DOI:
10.1039/C7RA01768E
(Paper)
RSC Adv., 2017,
7, 44124-44131
miRNA-21 promotes renal carcinoma cell invasion in a microfluidic device
Received
12th February 2017
, Accepted 29th July 2017
First published on 12th September 2017
Abstract
MicroRNAs (miR) play important roles in cancer invasion. In this study, we investigated the role of miR-21 in the invasion of renal cell carcinoma. The invasive abilities of renal carcinoma cell lines (786-O, Caki-1, A498), one normal epithelial kidney cell line (HK-2) and primary renal carcinoma cells were assessed in a microfluidic model. The expression of miR-21 was examined by quantitative real time PCR (qRT-PCR). We found that HK-2 cells with low miR-21 expression showed low invasive ability while renal carcinoma cell lines with high miR-21 expression showed high invasive ability. Primary renal cancer cells showed higher invasive ability than HK-2 cells, and this was consistent with the level of miR-21 expression. miR-21 knockdown reduced both renal carcinoma cell line and primary cancer cell invasion. Overall, this study shows that miR-21 promotes renal cancer cell invasion in both cell lines and primary cancer cells and suggests that miR-21 might be a new target in renal cancer therapy. In addition, we demonstrate that a microfluidic device provides a useful in vitro platform to study cancer cell invasion.
Introduction
Renal cell carcinoma (RCC) is the third most common urological cancer1 and clear cell RCC is the largest subtype of RCC, accounting for approximately 70% of RCC cases.2 Approximately 20–30% of RCC patients have a metastatic disease at the time of diagnosis.3 The average survival time from the diagnosis of distant metastasis is around 24 months, and less than 20% of patients survive more than 5 years.4 RCC is insensitive to chemotherapy and radiotherapy; therefore, effective post-operative adjuvant therapies are lacking.5 However, molecular-targeted therapy has been used in post-operative adjuvant therapy for patients with early-stage RCC or as palliative treatment for patients with advanced RCC.6 Although the objective effective rate has evidently increased, the overall survival rate among RCC patients is low. Therefore, further investigation of the RCC invasive mechanism and identification of new therapeutic methods are highly warranted.
Recent technological advances have shown great progress in the biomimetics field. As a new technology, microfluidics creates new opportunities for spatial and temporal control of cell growth and stimuli.7,8 Microfabricated devices have been developed to facilitate both applied and basic research concerning the biology of cells and tissues.9–13 By designing special microchannels, cell invasion can be observed in the microfluidic systems.14–16 For example, a microfluidic device was developed for monitoring cell migration across extracellular matrix-coated microgaps (ECM).14 Migration of invasive MDA-MB-231 cells was tracked by real-time light microscopy. Similarly, transendothelial invasion of tumor aggregates was successfully observed in a microfluidic system.16 For these reasons, the use of a microfluidic device provides a favorable method of studying the processes of RCC invasion and metastasis.
Although there has been widespread research into genetic biomarkers for RCC, epigenetic biomarkers including microRNAs (miR) have also received considerable attention because of their biological and clinical utility in diagnosis and treatment, especially miR-21.17 miR-21 was one of the first oncomiRs to be identified, and it has since been confirmed to be upregulated in numerous types of human cancers, including RCC.18 Previous studies have shown that miR-21 plays an important role in both RCC growth and invasion.19,20 Studies have shown that the higher the level of expression of miR-21, the greater the invasive ability of RCC cells,21,22 and at least two studies showed that elevated expression of miR-21 was associated with poor survival in RCC patients.23 miR-21 and its target programmed cell death 4 (PDCD4) will provide a strong candidate for therapeutic applications in renal cancer in the future.24,25 That miR-21 plays an important role in renal cell carcinomas has been proposed based on profiling studies using renal carcinoma cell lines and tumor tissue samples. However, the function of miR-21 in causing primary renal cancer cell invasion has rarely been shown. We used primary renal cancer cell and microfluidic model to mimic environment in body, and observed cancer cell invasion directly. The aims of this study were to use a microfluidics device to observe the invasion of RCC at the primary cellular level and to investigate whether knockdown of miR-21 could reduce the invasive ability of RCC.
Materials and methods
General cell culture and primary cell isolation
Our human cell lines (786-O, Caki-1, A498 and HK-2) were obtained from the Institute of Biochemistry and Cell Biology of the Chinese Academy of Sciences (Shanghai, China) as a commercial source. Normal renal HK-2 cells were cultured as a monolayer in Dulbecco's Modified Eagle Medium: nutrient mixture F-12 (DMEM/F12; Hyclone, Logan, UT, USA) with 10% fetal bovine serum (FBS; ScienCell, Carlsbad, CA, USA), 100 U mL−1 penicillin and 100 U mL−1 streptomycin (Hyclone). The RCC cell lines were maintained in RPMI 1640 medium supplemented with 10% FBS (ScienCell), 100 U mL−1 penicillin and 100 U mL−1 streptomycin (Hyclone). All cells lines were maintained in an incubator with a humidified atmosphere of 95% air and 5% CO2 at 37 °C.
Primary tumor cells were isolated from fresh RCC samples. This study was performed in strict accordance with the NIH guidelines for the care and use of laboratory animals (NIH Publication No. 85-23 Rev. 1985) and was approved by the Institutional Animal Care and Use Committee of National Tissue Engineering Center (Shanghai, China). The study protocol was approved by the Ethics Committee of the Second Affiliated Hospital of Dalian Medical University, all experiments were performed in compliance with relevant laws or guidelines and followed institutional guidelines. And we have informed consent of the patient was obtained. The patients did not receive any chemotherapy or radiotherapy before surgery. The tissues were minced and digested with collagenase IV (Invitrogen, Carlsbad, CA, USA) for 1 h at 37 °C with shaking. The dissociated tissues were then incubated without shaking for 5 min at room temperature, followed by separation of the supernatant to a new tube and centrifugation (1000 rpm, 5 min). The cell pellet was resuspended in fresh culture medium and incubated at 37 °C with 5% CO2 and 95% relative humidity.
Chip fabrication and cell invasion assay using a microfluidic model
We constructed a device composed of two layers: the polydimethylsiloxane (PDMS) layer and a glass substrate. The PDMS layer was fabricated by replicate molding of the master, which was prepared by spin-coating SU8-2035 negative photoresist (Microchem Corp., Westborough, MA, USA) onto a glass wafer, and then patterned by photolithography. Double coating and patterning SU8-2035, respectively, led to the formation of microstructures with heights of 100 and 50 μm. The lower microstructure was defined by patterning the first layer of SU8-2035 with a thickness of 100 μm. The second 50 μm thickness SU8-2035 layer was then patterned to form the higher microstructure. Sylgard 184 PDMS base and curing agent (Sylgard Silicone elastomer 184, Dow Corning Corp., Midland, MI, USA) were mixed thoroughly (10
:
1 by mass), degassed under vacuum, and poured onto the master. The polymer curing process was conducted in an oven for 1 h at 80 °C. After cooling, the PDMS layer was gently peeled off the master and trimmed to size. Inlet and outlet holes were created by punching through the PDMS with a razor-sharp punch. The piece of PDMS was irreversibly bonded to a glass slide after oxygen plasma treatment for 60 s. Prior to use, the device was sterilized with UV light for 30 min.
To evaluate the invasive potential of RCC, a microfluidic device developed in our previous study was used. Matrigel™ (Corning), as the substitute for ECM, was loaded into the matrix inlet. Matrigel was thawed at 4 °C in a refrigerator overnight. Next, the cold liquid state Matrigel was loaded into the matrix inlet of the microfluidic device. Driven by capillary force, the liquid flowed into stimulation channels and arrived at the junction between the main channel and side channels. Rapid enlargement of the height of the main channel changes the physical angle between the liquid interface and solid surface; hence, a temporary barrier for the advancing liquid is formed. Thus, Matrigel stopped at the interface of the main channel and side channels. Matrigel gelled in less than 30 min at room temperature. To assess the invasive activity of 786-O, Caki-1, A498, HK-2 and primary RCC cells, the cells were seeded into the cell culture channel in serum-free medium, the density of cell was 1 × 106 mL−1, and medium with 20% FBS was loaded into the stimulation channel. The cell invasion area was determined as the area invaded by cells in the matrix channel and measured by Image-Pro Plus 6.0 (Media Cybernetics, Rockville, MD, USA). The data represent at least three experiments performed in triplicate (mean ± standard error).
Cell invasion assay using Transwell® plates
Transwell chambers (Corning) pre-coated with Matrigel (Corning) were used to perform the Transwell assay, evaluating cell invasion. The cell suspension (1 × 106 cells per mL) was prepared in 1640, following which 200 μL cell suspension was added into the upper chamber, and 600 μL 1640 supplemented with 20% FBS was added into the lower chamber. After incubating for 24 h at 37 °C in 5% CO2, the medium in the chambers was removed, the cells were fixed in 4% paraformaldehyde for 20 min, washed three times with PBS, and stained with 0.1% crystal violet (Solarbio, Beijing, China) for 20 min. The cells adhering to the upper surface of the membrane were removed using a cotton applicator. The cells on the lower side of the membrane were counted. Five random high-power fields (×200) were selected and the mean number of cells was identified as the cell invasion number. The data represent at least three experiments performed in triplicate (mean ± standard error).
RNAi assay
Renal carcinoma cell lines and primary cells were incubated in a six-well tissue culture dish without antibiotics for 24 h prior to transfection respectively, when they had reached 60–80% confluence. Negative control (NC), specific miR-21 inhibitor and mimic transfection reagent complexes were mixed with Lipofectamine 2000 (Invitrogen) according to the manufacturer's recommendations and then added to the cells. After 6 h at 37 °C, the medium was changed and the cells were cultured in RPMI 1640 supplemented with 10% heat-inactivated fetal bovine serum for various times. Silencing of miRNA21 was determined by qRT-PCR.
Total RNA isolation and cDNA synthesis
TRIzol reagent (CWbio. Co. Ltd., Beijing, China) was used to isolate total RNA from the cells. The isolated RNA was treated with DNase I (Invitrogen). The RNA concentration and purity were determined using a NanoDrop® ND-1000 (Nanodrop Products, Wilmington, DE, USA). The ratio of 28S/18S was analyzed by Glyko Bandscan 5.0 (Glyko Biomedical Ltd., Novato, CA, USA). RNA quality and quantity were determined spectrophotometrically at 260 and 280 nm, respectively. Reverse transcription of RNA was performed using the NCode miRNA First-Strand cDNA Synthesis Kit (Invitrogen, Cat#: MIRC-50).
Primer design and quantitative real time PCR (qRT-PCR)
For miRNA-21 the primer sequence was 5′-TAGCTTATCAGACTGATGTTGA-3′. For U6, the sense primer sequence was 5′-CTCGCTTCGGCAGCACA-3′ and the antisense primer sequence was 5′-AACGCTTCACGAATTTGCGT-3′.
qRT-PCR was performed using the Light Cycler 2.0 Real-Time PCR System (Roche, Basel, Switzerland) in a total volume of 20 μL in glass capillaries containing 2 μL of cDNA, 0.8 μL of each primer, and 10 μL of Light Cycler TaqMan Master Mix (Invitrogen, Cat#: MIRC-50). The PCR for the miR-21 gene was initiated using a 10 min denaturation step at 95 °C followed by termination with a 30 s cooling step at 40 °C. The cycling protocol consisted of denaturation at 95 °C for 15 s and annealing at 60 °C for 60 s; 40 cycles. Fluorescence detection was performed at the end of each extension step. An additional extension step of 5 min at 72 °C was added following the completion of 40 cycles. All PCR reactions were performed in duplicate. The PCR products were confirmed by melting curve analysis. We used the mathematical delta–delta method (ratio = 2−ΔΔCT) developed by PE Applied Biosystems (Perkin Elmer, Waltham, MA, USA) to compare relative expression results between treatments in qRT-PCR.
Western blot
To prepare whole cell extracts, cells at 90% confluence were washed in phosphate-buffered saline (PBS) before incubation with lysis buffer (1% Triton X-100, 150 mM NaCl, 10 mM Tris, pH 7.4, 1 mM EDTA, 1 mM EGTA, pH 8.0, 0.2 mM Na3VO4, 0.2 mM phenylmethylsulfonyl fluoride, 0.5% Nonidet P-40) on ice for 10 min. The cell lysates were clarified by centrifugation at 12
000 rpm for 10 min and the supernatants were collected. The concentrations of total proteins were measured using a BCA Protein Assay Kit (Beyotime, Jiangsu, China). A total of 40 μg protein was separated using SDS-PAGE and transferred onto PVDF membranes; the membranes were then blocked in 5% fat-free milk at room temperature for 1 h. After incubation with rabbit primary antibodies against PDCD4 (Abcam, Cambridge, UK) at a dilution of 1
:
10
000 or GAPDH (Proteintech Group, Rosemont, IL, USA) at a dilution of 1
:
4000 at 4 °C overnight, the membranes were probed with goat anti-rabbit secondary antibodies at a dilution of 1
:
4000 at room temperature for 1 h. The signals were detected using BeyoECL Plus (Beyotime) determined by quantitative analysis using UVP software. The ratio of IODPDCD4/IODGAPDH indicated the relative expression of PDCD4 protein.
Statistical analysis
SPSS 13.0 software (SPSS, Chicago, IL, USA) was employed for the analysis of all data. Data are expressed as the mean ± standard deviation (SD), and Tamhane's T2 and analysis of variance (ANOVA) were used to determine the significance of the difference in multiple comparisons. An asterisk represents a P value < 0.05, which indicated a statistically significant result.
Results
Microfluidic device and renal cell line invasion
A microfluidic device developed in our previous study was used to investigate the lateral invasion of the renal cell lines and view the invasion process (Fig. 1A and B). The device is composed of a cell culture channel (red) and a stimulation channel (blue). The cell culture channel has both an outlet and an inlet while the stimulation channel has only inlet. The device is made of PDMS which is translucent. The device is sterilized and bonded to a 6 cm plate before use. The experimental design is shown in Fig. 1C. First, Matrigel™ was loaded into the stimulation channels, then HK-2 or renal carcinoma cell lines (786-O, Caki-1 and A498) in serum-free medium were seeded into the cell culture channel, while medium containing 20% serum was loaded into the stimulation channel. Using this microfluidic device (Fig. 1A–C), both the HK-2 and renal carcinoma cell lines were cultured successfully. Using this system we found that only renal carcinoma cell lines invaded into the matrix with long cellular processes (Fig. 2A). The invasion area of 786-O (21478.60 ± 7168.20 μm2), Caki-1 (22386.46 ± 4447.32 μm2), and A498 (23706.67 ± 6382.49 μm2) was significantly greater than that of HK-2 (235.24 ± 384.05 μm2). To evaluate invasion abilities of renal carcinoma cell lines and HK-2 further, we performed the Transwell® with Matrigel™ coating. And this revealed same results with microfluidic assay (Fig. 2B). Cell invasion number in pre view was used to evaluate cell invasion ability. The invasion ability of 786-O, Caki-1, A498 were higher than HK-2. These results suggest that renal carcinoma cell lines have distinct invasion abilities.
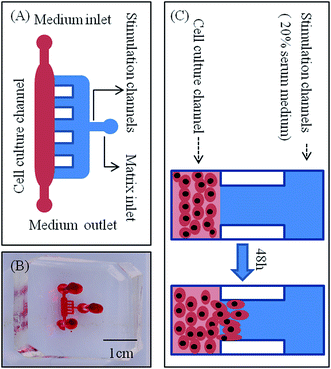 |
| Fig. 1 Microfluidic device and renal cell line invasion. (A) Schematic representation of the microfluidic chip. The height of the main channel (red) and side channels (blue) is 150 μm and 100 μm, respectively. (B) Photo of the microfluidic device filled with red ink. (C) Magnified illustration of the vessel unit, including one main channel, five side channels, and one matrix inlet. Scale bar = 20 μm. | |
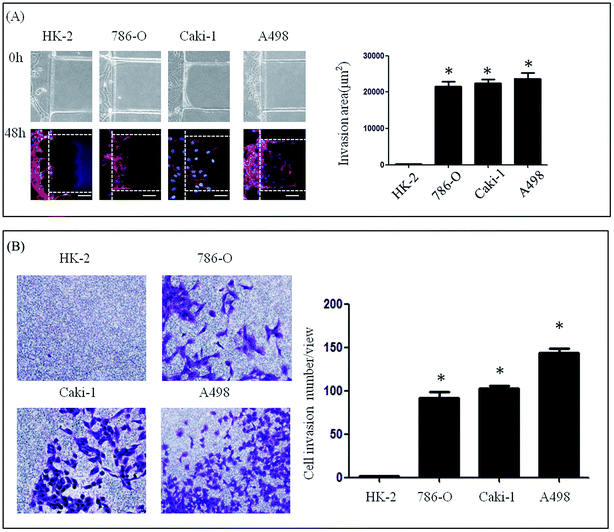 |
| Fig. 2 Invasion of renal carcinoma cell lines. (A) Microfluidic assay. The invasion ability of 786-O, Caki-1 and A498 showed significantly higher compared to HK-2. (B) Transwell® invasion assay. Significantly more 786-O, Caki-1 and A498 cells invaded through the matrix coating on the Transwell 1 membrane compared to HK-2. | |
Invasive ability of primary RCC cells
To further investigate the invasive ability of RCC, four samples of primary cells were isolated from RCC, and named as C995, C147, C316 and C413 according to each patient's admission number. These primary cells were cultured successfully using our microfluidic device under the same culture conditions as renal carcinoma cell lines. Medium containing 20% serum was then loaded into the stimulation channel, and 48 h later, the invasion area of primary cells and HK-2 cells was observed. The invasion areas of C147 (3881.56 ± 1776.59 μm2), C413 (7942.46 ± 3769.38 μm2), C316 (18999.70 ± 5068.86 μm2) and C995 (28196.62 ± 13691.84 μm2) were found to be significantly higher than that of HK-2 (235.24 ± 384.05 μm2) (Fig. 3A). Although the invasion areas of C147 and C143 were not as high as C316 or C995, these results suggest that primary cells also have distinct invasive abilities.
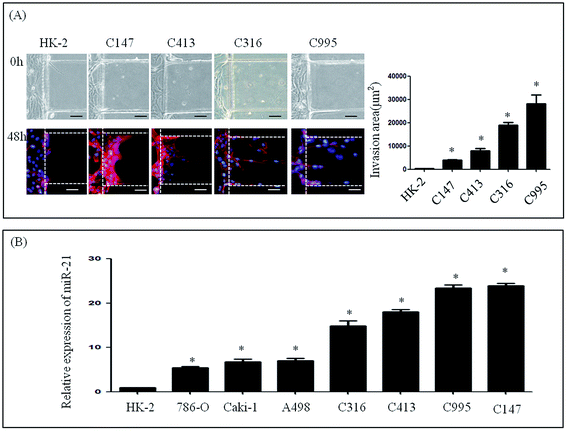 |
| Fig. 3 Invasion of primary renal carcinoma cells and expression of miR-21. (A) Invasion of HK-2 and primary renal carcinoma cells. (B) Expression of miR-21 in HK-2, renal carcinoma cell lines and primary renal carcinoma cells. | |
Expression of miR-21 in renal carcinoma cells and RNAi assay
We next determined the expression levels of miR-21 in renal cancer cells (786-O, Caki-1, A498, C995, C147, C316 and C413) and in a normal renal cell line (HK-2) (Fig. 3B). In the renal cancer cell lines and in primary cells levels of endogenous miR-21 were higher than HK-2 cells according to qRT-PCR analysis (Fig. 3B).
Invasive ability of renal cell lines after altering expression of miR-21
We cultured HK-2 cells and renal carcinoma cell lines that had been transfected with miR-21 mimic or miR-21 inhibitor in our microfluidic device and stimulated them with 20% serum for 48 h. HK-2 cells transfected with miR-21 mimic showed high invasive abilities compared to mimic NC (235.24 ± 384.05 μm2 vs. 2502.66 ± 1527.75 μm2) (Fig. 4A). This result demonstrated that the invasion area of 786-O, Caki-1 and A498 cells treated with miR-21 inhibitor was significantly smaller than that of 786-O Caki-1 and A498 cells treated with miR-21 inhibitor NC (21478.60 ± 7168.20 μm2 vs. 8603.51 ± 5461.17 μm2, 22386.46 ± 4447.32 μm2 vs. 7942.46 ± 3769.38 μm2, 23706.67 ± 6382.49 μm2 vs. 4949.94 ± 3681.27 μm2) (Fig. 4B–D).
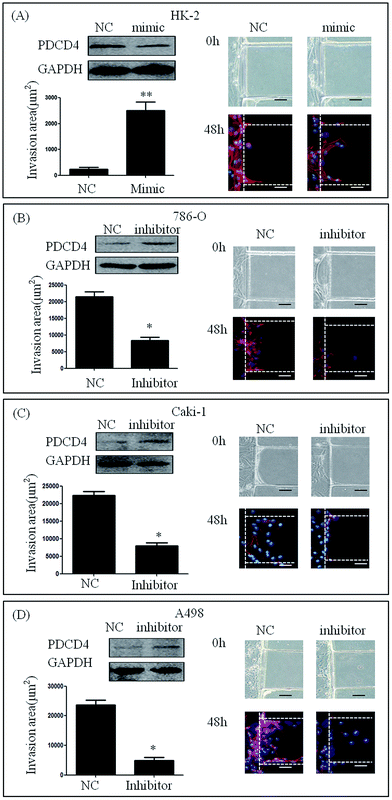 |
| Fig. 4 Invasion in renal cancer cell lines after regulation of miR-21. Western blot assay was used to detect ectopic PDCD4 in these cell lines, as indicated in the upper panel, where GAPDH was used as a loading control. (A) The result of invasion assay of the HK-2 cells transfected with miR-21 mimic NC, or miR-21 mimic after 48 h. The invasion area of HK-2 cells treated with mimic were significantly higher than those of the cells treated with mimic NC. (B–D) The result of invasion assay for the renal cancer cell lines transfected with miR-21 inhibitor NC, or miR-21 inhibitor, measured at 48 h. The invasion area of renal cancer cell lines treated with inhibitor NC was significantly higher than that of those treated with inhibitor. * indicates P < 0.05. Scale bar = 20 μm. | |
Invasive ability of primary RCC cells after knocking down miR-21
To test the involvement of miR-21 in the invasion of primary renal carcinoma cells (C147, C413, C316 and C995), we transfected them with miR-21 inhibitor and negative control siRNA. After stimulation with 20% serum for 48 h, the invasion areas of C147 cells and C413 cells treated with miR-21 inhibitor were smaller compared with those treated with inhibitor NC (3881.56 ± 1776.59 μm2 vs. 3189.62 ± 2078.81 μm2, 7942.46 ± 3769.38 μm2 vs. 5935.06 ± 4324.74 μm2), but the difference was not statistically significant (Fig. 5A and B). Further, treatment of C316 cells and C995 cells with miR-21 inhibitor resulted in a smaller invasion area compared with treatment with inhibitor NC (18999.70 ± 5068.86 μm2 vs. 5188.44 ± 3307.50 μm2, 28196.62 ± 13691.84 μm2 vs. 9277.06 ± 3920.32 μm2) (P < 0.05) (Fig. 5C and D). This result suggests that knockdown of the miR-21 gene could inhibit cell invasion by renal carcinoma.
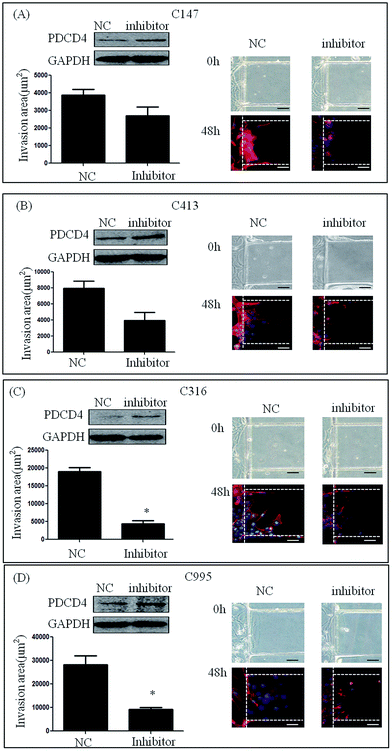 |
| Fig. 5 Invasion in primary cells after treatment with miR-21 inhibitor. Western blot assay was used to detect ectopic PDCD4 expression in these cell lines, as indicated in the upper panel, where GAPDH was used as a loading control. (A and B) Invasion areas of C147 and C413 treated with miR-21 inhibitor were lower than those of cells treated with inhibitor NC. (C and D) Invasion areas of C316 and C995 treated with miR-21 inhibitor were significantly lower than those of cells treated with inhibitor NC.* indicates P < 0.05. Scale bar = 20 μm. | |
Discussion
RCC is one of the most common urological cancers, and 20–30% of patients with RCC already have distant metastases with clinical symptoms upon diagnosis. For this reason, RCC, and especially its invasion and metastasis process or mechanism, is currently a hot topic of study.26–29 The Boyden chamber assay is widely used to study RCC invasion and metastasis but also has inherent limitations. This system lacks real-time observation and it is inherently difficult to directly assess tumor cell interaction with stromal cells.30 In addition, since cells move from the upper transwell to the lower culture chamber, cell invasion may be induced by gravity.31 For cell-based assays in a microfluidic device, one of the important advantages is to provide a well-controlled environment for precise study of cellular activities.32–34 Transendothelial invasion of tumor aggregates has been successfully observed in a microfluidic device,16 while another study used this microfluidic device to investigate whether HK-2 cells could migrate across the basement membrane following stimulation with transforming growth factor β1 (TGF-β1), C3a, or heat-inactivated healthy human serum (HHS).35 However, to our knowledge, such a microfluidic device has rarely been used to observe RCC invasion in real time. In this study, we cultured HK-2, renal carcinoma cell lines and primary renal carcinoma cells using a microfluidic device. After stimulating with 20% serum for 48 h and observing the cells under a microscope, we found that both renal carcinoma cell lines and primary renal carcinoma cells showed higher invasive ability compared with HK-2 cells. Most studies of RCC have been carried out using renal carcinoma cell lines such as A498, 786-O, or Caki-1 only. In this study, we used primary renal carcinoma cells and found that, compared with the effects observed in cell lines, the results obtained with primary cells were more persuasive. We concluded that we could use this microfluidic device to study factors such as miRs, drugs, and cytokines, that are more physiological factors influencing RCC invasion.
For RCC, miR-21 could be a promising, convenient and potentially non-invasive prognostic marker, and could allow monitoring of cancer progression or recurrence.36 In this study, we aimed to investigate whether miR-21 could be a therapeutic target. Our results showed that the invasion area of renal carcinoma cell lines treated with miR-21 inhibitor was significantly smaller than that of untreated renal carcinoma cell lines. This demonstrated that we could regulate the invasive ability of cells by controlling miR-21. Next, we attempted to control the miR-21 expression of primary renal carcinoma cells. The result showed that knockdown of miR-21 reduced the invasive ability of primary cells, implying that inhibition of miR-21 expression could inhibit RCC invasion. To our knowledge, RCC is insensitive to chemotherapy and radiotherapy; therefore, effective post-operative adjuvant therapies are lacking.5 The combined traditional immune therapy using interleukin (IL)-II and interferon does not improve survival of patients with relapsed or advanced RCC,37 with five- and 10-year survival rates of <10%.38 At present, molecular-targeted therapy is used in post-operative adjuvant therapy for patients with early-stage RCC or as palliative treatment for patients with advanced RCC.39–42 Although the objective effective rate has evidently increased, the overall survival rate among RCC patients is low.6 In addition, molecular-targeted therapy is not only expensive but also causes many complications (hand–foot syndrome, sicchasia and emesia).41–43 As time goes by, drug resistance of RCC for molecular-targeted therapy is inevitable. Consequently it is extremely urgent to develop a new drug for RCC. The results of this study indicate that miR-21 could be a therapeutic target of RCC.
There are some molecular pathways of miR-21 which may play an important role in the process of RCC invasion, such as PDCD4, CDKN1A, and PTEN.23,43,44 In our study, we only broadly observed the influence of miR-21 on RCC invasion, without investigating which one or more molecular pathways could be the key of RCC therapy. Additionally, the number of primary renal carcinoma cell samples was only four, causing our study to lack conviction. We will still collect renal carcinoma cell samples in our further study. For C147 cells and C413 cells, the invasion areas of cells treated with miR-21 inhibitor were smaller compared with those treated with inhibitor NC, but the difference was not statistically significant. The cell line about renal cell carcinoma was taken from the tumor tissue of the patient, so tumor heterogeneity of the patients may lead to the non-aligned phenomenon. Moreover, post-transcriptional modification about the target genes of miR-21 may be also related to the phenomenon. All these are limitations of our study, but could be a direction of our future studies.
Conclusions
In summary, we cultured renal cell lines and primary renal carcinoma cells in vitro using our microfluidic device, and using this technique we reproduced RCC invasion at the cellular level. This device offers a novel platform which is a rapid and inexpensive way to study the mechanism of RCC invasion in vitro. We found that knocking down miR-21 could lead to a reduction in the invasive ability of renal carcinoma cells. These data suggest that miR-21 could be a new therapeutic target of RCC.
Conflicts of interest
The authors have declared that no competing interests exist.
Acknowledgements
The study was funded by the Natural Science Foundation of China (No. 81572505).
References
- D. J. van Spronsen, P. F. Mulders and P. H. De Mulder, Novel treatments for metastatic renal cell carcinoma, Crit. Rev. Oncol. Hematol., 2005, 55(3), 177–191 CrossRef CAS PubMed.
- H. T. Cohen and F. J. McGovern, Renal-cell carcinoma, N. Engl. J. Med., 2005, 353(23), 2477–2490 CrossRef CAS PubMed.
- P. L. Crispen, R. H. Breau, C. Allmer, C. M. Lohse, J. C. Cheville and B. C. Leibovich, et al., Lymph node dissection at the time of radical nephrectomy for high-risk clear cell renal cell carcinoma: indications and recommendations for surgical templates, Eur. Urol., 2011, 59(1), 18–23 CrossRef PubMed.
- Z. Wotschofsky, J. Liep, H. A. Meyer, M. Jung, I. Wagner and A. C. Disch, et al., Identification of metastamirs as metastasis-associated microRNAs in clear cell renal cell carcinomas, Int. J. Biol. Sci., 2012, 8(10), 1363–1374 CrossRef CAS PubMed.
- B. Escudier, Advanced renal cell carcinoma: current and emerging management strategies, Drugs, 2007, 67(9), 1257–1264 CrossRef CAS PubMed.
- T. Cheng, L. Wang, Y. Li, C. Huang, L. Zeng and J. Yang, Differential microRNA expression in renal cell carcinoma, Oncol. Lett., 2013, 6(3), 769–776 CAS.
- J. El-Ali, P. K. Sorger and K. F. Jensen, Cells on chips, Nature, 2006, 442(7101), 403–411 CrossRef CAS PubMed.
- G. M. Whitesides, The origins and the future of microfluidics, Nature, 2006, 442(7101), 368–373 CrossRef CAS PubMed.
- T. Liu, C. Li, H. Li, S. Zeng, J. Qin and B. Lin, A microfluidic device for characterizing the invasion of cancer cells in 3-D matrix, Electrophoresis, 2009, 30(24), 4285–4291 CrossRef CAS PubMed.
- T. Liu, B. Lin and J. Qin, Carcinoma-associated fibroblasts promoted tumor spheroid invasion on a microfluidic 3D co-culture device, Lab Chip, 2010, 10(13), 1671–1677 RSC.
- J. M. Peyrin, B. Deleglise, L. Saias, M. Vignes, P. Gougis and S. Magnifico, et al., Axon diodes for the reconstruction of oriented neuronal networks in microfluidic chambers, Lab Chip, 2011, 11(21), 3663–3673 RSC.
- S. R. Khetani and S. N. Bhatia, Microscale culture of human liver cells for drug development, Nat. Biotechnol., 2008, 26(1), 120–126 CrossRef CAS PubMed.
- D. Huh, B. D. Matthews, A. Mammoto, M. Montoya-Zavala, H. Y. Hsin and D. E. Ingber, Reconstituting organ-level lung functions on a chip, Science, 2010, 328(5986), 1662–1668 CrossRef CAS PubMed.
- K. E. Sung, N. Yang, C. Pehlke, P. J. Keely, K. W. Eliceiri and A. Friedl, et al., Transition to invasion in breast cancer: a microfluidic in vitro model enables examination of spatial and temporal effects, Integr. Biol., 2011, 3(4), 439–450 RSC.
- K. C. Chaw, M. Manimaran, F. E. Tay and S. Swaminathan, Matrigel coated polydimethylsiloxane based microfluidic devices for studying metastatic and non-metastatic cancer cell invasion and migration, Biomed. Microdevices, 2007, 9(4), 597–602 CrossRef CAS PubMed.
- Q. Zhang, T. Liu and J. Qin, A microfluidic-based device for study of transendothelial invasion of tumor aggregates in realtime, Lab Chip, 2012, 12(16), 2837–2842 RSC.
- M. Li, Y. Wang, Y. Song, R. Bu, B. Yin and X. Fei, et al., MicroRNAs in renal cell carcinoma: a systematic review of clinical implications (Review), Oncol. Rep., 2015, 33(4), 1571–1578 CrossRef CAS PubMed.
- S. D. Selcuklu, M. T. Donoghue and C. Spillane, miR-21 as a key regulator of oncogenic processes, Biochem. Soc. Trans., 2009, 37(Pt 4), 918–925 CrossRef CAS PubMed.
- X. Lu, Y. Liu, F. Luo, A. Zhang, X. Liu and L. Lu, et al., MicroRNA-21 activation of Akt via PTEN is involved in the epithelial-mesenchymal transition and malignant transformation of human keratinocytes induced by arsenite, Toxicol. Res., 2016, 5(4), 1140–1147 RSC.
- J. Li, S. Tan, R. Kooger, C. Zhang and Y. Zhang, MicroRNAs as novel biological targets for detection and regulation, Chem. Soc. Rev., 2014, 43(2), 506–517 RSC.
- Z. A. Dotan, E. Fridman, Y. Spector, I. Barshack, A. Chajut and S. Rosenwald, et al., MicroRNAs as prognostic markers for survival in renal cell carcinoma conventional type T 2-4, J. Clin. Oncol., 2011, 29(suppl. 15), e21115 Search PubMed.
- N. Dey, F. Das, N. Ghoshchoudhury, C. C. Mandal, D. J. Parekh and K. Block, et al., microRNA-21 governs TORC1 activation in renal cancer cell proliferation and invasion, PLoS One, 2012, 7(6), 193–203 Search PubMed.
- L. Gu, H. Li, L. Chen, X. Ma, Y. Gao and X. Li, et al., MicroRNAs as prognostic molecular signatures in renal cell carcinoma: a systematic review and meta-analysis, Oncotarget, 2015, 6(32), 32545–32560 Search PubMed.
- X. Li, S. Xin, Z. He, X. Che, J. Wang and X. Xiao, et al., MicroRNA-21 (miR-21) post-transcriptionally downregulates tumor suppressor PDCD4 and promotes cell transformation, proliferation, and metastasis in renal cell carcinoma, Cell. Physiol. Biochem., 2014, 33(6), 1631–1642 CrossRef CAS PubMed.
- Y. Sakurai, K. Kajimoto and H. Harashima, Anti-angiogenic nanotherapy via active targeting systems to tumors and adipose tissue vasculature, Biomater. Sci., 2015, 3(9), 1253–1265 RSC.
- M. Ishida, S. Mikami, T. Shinojima, T. Kosaka, R. Mizuno and E. Kikuchi, et al., Activation of aryl hydrocarbon receptor promotes invasion of clear cell renal cell carcinoma and is associated with poor prognosis and cigarette smoke, Int. J. Cancer, 2015, 137(2), 299–310 CrossRef CAS PubMed.
- S. Mikami, M. Oya, R. Mizuno, T. Kosaka, K. Katsube and Y. Okada, Invasion and metastasis of renal cell carcinoma, Med. Mol. Morphol., 2014, 47(2), 63–67 CrossRef CAS PubMed.
- Y. Tomita, Treatment strategies for advanced renal cell carcinoma: A new paradigm for surgical treatment, Int. J. Urol., 2016, 23(1), 13–21 CrossRef CAS PubMed.
- R. Nishikawa, Y. Goto, S. Sakamoto, T. Chiyomaru, H. Enokida and S. Kojima, et al., Tumor-suppressive microRNA-218 inhibits cancer cell migration and invasion via targeting of LASP1 in prostate cancer, Cancer Sci., 2014, 105(7), 802–811 CrossRef CAS PubMed.
- T. Yu, Z. Guo, H. Fan, J. Song, Y. Liu and Z. Gao, et al., Cancer-associated fibroblasts promote non-small cell lung cancer cell invasion by upregulation of glucose-regulated protein 78 (GRP78) expression in an integrated bionic microfluidic device, Oncotarget, 2016, 7(18), 25593–25603 Search PubMed.
- K. F. Lei, H. P. Tseng, C. Y. Lee and N. M. Tsang, Quantitative study of cell invasion process under extracellular stimulation of cytokine in a microfluidic device, Sci. Rep., 2016, 6, 25557 CrossRef CAS PubMed.
- S. Halldorsson, E. Lucumi, R. Gomez-Sjoberg and R. M. Fleming, Advantages and challenges of microfluidic cell culture in polydimethylsiloxane devices, Biosens. Bioelectron., 2015, 63, 218–231 CrossRef CAS PubMed.
- M. Mehling and S. Tay, Microfluidic cell culture, Curr. Opin. Biotechnol., 2014, 25, 95–102 CrossRef CAS PubMed.
- C. W. Shields, C. D. Reyes and G. P. Lopez, Microfluidic cell sorting: a review of the advances in the separation of cells from debulking to rare cell isolation, Lab Chip, 2015, 15(5), 1230–1249 RSC.
- M. Zhou, H. Ma, H. Lin and J. Qin, Induction of epithelial-to-mesenchymal transition in proximal tubular epithelial cells on microfluidic devices, Biomaterials, 2014, 35(5), 1390–1401 CrossRef CAS PubMed.
- L. Gu, H. Li, L. Chen, X. Ma, Y. Gao and X. Li, et al., MicroRNAs as prognostic molecular signatures in renal cell carcinoma: a systematic review and meta-analysis, Oncotarget, 2015, 6(32), 32545–32560 Search PubMed.
- E. L. Broghammer and T. L. Ratliff, Immunotherapy of urologic tumors: principles and progress, Urol. Oncol., 2002, 7(2), 45–56 CrossRef PubMed.
- P. Mulders, R. Figlin, J. B. deKernion, R. Wiltrout, M. Linehan and D. Parkinson, et al., Renal cell carcinoma: recent progress and future directions, Cancer Res., 1997, 57(22), 5189–5195 CAS.
- B. Escudier, A. Pluzanska, P. Koralewski, A. Ravaud, S. Bracarda and C. Szczylik, et al., Bevacizumab plus interferon alfa-2a for treatment of metastatic renal cell carcinoma: a randomised, double-blind phase III trial, Lancet, 2007, 370(9605), 2103–2111 CrossRef.
- B. Escudier, T. Eisen, W. M. Stadler, C. Szczylik, S. Oudard and M. Staehler, et al., Sorafenib for treatment of renal cell carcinoma: final efficacy and safety results of the phase III treatment approaches in renal cancer global evaluation trial, J. Clin. Oncol., 2009, 27(20), 3312–3318 CrossRef CAS PubMed.
- C. N. Sternberg, I. D. Davis, J. Mardiak, C. Szczylik, E. Lee and J. Wagstaff, et al., Pazopanib in locally advanced or metastatic renal cell carcinoma: results of a randomized phase III trial, J. Clin. Oncol., 2010, 28(6), 1061–1068 CrossRef CAS PubMed.
- G. Hudes, M. Carducci, P. Tomczak, J. Dutcher, R. Figlin and A. Kapoor, et al., Temsirolimus, interferon alfa, or both for advanced renal-cell carcinoma, N. Engl. J. Med., 2007, 356(22), 2271–2281 CrossRef CAS PubMed.
- M. Santoni, A. Conti, U. De Giorgi, R. Iacovelli, F. Pantano and L. Burattini, et al., Risk of gastrointestinal events with sorafenib, sunitinib and pazopanib in patients with solid tumors: a systematic review and meta-analysis of clinical trials, Int. J. Cancer, 2014, 135(4), 763–773 CrossRef CAS PubMed.
- M. S. Zaman, V. Shahryari, G. Deng, S. Thamminana, S. Saini and S. Majid, et al., Up-regulation of microRNA-21 correlates with lower kidney cancer survival, PLoS One, 2012, 7(2), e31060 CAS.
Footnote |
† Co-first authors. |
|
This journal is © The Royal Society of Chemistry 2017 |
Click here to see how this site uses Cookies. View our privacy policy here.