DOI:
10.1039/C7RA02730C
(Paper)
RSC Adv., 2017,
7, 20354-20359
H-Shape mesogenic dimers – the spacer parity effect†
Received
6th March 2017
, Accepted 31st March 2017
First published on 7th April 2017
Abstract
It was found that for H-shape dimers, the parity of the number of atoms in the spacer linking the mesogenic cores has important influence on the mesogenic properties only for the molecules with weak mesogenic core anisotropy. In the case of stronger core anisotropy, the parallel arrangement of mesogenic units is induced regardless of the spacer parity. As a result, phase transition temperatures and layer spacing are only weakly affected by spacer parity. By studying the properties of six new homologue series it was found that for H-shape dimers the orthogonal smectic (SmA) is strongly disfavoured: most compounds form a tilted smectic (SmC) phase, which is related to hindrances in molecular rotation for molecules with large breath to length ratio. For dimers with very long spacer, and thus decoupled mesogenic units, the smectic phase is replaced by a nematic phase, and for stiff polycatenar molecules with very short spacers the cubic phase can appear.
Introduction
Liquid crystalline dimers and oligomers, as compounds with intermediate structures between monomers and polymers, have always been an important class of mesogenic compounds. Their unique properties result from the additional degree of freedom due to the presence of flexible chain linking mesogenic cores.1–3 Dimers often exhibit phases rarely observed for monomers; they easily form modulated smectics,4 polar smectics5 and broken-layer type columnar phases;6 there is also a limited number of reports that dimers might exhibit cubic phases.7 As mesogenic cores the rod-like,2,3,8 disc-like9 or bent-shape10 units were applied in molecular design. For the most studied dimers, with end-to-end connected rod-like mesogenic units, it was found the number of atoms in spacer is of primary importance for the type of the phase formed as well as transition temperatures.2,3,11 The parity of spacer affects the overall molecular shape2 (Fig. 1), for even number of atoms in the spacer molecules adopt zigzag geometry, in which the mesogenic units are anti-parallel, whereas the spacer with odd number of atoms results in bent shape of the molecule, with the mesogenic units being inclined to each other.2,3 The interest in dimers having bent shape was strongly stimulated by recent discovery of twist-bend nematic (NTB) phase, the heliconical phase made by achiral molecules.8,12 Apart of dimers, in which two mesogenic cores are connected end-to-end also other molecular geometries were recently investigated, e.g. T-shape,13 U-shape14 or H-shape15 molecules (Fig. 1).
 |
| Fig. 1 Types of dimers and different conformations of molecules due to the odd–even effect. | |
Herein, we describe 6 new series of laterally linked, symmetric dimeric molecules (H-shape), built of rod-like mesogenic cores interconnected through flexible alkoxy spacers of different length (n = 1, 3–10) (Scheme 1).
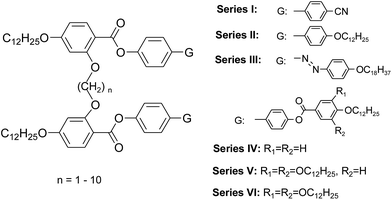 |
| Scheme 1 Molecular structures of the investigated compounds. | |
The rod-like units having different number of phenyl rings were used in order to study influence of geometrical anisotropy of mesogenic core on the phase properties. Further modification of molecular geometry was obtained by a variation of number and length of terminal chains. The phase transition temperatures for studied materials were collected inTables 1–6 and the phase sequences are presented in Fig. 2.
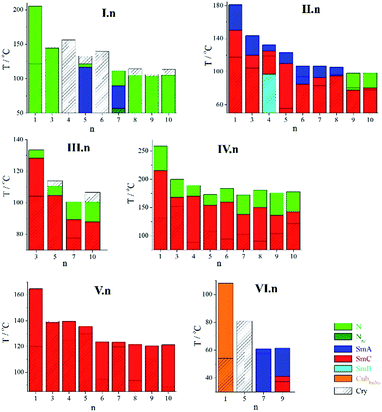 |
| Fig. 2 Phase diagrams for the studied homologue Series I–VI. | |
Results and discussion
Most of the compounds of Series I with simple cyanobiphenyl unit in the mesogenic cores exhibited liquid crystalline phases (Table 1, Fig. 2) except those with short even spacers (n = 4, 6). For longer homologues with even spacers, monotropic nematic phase was observed. Molecules with odd spacers, in general, had better mesogenic properties, they all formed nematic phase, and additionally for homologues n = 5 and 7 a partially bilayer smectic Ad phase was found, with the layer spacing d slightly larger than the estimated molecular length (d/L ∼ 1.1). Interestingly, compound I.7 exhibited rare, for dimers, phase sequence with re-entrant nematic phase (Nre) formed below the smectic Ad phase. The N–SmAd–Nre phase sequence has been confirmed by XRD measurements, the transitions between smectic and nematic phases were signified by the broadening of the diffraction signal related to the lamellar structure, due to the loss of long-range positional order (Fig. 3). The half-width of XRD signal measured in both nematic phases was similar (Fig. 3), indicating comparable correlation length of positional order in N and Nre phases.
Table 1 Series I phase transitions temperatures (in °C) and in parenthesis transition enthalpies (in kJ mol−1)
I.n |
mp |
Nre |
|
SmA |
|
N |
|
Iso |
I.1 |
121.7 (66.4) |
|
|
|
|
● |
205.2 (2.1) |
● |
I.3 |
145.6 (79.8) |
|
|
|
|
● |
144.4 (2.0) |
● |
I.4 |
156.4 (108.4) |
|
|
|
|
|
|
● |
I.5 |
132.8 (83.4) |
|
|
● |
116.6 (0.06) |
● |
121.8 (1.9) |
● |
I.6 |
139.8 (41.0) |
|
|
|
|
|
|
● |
I.7 |
87.9 (78.5) |
● |
56.7 (0.1) |
● |
89.8 (0.03) |
● |
110.8 (0.1) |
● |
I.8 |
114.4 (58.1) |
|
|
|
|
● |
104.5 (0.7) |
● |
I.9 |
106.2 (80.6) |
|
|
|
|
● |
103.6 (0.9) |
● |
I.10 |
113.8 (81.3) |
|
|
|
|
● |
105.0 (0.7) |
● |
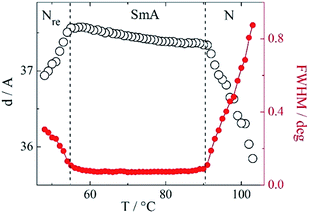 |
| Fig. 3 Smectic layer spacing and width of corresponding diffraction signal vs. temperature for compound I.7. | |
The phase properties of homologues of the Series II, having the dodecyloxy chains instead of CN group, attached to the biphenyl units are collected in Table 2.
Table 2 Series II – phase transitions temperatures (in °C) and in parenthesis transition enthalpies (in kJ mol−1)
II.n |
mp |
SmB |
|
SmC |
|
SmA |
|
N |
|
Iso |
From microscopic observations. |
II.1 |
117.6 (37.5) |
|
|
● |
150a |
● |
181.0 (14.8) |
|
|
● |
II.3 |
104.3 (77.2) |
|
|
● |
120 m |
● |
143.7 (16.8) |
|
|
● |
II.4 |
118.9 (111.7) |
● |
96.7 (14.1) |
● |
125 m |
● |
132.6 (16.6) |
|
|
● |
II.5 |
122.0 (49.6) |
|
|
● |
114.5 (0.1) |
● |
124.6 (15.2) |
|
|
● |
II.6 |
94.2 (74.3) |
|
|
● |
85 m |
● |
106.9 (4.0) |
|
|
● |
II.7 |
83.1 (79.6) |
|
|
● |
93 m |
● |
106.6 (9.4) |
|
|
● |
II.8 |
96.6 (86.7) |
|
|
● |
95 m |
● |
105.6 (8.6) |
|
|
● |
II.9 |
80.3 (35.8) |
|
|
● |
77.6 (0.1) |
|
|
● |
98.3 (3.6) |
● |
II.10 |
78.3 (56.0) |
|
|
● |
80.4 (0.4) |
|
|
● |
98.4 (3.4) |
● |
Compounds II.1–II.8 exhibited the SmA–SmC phase sequence on cooling, while for the longest spacer homologues, II.9–II.10, a SmA phase was replaced by nematic phase. The richest polymorphism was found for even spacer compound II.4, which formed additional orthogonal smectic B phase below the SmC, however monotropic character of the phase prevented its detailed characterization. The temperature dependence of smectic layer thickness evaluated from XRD experiments confirmed continuous character of the SmA–SmC phase transition (Fig. 4) for all the homologues. Clear odd–even effect regarding the layer spacing in smectic phase was observed (Fig. 4), even spacer homologues showed slightly larger d values.
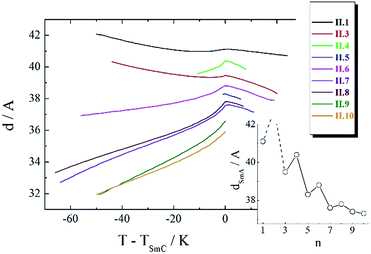 |
| Fig. 4 Smectic layer spacing vs. temperature for homologues II.n. In the inset the layer spacing in SmA phase at the transition to SmC phase vs. spacer length, n. | |
For all the homologues the layer spacing was found to be much smaller than molecular length (estimated to be ∼60 A for even spacer and ∼50 A for odd spacer homologues) showing strong interdigitation of terminal chains between neighbouring layers. In SmA phase the layer expansion is negative and similar for all materials, while the thermal expansion coefficient in SmC phase changes its sign upon elongation of the spacer, on heating, far from SmA phase transition temperature, the contraction of the layers was found for compounds II.1 and II.3, and for longer homologues layer thickness monotonically increased in whole temperature range (Fig. 4). The effect might be explained by different degree of terminal chain interdigitation between layers, that is more significant for molecules with longer spacer between mesogenic cores.
For compounds of Series III, the azo group was introduced into mesogenic core, such elongation of mesogenic core resulted in destabilization of the SmA phase on behave of nematic phases, the phase sequence with N and SmC phase was observed for all homologues (Table 3). Due to the presence of azo group the materials were photosensitive, absorption of UV light caused the trans–cis isomerization of azo unit (see ESI†) that disrupted the mesogenic properties.
Table 3 Series III – phase transitions temperatures (in °C) and in parenthesis transition enthalpies (in kJ mol−1)
III.n |
mp |
SmC |
|
N |
|
Iso |
III.3 |
104.3 (89.3) |
● |
128.3 (0.3) |
● |
133.5 (15.5) |
● |
III.5 |
114 (161.4) |
● |
104.7 (1.0) |
● |
110.6 (7.9) |
● |
III.7 |
77.6 (105.5) |
● |
89.5 (0.3) |
● |
100.6 (1.6) |
● |
III.10 |
106.7 (139.0) |
● |
87.9 (1.8) |
● |
100.6 (4.8) |
● |
Elongation of the rigid mesogenic cores by additional phenyl ring attached to biphenyl unit through the ester linkage (Series IV) caused strong increase of liquid crystalline phases stability, the clearing temperatures for compounds IV.n were higher by more than 50 K comparing to their II.n analogues.
All the compounds of Series IV exhibited similar LC polymorphism, smectic C and nematic phases were observed (Table 4). Contrary to Series II, for the homologues of Series IV clear odd–even effect was found regarding the phase transition temperatures, the effect is clearly visible for intermediate homologues (Fig. 2), interestingly changing the parity of the linkage chain has opposite influence on the melting and clearing temperatures – the clearing temperature of even spacer homologues was higher while melting temperature lower than for odd spacer compounds.
Table 4 Series IV phase transitions temperatures (in °C) and in parenthesis transition enthalpies (in kJ mol−1)
IV.n |
mp |
SmC |
|
N |
|
Iso |
IV.1 |
132.5 (59.3) |
● |
215.2 (4.2) |
● |
258.6 (4.0) |
● |
IV.3 |
152.2 (75.2) |
● |
167.8 (2.9) |
● |
199.6 (4.6) |
● |
IV.4 |
88.3 (17.9) |
● |
170.1 (6.1) |
● |
188.8 (5.1) |
● |
IV.5 |
108.4 (24.9) |
● |
154.3 (5.0) |
● |
173.0 (2.9) |
● |
IV.6 |
94.3 (21.0) |
● |
159.8 (5.2) |
● |
183.4 (3.4) |
● |
IV.7 |
102.7 (25.1) |
● |
137.8 (5.1) |
● |
172.1 (3.3) |
● |
IV.8 |
90.4 (54.6) |
● |
150.3 (3.5) |
● |
180.3 (3.0) |
● |
IV.9 |
103.6 (38.3) |
● |
136.0 (3.7) |
● |
175.4 (3.5) |
● |
IV.10 |
122.1 (84.8) |
● |
142.5 (3.3) |
● |
178.0 (2.9) |
● |
Table 5 Series V phase transitions temperatures (in °C) and in parenthesis transition enthalpies (in kJ mol−1)
V.n |
mp |
SmC |
|
Iso |
V.1 |
120.2 (69.2) |
● |
164.9 (8.3) |
● |
V.3 |
140.0 (69.2) |
● |
138.8 (24.6) |
● |
V.4 |
125.0 (14.7) |
● |
139.6 (22.3) |
● |
V.5 |
130.1 (6.6) |
● |
135.6 (15.3) |
● |
V.6 |
95.0 (52.4) |
● |
123.7 (20.0) |
● |
V.7 |
119.8 (52.0) |
● |
123.3 (21.6) |
● |
V.8 |
93.7 (41.1) |
● |
121.7 (14.7) |
● |
V.9 |
110.3 (37.5) |
● |
120.5 (20.5) |
● |
V.10 |
88.9 (34.9) |
● |
121.4 (21.0) |
● |
Table 6 Series VI phase transitions temperatures (in °C) and in parenthesis heat transition enthalpies (in kJ mol−1)
VI.n |
mp |
SmC |
|
SmA |
|
CubIm3m |
|
Iso |
VI.1 |
54.1 (80.8) |
|
|
|
|
● |
108.0 (3.9) |
● |
VI.5 |
80.9 (13.2) |
|
|
|
|
|
|
● |
VI.7 |
57.6 (52.9) |
|
|
● |
61.0 (2.1) |
|
|
● |
VI.9 |
37.4 (94.8) |
● |
41.1 (1.6) |
● |
61.4 (3.1) |
|
|
● |
Further modification of the molecular architecture by attaching additional terminal dodecyloxy chains (one in Series V and two in Series VI) to each mesogenic core resulted in destabilization of LC phases; lowering of the clearing temperature was the strongest in case of molecules with three dodecyloxy chains. Compounds of Series V showed exclusively tilted smectic C phase, with clearing temperatures lower by 40–90 K in comparison to analogue compounds of Series IV; while an orthogonal smectic A phase was observed for two homologues of Series VI with long spacers n = 7 and 9.
For the compound VI.1, with three terminal chains and the shortest linkage, another type of LC phase was found – instead of lamellar phases, a cubic LC phase was formed. The phase was optically isotropic, while the XRD experiments indicated presence of long-range positional order of molecules. The XRD patterns showed series of sharp diffraction signals at low angle range and a diffused signal at the high angle range proving the liquid crystalline character of the phase (Fig. 5). The best fitting of the low-angle part of the diffractogram was obtained assuming 3D crystallographic lattice of Im3m symmetry and lattice parameter a = 176.8 A (at T = 50 °C), corresponding roughly to 3 molecular lengths. Assuming the density of the cubic phase 1 g cm−3, the number of dimeric molecules in the unit cell was estimated to be as high as 1440.
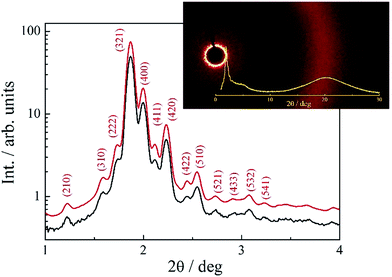 |
| Fig. 5 Low angle X-ray diffractogram (black line) registered for compound VI.1 at 50 °C. The fitting curve (red line) was obtained assuming crystallographic lattice with Im3m symmetry and unit cell parameter a = 176.8 A. In the inset 2D XRD pattern recorded in broad diffraction angle range; diffused high angle signal evidences liquid crystalline character of the phase. | |
Experimental
Synthetic procedures
Detailed synthetic procedures are shown in Scheme 2. Series I–VI were prepared by condensation of two parts: rod-like ligands providing liquid crystalline properties of final compounds, and H-shaped dimeric units with an alkyl spacer providing odd–even behavior. Series I was prepared with commercially available 4-cyanobiphenol (9). The 4,4′-biphenol (1) was used as a main rigid fragment of rod-like compounds. The one exemption was 4-hydroxy-4′(4-octadecyloxy)diazobenzene (12) prepared in 3-steps: alkylation of 4-nitrophenol, reduction of nitro group to amine group (11), and final azo coupling with phenol (12). All alkoxy derivatives were obtained via Williamson reaction, carried out in anhydrous DMF in presence of KI and K2CO3 (a). All ester derivatives were prepared by esterification reaction in anhydrous THF in presence of TEA and DMAP (b). The compounds were purified by recrystallization from MeOH, toluene, hexane or by column chromatography using CH2Cl2, toluene, and mixture of MeOH/CHCl3 as eluents. All H-shaped units were prepared via alkylation reaction of ethyl 4-dodecyloxy-2-hydroxybenzoate (13) with appropriate dihalogenic alkanes. Hydrolysis and further acylation reaction allowed receiving H-shaped acidic chlorides (16/n) common for all Series I–VI and used in final esterification reaction with characteristic rod-like ligands. For more details see ESI.†
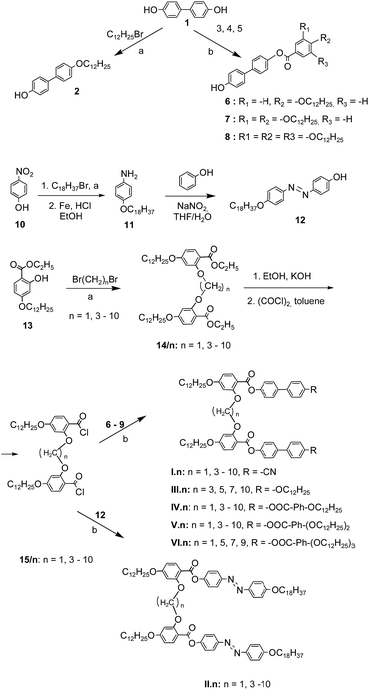 |
| Scheme 2 Synthesis of the H-dimers; (a): K2CO3, KI, DMF, (b): DMAP, TEA, THF. | |
Materials and methods
Temperatures and thermal effects of the phase transitions were determined by calorimetric studies using TA Q200 calorimeter, samples of mass 1–3 mg were sealed in aluminum pans and kept in nitrogen atmosphere during measurement. Both heating and cooling scans with rate 10 K min−1 were applied. The optical studies were performed using Zeiss Imager A2m polarizing microscope equipped with Linkam heating stage. The small angle X-ray diffraction patterns were obtained with Bruker Nanostar system (CuKα radiation, three pinhole collimation system, area detector VANTEC 2000, MRI TCP-U heating stage), while wide angle diffraction experiments were performed with Bruker GADDS diffractometer (CuKα radiation, point collimator, Vantec 2000 area detector, modified Linkam heating stage). Samples were prepared in a form of droplet on heated surface, their temperature was controlled with precision 0.1 K. For UV-vis experiment the Shimadzu UV-3101PC spectrometer was used and the conformational changes were induced upon illumination with UV Hamamatsu lamp.
Conclusions
It was expected that the mesogenic properties of H-shape dimers should depend on the length of the spacer. Indeed for all homologue series the clearing temperature steeply decreases with elongation of the spacer, showing that less stiff molecular structure disfavours mesophase formation. Moreover, it was also observed that for longer spacers the smectic A phase is replaced by nematic phase (Series II), showing that for decoupled mesogenic cores formation of smectic layers is more difficult. Also the spacer parity should affect mesogenic properties, as for odd and even number of atoms in the linkage the molecular geometry is expected to be much different (Fig. 6).
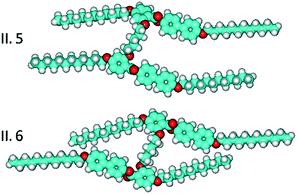 |
| Fig. 6 Molecular structure of odd (II.5) and even (II.6) spacer homologues of Series II, with parallel and antiparallel orientation of mesogenic cores. | |
However, only for Series I, with molecules having weak core anisotropy, the odd–even effect was pronounced; all odd spacer homologues exhibited LC phases in broad temperature range, while short, even spacer homologues were not mesogenic; apparently the molecules with mesogenic units in antiparallel and laterally shifted configuration disfavoured formation of LC phases. For other studied homologue series the odd–even effect was less pronounced. For compounds of Series IV the influence of spacer parity was opposite to this observed in Series I, the even spacer homologues had slightly higher clearing temperatures and thus larger range of LC phases. Since the odd–even effect is weak one can conclude that for molecules with cores of larger anisotropy the geometry of even and odd spacer dimers is not much different, in both cases probably nearly parallel arrangement of mesogenic units is obtained, for even spacers this requires gauche conformations of alkyl linkage. Similar molecular geometry of odd and even spacer homologues is also consistent with only small differences of the smectic layer thickness of consecutive homologues of Series II and IV. Clear, but small parity effect has been observed only for Series II – even spacer dimers have slightly higher layer spacing.
Regarding the phase situation, for all homologue series it was observed that tilted smectic (SmC) phase is more favourable than the orthogonal one (SmA). The formation of uniaxial SmA phase requires that molecules rotate freely along their long molecular axis, and such rotation for molecules with large breath to length ratio, might be energetically disfavourable. For molecules with hinderent rotation the packing into the biaxial structure of SmC phase seems to be more favourable.
For the most stiff, polycatenar dimer VI.1 with three terminal chains, instead of lamellar phase a cubic phase was observed, indicating that efficient packing of molecules required curving of the interface between areas occupied by aromatic cores and alkyl tails, which is realized by formation of tri-continuous type cubic Im3m structure.
Acknowledgements
The studies were supported by Polish National Science Centre, grant no. 2011/01/N/ST5/03314.
Notes and references
- C. T. Imrie andG. R. Luckhurst,Handbook of Liquid Crystals,Wiley-VCH,Weinheim, 2014,vol. 7,pp. 137–210, and references cited therein Search PubMed.
- C. T. Imrie and P. A. Henderson, Chem. Soc. Rev., 2007, 36, 2096 RSC.
- P. A. Henderson and C. T. Imrie, Liq. Cryst., 2011, 38, 1407 CrossRef CAS.
- D. Chen, M. Nakata, R. Shao, M. R. Tuchband, M. Shuai, U. Baumeister, W. Weissflog, D. M. Walba, M. A. Glaser, J. E. Maclennan and N. A. Clark, Phys. Rev. E: Stat., Nonlinear, Soft Matter Phys., 2014, 89, 022506 CrossRef CAS PubMed; D. Pociecha, D. Kardas, E. Gorecka, J. Szydlowska, J. Mieczkowski and D. Guillon, J. Mater. Chem., 2003, 13, 34 RSC.
- Y. Shimbo, E. Gorecka, D. Pociecha, F. Araoka, M. Goto, Y. Takanishi, K. Ishikawa, J. Mieczkowski, K. Gomola and H. Takezoe, Phys. Rev. Lett., 2006, 97, 113901 CrossRef CAS PubMed; J. Mieczkowski, K. Gomola, J. Koseska, D. Pociecha, J. Szydlowska and E. Gorecka, J. Mater. Chem., 2003, 13, 2132 RSC; H. N. S. Murthy, M. Bodyagin, S. Diele, U. Baumeister, G. Pelzl and W. Weissflog, J. Mater. Chem., 2006, 16, 1634 RSC.
- Y. Takanishi, M. Toshimitsu, M. Nakata, N. Takada, T. Izumi, K. Ishikawa, H. Takezoe, J. Watanabe, Y. Takahashi and A. Iida, Phys. Rev. E: Stat., Nonlinear, Soft Matter Phys., 2006, 74, 051703 CrossRef CAS PubMed; T. Izumi, Y. Naitou, Y. Shimbo, Y. Takanishi, H. Takezoe and J. Watanabe, J. Phys. Chem. B, 2006, 110, 23911 CrossRef PubMed.
- B.-K. Cho, J.-H. Ryu, W.-C. Zin and M. Lee, Polym. Bull., 2000, 44, 393 CrossRef CAS; T. Kajitani, S. Kohmoto, M. Yamamoto and K. Kishikawa, Chem. Mater., 2005, 17, 3812 CrossRef; J. M. Wolska, D. Pociecha, J. Mieczkowski and E. Gorecka, Liq. Cryst., 2016, 43, 235 CrossRef.
- M. G. Tamba, S. M. Salili, C. Zhang, A. Jakli, G. H. Mehl, R. Stannarius and A. Eremin, RSC Adv., 2015, 5, 11207 RSC; M. Cestari, S. Diez-Berart, D. A. Dunmur, A. Ferrarini, M. R. de la Fuente, D. J. B. Jackson, D. O. Lopez, G. R. Luckhurst, M. A. Perez-Jubindo, R. M. Richardson, J. Salud, B. A. Timimi and H. Zimmermann, Phys. Rev. E: Stat., Nonlinear, Soft Matter Phys., 2011, 84, 031704 CrossRef CAS PubMed; V. Borshch, Y.-K. Kim, J. Xiang, M. Gao, A. Jakli, V. P. Panov, J. K. Vij, C. T. Imrie, M. G. Tamba, G. H. Mehl and O. D. Lavrentovich, Nat. Commun., 2013, 4, 2635 Search PubMed.
- T. Sakurai, K. Tashiro, Y. Honsho, A. Saeki, S. Seki, A. Osuka, A. Muranaka, M. Uchiyama, J. Kim, S. Ha, K. Kato, M. Takata and T. Aida, J. Am. Chem. Soc., 2011, 133, 6537 CrossRef CAS PubMed; J. Vergara, J. Barbera, J. L. Serrano, M. B. Ros, N. Sebastian, R. de la Fuente, D. O. Lopez, G. Fernandez, L. Sanchez and N. Martin, Angew. Chem., Int. Ed., 2011, 50, 12523 CrossRef PubMed.
- N. Sebastián, N. Gimeno, J. Vergara, D. O. Lopez, J. L. Serrano, C. L. Folcia, M. R. de la Fuente and M. B. Ros, J. Mater. Chem. C, 2014, 2, 4027 RSC; C. M. Hegguilustoy, M. B. Darda, R. S. Montani, B. Heinrich, B. Donnio, D. Guillon and R. O. Garay, Liq. Cryst., 2015, 42, 1013 CrossRef CAS; M. Horcic, J. Svoboda, V. Novotna, D. Pociecha and E. Gorecka, Chem. Commun., 2017, 53, 2721 RSC.
- R. W. Date, C. T. Imrie, G. R. Luckhurst and J. M. Seddon, Liq. Cryst., 1992, 12, 203 CrossRef CAS.
- D. Chen, J. H. Porada, J. B. Hooper, A. Klittnick, Y. Shen, M. R. Tuchband, E. Korblova, D. Bedrov, D. M. Walba, M. A. Glaser, J. E. Maclennan and N. A. Clark, Proc. Natl. Acad. Sci. U. S. A., 2013, 110, 15931 CrossRef CAS PubMed.
- A. Yoshizawa, M. Sato and J. Rokunohe, J. Mater. Chem., 2005, 15, 3285 RSC.
- G. S. Attard and A. G. Douglass, Liq. Cryst., 1997, 22, 349 CrossRef CAS.
- W. Weissflog, D. Demus, S. Diele, P. Nitschke and W. Wedler, Liq. Cryst., 1989, 5, 111 CrossRef CAS; S.-M. Huh and J.-I. Jin, Liq. Cryst., 1998, 25, 285 CrossRef; W. Wedler, D. Demus, H. Zaschke, K. Mohr, W. Schafer and W. Weissflog, J. Mater. Chem., 1991, 1, 347 RSC; W.-S. Bae, J.-W. Lee and J.-I. Jin, Liq. Cryst., 2001, 28, 59 CrossRef; J. Andersch, C. Tschierske, S. Diele and D. Lose, J. Mater. Chem., 1996, 6, 1297 RSC; B. Kumar, A. K. Prajapati, M. C. Varia and K. A. Suresh, Langmuir, 2009, 25, 839 CrossRef PubMed; Y. Zhang, J. Martinez-Perdiguero, U. Baumeister, C. Walker, J. Etxebarria, M. Prehm, J. Ortega, C. Tschierske, M. J. O'Callaghan, A. Harant and M. Handschy, J. Am. Chem. Soc., 2009, 131, 18386 CrossRef PubMed; A. K. Prajapati, M. C. Varia and S. P. Sahoo, Liq. Cryst., 2011, 38, 861 CrossRef; M. Kolpaczynska, K. Madrak, J. Mieczkowski, E. Górecka and D. Pociecha, Liq. Cryst., 2011, 38, 149 CrossRef; T. Sakurai, K. Tashiro, Y. Honsho, A. Saeki, S. Seki, A. Osuka, A. Muranaka, M. Uchiyama, J. Kim, S. Ha, K. Kato, M. Takata and T. Aida, J. Am. Chem. Soc., 2011, 133, 6537 CrossRef PubMed; J. Vergara, J. Barbera, J. L. Serrano, M. B. Ros, N. Sebastian, R. de la Fuente, D. O. Lopez, G. Fernandez, L. Sanchez and N. Martin, Angew. Chem., Int. Ed., 2011, 50, 12523 CrossRef PubMed.
Footnote |
† Electronic supplementary information (ESI) available: Chemical characterization of obtained materials and detailed description of experimental methods used for structure studies. See DOI:10.1039/c7ra02730c |
|
This journal is © The Royal Society of Chemistry 2017 |