium (DMEM), curcumin and rhodamine 6G (Rh6g) were ordered from Sigma Aldrich (Munich, Germany). Alpha Minimum Essential Media (Alpha-MEM) was purchased from Life Technologies (Darmstadt, Germany). Doxorubicin hydrochloride was purchased from Cayman Chemical (Michigan, USA). tLyP-1 peptide (CGNKRTR) was ordered from GeneCust (Ellange, Luxembourg).
Preparation of niosomes
The thin film hydration method was used to prepare PEGylated niosomes.21 Span 60, cholesterol and DSPE-PEG(2000) Maleimide were dissolved in 1.0 mL chloroform in a round-bottom flask with the mM ratio (4.95
:
4.95
:
0.1). The solvent was evaporated with constant rotation under reduced pressure to form a thin lipid film. Doxorubicin or Rh6g loaded niosomes were obtained by hydrating the thin lipid film with 1.0 mL of doxorubicin or Rh6g (0.22 × 10−3 and 0.42 × 10−3 M, respectively) aqueous solution at 60 °C for 60 min.14,22 To obtain doxorubicin–curcumin-loaded niosomes, 200 μL of curcumin solution (2.18 × 10−3 M) was added to the initial chloroform mixture.14 After vortexing, the solvent was evaporated under reduced pressure, and the obtained film was then hydrated with 1.0 mL of doxorubicin aqueous solution at 60 °C for 60 min. Afterward, the niosomal solution was equilibrated at room temperature overnight to complete annealing and partitioning of the drug between the lipid bilayer and the aqueous phase.23 Small unilamellar vesicles (SUVs) were prepared starting from multilamellar vesicles (MLVs) by sonication in an ultrasonic bath and fallowing extruding the niosomes through 0.4 μm and 0.1 μm pore size polycarbonate filters (mini-extruder set Avanti polar lipids, sample volume 1.0 mL).
Niosomes were purified by a flow of niosome suspensions across a Sephadex G-25 gel column and volume of eluted niosomes was adjusted to 1.0 mL.
Preparation and characterization of tLyp-1 conjugated niosomes
For the preparation of tLyp-1 targeted niosomes, the thiol group of tLyp-1 was coupled with the maleimide group of PEG chains on niosomes. tLyp-1 peptide was dissolved in 50 mM HEPES buffer, pH 6.5 at 200 μg mL−1 concentration (50 μL) were reacted with niosomes (950 μL) for overnight at room temperature resulting in the formation of a thioether linkage.24,25 The products were then purified using a 14 kDa dialysis bag to remove the unconjugated peptides. Schematic representation of co-drug encapsulation and the bioconjugation processes are shown in Scheme 1.
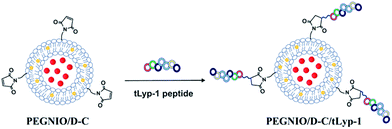 |
| Scheme 1 Schematic representation of co-drug encapsulation and the bioconjugation process (yellow dots: curcumin, red dots: doxorubicin). | |
The size of the niosomes were measured by dynamic light scattering (DLS) analysis using Malvern Zetasizer Nano ZSP. The polydispersity index (PDI) was used as a measure of the width of size distribution. Each sample was measured three times. The morphology of large unilamellar PEGNIO (before extrusion) was monitored via Olympus BX41 upright microscope. 100X immersion oil objective was used.
To estimate the tLyp-1 conjugation efficiency, BCA Protein Assay was used.4 25 μL of BSA standards, PEGNIO (as blank) and PEGNIO/tLyp-1 were added in triplicate wells (96-well plate) and 200 μL of BCA Protein Assay Reagent were added to the samples. After incubation at 37 °C for 30 min, the absorption was measured via microplate reader (BioTek Epoch) at 562 nm. Conjugation efficiency was expressed as the percentage of the peptide bound to the surface of noisome, referred to the amount of peptide that is present initially.
Stability
The stability of PEGNIO/D–C/tLyp-1 was tested via DLS analysis. PEGNIO/D–C/tLyp-1 was stored at 4 °C in the dark. The particle size and PDI values were measured after 30 days. Moreover, the particle size of PEGNIO/D–C/tLyp-1 was measured in cell culture media after the incubation at 37 °C for 24 h.
Entrapment efficiency
After encapsulation, the D–C loaded niosomes were purified using Sephadex G-25 gel column. The percent of encapsulation efficiency (E%) was expressed as the percentage of the drug entrapped in niosomes (and thus not removed via Sephadex column) referred to the initial amount of drug that is present in the non-purified sample (eqn (1)). 200 μL of non-purified and purified niosomes diluted in 600 μL of methanol and niosomes were separated by centrifugation at 12
000 rpm for 5 min. This step allows the breaking of niosomal membranes and the release of the encapsulated drug. A calibration curve was derived with a known concentration of free doxorubicin by fluorescence emission measurements at 595 nm using NanoDrop 3300. The stock solutions of doxorubicin were prepared at 1.0 mg mL−1 in methanol and further diluted with methanol in the concentration range 1.0–50 μg mL−1. The amount of doxorubicin in purified and non-purified samples was calculated according to the calibration curve (y = 850.94x + 12.092, R2 = 0.9973) via measuring fluorescence emission at 595 nm. The limit of detection (LOD) and limit of quantification (LOQ) for doxorubicin were found to be 4.92 μg mL−1 and 14.92 μg mL−1 respectively, based on 3.3σ/slope and 10sσ/slope formulations. The amount of curcumin in purified and non-purified samples was calculated according to the calibration curve (y = 0.1459x + 0.0058, R2 = 1, LOD = 0.15 μg mL−1 and LOQ = 0.46 μg mL−1), which was established with a known concentration of free curcumin (0.5–10 μg mL−1) by absorbance measurements at 426 nm using a microplate reader (BioTek Epoch). |
 | (1) |
Drug release
The in vitro release profiles of curcumin and doxorubicin from PEGNIO/D–C/tLyp-1 were studied using a dialysis method. PEGNIO/D–C/tLyp-1 solutions were prepared and transferred into a dialysis membrane tubing (Thermo, Slide-ALyzer MINI Dialysis Devices, 10k MWCO). The tubing was immersed in 10 mL of the PBS buffer (pH 5.6 and 7.4) containing Tween80 (1.0%, v/v), placed in an incubator at 37 °C and stirred at 100 rpm. Here, Tween 80 was added to obtain optimal release conditions since curcumin has limited solubility in PBS.26 At predetermined time intervals, 0.5 mL samples were removed from the release medium and replaced with the same volume of fresh buffer. The amount of released doxorubicin and curcumin was calculated as % according to the calibration curves and in respect to loading concentration (the drug concentration in niosomes, before starting the release). They were established with a known concentration of free doxorubicin and free curcumin by fluorescence emission measurements at 595 nm using NanoDrop 3300 and absorbance measurements at 426 nm using a microplate reader respectively.
Cell culture
U87 cell lines were provided from German Collection of Microorganisms and Cell Cultures (DSMZ). U87 cells were grown in DMEM containing 10% fetal calf serum (FCS) (Biochrom GmbH, Germany) and 1.0% penicillin/streptomycin (P/S). Human adipose-derived mesenchymal stem cells (hMSCs) were isolated from subcutaneous adipose tissues of 3 different patients scheduled for abdominoplasty after obtaining informed written consent, as approved by the Institutional Review Board, project #2251-2014 on 15th May, 2014. The isolated populations have been extensively characterized as mesenchymal stem cells by surface marker analysis and functional properties (differentiation capacity). hMSCs were cultured in alpha-MEM including 10% human serum (HS) (HS, c.c.pro GmbH, Germany) and 0.5% gentamicin. Both cell lines were cultivated and incubated with samples at 37 °C in a humidified environment with 5.0% CO2.
Cellular uptake
Rh6g was used as a model fluorescent dye and encapsulated into niosomes. The uptake of the Rh6g loaded niosomal formulations by U87 and hMSC cells was analyzed by flow cytometry. The cells (4 × 104) were treated with PEGNIO/Rh6g and PEGNIO/Rh6g/tLyp-1 for 2 h and treated cells were washed two times with PBS, and then analyzed in a BD Accuri C6 cytometer.
Cytotoxicity
The cytotoxic effects of niosomal formulations and free drugs were tested on U87 and hMSC cells using MTT assay. Cells (8 × 103) were seeded out in 96-well tissue plates (Sarstedt, USA) in a volume of 200 μL and cultivated for three days. After this cultivation time, cells were washed once with PBS and treated with PEGNIO/D–C, PEGNIO/D–C/tLyp-1 and free D–C for 24 h. The equivalent concentration of free doxorubicin and curcumin was used in niosomal formulations. Then the samples were removed and cells were incubated in 110 μL per well 10% MTT solution (5.0 mg mL−1 in PBS) in the medium for 4 h. During this incubation time, formazan complex was produced by the cells. 100 μL SDS solution (1.0 g SDS in 10 mL 0.01 M HCl) were added to each well to release the purple colored salt from the cells. After 24 h of incubation, UV-vis absorption was measured at 570 nm to 630 nm as the reference wavelength using a microplate reader Epoch Biotek. Besides, IC50 values (the drug concentration required for 50% inhibition of cell viability) were calculated for U87 cells using the growth sigmoidal/dose response function of Origin software.
Effect on tumor-like multicellular spheroids
The effect of niosomal formulations was tested on 3D spheroid cultures. U87 cells (8 × 103 per well) in 100 μL of culture medium were seeded into 96-well round-bottom ultra low attachment plates (Sarstedt, Germany) and incubated for 2 days to form spheroids. Afterward, 100 μL of each sample (D–C, PEGNIO/D–C, PEGNIO/D–C/tLyp-1) was added to the spheroids and incubated further for 2, 4 and 6 days. Bright-field images of U87 tumor-like spheroids treated with the samples were taken with Olympus IX50 inverted light microscope equipped with an Olympus camera (SC30, Japan) by using cellSens Standard software (Olympus Co. Japan).
Statistical analysis
Statistical data analysis was performed using the Student's t-test. The difference between two groups was considered significant when the p value was less than 0.05.
Results and discussion
Characterizations of niosomal formulations
The morphology of large unilamellar PEGNIO was monitored using an upright microscope (Fig. S1†). Fig. S1† indicated that PEGNIOs were spherical and homogeneous in shape. The mean diameters of empty and co-drug-loaded niosomal formulations, along with the corresponding polydispersity index (PDI) and drug entrapment efficiency (E%) values, are listed in Table 1. The empty vesicle size was analyzed to be 150.3 nm. When doxorubicin and curcumin were co-encapsulated, a slightly smaller hydrodynamic diameter as 144.1 nm was obtained. In our previous study we demonstrated, that the encapsulation of doxorubicin in PEGNIO did not resulted in an alteration of niosomal size.22 However, when a hydrophobic drug is encapsulated in the colloidal system, they were claimed to lead to H bonding between their hydroxyl groups and niosomal matrices, resulting in an increase in the niosomal cohesion and then a decrease in the diameter.14 Here, the decrease in the size can be attributed to the entrapment of hydrophobic curcumin in the bilayer. After the conjugation of tLyp-1, the size of the niosomes did not change significantly. The size was measured to be 146.1 nm for PEGNIO/D–C/tLyp-1. PDI less than 0.3 corresponds to a homogeneous population for colloidal systems.27 PDI ranged from 0.175 to 0.140 for the niosomal formulations, demonstrating that the vesicle population is relatively homogeneous in size. The entrapment efficacy (E%) was calculated to be 32.6 ± 1.9% for curcumin and 23.3 ± 1.6% for doxorubicin.
Table 1 Characterization of PEGNIO formulations
Samples |
Size (nm) intensity (%) (mean ± SD) |
Poly-dispersity index (PDI) |
Entrapment efficiency E% |
PEGNIO |
150.3 ± 51 |
0.175 |
— |
PEGNIO/D–C |
144.1 ± 61 |
0.152 |
D:23.3 ± 1.6, C:32.6 ± 1.9 |
PEGNIO/D–C/tLyp-1 |
146.1 ± 69 |
0.140 |
D:22.0 ± 1.5, C:31.2 ± 1.8 |
Under the determined experimental conditions, the t-Lyp-1 peptide conjugation efficiency was calculated to be 23.9%.
The stability of PEGNIO/D–C/tLyp-1 was tested via DLS analysis and no changes were observed in the size and PDI values after 30 days storage at 4 °C in the dark (data not shown). Furthermore, PEGNIO/D–C/tLyp-1 was diluted in cell culture media and was incubated at 37 °C for 24 hours. The size of the sample was measured and after incubation and no changes were observed.
Drug release
Prolonged drug retention and sustained drug release are important properties for nanoscale drug delivery systems that will minimize side effects of the drug.22 The unique structure of niosomes allows to control the release of the encapsulated drug combinations to increase antitumor activity.14 The release of doxorubicin and curcumin from PEGNIO/D–C/t-Lyp-1 was investigated using dialysis methods at pH 7.4, which was chosen in accordance with physiological conditions and in an acidic environment (pH 5.6) similar to the tumor. The release profiles of doxorubicin and curcumin from PEGNIO/D–C/t-Lyp-1 showed the faster release of both drugs under acidic environments than that at neutral pH (Fig. 1). Within 32 h, the release of doxorubicin and curcumin was 74 ± 1.2% and 62 ± 0.6% at pH 5.6 respectively. At physiological pH, the release of curcumin and doxorubicin was 36 ± 1.7% and 68 ± 2.9%. According to results, this conjugate is expected to be a promising co-drug delivery system for the tumor-targeted therapy.
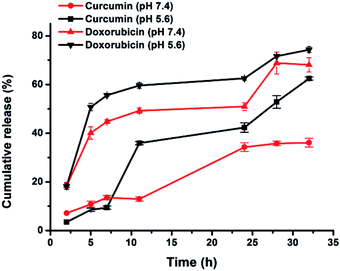 |
| Fig. 1 In vitro cumulative release of doxorubicin and curcumin from PEGNIO/D–C/t-Lyp-1 at pH 7.4 and 5.6. | |
Cellular uptake
To test the specificity of t-Lyp-1 targeted niosomes, Rh6g was encapsulated in PEGylated niosomes and further t-Lyp-1 was conjugated to PEG chains on niosomes. Flow cytometry was used to investigate the Rh6g uptake by hMSC and U87 cells to evaluate receptor-mediated cell targeting. The cells were treated with PEGNIO/Rh6g for 2 h. Untreated control cells and treated cells were analyzed using a BD Accuri C6 flow cytometer. No differences were observed between targeted and non-targeted niosomal formulations treated hMSC (Fig. 2a). In the case of U87 cells, the uptake of PEGNIO/Rh6g/tLyp-1 was higher than PEGNIO/Rh6g (Fig. 2b). Fig. 2c indicates that PEGNIO/Rh6g/tLyp-1 was uptaken by U87 cells specifically. tLyP-1 is able to selectively home in and penetrate into tumor cells mediated NRP-1 receptor which is overexpressed in tumor cells.20,28 The expression of NRP-1 receptor, on the surface of the U87 cells would affect the enhanced cellular uptake of PEGNIO/Rh6g/tLyp-1.
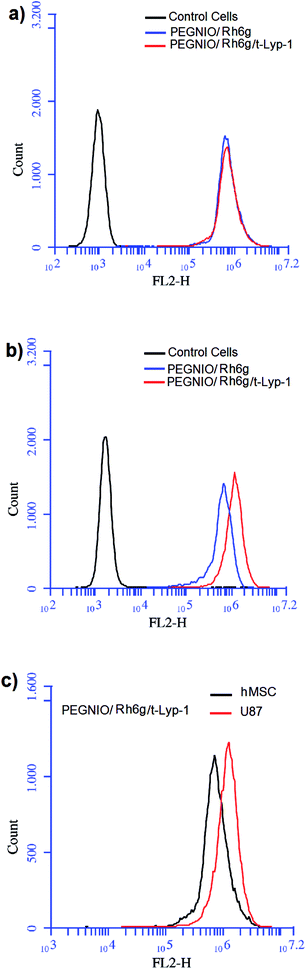 |
| Fig. 2 Flow cytometry measurements of Rh6g uptake by hMSC (a) and U87 cells (b) after incubating with PEGNIO/Rh6g and PEGNIO/Rh6g/t-Lyp-1. Histogram of binding of PEGNIO/Rh6g/t-Lyp-1 to hMSC and U87 cells (c). | |
Cytotoxicity
Doxorubicin is classified as a topoisomerase-2 inhibitor and one of the most extensively used broad-spectrum anticancer drugs. However, it has some limitations in clinical use. Long treatment durations cause the development of resistance by tumor cells and toxicity for healthy tissues. Therefore, combinations of two or more agents have been used to overcome toxicity and other side effects of doxorubicin.29,30 Curcumin's therapeutic characteristics have been demonstrated against a wide range of cancers.31–33 The major drawback of curcumin is its poor solubility and stability in water. Thus, here doxorubicin and curcumin were encapsulated in PEGNIO and further the surface of niosome was modified with t-Lyp-1 peptide.
After confirming the NRP-1 receptor-mediated glioma cell binding efficiencies of the PEGNIO/Rh6g/tLyp-1 via flow cytometry, MTT assays were performed to evaluate relative abilities of the various niosomal formulations in inhibiting growth of tumor (U87) and non-cancer (hMSC) cells (Fig.3). PEGNIO/D–C was more toxic than free D–C on both cell lines. Due to the conjugation of the targeting ligand to PEGNIO/D–C, PEGNIO/D–C/t-Lyp-1 was more toxic to U87 cells than to hMSC cells after 24 h (p < 0.05). PEGNIO/D–C/t-Lyp-1 significantly increased the cytotoxicity for U87 cells in comparison to free D–C (p < 0.05). Moreover, U87 cells were treated with samples (D–C, PEGNIO/D–C and PEGNIO/D–C/t-Lyp-1) for 48 h at concentration range of doxorubicin (0–50 μg mL−1). IC50 values were calculated as to be 0.96 μg mL−1, 0.9 μg mL−1 and 0.76 μg mL−1 for D–C, PEGNIO/D–C and PEGNIO/D–C/t-Lyp-1 respectively.
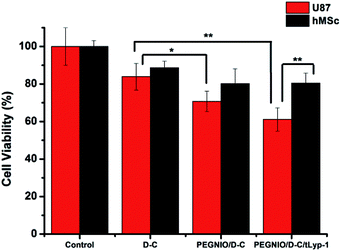 |
| Fig. 3 Cytotoxicity of the free drug and niosomal formulations on hMSC and U87 cells. Cells were incubated with, PEGNIO/D–C, PEGNIO/D–C/t-Lyp-1 and free D–C (equivalent concentration of loaded D–C) for 24 h. MTT assay was applied. Error bars represent the standard deviation from the mean (N = 3). Data were analyzed using t-test, and *p < 0.05, **p < 0.01 was considered significant and very significant respectively. | |
Effect on tumor-like multicellular spheroids
According to results obtained in 2D cell cultures, it is clear that the tLyp-1 conjugated niosomal formulation acted as a targeted multi-drug delivery platform for NRP-1 expressing tumor cells. Further, we have used 3D spheroid model which is found to be relevant for therapeutic evaluation, as it reflects better the in vivo conditions both in structural and molecular aspects.34 The effect on tumor-like spheroids was evaluated following the treatment with cell culture media, free D–C, PEGNIO/D–C and PEGNIO/D–C/tLyp-1 for 6 days. As shown in Fig. 4a, the tumor spheroids treated with cell culture media retained their morphology (compact spheroids with smooth surface) over entire period of cultivation. The spheroids treated with D–C became smaller in diameter (Fig. 4b). The tumor spheroids exposed to niosomal formulations exhibited no more tightly organised structure (Fig. 4c and d). Especially, PEGNIO/D–C/tLyp-1 treated spheroids became distorted, with many disassociated cells (Fig. 4d). These results demonstrated enhanced effects of PEGNIO/D–C/tLyp-1 on 3D tumor-like tissues in compare to other formulations.
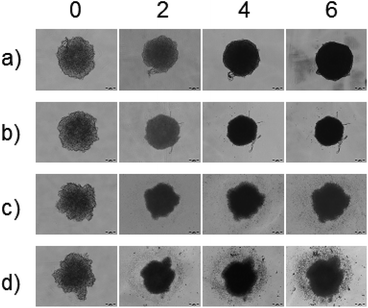 |
| Fig. 4 Morphology of U87 tumor spheroids treated with cell culture media (a), D–C (b), PEGNIO/D–C (c) and PEGNIO/D–C/t-Lyp-1 (d) on days 0, 2, 4 and 6, respectively. | |
Conclusion
In this work, a targeted niosomal co-drug delivery system was developed to improve the therapeutic efficacy of anti-glioma drug delivery. Curcumin and doxorubicin were encapsulated into PEGNIO by the thin film hydration method. The surface of PEGNIO was decorated with tLyp-1, which is tumor homing and penetrating peptide. Tumor-like structure dissociation in 3D tumor spheroids, enhanced cellular interaction, and increased cytotoxicity of the drugs in U87 cells were achieved by PEGNIO/D–C/tLyp-1. These data indicated that the formulation PEGNIO/D–C/tLyp-1 might be a promising and efficient strategy for drug delivery in anti-glioma therapy.
Acknowledgements
The Deutsche Forschungsgemeinschaft (DFG) within the REBIRTH cluster of excellence funded part of this work. Konrad Adenauer Foundation is acknowledged for the financial support to Didem Ag Seleci. We wish to thank André Jochums for his help with flow cytometry analysis. Special thank to Rebecca Jonczyk and Johanna-Gabriela Walter for technical checking of the manuscript. The publication of this paper was funded by the Open Access Fund of the Leibniz Universitat Hannover.
Notes and references
- X. Wei, X. Chen, M. Ying and W. Lu, Acta Pharm. Sin. B, 2014, 4, 193–201 CrossRef PubMed.
- B. Mujokoro, M. Adabi, E. Sadroddiny, M. Adabi and M. Khosravani, Mater. Sci. Eng., C, 2016, 69, 1092–1102 CrossRef CAS PubMed.
- B. Al-Lazikani, U. Banerji and P. Workman, Nat. Biotechnol., 2012, 30, 679–692 CrossRef CAS PubMed.
- Q. Hu, G. Gu, Z. Liu, M. Jiang, T. Kang, D. Miao, Y. Tu, Z. Pang, Q. Song and L. Yao, Biomaterials, 2013, 34, 1135–1145 CrossRef CAS PubMed.
- D. Miao, M. Jiang, Z. Liu, G. Gu, Q. Hu, T. Kang, Q. Song, L. Yao, W. Li and X. Gao, Mol. Pharmaceutics, 2013, 11, 90–101 CrossRef PubMed.
- F. Dilnawaz and S. K. Sahoo, Eur. J. Pharm. Biopharm., 2013, 85, 452–462 CrossRef CAS PubMed.
- Y. Cui, Q. Xu, P. K.-H. Chow, D. Wang and C.-H. Wang, Biomaterials, 2013, 34, 8511–8520 CrossRef CAS PubMed.
- Y. Xu, M. Shen, Y. Sun, P. Gao and Y. Duan, J. Nanosci. Nanotechnol., 2015, 15, 9777–9787 CrossRef CAS PubMed.
- A. Sankhyan and P. Pawar, J. Appl. Pharm. Sci., 2012, 02, 20–32 Search PubMed.
- D. Pando, M. Matos, G. Gutiérrez and C. Pazos, Colloids Surf., B, 2015, 128, 398–404 CrossRef CAS PubMed.
- L. Tavano, R. Muzzalupo, N. Picci and B. de Cindio, Colloids Surf., B, 2014, 114, 144–149 CrossRef CAS PubMed.
- L. Tavano, L. Gentile, C. O. Rossi and R. Muzzalupo, Colloids Surf., B, 2013, 110, 281–288 CrossRef CAS PubMed.
- D. Ag Seleci, M. Seleci, J.-G. Walter, F. Stahl and T. Scheper, J. Nanomater., 2016, 2016, 7372306 Search PubMed.
- L. Tavano, L. Mauro, G. D. Naimo, L. Bruno, N. Picci, S. Ando and R. Muzzalupo, Langmuir, 2016, 32, 8926–8933 CrossRef CAS PubMed.
- P. Li and T. G. Rossman, Toxicol. Sci., 2001, 64, 90–99 CrossRef CAS PubMed.
- C. Nasarre, M. Roth, L. Jacob, L. Roth, E. Koncina, A. Thien, G. Labourdette, P. Poulet, P. Hubert and G. Cremel, Oncogene, 2010, 29, 2381–2392 CrossRef CAS PubMed.
- Q. Hu, X. Gao, G. Gu, T. Kang, Y. Tu, Z. Liu, Q. Song, L. Yao, Z. Pang and X. Jiang, Biomaterials, 2013, 34, 5640–5650 CrossRef CAS PubMed.
- E. Ruoslahti, Adv. Mater., 2012, 24, 3747–3756 CrossRef CAS PubMed.
- L. Roth, L. Agemy, V. R. Kotamraju, G. Braun, T. Teesalu, K. N. Sugahara, J. Hamzah and E. Ruoslahti, Oncogene, 2012, 31, 3754–3763 CrossRef CAS PubMed.
- B. Xu, Y. Ju, G. Song and Y. Cui, J. Nanopart. Res., 2013, 15, 2105 CrossRef.
- M. N. Azmin, A. T. Florence, R. M. Handjani Vila, J. F. B. Stuart, G. Vanlerberghe and J. S. Whittaker, J. Pharm. Pharmacol., 1985, 37, 237–242 CrossRef CAS PubMed.
- D. A. Seleci, M. Seleci, A. Jochums, J.-G. Walter, F. Stahl and T. Scheper, RSC Adv., 2016, 6, 87910–87918 RSC.
- L. Tavano, R. Muzzalupo, L. Mauro, M. Pellegrino, S. Ando and N. Picci, Langmuir, 2013, 29, 12638–12646 CrossRef CAS PubMed.
- R. Nallamothu, G. C. Wood, C. B. Pattillo, R. C. Scott, M. F. Kiani, B. M. Moore and L. A. Thoma, AAPS PharmSciTech, 2006, 7, E7–E16 CrossRef PubMed.
- Q. Hu, X. Gao, T. Kang, X. Feng, D. Jiang, Y. Tu, Q. Song, L. Yao, X. Jiang and H. Chen, Biomaterials, 2013, 34, 9496–9508 CrossRef CAS PubMed.
- M. Tan, J. Luo and Y. Tian, RSC Adv., 2014, 4, 61948–61959 RSC.
- L. Tavano, R. Aiello, G. Ioele, N. Picci and R. Muzzalupo, Colloids Surf., B, 2014, 118, 7–13 CrossRef CAS PubMed.
- B. Xu, Y. Ju, Y. Cui, G. Song, Y. Iwase, A. Hosoi and Y. Morita, Langmuir, 2014, 30, 7789–7797 CrossRef CAS PubMed.
- L. Sun, X. Deng, X. Yang, Z. Li, Z. Wang, L. Li, Q. Wu, F. Peng, L. Liu and C. Gong, RSC Adv., 2014, 4, 46737–46750 RSC.
- X. Zhao, Q. Chen, Y. Li, H. Tang, W. Liu and X. Yang, Eur. J. Pharm. Biopharm., 2015, 93, 27–36 CrossRef CAS PubMed.
- M. Singh and N. Singh, Mol. Cell. Biochem., 2011, 347, 1–11 CrossRef CAS PubMed.
- N. Dhillon, B. B. Aggarwal, R. A. Newman, R. A. Wolff, A. B. Kunnumakkara, J. L. Abbruzzese, C. S. Ng, V. Badmaev and R. Kurzrock, Clin. Cancer Res., 2008, 14, 4491–4499 CrossRef CAS PubMed.
- Y. G. Lin, A. B. Kunnumakkara, A. Nair, W. M. Merritt, L. Y. Han, G. N. Armaiz-Pena, A. A. Kamat, W. A. Spannuth, D. M. Gershenson and S. K. Lutgendorf, Clin. Cancer Res., 2007, 13, 3423–3430 CrossRef CAS PubMed.
- J. Friedrich, C. Seidel, R. Ebner and L. A. Kunz-Schughart, Nat. Protoc., 2009, 4, 309–324 CrossRef CAS PubMed.
Footnote |
† Electronic supplementary information (ESI) available. See DOI: 10.1039/c7ra05071b |
|
This journal is © The Royal Society of Chemistry 2017 |
Click here to see how this site uses Cookies. View our privacy policy here.