DOI:
10.1039/C7RA03741D
(Paper)
RSC Adv., 2017,
7, 30289-30294
Quantification analysis of protein and mycelium contents upon inhibition of melanin for Aspergillus niger: a study of matrix assisted laser desorption/ionization mass spectrometry (MALDI-MS)†
Received
31st March 2017
, Accepted 19th May 2017
First published on 12th June 2017
Abstract
Mass spectrometry (MS) provides a simple discrimination method for microorganisms. However, the presence of species such as melanin in fungal spores of Aspergillus niger (melanotic fungal) suppress ionization for matrix assisted laser desorption/ionization mass spectrometry (MALDI-MS). Inhibition of melanin synthesis pathways by tricyclazole enhances mycelium growth and protein contents of Aspergillus niger for four different media; namely sabouraud dextrose agar medium (SDA), potato dextrose agar (PDA), czapek dox agar (CDA) and yeast extract agar (YEA) media. The cell contents of protein and mycelium growth of Aspergillus niger are increased with the addition of a low concentration of tricyclazole (25–50 mg L−1). Furthermore, it improves the ionization signals of A. niger for MALDI-MS. This study reveals that inhibition of melanin using tricyclazole leads to the increase of protein content, mycelium growth and enhanced peak signals of MALDI-MS.
Introduction
Matrix assisted laser desorption/ionization mass spectrometry (MALDI-MS) has been applied for several applications such as biotechnology,1–5 nanoscience, analytical chemistry and material science.6–15 Identification and detection of intact cells using MALDI-MS is promising for clinical laboratories.16–23 MALDI-MS has been established as the standard identification technology for cultivated microorganisms in most clinical laboratories around the world.24 MALDI-MS has a prominent role in the field of biomedical science and medicine.25 Mass spectra provide a fingerprint of the investigated microorganism; such as bacteria20,26–30 and fungal species.31,32 The identification of fungal species is important for clinical medicine and food production. Compared to traditional methods, analysis using MALDI-MS is rapid, simple and offers an useful diagnostic tool for microorganism.33 MALDI-MS provides an alternative method for other expensive methods such as molecular based methods or antibody based technologies. MALDI-MS offers an interested cost-per-analysis,34 and high throughput proteomics analysis.35 Spectra of MALDI-MS provide several information regarding to taxonomic identification, microbial interaction, dereplication and drug discovery.36 The detection of microorganism using mass spectrometry showed an identification error of 0% and 1.4% for re-substitution and cross-validation methods, respectively.37 A study showed that the correlation between MALDI-MS and conventional identification for a 125 fungal isolates was 87.2% at the species level and 90.4% at the genus level.38 Detect of a cell contamination using MALDI-MS is sensitive method and shows low limit of detection.39
Water contaminated with fungal species is a serious threats to human health especially for immuno-compromised patients.40,41 Infection by Aspergillus species was considered one of the major infection in hospitals.42 Aspergillus species are a leading cause of invasive fungal infections.43 However, it is important to mention that these species showed potential applications for biotechnology. Among Aspergillus species, Aspergillus niger is reported as an important fungi with a high capacity for decomposing plant materials due to many of the secreted depolymerizing enzymes.44 Conventional methods of identifying Aspergillus species are based on macroscopic or microscopic morphology. These methods showed limited ability to identify some of Aspergillus species. Advanced techniques; included matrix assisted laser desorption/ionization mass spectrometry (MALDI-MS), fluorescence in situ hybridization (FISH), have improved species identification and analysis of microorganism at clinical microbiological routine laboratories. However, various challenges are still associated with performance and interpretation of the testing species using MALDI-MS.45
Presence of species such as melanin, is a heterogeneous and dark pigments of high molecular weight of oxidative phenolic polymers, causes ions signal suppression of A. niger.46 They behave as “ghosts”.46,47 Melanin provides as a defense wall against environmental stresses such as ultraviolet (UV) light, and ionizing radiation.48 Melanin dissipates the absorbed laser energy as vibration, rotation motion or as heat. Thus, it prevents the ionization of the biomolecules of A. niger. The analysis of A. niger using MALDI-MS is a challenging and showed difficulties in the detection of biomarkers for this species.49 This observations encouraged us to investigate the inhibition effect of melanin using tricyclazole on A. niger (melanotic fungal) via measuring the protein and mycelium contents. The present study showed that the inhibition of melanin for Aspergillus niger using fungicide (tricyclazole; 5-methyl-l,2,4-triazolo-[3,4-b]-benzothiazole]) in four different culture media; sabouraud dextrose agar medium (SDA), potato dextrose agar (PDA), czapek dox agar (CDA) and yeast extract agar (YEA) media, increased of the cell protein contents and mycelium growth. To give a direct and simple view of the change in cell lysate, MALDI-MS spectra are recorded. Different concentrations of tricyclazole showed an increase of the cell proteins and mycelium contents. Inhibition of melanin improved the signals of MALDI-MS.
Experimental
Chemicals and reagents
Tricyclazole was purchased from Wako Pure Chemical Industries (Osaka, Japan). Sabouraud dextrose agar medium (SDA), potato dextrose agar (PDA), czapek dox agar (CDA) and yeast extract agar (YEA) media were purchased from Difco (Sparks, MD, USA). α-Cyano 4-hydroxycinnamic acid (CHCA), trifluoroacetic acid (TFA), formic acid, 2,5-dihydroxybenzoic acid (DHB), formic acid were purchased from Sigma (USA). De-ionized (DI) water was purified using Milli-Q system (Millipore, Bedford, MA, USA). All reagents were purchased from Sigma-Aldrich (St. Louis, MO, USA) and were used without further purification.
Fungal growth
Aspergillus niger (BCRC 30402) was purchased from Bioresource Collection and Research Center (BCRC, Hsinchu, Taiwan). Before MALDI-MS detection, reference strain was cultured in PDA medium, and then transferred to SDA, YEA and CDA media (500 mL) at 30 °C with shaking condition at 200 rpm. Different concentration of tricyclazole was added to the cell cultures for 12 days. Mycelia were harvested, filtered under pressure, and washed with phosphate buffer (100 mM, pH value of 7.2). The extract was re-suspended in lysis buffer (5 mL of lysis buffer per gram of mycelia) containing Tris–HCl buffer (100 mM of Tris–HCl, 50 mM of NaCl, 20 mM of ethylenediaminetetraacetic acid (EDTA), 10% (v/v) glycerol, 30 mM of dithiothreitol (DTT), 1 mM of phenylmethanesulfonylfluoride or phenylmethylsulfonyl fluoride (PMSF), pH 7.5). Then, formic acid (70%) and acetonitrile were added. The medium for growing fungal spores was composed of PDA mixed with granulated agar (15 g L−1, Becton Dickinson, MD, USA) and 25 mg L−1 of tricyclazole. Cultivation on agar plates was performed with this medium at ca. 30 °C for 1–7 days. A pipette tip was employed to scratch the spores cultured on a medium plate. Data was statistically analysis for the four media using one-way ANOVA (MiniTab® 17) that showed significance level (α), F-value and P-value of 0.05, 0.08, and 0.968, respectively.
Protein estimation
The total protein content of mycelial extracts were estimated using the method of Bradford. The protein contents were analyzed using UV spectrophotometer (U-3501 spectrophotometer (Hitachi Corporation, Japan)). For quantitative analysis, bovine serum albumin (BSA) was used as a standard protein. Data was statistically analysis for the four media using one-way ANOVA (MiniTab® 17) that showed significance level (α), F-value and P-value of 0.05, 0.12, and 0.949, respectively.
MALDI-MS
Results of MALDI-TOF-MS analyses of A. niger (BCRC 30402) strain grown on PDA (potato dextrose agar with 50 mg L−1 of tricyclazole) at 30 °C, at varying incubation times (2 to 10 days), showed that the best profile spectra (quality and number of peaks) were obtained using 6 days young mycelium. Afterwards, the mycelium were harvested from Petri plate and washed with phosphate buffer (50 mM, pH value of 7.2). The harvest was suspended in formic acid (70%) followed by acetonitrile. The colloid solution was centrifuge and the supernatant were spotted onto stainless steel Bruker target plate (Bruker, Germany). Then, 1 μL of matrix solution of 2,5-dihydroxybenzoic acid (DHB, 75 mg mL−1), α-cyano-4-hydroxycinnamic acid (CHCA, 75 mg mL−1) and sinapinic acid (SA, 75 mg mL−1) prepared in acetonitrile/ethanol/water (1
:
1
:
1) supplemented with 3% trifluoroacetic acid was immediately added on the top of the sample spots. Mass spectra were obtained in positive ion mode using MALDI-MS (Microflex, Bruker Daltonics, Bremen, Germany). MALDI ionization source was equipped with a nitrogen laser (wavelength of 337 nm), time of flight tube (TOF) and accelerating voltages was set at +20 kV. All experiments were performed in the linear mode with laser energy of 63.2 μJ. In order to decrease false positive identification of cell biomolecules, experiments were done at least in duplicate.
Results and discussion
Aspergillus niger is a darkly pigmented fungi. The darkness of Aspergillus niger is due to the presence of melanin (Fig. S1†). Melanin has chromophoric groups that have absorption in the range of 200–800 nm. The presence of melanin in the cell suppresses ionization of the intact cell. After 6 days of the fungus culture in media without tricyclazole; the fungi shows a dark color due to accumulation of melanin in their cell wall (Fig. S1†). Alviano et al. reported that the dark color depends on the culture conditions.50 The presence of these species can be inhibited using fungicides such as tricyclazole.46
Fig. 1a shows the difference in fungal mycelium mass accumulation in the different culture media (SDA, PDB, YEA, and CDA) tested amended with several of concentration of tricyclazole (0–100 mg L−1). We observed that there are low accumulations of fungal mass in the absence of tricyclazole (Fig. S1†). In contrast, in the presence of tricyclazole, there are higher accumulations of fungal mass. In the presence of 25 mg L−1 of tricyclazole in all the media, the growth of fungal biomass was high followed by 50 mg L−1 of tricyclazole (Fig. 1a). Further, we found that the mycelium growth in SDA amended with 25 mg L−1 of tricyclazole show highest biomass on 6th day (Fig. 1a). However, the low biomass in higher concentration of tricyclazole amended media was noted (Fig. 1a). Alternatively and during log phase growth, a detoxification mechanism of the cell could take place. Thus, tricyclazole becomes less effective in older cultures after the inhibition of melanin production.
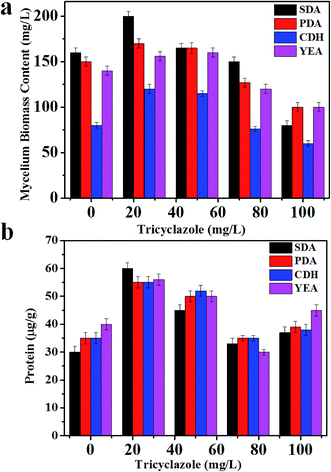 |
| Fig. 1 (a) Mycelia biomass of A. niger BCRC 30402 in different media supplemented with different concentration of tricyclazole; and (b) protein content of A. niger BCRC 30402 in different media supplemented with different concentration of tricyclazole. | |
Protein content
Highest amount of the intracellular protein content (60 μg g−1) was observed for 25 mg L−1 of tricyclazole amended in both PDA and SDA media followed by 50 mg L−1 of tricyclazole (55 μg g−1) (Fig. 1b). According to the previous study of A. niger cultivated on SDA, it has been produced more protein compared to other culture media. We found highest intracellular protein content for 50 mg L−1 of tricyclazole followed by 25 mg L−1 tricyclazole for the media of YEA and CDA (Fig. 1b). These results indicate that the protein contents increased using low concentration of tricyclazole. However, the higher concentration of tricyclazole amended mycelium showed decrease of the protein content in those media (Fig. 1b). The main reason for the decrease of the protein secretion in higher tricyclazole administration is due to unknown reasons. The secretory potential of the A. niger proteins was reported by studying the impact of secretion stress-inducing chemicals, temperature shifts, protein overproduction, or growth on carbon sources.51,52 They referred these changes to transcriptional and translational responses in A. niger.
MALDI-MS profiling of A. niger upon addition of tricyclazole
The selection of matrix and their solvent affect the results of MALDI mass spectra.53 As a first step, several matrices (SA, 2,5-DHB and CHCA) were tested for the analysis of A. niger fungal spores. Fig. 2(a)–(d) presents MALDI mass spectra of fungal spores from A. niger amended with 50 mg L−1 of tricyclazole. The cells were directly scratched from agar plates and then mixed with solutions of SA, 2,5-DHB and CHCA, respectively. Laser desorption/dionization mass spectrometry (LDI-MS) of A. niger is not show any signal (Fig. 2a). Spectra using 2,5-DHB matrix show more peaks compared to SA and CHCA matrix. It is important to keep in mind that the signals of A. niger without the addition of tricyclazole is weak due to the presence of melanin.46 These results point out that the solution of 2,5-DHB is a suitable matrix for MALDI-MS analyses. The peak identification agrees with our previous report.54
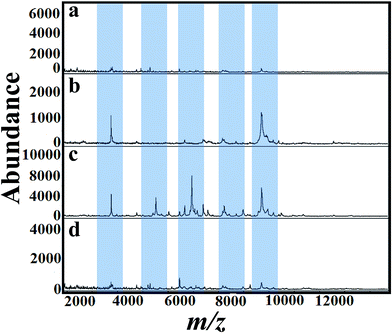 |
| Fig. 2 MALDI mass spectra of fungal spores scratched directly in Petri plate from A. niger (BCRC 30402) amended with tricyclazole mixed with matrices (a) control (b) SA (c) 2,5-DHB and (d) CHCA. Significant peak changes are highlighted. | |
For a clear identification using MALDI-MS, spectrum of A. niger as a reference strain was recorded. The cells were cultured in potato dextrose medium then transferred to different fungal growth media like SDA, PDA, YEA and CDA broth (500 mL, 30 °C). Different concentrations of tricyclazole were amended for inhibition of melanin synthesis for 1–7 days. The effect of age of the mycelium and media on MALDI patterns obtained on 6th days using different media was analyzed. Before 5 days, we couldn't find any observable peaks in those media. Although after the 6th day of mycelium, the peaks signals showed higher intensities for all the growth media. Fig. 3(a)–(e) presents spectra of fungal mycelium from A. niger (BCRC 30402) in SDA broth medium amended with 0, 25, 50, 75 and 100 mg L−1 of tricyclazole, respectively. Fig. 3(a)–(e) shows that 50 mg L−1 of tricyclazole is the optimum amount of the fungicide that causes high ionization of the cells biomolecules (Fig. 3c). All the spectra show high reproducibility and are matched with each other.
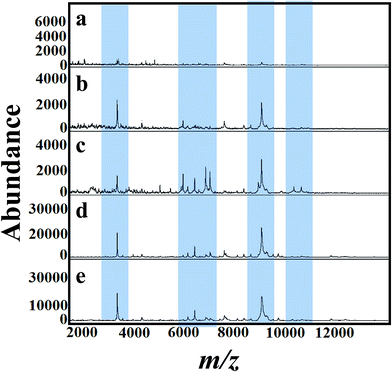 |
| Fig. 3 MALDI mass spectra of fungal mycelium from A. niger (BCRC 30402) cultured in SDA supplemented with different concentration of tricyclazole; (a) control (b) 25 mg L−1, (c) 50 mg L−1, (d) 75 mg L−1 and (e) 100 mg L−1, respectively. Significant peak changes are highlighted. | |
Fig. 4(a)–(e) presents MALDI mass spectra of A. niger mycelium from PDA broth medium amended with different concentration of tricyclazole i.e. 0, 25, 50, 75, and 100 mg L−1, respectively. The lowest concentrations of tricyclazole (25 and 50 mg L−1) amended culture induced a lot of mass peaks. In other side, ion suppression was observed for higher concentrations (75 and 100 mg L−1 of tricyclazole amended culture) (Fig. 4a). These observations agree with the quantitative data from the mycelium (Fig. 1a) and protein contents (Fig. 1b).
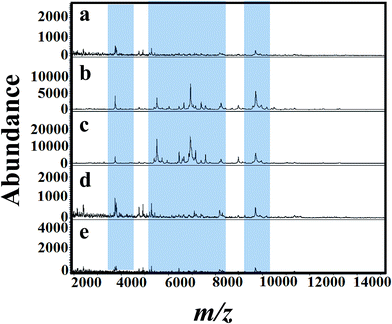 |
| Fig. 4 MALDI mass spectra of fungal mycelium from strains of A. niger (BCRC 30402) cultured in PDA supplemented with different concentration of tricyclazole; (a) control (b) 25 μgmL−1, (c) 50 μg mL−1, (d) 75 μg mL−1 and (e) 100 mg L−1 respectively. Significant peak changes are highlighted. | |
Fig. 5(a)–(e) shows MALDI mass spectra of A. niger (BCRC 30402) mycelium from YED broth medium supplemented with different concentrations of tricyclazole, i.e. 0, 25, 50, 75, and 100 mg L−1, respectively. A. niger without tricyclazole show low signals peaks due to melanin (Fig. 5a). The signals have been improved via the addition of tricyclazole (50 mg L−1, Fig. 5c). Fig. 6(a)–(e) shows MALDI mass spectra of A. niger mycelium from CDA broth amended with different concentration of tricyclazole including 0, 25, 50, 75, and 100 mg L−1. A. niger of CDA (Fig. 6a–e) plays the same profile as in YED.
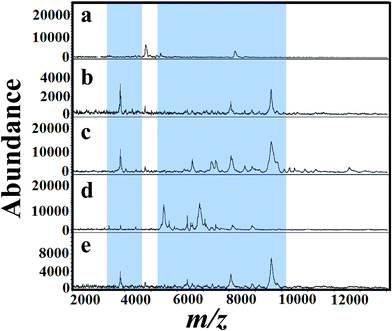 |
| Fig. 5 MALDI mass spectra of fungal mycelium from A. niger (BCRC 30402) cultured in YEA addition with various concentration of tricyclazole; (a) control (b) 25 mg L−1, (c) 50 mg L−1, (d) 75 mg L−1 and (e) 100 mg L−1, respectively. Significant peak changes are highlighted. | |
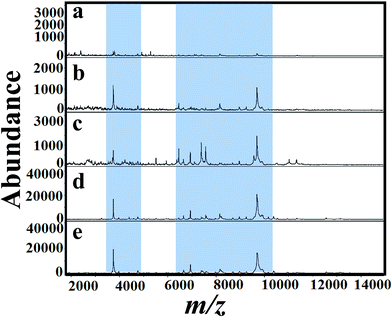 |
| Fig. 6 MALDI mass spectra of fungal mycelium from A. niger (BCRC 30402) cultured in CDA amended with different concentration of tricyclazole; (a) control (b) 25 mg L−1, (c) 50 mg L−1, (d) 75 mg L−1 and (e) 100 mg L−1, respectively. Significant peak changes are highlighted. | |
Fig. 1 shows approximately the same results as in the case of 25 and 50 mg L−1 of tricyclazole. MALDI-MS spectra show significant difference of the two concentrations (Fig. 2–6). The results indicate that MALDI-MS is a powerful tool to record the changes of the intact cell. It is important to note that the fungal cells are typically larger than bacterial cells and have rigid walls which architecturally, albeit not chemically. Furthermore, the fungal cell walls are generally made up of 80–90% polysaccharide, including the presence of the long chain carbohydrate polymers which increase the intact cells' rigidity and serve as a structural support to the thin cells. It is important to mention that melanin granules are localized to the cell wall where they are likely cross-linked to polysaccharides.48 Inhibition of melanin using tricyclazole weakens the cell wall and change the surface charge of A. niger. These changes can be reasons for the signal enhancement. Inhibition of melanin of Fonsecaea pedrosoi using tricyclazole was reported.55 Authors reported that the inhibition of melanin pathways reduced the strength of the fungus against macrophages, by weakening the fungus cell wall and altering the surface charge of the fungus 55. Analyses of intact microorganism cells using MALDI-MS face some limitations, especially intact cells analysis. Firstly, the presence of large number of biomolecules may leads to ion suppression. The analysis can be improved using extraction or separation of the cell biomolecules using nanoparticles.56–58 The ion suppression could be also due to laser absorbed species such as melanin as shown here. The inhibition of melanin using tricyclazole for the analysis the intact cells of A. niger is a new strategy without the need of expensive or toxic reagents.46 Second, data collection requires expertise to obtain reproducible results. The lack of high reproducibility is mainly due to presence of traces species of melanin. Thus, using the current approach we found high reproducibility compared to the direct analysis of the intact cells. Third, the standardization of direct detection against the reference test is not perfect for A. niger and leading to misclassification. Using the current method, we believe that the analysis of A. niger will be easier and show high reproducibility with better identification.
Conclusions
In summary, tricyclazole amended A. niger showed increase of the fungal mycelium growth and protein contents for four different media. Statistical analysis (one way ANOVA) indicates that the protein and mycelium upon the addition of tricyclazole are the same for the four investigated medium. This observation indicates that the cell medium did not dramatically affect the results. The inhibition of melanin using tricyclazole overcome the ion suppression of A. niger and improved the signals of the ionized-desorbed species using laser shots. The signal improvements enhance the cell identification and can reduce the errors. A further study of the cell changes upon the addition of tricyclazole is important. It may open a new venue for clinical and biomedical applications.
Acknowledgements
The authors gratefully acknowledge the financial support from National Science Council, Taiwan. H. N. Abdelhamid thanks Assuit University, Egypt for the support to carry this work.
References
- C. Fenselau and P. A. Demirev, Mass Spectrom. Rev., 2001, 20, 157–171 CrossRef CAS PubMed
. - O. Šedo, I. Sedláček and Z. Zdráhal, Mass Spectrom. Rev., 2011, 30, 417–434 CrossRef PubMed
. - R. J. Arnold, J. A. Karty, A. D. Ellington and J. P. Reilly, Anal. Chem., 1999, 71, 1990–1996 CrossRef CAS PubMed
. - T. C. Dingle and S. M. Butler-Wu, Clin. Lab. Med., 2013, 33, 589–609 CrossRef PubMed
. - J. Krismer, R. Steinhoff and R. Zenobi, CHIMIA International Journal for Chemistry, 2013, 33, 589–609 CrossRef PubMed
. - T. C. Baker, J. Han and C. H. Borchers, Curr. Opin. Biotechnol., 2017, 43, 62–69 CrossRef CAS PubMed
. - C. Y. Shi and C. H. Deng, Analyst, 2016, 141, 2816–2826 RSC
. - F. Chen, B. Gülbakan, S. Weidmann, S. R. Fagerer, A. J. Ibáñez and R. Zenobi, Mass Spectrom. Rev., 2016, 35, 48–70 CrossRef CAS PubMed
. - K. O. Schubert, F. Weiland, B. T. Baune and P. Hoffmann, Proteomics, 2016, 16, 1747–1758 CrossRef CAS PubMed
. - I. C. Santos, Z. L. Hildenbrand and K. A. Schug, Analyst, 2016, 141, 2827–2837 RSC
. - D. Sturtevant, Y. J. Lee and K. D. Chapman, Curr. Opin. Biotechnol., 2016, 37, 53–60 CrossRef CAS PubMed
. - H. N. Abdelhamid, J. Data Min. Genomics Proteomics, 2016, 7, 1–6 Search PubMed
. - H. N. Abdelhamid and H. F. Wu, Microchim. Acta, 2017, 184, 1517–1527 CrossRef CAS
. - H. N. Abdelhamid, A. Talib and H.-F. Wu, Talanta, 2016, 166, 357–363 CrossRef PubMed
. - H. N. Abdelhamid, TrAC, Trends Anal. Chem., 2017, 89, 68–98 CrossRef CAS
. - S. Chen, C. Xiong, H. Liu, Q. Wan, J. Hou, Q. He, A. Badu-Tawiah and Z. Nie, Nat. Nanotechnol., 2015, 10, 176–182 CrossRef CAS PubMed
. - P. J. Jannetto and R. L. Fitzgerald, Clin. Chem., 2015, 62, 92–98 Search PubMed
. - H. N. Abdelhamid, S. Kumaran and H.-F. Wu, RSC Adv., 2016, 6, 97629–97635 RSC
. - H. N. Abdelhamid, M. S. Khan and H.-F. Wu, Anal. Chim. Acta, 2014, 823, 51–60 CrossRef CAS PubMed
. - B.-S. Wu, H. N. Abdelhamid and H.-F. Wu, RSC Adv., 2014, 4, 3722 CAS
. - H. N. Abdelhamid and H.-F. Wu, TrAC, Trends Anal. Chem., 2014, 65, 30–46 CrossRef
. - H. N. Abdelhamid, A. Talib and H.-F. Wu, RSC Adv., 2015, 5, 34594–34602 RSC
. - M. L. Bhaisare, H. N. Abdelhamid, B.-S. Wu and H.-F. Wu, J. Mater. Chem. B, 2014, 2, 4671 RSC
. - M. Kostrzewa and E. Nagy, Expert Rev. Mol. Diagn., 2016, 16, 509–511 CrossRef CAS PubMed
. - A. Karger, Proteomics: Clin. Appl., 2016, 10, 982–993 CrossRef CAS PubMed
. - H. N. Abdelhamid, TrAC, Trends Anal. Chem., 2016, 77, 122–138 CrossRef CAS
. - H. N. Abdelhamid, Mass Spectrometry & Purification Techniques, 2015, 1, 109–119 Search PubMed
. - H. N. Abdelhamid, M. L. Bhaisare and H.-F. Wu, Talanta, 2014, 120, 208–217 CrossRef CAS PubMed
. - H. N. Abdelhamid, J. Gopal and H. F. Wu, Anal. Chim. Acta, 2013, 767, 104–111 CrossRef CAS PubMed
. - H. N. Abdelhamid and H.-F. Wu, Anal. Chim. Acta, 2012, 751, 94–104 CrossRef CAS PubMed
. - P. T. Becker, A. de Bel, D. Martiny, S. Ranque, R. Piarroux, C. Cassagne, M. Detandt and M. Hendrickx, Med. Mycol., 2014, 52, 826–834 CrossRef PubMed
. - S. Ulrich, B. Biermaier, O. Bader, G. Wolf, R. K. Straubinger, A. Didier, B. Sperner, K. Schwaiger, M. Gareis and C. Gottschalk, Anal. Bioanal. Chem., 2016, 408, 7565–7581 CrossRef CAS PubMed
. - V. Sandalakis, I. Goniotakis, I. Vranakis, D. Chochlakis and A. Psaroulaki, Expert Rev. Proteomics, 2017, 14, 253–267 CrossRef CAS PubMed
. - A. van Belkum, S. Chatellier, V. Girard, D. Pincus, P. Deol and W. M. Dunne, Expert Rev. Proteomics, 2015, 12, 595–605 CrossRef CAS PubMed
. - R. Aebersold and M. Mann, Nature, 2003, 422, 198–207 CrossRef CAS PubMed
. - T. Luzzatto-Knaan, A. V. Melnik and P. C. Dorrestein, Analyst, 2015, 140, 4949–4966 RSC
. - J. M. Hettick, B. J. Green, A. D. Buskirk, J. E. Slaven, M. L. Kashon and D. H. Beezhold, in Rapid Characterization of Microorganisms by Mass Spectrometry, ACS Symposium Series, 2011, pp. 35–50 Search PubMed
. - A. Panda, A. K. Ghosh, B. R. Mirdha, I. Xess, S. Paul, J. C. Samantaray, A. Srinivasan, S. Khalil, N. Rastogi and Y. Dabas, J. Microbiol. Methods, 2015, 109, 93–105 CrossRef CAS PubMed
. - J. M. P. Ferreira de Oliveira, M. W. J. van Passel, P. J. Schaap and L. H. de Graaff, Appl. Environ. Microbiol., 2010, 76, 4421–4429 CrossRef CAS PubMed
. - P. De Costa, P. Bezerra, I. Skaar and N. Lima, Fungicides : chemistry, environmental impact, and health effects, Nova Biomedical Books, New York, NY, USA, 2009 Search PubMed
. - M. C. Fisher, D. A. Henk, C. J. Briggs, J. S. Brownstein, L. C. Madoff, S. L. McCraw and S. J. Gurr, Nature, 2012, 484, 186–194 CrossRef CAS PubMed
. - J. Kelley, Identification and control of fungi in distribution systems, AWWA Research Foundation and American Water Works Association, Denver, CO, USA, 2003 Search PubMed
. - M. C. Arendrup, Clin. Microbiol. Infect., 2014, 8, 42–48 CrossRef PubMed
. - D. B. Archer, I. F. Connerton and D. A. MacKenzie, in Food Biotechnology, Springer Berlin Heidelberg, Berlin, Heidelberg, 2008, vol. 111, pp. 99–147 Search PubMed
. - N. B. Valentine, J. H. Wahl, M. T. Kingsley and K. L. Wahl, Rapid Commun. Mass Spectrom., 2002, 16, 1352–1357 CrossRef CAS PubMed
. - A. D. Buskirk, J. M. Hettick, I. Chipinda, B. F. Law, P. D. Siegel, J. E. Slaven, B. J. Green and D. H. Beezhold, Anal. Biochem., 2011, 411, 122–128 CrossRef CAS PubMed
. - Y. Wang, P. Aisen and A. Casadevall, Infect. Immun., 1996, 64, 2420–2424 CAS
. - H. C. Eisenman and A. Casadevall, Appl. Microbiol. Biotechnol., 2012, 93, 931–940 CrossRef CAS PubMed
. - J. Chalupová, M. Raus, M. Sedlářová and M. Šebela, Biotechnol. Adv., 2014, 32, 230–241 CrossRef PubMed
. - D. S. Alviano, A. J. Franzen, L. R. Travassos, C. Holandino, S. Rozental, R. Ejzemberg, C. S. Alviano and M. L. Rodrigues, Infect. Immun., 2004, 72, 229–237 CrossRef CAS PubMed
. - T. R. Jørgensen, T. Goosen, C. A. M. J. J. van den Hondel, A. F. J. Ram and J. J. L. Iversen, BMC Genomics, 2009, 10, 44 CrossRef PubMed
. - D. I. Jacobs, M. M. A. Olsthoorn, I. Maillet, M. Akeroyd, S. Breestraat, S. Donkers, R. A. M. van der Hoeven, C. A. M. J. J. van den Hondel, R. Kooistra, T. Lapointe, H. Menke, R. Meulenberg, M. Misset, W. H. Müller, N. N. M. E. van Peij, A. Ram, S. Rodriguez, M. S. Roelofs, J. A. Roubos, M. W. E. M. van Tilborg, A. J. Verkleij, H. J. Pel, H. Stam and C. M. J. Sagt, Fungal Genet. Biol., 2009, 46(suppl 1), S141–S152 CrossRef CAS PubMed
. - T. L. Williams, D. Andrzejewski, J. O. Lay and S. M. Musser, J. Am. Soc. Mass Spectrom., 2003, 14, 342–351 CrossRef CAS PubMed
. - J. Gopal, M. Manikandan and H.-F. Wu, RSC Adv., 2014, 4, 10982–10989 RSC
. - M. M. L. Cunha, A. J. Franzen, D. S. Alviano, E. Zanardi, C. S. Alviano, W. De Souza and S. Rozental, Microsc. Res. Tech., 2005, 68, 377–384 CrossRef CAS PubMed
. - H. N. Abdelhamid and H.-F. Wu, Colloids Surf., B, 2013, 115, 51–60 CrossRef PubMed
. - G. Gedda, H. N. Abdelhamid, M. S. Khan and H.-F. Wu, RSC Adv., 2014, 4, 45973–45983 RSC
. - M. L. Bhaisare, H. N. Abdelhamid, B.-S. Wu and H.-F. Wu, J. Mater. Chem. B, 2014, 2, 4671–4683 RSC
.
Footnotes |
† Electronic supplementary information (ESI) available. See DOI: 10.1039/c7ra03741d |
‡ Authors share equal contribution. |
|
This journal is © The Royal Society of Chemistry 2017 |
Click here to see how this site uses Cookies. View our privacy policy here.