DOI:
10.1039/C7RA02638B
(Paper)
RSC Adv., 2017,
7, 23515-23522
Combination of RuCl3·xH2O with PEG – a simple and recyclable catalytic system for direct arylation of heteroarenes via C–H bond activation†
Received
3rd March 2017
, Accepted 11th April 2017
First published on 28th April 2017
Abstract
A simple and recyclable catalytic system for direct arylation of heteroarenes via C–H bond activation was developed with a relatively inexpensive RuCl3·xH2O as a catalyst and PEG-400 as a green medium without any additive or ligand. This system not only showed excellent functional group compatibility, but also the ratio of mono- to diarylated product was easily regulated by varying the reaction conditions. Moreover, this transformation could proceed under air and be easily scaled up to gram-scale in a low catalyst loading of 0.3 mol%. Particularly, a good yield of 85% was obtained after this catalyst was recycled six times.
1. Introduction
In recent years, N-heteroaromatic scaffolds are becoming an increasing necessity, due to the structural motifs for a wide range applications in pharmaceuticals, natural products, functional materials1 and ligands for highly efficient and selective catalysis.2 Thus, there is an important need to develop an efficient synthetic methodology to make up the building blocks. Although transition metal catalyzed cross-coupling reaction of aryl halides with organometallic reagents to synthesize a series of heterocyclic compounds has matured to being a reliable tool,3 catalytic direct arylation via activation of C–H bond still garners tremendous interest due to obviating prefunctionalization,4,5 which addresses the issues on prolix steps of organic synthesis, formation of undesired side-product and use of auxiliary chemicals. Nevertheless, transition-metal-complexes catalyzed direct arylation via C–H activation still confronts various challenges. The preparation of complexes suffers from a tedious synthesis process and they are difficult to be recycled after reaction. For direct arylation in heterogeneous catalysis, polymer supported metal catalysts, such as PVP supported Pd-nanoparticle,6a Pd(II)@microporous polymers6b and heterogenization of homogeneous catalysts, such as polystyrene-supported palladium complex,6c polymer-supported pyridine-Pd,6d poly(ethyleneglycol)-bound SCS-Pd complexes6e and MOP-PPh3-Pd6f seem to be extremely effective ways to accomplish the separation and recycling of the catalysts. To date, the preparation of these catalysts is not easy and leaching of metals cannot be inhibited completely. In addition, NMP or toluene as solvent7 is highly toxic and is usually classified to be detrimental to the environment. To meet the requirements of green chemistry, some eco-friendly solvents such as CPME, 2-MeTHF and dialkyl carbonates have been chosen as the reaction media in direct arylation.8 Although water is a better alternative, the metal complex as a catalyst must be water-soluble and insensitive to water.9
Polyethylene glycol has been the perfect reaction media, which offers a suitable strategy to meet the requirement of green chemistry.10 To date, several successful examples have been reported using PEG as reaction medium, such as direct alkynylation of heteroarene,10a one-pot synthesis of propargylamines, aminoindolizine, 2,4,6-triaryl-pyridines, piperidines, and 3,4-dihydro-pyrimidinones,10b–f oxidative coupling of 2-arylbenzimidazoles with alkynes10g and hydroxylation of 2-arylpyridine.10h However, ruthenium catalyzed direct arylation in PEG, which was reported by Ackermann et al.11a and Hiebel et al.,11b under nitrogen atmosphere gave the arylated products with a moderate yield but in air it was inactive.11a In addition, most of the catalytic systems for direct arylation are required to be performed in oxygen-free conditions.12 Hence, to develop a simple and sustainable method was highly desirable with green chemistry into consideration. Herein, in continuation to our earlier studies on developing improved methodologies for direct arylation,16 we propose a simple and recyclable catalytic system for direct arylation of heteroarenes by employing RuCl3·xH2O as the catalyst, inexpensive aryl chlorides as the arylating reagents and PEG-400 as the “green” reaction medium without any additives or ligands. The strategy that was accomplished in air gave a satisfactory yield and a relatively high selectivity. Particularly, the ratio of mono- to diarylated product was easily regulated by varying the reaction conditions.
2. Results and discussion
2.1 Effect of the reaction conditions
In initial investigation, the model reaction of 2-phenylpyridine 1a (1 mmol) with coupling partner 1-chloro-3-fluorobenzene 2a (2.3 equiv.) was conducted in 1 mL PEG-400 to determine the optimum conditions. First, effect of various bases on this catalytic system was tested without any additive or ligand (Table 1). In the beginning, CsF, K3PO4, K2CO3, NaHCO3, Na2SO3 and EtONa were substantially less effective on the transformation (Table 1, entries 1–6). Interestingly, when CH3COOK was applied in the system under similar conditions, an excellent yield of 94% for target products 3aa and 4aa, and relatively high selectivity towards monoarylation were obtained in 1 h (Table 1, entry 7-II). However, prolonging the reaction time resulted in a decrease of monoarylated product 3aa (Table 1, entries 7-IV and 7-VI), which was mainly attributed to the formation of dehydrogenative cross-coupling products 5aa, 6aa, 7aa. Interestingly, this system also afforded the target products 3aa and 4aa in the yield of 82% even if the reaction time was shortened to 15 min (Table 1, entry 7-I). To the best of our knowledge, the activity of this transformation was unprecedented. Among the reported cases,7 to obtain a satisfactory yield for direct arylation, the reaction time was at least 60 minutes,7a usually 20–40 hours7b–e under similar conditions. The ratio of monoarylation to diarylation was further improved to 5.3/1 when 1a/2a was adjusted to 1.2/1 (see ESI†). Compared with CH3COOK, CH3COONa as a base also displayed similar activity and selectivity (Table 1, entry 8). Furthermore, it was notable that LiOH·H2O (3 equiv.) afforded a high yield of 98% and proved highly efficient to form diarylated product 4aa (Table 1, entries 9-II and 9-III). Apart from LiOH·H2O, the introduction of KOH, NaOH, CsOH, Li2CO3, and t-BuOLi resulted in discontented yields (Table 1, entries 10–14). Considering the promotional effect of Li+ and CH3COO− on the reaction, we were motivated to probe the role of CH3COOLi in the reaction. However, it gave the target products with a moderate yield of 50% (Table 1, entry 15). NEt3 was also a good base in this transformation, but it was unfavorable to improve the selectivity towards monoarylated or diarylated product (Table 1, entry 16). In the absence of a base, almost no target products were obtained, and only oligomerization products 8, 9 and 10 were observed in this process, highlighting the importance of a base for the direct arylation process (Table 1, entry 17) (Fig. 1). Based on the above findings, it was clearly indicated that the system took advantage of high reactivity and easily regulated the ratio of diarylated to monoarylated product. The diarylation was facilitated using 5 mol% RuCl3·xH2O as a catalyst and 3 equiv. LiOH·H2O as a base at 120 °C for 24 h. Intriguingly, the system strongly favoured the monoarylation just by switching the base to CH3COOK and shortened the reaction time to 1 h. In this reaction process, the hydroxyl group of PEG-400 played the role of a reductant for the precatalyst RuCl3·xH2O.13
Table 1 Effect of reaction conditions on arylation of 2-phenylpyridine with 1-chloro-3-fluorobenzenea
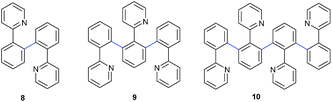 |
| Fig. 1 The oligomerizations of 2-phenylpyridine. | |
The further solvent screening indicated that target products could not be generated in toluene or water (Table 1, entry 18-I). It was found that a moderate yield of 57% was also obtained in ethylene glycol, but the direct arylation proved to be ineffective in MeOH as its low boiling point led to a low reaction temperature (Table 1, entries 18-I and 18-II). Compared to ethylene glycol, PEG was a better solvent. The weak interaction of PEG with ruthenium species might enhance the reactivity, analogous to the coordination of crown ether with ruthenium. In order to demonstrate it, 18-crown-6 was added to ethylene glycol, and it was found that this transformation was siginificantly improved (Table 1, entry 18-III). The above results suggested that the coordination of PEG with ruthenium, which was similar to the role of crown ether, promoted the performance of ruthenium in addition to the reduction role of hydroxyl group in PEG. Furthermore, the effect of reaction temperature indicated that a higher temperature of 150 °C resulted in more dehydrogenative cross-coupling products (DCCP) and a lower temperature of 90 °C caused a decrease of the catalytic activity (see ESI†). The catalytic performance of this simple system under optimum conditions was comparable to NHC-ruthenium(II) complexes,14 phosphine-[RuCl2(p-cymene)]2,15 [RuCl2(η6-benzene)MOTPP],16 and iridium–ruthenium bimetallic complexes bearing triazolediylidene.17
On taking catalyst loading into consideration, when the amount of ruthenium was decreased to 3 mol%, this system gave a satisfactory yield of 86% (Table 1, entry 7-III). Gratifying, we were surprised to find that this transformation could be easily scaled up to gram level with a comparable yield of 93% when S/C was extended to 300/1 for 4 h (Table 2).
Table 2 Effect of substrate to catalyst ratio on direct arylation of 2-phenylpyridine with 1-chloro-3-fluorobenzenea
Entry |
S/C |
Time (h) |
Yield [%] |
3aa/4aa [%] |
Reaction conditions: 2-phenylpyridine (n mmol), 3-fluoro-chloro-benzene (n + 0.3 mmol), PEG-400 as reaction medium (1 mL), RuCl3·xH2O (0.05 mmol), and CH3COOK (2 equiv.) and the reaction mixture was heated to 120 °C under air. The yield and ratio of mono- to diarylated product determined by HPLC. |
1 |
20 |
1 |
90 |
77 : 23 |
2 |
40 |
1 |
91 |
80 : 20 |
3 |
160 |
1 |
89 |
81 : 19 |
4 |
300 |
1 |
50 |
84 : 16 |
4 |
92 |
78 : 22 |
5 |
600 |
10 |
32 |
82 : 18 |
24 |
34 (40) |
80 : 20 |
2.2 The scope of the proposed direct arylation and recyclability of the catalyst
With optimum reaction conditions, we explored the scope of direct arylation protocol. It was found that this system was of wide substrate suitability. Moreover, diarylated derivatives of heteroarene were predominantly achieved by employing aryl chlorides as arylating reagents containing a variety of functional groups (Table 3). Overall, the reaction provided the desired products in high yields (77–99%). It was important to note that direct arylation of 2-phenylpyridine proceeded with high efficiency under an ambient atmosphere, highlighting the user-friendly nature of the proposed protocol. Chlorobenzene 2b was used as arylating reagent to give an impressive yield of 92% (Table 3, entry 1). Notably, all aryl chlorides with substituents derived from electron-rich groups (–OCH3, –CH3) as well as electron-deficient groups (–CF3, –F, –COCH3, –CN) were converted into the desired products with high yields under the reaction conditions (Table 3, entries 3, 4, 6, 7, 9, 10, 12–15 and 21). Those results clearly indicated that this system was insensitive to electronic factor. At the same time, heteroaryl chlorides were also surveyed to furnish the desired products with a comparably better yield (Table 3, entries 16–18). However, for aryl chlorides containing a substituent in ortho-position, they afforded arylated products with relatively low yields due to steric hindrance of ortho-substituent9e (Table 3, entries 2, 5 and 11). However, for 1-chloro-2-fluoro-benzene and 3-chloropyridine (Table 3, entries 8 and 19), only a trace amount of product was obtained. Furthermore, 4-chloronitrobenzene also gave a trace amount of target products in accordance with the literature9e (Table 3, entry 20). It was noteworthy that the use of (hetero)aryl chlorides bearing various electron-deficient or electron-rich groups resulted in relatively high selectivity towards diarylation in the presence of LiOH·H2O except for 2n, 2o, 2q, 2r. Even so, the relatively high yield of diarylated product was obtained with 3 equiv. CH3COOK as the base for 1 h (Table 3, entries 14, 15, 17 and 18). On using LiOH with CH3COOK (2 equiv.), high selectivity towards monoarylation was achieved (Table 3, entries 13, 15 and 18). To explore the versatility of this transformation, 1-phenylpyrazole and 2-phenyl-2-oxazoline were also surveyed to afford the desired products in moderate yields of 72% and 57%, respectively (Table 3, entries 22 and 23). Moreover, the results were comparable to catalytic systems generated from Rh(III) complex in the presence of Ag salts under nitrogen atmosphere5b and [Ru(O2CMes)2(p-cymene)] with p-TsCl5f for direct arylation of 1-phenylpyrazole and 2-phenyl-2-oxazoline. Moreover, 2-phenylpyridine derivatives bearing various substituents on the phenyl ring were well tolerated (Table 3, entries 24, 25, 27 and 28). Substrate 1f was almost completely converted to monoarylated product due to steric hindrance of the methyl group (Table 3, entry 26). The high selectivity towards monoarylation, being caused by the steric hindrance of methyl group, was similar to the reported results.7b,18
Table 3 Ruthenium-catalyzed ortho-arylation of heteroarenes with (hetero)aryl chloridesa
On the other hand, this system could be conveniently re-used (Table 4). A good yield of 85% was still obtained with decreased selectivity after the catalyst was recycled 6 times. To the best of our knowledge, this is the first successful example for arylation achieving both gram-scale preparation with a low catalyst loading of 0.3 mol% and maintaining its high activity after several recycles.
Table 4 Recyclability of the catalyst for direct arylation of 2-phenylpyridine with 1-chloro-3-fluorobenzenea
Entry |
Run |
Time [h] |
Yield [%] |
Ratio (3aa/4aa) |
Reaction conditions: 2-phenylpyridine (1 mmol), 3-fluoro-chloro-benzene (2.3 mmol), PEG-400 as reaction medium (1 mL), RuCl3·xH2O (5 mol-%), LiOH·H2O (3 equiv.), 120 °C, and under air. The ratio of mono- to diarylation product was determined by HPLC. Equivalent base needed to be added in every recycling. |
1 |
1 |
5 |
94 |
22 : 78 |
2 |
2 |
5 |
85 |
23 : 77 |
3 |
3 |
12 |
93 |
30 : 70 |
4 |
4 |
12 |
94 |
37 : 63 |
5 |
5 |
12 |
81 |
57 : 43 |
6 |
6 |
24 |
85 |
64 : 36 |
2.3 Mechanism of direct arylation
It was reported that catalytically active species were palladium nanoparticles for palladium-catalyzed direct arylation in PEG.11a In this case, the mercury poison experiment,19 being an efficient method to distinguish nanoparticle catalysis and homogeneous catalysis, showed that the activity of this catalytic system did not have any loss. The result proved that the catalytically active species were not ruthenium nanoparticles. Both experimental studies and density functional theory (DFT) calculations on ruthenium or palladium catalyzed direct arylation of heteroarene via C–H activation have proposed that the reaction progressed via path 1 or path 2 (Scheme 1).20–22 In both paths, formation of cyclometalated ruthenium intermediate C was a key step. The intermediate C reacted with chlorobenzene via oxidative addition/reductive elimination sequence to give target products or 2-arylpyridine through the similar ortho-cyclometallated process to afford dehydrogenative cross-coupling products. Therefore, path 1 and path 2 were competitive routes in this transformation. In the proposed catalytic system, apart from the desired products 3aa and 4aa, formation of by-product DCCP revealed that the reaction routes were in good agreement with the reported results.21
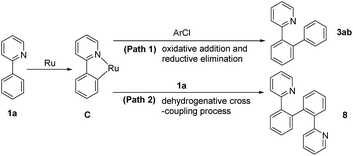 |
| Scheme 1 Two competitive paths for arylation of 2-phenylpyridine. | |
When CH3COOK was employed as the base, the reaction proceeded preferentially in path 1 (Table 1, entries 7-I and 7-II) and no DCCP was observed at the beginning of the reaction. If the reaction time was prolonged to 5 h, the expended monoarylated product 3aa was almost completely transferred to DCCP (Table 1, entry 7-IV). This possibly resulted from the decrease in the chlorobenzene concentration, which slowed its oxidative addition rate with cyclometalated ruthenium intermediate. Moreover, the accumulated 3aa would promoted the formation of DCCP. It was thought that extra 1-chloro-3-fluorobenzene (2.3 equiv.) was introduced into the system after the reaction began for 0.5 h; the yield of diarylated product 4aa was significantly improved and dehydrogenation crossing-coupling processes were greatly inhibited (Table 1, entry 7-V). The result indicated that high concentration chlorobenzene was beneficial to direct the arylated reaction in path 1. Based on concerted-metalation–deprotonation mechanism (CMD) with the assistance of carboxylate,22 a mechanism in the presence of potassium acetate was proposed in Scheme 2. First, Ru(III) species was reduced to Ru(II) by hydroxyl group in PEG-400. Moreover, the electron density of Ru was increased due to the weakly coordinated effect of PEG with Ru, which resulted in enhancing the reactivity, similar to the role of a ligand. The reaction began with the cyclometalation of 2-phenylpyridine 1a to form intermediate C through acetate-assisted dehydrogenation process. Subsequently, intermediate C reacted with chlorobenzene 2b involving an oxidative-addition process to generate intermediate D, which progressed by reduction elimination to give the monoarylated product 3ab and active ruthenium species A. When most of 2b was used up, intermediate C easily reacted with 3ab or 1a to afford DCCP. Monoarylated product 3ab could also undergo the similar process to give the corresponding diarylated product and DCCP.
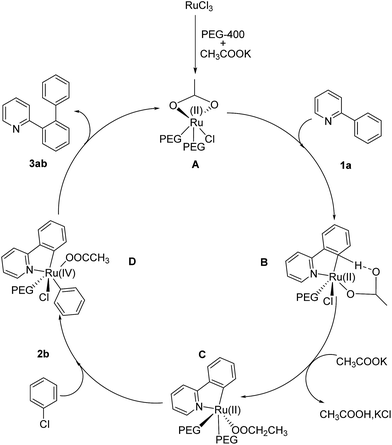 |
| Scheme 2 Proposed mechanism for ruthenium-catalyzed direct arylation of 2-phenylpyridine. | |
When the reaction was conducted with LiOH, the rate of this reaction was dramatically decreased, but this system gave a relatively high selectivity to diarylated product and the formation of DCCP was greatly inhibited. It was suggested that the coordination of pyridyl group with Li+ could slow the chelation-directed C–H activation process. Based on the same reason, it also blocked the dehydrogenation cross-coupling route owing to a need to activate a second 2-phenylpyridine C–H bond by a chelation-directed process, but it did not affect oxidative addition with chlorobenzene. Consequently, the system with LiOH was not only in favour of diarylation, but also greatly inhibited the formation of DCCP. To obtain some experimental evidence, PEG-400 (200 μL), 2-phenylpyridine (1 mmol) and Li+ (1 mmol) were added to a Schlenk tube and stirred under air at 120 °C for 17 h. Evidently, the coordination of the Li+ with 2-phenylpyridine was clearly observed in both 1H and 13C NMR spectra (Fig. 2 and 3). For other lithium salts, t-BuOLi as base also strongly favors the diarylation of 2-phenylpyridine, analogous to the promotional role of LiOH (Table 1, entry 10). However, Li2CO3 or CH3COOLi as base showed a different result with LiOH and t-BuOLi (Table 1, entries 11 and 15). The promotional mechanism of LiOH was not in agreement with the CMD mechanism. The reaction possibly proceeded by a chelation-directed C–H activation with OH− to abstract proton to generate a cyclometalated ruthenium intermediate. Next, the intermediate could react with chlorobenzene via oxidative addition/reductive elimination sequence to give the mono- and di-arylated product or a second 2-arylpyridine through the similar ortho-cyclometallated process to afford DCCP.
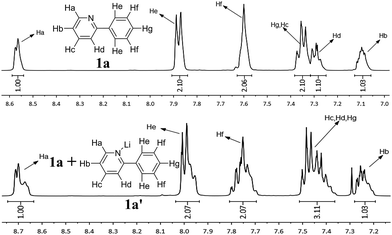 |
| Fig. 2 1H NMR spectra for 2-phenylpyridine 1a in PEG and the mixture of 2-phenylpyridine and Li salt 1a′ with 1a in PEG. | |
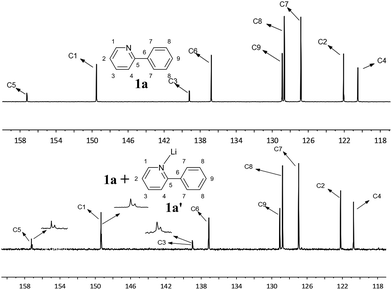 |
| Fig. 3 13C NMR spectra for 2-phenylpyridine 1a in PEG and the mixture of 2-phenylpyridine and Li salt 1a′ with 1a in PEG. | |
3. Conclusions
We developed a simple and recyclable catalytic system for direct arylation using RuCl3·xH2O as a precatalyst, PEG-400 as environmental benign medium and inexpensive aryl chlorides as electrophilic reagents. The reaction met the environment friendly requirements and the strategy was facile and did not require the introduction of any additive or ligand. This system showed high tolerance towards various functional groups. Particularly, selectivity towards mono- or diarylated product could be regulated by varying the reaction conditions. Furthermore, this transformation could be easily scaled up to the gram level with 0.3 mol% loading of ruthenium. A reasonable mechanism for this transformation was also proposed.
4. Experimental
4.1 Typical experimental procedure for direct arylation of 2-phenylpyridine under air
RuCl3·xH2O (104 mg, 0.5 mmol) and PEG-400 (10 mL) was taken in an oven-dried and clean round-bottom flask. The mixture was stirred at room temperature until RuCl3·xH2O was dispersed to PEG-400 completely. Furthermore, 2-phenylpyridine (1a, 1 mmol), 1-chloro-3-fluorobenzene (2a, 2.3 mmol), LiOH·H2O (126 mg, 3 mmol) and PEG-400 (1 mL) containing RuCl3·xH2O (0.05 mmol) were added to a Schlenk tube. The mixture was stirred at 120 °C under air for 24 h. After cooling to room temperature, the reaction mixture was diluted with 10 mL water. The aqueous layer was extracted with Et2O (20 mL) and EtOAc (30 mL). The combined organic phase was washed with H2O (20 mL) and brine (20 mL) and dried with Na2SO4 and concentrated under reduced pressure. The resulting residue was separated through a flash silica gel column chromatography (petroleum ether/EtOAc = 16
:
1) to obtain arylated products (306 mg, 95%). The ratio of mono- to diarylated product was determined by 1H NMR spectroscopy. The arylated products were separated using silica gel column chromatography (petroleum ether/CH2Cl2 = 1
:
16) to afford target products 3aa and 4aa, respectively. The product was characterized by 1H NMR and 13C NMR spectroscopy.
4.2 Recycling experimental procedures
In a reaction tube, 2-phenylpyridine (1a, 1 mmol), 1-chloro-3-fluorobenzene (2a, 2.3 mmol), LiOH·H2O (126 mg, 3 mmol) and PEG-400 (1 mL) containing RuCl3·xH2O (0.05 mmol) were stirred at 120 °C under air for 24 h. At the end of the reaction, reaction mixture was cooled to room temperature and extracted by employing Et2O (50 mL). Furthermore, 2-phenylpyridine (1a, 1 mmol), 1-chloro-3-fluorobenzene (2a, 2.3 mmol), LiOH·H2O (126 mg, 3 mmol) was added to PEG solution, the reaction and operation were repeated as mentioned above.
Acknowledgements
We gratefully acknowledge National Natural Science Foundation of China (No. 21572137).
Notes and references
-
(a) S. A. Bahashwan, A. A. Fayed, A. E.-G. E. Amr, E. M. Flefel and A. Kalmouch, Molecules, 2013, 18, 15051 CrossRef CAS PubMed;
(b) J. Alvarez-Builla, J. J. Vaquero and J. Barluenga, Mod. Heterocycl. Chem., 2011, 4, 1989 Search PubMed;
(c) A. Takfaoui, L. Zhao, R. Touzani, P. H. Dixneuf and H. Doucet, Tetrahedron Lett., 2014, 55, 1697 CrossRef CAS;
(d) M. Facompre, C. Tardy, C. Bal-Mahieu, P. Colson, C. Perez, I. Manzanares, C. Cuevas and C. Bailly, Cancer Res., 2003, 63, 7392 CAS;
(e) R. Wilcken, M. O. Zimmermann, A. Lange and A. C. Joerger, J. Med. Chem., 2013, 56, 1363 CrossRef CAS PubMed;
(f) Y. Segawa, K. Itami and T. Maekawa, Angew. Chem., Int. Ed., 2015, 54, 66 CrossRef CAS PubMed.
-
(a) J. C. Garrison and W. J. Youngs, Chem. Rev., 2005, 105, 3978 CrossRef CAS PubMed;
(b) O. Schuster, L. Yang, H. G. Raubenheimer and M. Albrecht, Chem. Rev., 2009, 109, 3445 CrossRef CAS PubMed;
(c) Z.-S. Gu, W.-X. Chen and L.-X. Shao, Eur. J. Org. Chem., 2014, 79, 5806 CrossRef CAS PubMed;
(d) J. J. Concepcion, M. K. Tsa, J. T. Muckerman and T. J. Meyer, J. Am. Chem. Soc., 2010, 132, 1545 CrossRef CAS PubMed.
-
(a) N. Marion and S. P. Nolan, Acc. Chem. Res., 2008, 41, 1440 CrossRef CAS PubMed;
(b) J. Hassan, M. Sevignon, C. Gozzi, E. Schulz and M. Lemaire, Chem. Rev., 2002, 102, 1359 CrossRef CAS PubMed;
(c) L. Ackermann, J. H. Spatz, C. J. Gschrei, R. Born and A. Althammer, Angew. Chem., Int. Ed., 2006, 118, 7789 CrossRef;
(d) L. Ackermann, R. Born, J. H. Spatz and D. Meyer, Angew. Chem., Int. Ed., 2005, 117, 7382 CrossRef.
- For recent reviews on direct arylation of heterocycles
(a) P. B. Arockiam, C. Bruneau and P. H. Dixneuf, Chem. Rev., 2012, 112, 5879 CrossRef CAS PubMed;
(b) C.-L. Sun, B.-J. Li and Z.-J. Shi, Chem. Rev., 2011, 111, 1293 CrossRef CAS PubMed;
(c) L. Ackermann, R. Vicente and A. R. Kapdi, Angew. Chem., Int. Ed., 2009, 48, 9792 CrossRef CAS PubMed;
(d) Y. Liang and S. F. Wnuk, Molecules, 2015, 20, 4874 CrossRef CAS PubMed;
(e) M. He, J.-F. Soulé and H. Doucet, ChemCatChem, 2014, 6, 1824 CrossRef CAS.
-
(a) L. Ackermann, J. Org. Chem., 2014, 79, 8948 CrossRef CAS PubMed;
(b) M.-Z. Lu, P. Lu, Y.-H. Xu and T.-P. Loh, Org. Lett., 2014, 16, 2614 CrossRef CAS PubMed;
(c) W. Hagui, N. Besbes, E. Srasra, J.-F. Soulé and H. Doucet, RSC Adv., 2016, 6, 17110 RSC;
(d) H. Xu, M. Shang, H.-X. Dai and J.-Q. Yu, Org. Lett., 2015, 17, 3830 CrossRef CAS PubMed;
(e) P.-X. Shen, X.-C. Wang, P. Wang, R.-Y. Zhu and J.-Q. Yu, J. Am. Chem. Soc., 2015, 137, 11574 CrossRef CAS PubMed;
(f) L. Ackermann, J. Pospech and H. K. Potukuchi, Org. Lett., 2012, 14, 2146 CrossRef CAS PubMed.
-
(a) C. G. Baumann, S. D. Ornellas, J. P. Reeds, T. E. Storr, T. J. Williams and I. J. S. Fairlamb, Tetrahedron, 2014, 70, 6174 CrossRef CAS;
(b) T.-H. Park, A. J. Hickman, K. Koh, S. Martin, A. G. Wong-Foy, M. S. Sanford and A. J. Matzger, J. Am. Chem. Soc., 2011, 133, 20138 CrossRef CAS PubMed;
(c) C. P. Perumgani, S. P. Parvathaneni, B. Kodicherla, S. Keesara and M. R. Mandapati, Inorg. Chim. Acta, 2017, 455, 105 CrossRef CAS;
(d) L.-C. Lee, J. He, J.-Q. Yu and C. W. Jones, ACS Catal., 2016, 6, 5245 CrossRef CAS;
(e) D. E. Bergbreiter, P. L. Osburn and Y.-S. Liu, J. Am. Chem. Soc., 1999, 121, 9531 CrossRef CAS;
(f) F. Liu, C. Liu, W. Kong, C. Qi, A. Zheng and S. Dai, Green Chem., 2016, 18, 6536 RSC.
-
(a) F. Pozgan and P. H. Dixneuf, Adv. Synth. Catal., 2009, 351, 1737 CrossRef CAS;
(b) S. Oi, S. Fukita, N. Hirata, N. Watanuki, S. Miyano and Y. Inoue, Org. Lett., 2001, 3, 2579 CrossRef CAS PubMed;
(c) L. Ackermann, R. Vicente and A. Althammer, Org. Lett., 2008, 10, 2299 CrossRef CAS PubMed;
(d) B. Li, C. B. Bheeter, C. Darcel and P. H. Dixneuf, Top. Catal., 2014, 57, 833 CrossRef CAS;
(e) S. Rajkumar, S. Karthik and T. Gandhi, J. Org. Chem., 2015, 80, 5532 CrossRef CAS PubMed.
-
(a) C. Fischmeister and H. Doucet, Green Chem., 2011, 13, 741 RSC;
(b) A. Hfaiedh, K. Yuan, H. B. Ammar, B. B. Hassine, J.-F. Soulé and H. Doucet, ChemSusChem, 2015, 8, 1794 CrossRef CAS PubMed;
(c) P. Arockiam, V. Poirier, C. Fischmeister, C. Bruneau and P. H. Dixneuf, Green Chem., 2009, 11, 1871 RSC;
(d) W. Muramatsu, K. Nakanoa and C.-J. Li, Org. Biomol. Chem., 2014, 12, 2189 RSC.
-
(a) H. Gong, H. Zeng, F. Zhou and C.-J. Li, Angew. Chem., Int. Ed., 2015, 54, 5718 CrossRef CAS PubMed;
(b) Y.-B. Huang, M. Shen, X. Wang, P. Huang, R. Chen, Z.-J. Lin and R. Cao, J. Catal., 2016, 333, 1 CrossRef CAS;
(c) P. B. Arockiam, C. Fischmeister, C. Bruneau and P. H. Dixneuf, Angew. Chem., Int. Ed., 2010, 49, 6629 CrossRef CAS PubMed;
(d) Z. Xu, T. Yang, X. Lin, J. D. Elliott and F. Ren, Tetrahedron Lett., 2015, 56, 475 CrossRef CAS;
(e) P. B. Arockiam, C. Fischmeister, C. Bruneau and P. H. Dixneuf, Green Chem., 2013, 15, 67 RSC.
-
(a) G. C. Reddy, P. Balasubramanyam, N. Salvanna and B. Das, Eur. J. Org. Chem., 2012, 3, 471 CrossRef;
(b) Q. Zhang, J.-X. Chen, W.-X. Gao, J.-C. Ding and H.-Y. Wu, Appl. Organomet. Chem., 2010, 24, 809 CrossRef CAS;
(c) S. Mishra, B. Naskar and R. Ghosh, Tetrahedron Lett., 2012, 53, 5483 CrossRef CAS;
(d) N. M. Smith, C. L. Raston, C. B. Smith and A. N. Sobolev, Green Chem., 2007, 9, 1185 RSC;
(e) S. Verma, S. L. Jain and B. Sain, Beilstein J. Org. Chem., 2011, 7, 1334 CrossRef CAS PubMed;
(f) S. L. Jain, S. Singhal and B. Sain, Green Chem., 2007, 9, 740 RSC;
(g) N. Kavitha, G. Sukumar, V. P. Kumar, P. S. Mainkar and S. Chandrasekhar, Tetrahedron Lett., 2013, 54, 4198 CrossRef CAS;
(h) S. H. Kim, H. S. Lee, S. H. Kim and J. N. Kim, Tetrahedron Lett., 2008, 49, 5863 CrossRef CAS.
-
(a) L. Ackermann and R. Vicente, Org. Lett., 2009, 11, 4922 CrossRef CAS PubMed;
(b) M.-A. Hiebel, Y. Fall, M.-C. Scherrmann and S. Berteina-Raboin, Eur. J. Org. Chem., 2014, 21, 4643 CrossRef.
-
(a) Y. Yang, X. Qiu, Y. Zhao, Y. Mu and Z. Shi, J. Am. Chem. Soc., 2016, 138, 495 CrossRef CAS PubMed;
(b) Z. Liang, R. Feng, H. Yin and Y. Zhang, Org. Lett., 2013, 15, 4544 CrossRef CAS PubMed;
(c) B. N. Laforteza, K. S. L. Chan and J.-Q. Yu, Angew. Chem., Int. Ed., 2015, 54, 11143 CrossRef CAS PubMed;
(d) J. Hubrich, T. Himmler, L. Rodefeld and L. Ackermann, Adv. Synth. Catal., 2015, 357, 474 CrossRef CAS.
- W. Li, Y. Guo and P. Zhang, J. Phys. Chem. C, 2010, 114, 6413 CAS.
- I. Ozdemir, S. Demir, B. Cetinkaya, C. Gourlaouen, F. Maseras, C. Bruneau and P. H. Dixneuf, J. Am. Chem. Soc., 2008, 130, 1156 CrossRef PubMed.
- L. Ackermann, Org. Lett., 2005, 7, 3123 CrossRef CAS PubMed.
- J. Zhang, Q. Yang, Z. Zhu, M. L. Yuan, H. Y. Fu, X. L. Zheng, H. Chen and R. X. Li, Eur. J. Org. Chem., 2012, 34, 6702 CrossRef.
- S. Sabater, J. A. Mata and E. Peris, Organometallics, 2012, 31, 6450 CrossRef CAS.
-
(a) D. Kalyani, N. R. Deprez, L. V. Desai and M. S. Sanford, J. Am. Chem. Soc., 2005, 127, 7330 CrossRef CAS PubMed;
(b) L. Ackermann, R. Vicente, H. K. Potukuchi and V. Pirovano, Org. Lett., 2010, 12, 5032 CrossRef CAS PubMed;
(c) D. Zell, S. Warratz, D. Gelman, S. J. Garden and L. Ackermann, Chem.–Eur. J., 2016, 22, 1248 CrossRef CAS PubMed.
- L. Zhang, L. Wang, X.-Y. Ma, R.-X. Li and X.-J. Li, Catal. Commun., 2007, 8, 2238 CrossRef CAS.
-
(a) L. Ackermann, N. Hofmann and R. Vicente, Org. Lett., 2011, 13, 1875 CrossRef CAS PubMed;
(b) E. Diers, N. Y. P. Kumar, T. Mejuch, I. Marek and L. Ackermann, Tetrahedron, 2013, 69, 4445 CrossRef CAS.
-
(a) K. L. Hull, E. L. Lanni and M. S. Sanford, J. Am. Chem. Soc., 2006, 128, 14047 CrossRef CAS PubMed;
(b) X. Guo, G. Deng and C.-J. Li, Adv. Synth. Catal., 2009, 351, 2071 CrossRef CAS;
(c) X. Chen, G. Dobereiner, X.-S. Hao, R. Giri, N. Maugel and J.-Q. Yu, Tetrahedron, 2009, 65, 3085 CrossRef CAS.
-
(a) L. Ackermann, Chem. Rev., 2011, 111, 1315 CrossRef CAS PubMed;
(b) D. L. Davies, S. M. A. Donald and S. A. Macgregor, J. Am. Chem. Soc., 2005, 127, 13754 CrossRef CAS PubMed;
(c) J. Hubrich, T. Himmler, L. Rodefeld and L. Ackermann, ACS Catal., 2015, 5, 4089 CrossRef CAS;
(d) L. Ackermann, Org. Process Res. Dev., 2015, 19, 260 CrossRef CAS.
Footnote |
† Electronic supplementary information (ESI) available. CCDC 1481843. For ESI and crystallographic data in CIF or other electronic format see DOI: 10.1039/c7ra02638b |
|
This journal is © The Royal Society of Chemistry 2017 |
Click here to see how this site uses Cookies. View our privacy policy here.