DOI:
10.1039/C7RA01476G
(Paper)
RSC Adv., 2017,
7, 34236-34243
Bioassay-guided isolation of bisepoxylignans from the flower buds of Magnolia biondii Pamp and their antiallergic effects†
Received
5th February 2017
, Accepted 4th May 2017
First published on 7th July 2017
Abstract
The dried flower buds of Magnolia biondii Pamp (herbal name, Xin-Yi) are a traditional Chinese medicine with a long history of clinical use in the treatment of allergic rhinitis and sinusitis. However, the constituents responsible for its antiallergic effects remain unidentified. In the present study, a novel bisepoxylignan, isoeudesmin (1), and eight known bisepoxylignans, pluviatilol (2), eudesmin (3), magnolin (4), lirioresinol-B dimethyl ether (5), epimagnolin B (6), kobusin (7), aschantin (8), fargesin (9), were isolated from effective fractions through antiallergic bioassay-guided procedures using mouse bone marrow-derived mast cells (BMMCs) and IgE-induced passive cutaneous anaphylaxis mice. The compound structures were elucidated through nuclear magnetic resonance and mass spectrometry analyses. Compound 1 and compounds 2–6 and 9 exhibited antiallergic effects without affecting the viability of BMMCs. Compounds 2 and 9 showed the strongest effects with IC50 values of 52.18 and 93.03 μM, respectively. In this paper, the structure–activity relationship between these bisepoxylignans and their antiallergic effects is discussed. After evaluating the intensity of the antiallergic effects of the extracts, further separating the fractions, and isolating the purified compounds, we concluded that biosepoxylignans are the main constituents in Xin-Yi contributing to its anti-allergic efficacy.
Introduction
Magnolia biondii Pamp of the family Magnoliaceae is mainly distributed in Henan, China.1 The dried flower buds of Magnolia biondii Pamp (herbal name, Xin-Yi) are among the most commonly used herbs in Chinese medicine. They have a long history of clinical use in the treatment of allergic diseases.2,3 In ancient China, Xin-Yi was reported to be efficacious in the treatment of cutaneous pruritus (recorded in the book Solar Corona Medicine, published in 900 A.D.), allergic rhinitis, and sinusitis (recorded in the book Austroyunnanese Medicine, published in 1300 A.D.). The antiallergic effects of Xin-Yi have also been reported in some prescriptions in ancient China. For example, Xanthium pulvis, XinQin decoction, and Xin-Yi decoction, which are mainly composed of Xin-Yi, were used in the treatment of allergic rhinitis, sinusitis, and asthma. These prescriptions are still used in clinical medicine today.4 Animal experiments have confirmed that these prescriptions exert their antiallergic effects by inhibiting the degranulation of mast cells,5 alleviating homologous passive skin anaphylaxis, and suppressing histamine-induced metatarsal swelling in rats.6,7 A clinical study reporting on the effects of Xin-Yi decoction in 40 patients with allergic asthma showed that patients who received the treatment exhibited improved pulmonary function and vital capacity, and their forced expiratory volume 1 (FEV1) and FEV1% were more significantly altered (P < 0.01) than those in a group of controls, indicating that Xin-Yi is highly effective in the treatment of allergies.8 Therefore, determining the exact constituents responsible for its antiallergic effects requires additional research.
Phytochemical research has revealed that Xin-Yi is a prolific source of bisepoxylignans, which exhibit a broad range of biological activities,9 such as anti-inflammatory,10 antioxidant, antimicrobial, anticancer, and antiplatelet activities as well as liver protection.11 Recent studies have revealed that various types of lignans, such as gomisin N (dibenzocylooctadiene lignan), mesodihydroguaiaretic acid (dibenzylbutane lignan), and pinusthunbergiside A (benzodioxane lignan),12–14 exert their antiallergic effects by suppressing mast cell activation. Bisepoxylignans from the stems of Lindera obtusiloba Blume were reported to play a crucial role in antiallergic inflammation through the suppression of A23187 – stimulated human mast cell degranulation.15 Among a bioactive screen of 95% ethanol (EtOH) extract, water extract, and volatile oil components from Xin-Yi, 95% EtOH extract was reported to exert the most potent antiallergic effects in vivo; however, the exact constituents contributing to this effect remain unidentified.16 Biosepoxylignans are the main constituents of Xin-Yi; therefore, they might play a crucial role in the antiallergic effects of Xin-Yi.
The present study investigated the antiallergic effects of 95% EtOH extract of Xin-Yi, further separated the fractions, and isolated the purified compounds by using an acknowledged antiallergic test model—the mouse bone marrow-derived mast cell (BMMC) degranulation test—and the passive cutaneous anaphylaxis (PCA) mice model. We obtained one novel bisepoxylignan, isoeudesmin (1), and eight known biosepoxylignans, pluviatilol (2), eudesmin (3), magnolin (4), lirioresinol-B dimethyl ether (5), epimagnolin B (6), kobusin (7), aschantin (8), and fargesin (9) (Fig. 1A), through bioassay-guided isolation procedures. The bioassay results revealed that compound 1 and compounds 2–6 and 9 exerted inhibitory effects on BMMC degranulation and tumour necrosis factor α (TNF-α) expression. Furthermore, we also discussed the structure–activity relationship between these bisepoxylignans and their antiallergic effects. The results indicated that biosepoxylignans, as the main constituents in Xin-Yi contribute to its antiallergic efficacy.
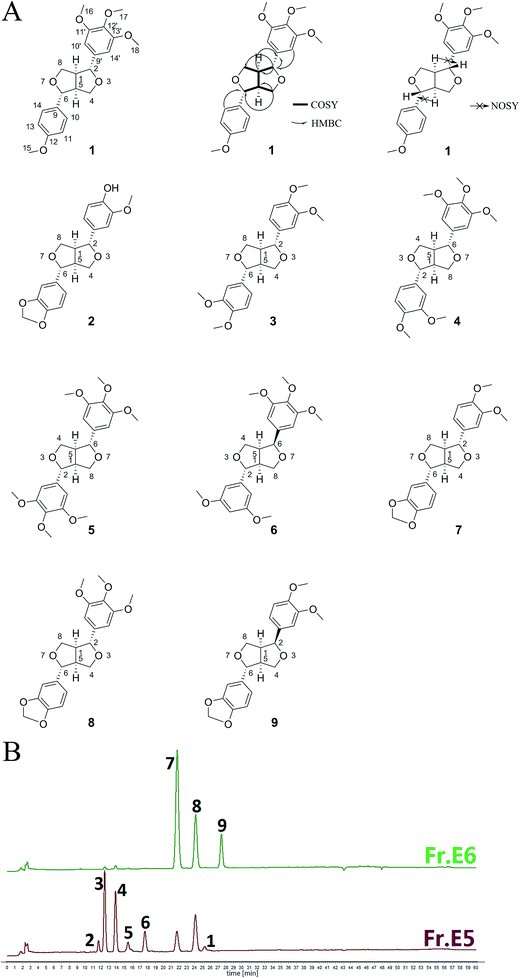 |
| Fig. 1 Chemical structures of nine compounds 1–9 extracted from Xin-Yi (A) and HPLC fingerprints of the effective fractions, Frs. E5 and E6 (B). | |
Experimental section
General experimental procedures
IR spectra were obtained using a Nicolet-Magna-750-FTIR spectrometer (KBr pellets) (Thermo Fisher). Optical rotations were recorded using a Perkin-Elmer 341 polarimeter. Silica gel (200–300 mesh; Qingdao Haiyang Chemical Company), Sephadex LH-20 (GE Biosciences), MCI Gel Chp20p (Mitsubishi Chemical, Tokyo, Japan), and ODS (Fuji Silica Chemical Company) were purchased for column chromatography. Thin layer chromatography (TLC) plates were obtained from the Yantai Jiangyou Company. Electron ionisation-mass spectrometry (EI-MS) and high-resolution EI-MS (HR-EI-MS) data were obtained using a Thermo DFS mass spectrometer (in m/z). Electrospray ionisation (ESI)-MS and HR-ESI-MS analyses were performed using a Finnigan LCQ Deca XP equipped with an ESI source mass ion-trap spectrometer and Waters Micromass Q-TOF Ultima Globe spectrometer, respectively. 1H, 13C, and 2D nuclear magnetic resonance (NMR) spectra were obtained using Bruker AV-400 and AV-600 instruments. Semipreparative high-performance liquid chromatography (HPLC) was performed using an Agilent 1200 HPLC system, and samples were separated on an Agilent Eclipse XDB-C18 (5 μm, 9.4 × 250 mm) column. The HPLC analysis of effective fractions was performed using an Agilent ZORBAX SB-C18 (5 μm, 4.6 × 250 mm) column with a mobile phase of CH3CN and H2O [0–20 min, 40
:
60 (CH3CN
:
H2O, v/v); 20–40 min, 40
:
60–60
:
40; 40–50 min, 60
:
40–95
:
5; 50–53 min, 95
:
5; 53–55 min, 95:5–40
:
60; 55–60 min, 40
:
60] at a flow rate of 1.0 mL min−1. UV spectroscopy data were collected at 260 nm.
Plant material
Xin-Yi was collected from Nanzhao, Henan, China, which is the main Xin-Yi-producing area in China. The authenticity of this medicinal material was verified by Professor Zhili Zhao at the School of Pharmacy, Shanghai University of Traditional Chinese Medicine, Shanghai, China. A voucher specimen (20150101) has been deposited in the Department of Phytochemistry, School of Pharmacy, Shanghai University of Traditional Chinese Medicine, Shanghai, China.
Extraction and isolation
Dried and powdered Xin-Yi (17.5 kg) was extracted three times under reflux conditions in 95% EtOH (60 L and 2 h each at 80 °C). The combined extracts were evaporated at reduced pressure to yield a crude extract in vacuo at 60 °C. The crude extract was suspended in water (20 L) and subsequently partitioned with petroleum ether (PE; 20 L × 3) and ethyl acetate (EtOAc; 20 L × 3) successively. The combined EtOAc extract (1 kg) was subjected to MCI column chromatography and eluted with EtOH–H2O mixtures of decreasing polarity (EtOH
:
H2O = 1
:
9, 2
:
8, 4
:
6, 5
:
5, 6
:
4, 7
:
3, and 9
:
1) to yield six fractions (Frs. E1–E6) according to the TLC monitor. Frs. E5–E6 exhibited higher antiallergic activity than did Frs. E1–E4; therefore, they were subjected to further purification. Purifying Fr. E5 (24 g) through Sephadex LH-20 chromatography and eluting it with MeOH yielded compound 3 (117 mg) and six fractions (Frs. E5A–E5F). Purifying Fr. E5B (16 g) through ODS chromatography and eluting it with a MeOH–H2O system afforded compound 5 (529 mg) and six fractions (Frs. E5B1–E5B6). A 30 mg quantity of Fr. E5B5 (4.2 g) was purified through semipreparative HPLC in an ACN–H2O system, yielding compounds 4 (16 mg) and 6 (8 mg). Purifying Fr. E5C (2 g) through silica gel chromatography and eluting it with a PE–EtOAc system yielded compound 2 (24 mg). Subjecting Fr. E6 (6 g) to Sephadex LH-20 chromatography and eluting it with MeOH yielded 10 fractions (Frs. E6A–E6J). Fr. E6D (3 g) was further separated through ODS chromatography, eluted with MeOH–H2O, and then subjected to semipreparative HPLC in an ACN–H2O system to yield compounds 1 (5 mg), 7 (15 mg), and 8 (21 mg). A 20 mg quantity of Fr. E6F (1 g) was purified through semipreparative HPLC in ACN–H2O system to obtain compound 9 (6 mg).
Isoeudesmin (1). Yellowish wax, [α]25D: +70 (c 0.1, MeOH); UV (MeOH) λmax nm: 207, 232, 273. IR (KBr) νmax cm−1: 1590, 1512, 1462, 1417, 1373, 1329, 1246, 1175, 1128, 1061, 1035, 1007, 825; 1H-NMR (CDCl3, 600 MHz) and 13C-NMR (CDCl3, 151 MHz) NMR data (Table 1); HR-EI-MS at m/z: 386.1725 [M]+ (calculated for C22H26O6, 386.1729).
Table 1 1H-NMR (600 MHz) and 13C-NMR (151 MHz) spectral data of compound (1) in CDCl3
Position |
1H |
13C |
δH (multiplicity, J in Hz) |
δC,type |
1 |
3.07 (m) |
54.63 CH |
2 |
4.73 (d, 5.0) |
86.24 CH |
4 |
4.29 (dd, 9.1, 6.8), 3.88–3.94 (m) |
72.21 CH2 |
5 |
3.11 (m) |
54.16 CH |
6 |
4.78 (d, 4.7) |
85.66 CH |
8 |
4.25 (dd, 9.2, 6.6), 3.88–3.94 (m) |
71.71 CH2 |
9 |
— |
133.11 C |
9′ |
— |
136.99 C |
10 |
7.28 (d, 8.6) |
127.47 CH |
10′ |
6.57 (s) |
102.91 CH |
11 |
6.89 (d, 8.6) |
114.10 CH |
11′ |
— |
153.56 C |
12 |
— |
159.35 C |
12′ |
— |
137.55 C |
13 |
6.89 (d, 8.6) |
114.10 CH |
13′ |
— |
153.56 C |
14 |
7.28 (d, 8.6) |
127.47 CH |
14′ |
6.57 (s) |
102.91 CH |
15 |
3.84 (s) |
55.45 CH3 |
16 |
3.87 (s) |
56.31 CH3 |
17 |
3.81 (s) |
60.99 CH3 |
18 |
3.87 (s) |
56.31 CH3 |
BMMC culture
BMMCs were derived from the bone marrow of BALB/c mice. Cells were maintained in RPMI-1640 medium (Gibco) supplemented with 50 μM 2-mercaptoethanol (Sigma-Aldrich), 1 mM sodium pyruvate, 100 U per mL penicillin/100 μg mL−1 streptomycin, 0.1 mM nonessential amino acids, 25 mM N-2-hydroxyethylpiperazine-N-2′-ethanesulfonic acid (HEPES), 10% foetal bovine serum, and 10 ng mL−1 mouse recombinant interleukin-3 (PeproTech, Rocky Hill, NJ, USA). Cells were fed every 5 days. After 4 weeks, cell purity was assessed by measuring CD117 and FcεRI expression levels though flow cytometry. Of the cells, 99% were double-positive for CD117 and FcεRI.
Cell viability analysis using XTT assay
BMMCs were dispensed into 96-well plates (5 × 104 cells per well) and treated with the isolated compounds (100 μM) for 24 h, and cell viability was assessed using a 2.3-bis-(2-methoxy-4-nitro-5-sulfophenyl)-2H-tetrazolium-5-carboxyanilide salt (XTT)-based cell proliferation assay (Life Technologies, Mannheim, Germany), following the manufacturer's instructions. Cell viability is expressed as the percentage of ODtest relative to ODcontrol, where ODtest is the optical density of cells exposed to compounds (test samples), and ODcontrol is the optical density of the control samples.
Degranulation assay
First, BMMCs at 5 × 105 cells per mL were incubated with 0.5 μg mL−1 antidinitrophenol-IgE (anti-DNP-IgE) (Sigma Aldrich) in complete media overnight for sensitisation. Cells were resuspended in HEPES buffer (10 mM HEPES, 137 mM NaCl, 2.7 mM KCl, 0.4 mM Na2HPO4·7H2O, 5.6 mM glucose, 1.8 mM CaCl2·2H2O, and 1.3 mM MgSO4·7H2O) and transferred to a round-bottom 96-well plate. Subsequently, cells were treated with extracts (50 mg L−1) or compounds (25, 50, or 100 μM) for 30 min. Finally, cells were stimulated with 0.1 μg mL−1 DNP-bovine serum albumin (DNP-BSA; Biosearch Technologies) for 30 min. After sensitisation and stimulation, BMMC degranulation was assessed by measuring the β-hexosaminidase (β-hex) release. The β-hex concentration in the supernatants and pellets was quantified through p-nitrophenyl N-acetyl-β-D-glucosamide (Sigma-Aldrich, St. Louis, MO, USA) hydrolysis in 0.1 M sodium citrate buffer (pH 4.5) for 90 min at 37 °C. After 50 glycine (pH 10.7, Amresco) was added to each fraction, the absorbance was measured at A405 and A570 using a Multiskan Ascent 354 microplate reader (Thermo Labsystems, Vantaa, Finland). The amount of total β-hex released was calculated using the following formula: % release = 100 × supernatant absorbance/(supernatant absorbance + pellet absorbance).
Cytokine enzyme-linked immunosorbent assay
BMMCs were dispensed into 48-well plates (1 × 105 cells per well) and sensitised with 0.5 μg mL−1 anti-DNP-IgE for 24 h. The isolated compounds were dissolved in media containing 0.1% dimethyl sulfoxide and added to the cells to obtain a final concentration of 100 μM for 30 min. Cells were stimulated with DNP-BSA for 24 h for TNF-α expression. The supernatant was collected, centrifuged at 300 × g for 5 min to remove any cell debris, and stored at −80 °C until ELISA analysis. The supernatant was analysed for TNF-α expression using ELISA kits (eBioscience), following the manufacturer's instructions.
IgE-mediated local anaphylaxis in mice
ICR mice (6 week-old, male, 20–22 g) were obtained from the B&K Laboratory Animal Corp. Ltd. (Shanghai, China). All animal experiments were performed according to the Health Guidelines of the Shanghai University of Traditional Chinese Medicine, China, and protocols were approved by the Institutional Animal Ethics Committee of Shanghai University of Traditional Chinese Medicine. All mice were kept under a 12 hour light–dark cycle, temperature of 22 °C to 23 °C, and humidity of 50% to 60%. The animals were allowed free access to food and water and allowed to acclimatize for at least 7 days before the experiments.
IgE-induced PCA was performed as described previously.17 Briefly, 1 μg anti-DNP-IgE was intradermally injected into the right ear of 7 week old male mice. The next day, the mice received oral administration of 100 mg kg−1 Frs. E5 and E6 (in that order) or 50 mg kg−1 Ketotifen fumarate salt (Sigma-Aldrich). After 1 hour, the mice were challenged for 30 min by intravenous injection of 100 μg of DNP–HSA in 300 μL saline containing Evans blue (0.5%). Finally, Evans blue was extracted after 24 h at room temperature with 300 μL of formanide and measured by absorbance at 630 nm.
Statistical analysis
Experiments were conducted in triplicate. Values are presented as the mean ± standard error of the mean. Significant differences were determined using the Student t test with the level of significance set at *P ≤ 0.05, **P ≤ 0.01, and ***P ≤ 0.001.
Results and discussion
The EtOAc-soluble portion of the 95% EtOH extract of Xin-Yi was purified separately by column chromatography over MCI gel, silica gel, reversed-phase C18 silica gel, Sephadex LH-20, and by preparative HPLC, yielding six fractions and nine compounds, including one novel biosepoxylignan (1) and eight known biosepoxylignans (2–8) (see Extraction and isolation).
According to the effect of 95% EtOH extract on the allergic mouse model,16 we examined the effects of 95% EtOH extract on BMMC degranulation. The results revealed that 95% EtOH extract exerted a weak antiallergic effect; it inhibited β-hex release by 22.77% relative to that of the control at 50 mg L−1 for 30 min in the BMMC model. Moreover, we examined the effects of 50 mg L−1 EtOAc and PE extracts from 95% EtOH extract on BMMC degranulation. The results revealed that treatment of BMMCs with EtOAc extract for 30 min considerably reduced the β-hex release by up to 51.4% relative to that of the control, whereas PE extracts slightly inhibited the β-hex release, reducing it by 13.06% relative to that of the control. On the basis of these results, we hypothesised that EtOAc extract contains the active constituents. Therefore, we examined the effects of the six fractions from EtOAc extract on BMMC degranulation. Treatment of BMMCs with Frs. E2, E3, E5, and E6 at 50 mg L−1 for 30 min considerably affected the BMMC β-hex release (Fig. 2). In particular, Frs. E5 and E6 inhibited β-hex release by up to 65.5% and 85.6% relative to that of the control, respectively.
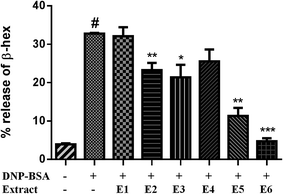 |
| Fig. 2 Effects of fractions from EtOAc extracts on BMMC degranulation. | |
Based on the in vitro results, we evaluated the antiallergic activity of Frs. E5 and E6 in a PCA model. As shown as Fig. 3, the colour in the ear of Frs. E5 and E6 group became lighter than that of the model group. Additionally, Frs. E5 and E6 significantly reduced the dye extravasations by 26.15% (P < 0.05) and 38.94% (P < 0.001), respectively. These results suggest that Frs. E5 and E6 exert in vivo antiallergic effects by suppressing mast cell activation.
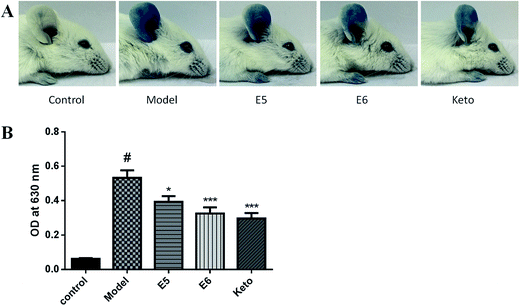 |
| Fig. 3 Effect of the fractions of Xin-Yi on antigen (Ag) induced PCA in ICR mice. (A) Representative photos of ears showing dye extravasation. (B) Evans blue dye was extracted in formaldehyde and quantified as absorbance at 630 nm. | |
Next, nine compounds were obtained from the active Frs. E5, and E6, namely isoeudesmin (1), pluviatilol (2),18 eudesmin (3),19 magnolin (4),20 lirioresinol-B dimethyl ether (5),21 epimagnolin B (6),20 kobusin (7),20 aschantin (8),22 and fargesin (9).20 Their chemical structures are illustrated in Fig. 1A. Compounds 2–9 were identified by comparing their 1H-NMR and MS data (details show in the ESI†) with those reported in the literature. Compound 1 was identified as follows.
Compound 1 was a yellowish wax with a molecular formula of C22H26O6, as determined through HR-EI-MS (m/z: 386.1725 [M]+). The 13C-NMR and DEPT spectrum (Table 1) exhibited four methoxyl signals at δC 55.45 (C-15), 56.31 (C-16, 18), and 60.99 (C-17); two methylenes at δC 71.71 (C-8) and 72.21 (C-4); two methynes at δC 54.16 (C-5) and 54.63 (C-1); two oxygenated methynes at δC 85.66 (C-6) and 86.24 (C-2); three coupled methynes at δC 102.91 (C-10′, 14′), 114.1 (C-11, 13), and 127.47 (C-10, 14); and six quaternary carbons at δC 133.11 (C-9), 136.99 (C-9′), 137.55 (C-12′), 153.56 (C-11′, 13′), and 159.35 (C-12). The 1H-NMR spectrum (Table 1) revealed four methoxyl signals at δH 3.81 (3H, s, H-17), 3.84 (3H, s, H-15), and 3.87 (6H, s, H-16, 18). The signals of two aromatic protons, including δH 6.57 (s, 2H, H-10′, 14′), indicated the formation of a 1,3,4,5-tetrasubstituted benzene ring, and the AA'XX′-type aromatic proton signals at δH 6.89 (2H, d, J = 8.6 Hz, H-11, 13) and 7.28 (2H, d, J = 8.6 Hz, H-10, 14) as well as the signals at δH 3.07 (1H, m, H-1), 3.11 (1H, m, H-5), 3.88–3.94 (2H, m, H-4a, 8a), 4.25 (1H, dd, J = 9.2, 6.6 Hz, H-8e), and 4.29 (1H, dd, J = 9.1, 6.8 Hz, H-4e) indicated that compound 1 contains two furan rings. Furthermore, analysis of the HMBC correlations also revealed that there are two furan rings in the compound 1 (δ 4.73 → 54.63, 71.71, 3.88, 4.29 → 54.16, 85.66), and two benzene rings were linked by these two furan rings (δ 7.28 → 85.66, 6.57 → 86.24, 4.73 → 136.99; Fig. 1A). Therefore, the planar structure of compound 1 was determined. H-2 = H-6: δ 4.67–4.78 (d, J = 5 Hz), H-1 = H-5: δ 3.07–3.11, H-4a = H-8a: δ 3.88–3.94, and H-4e = H-8e: δ 4.25–4.29 (dd, J = 9.1, 6.8 Hz) revealed that compound 1 belonged to the symmetrical bisepoxylignan group.23 NOSY revealed that H-1, 5 and H-2, 6 were unrelated and also confirmed that compound 1 was a symmetrical bisepoxylignan (Fig. 1A). Therefore, the absolute configuration of compound 1 was determined, and it was named “isoeudesmin”.
Next, the antiallergic effects of compounds 1–9 obtained from Frs. E5 and E6 extracts on BMMC degranulation were evaluated. Results revealed that compounds 1–6 and 9 at 100 μM reduced the β-hex release without affecting the BMMC viability (Fig. 4A). In addition, treatment of BMMCs with compounds 2 and 9 for 30 min suppressed the β-hex release in a dose-dependent manner; the inhibition was up to 67.8% and 46.5% relative to the control, respectively (Fig. 4B). Furthermore, compounds 2 and 9 were the most effective one, with IC50 values of 52.18 and 93.03 μM, respectively.
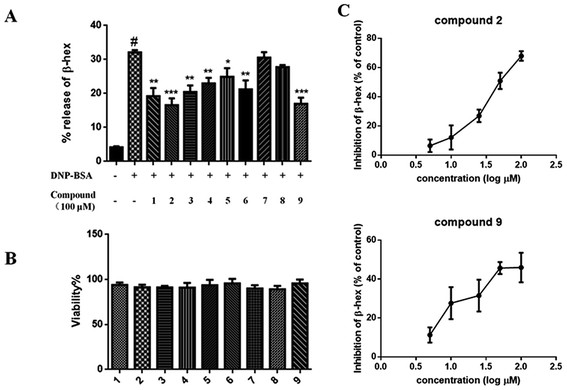 |
| Fig. 4 Effects of isolated compounds on degranulation and cell viability. (A) BMMCs were treated with 0.5 μg mL−1 anti-DNP-IgE 24 h, and various compounds (100 μmol mL−1) for 30 min, then 0.1 μg mL−1 DNP-BSA for 30 min. (B) BMMCs were treated with various compounds (100 μmol mL−1) for 24 h. Cell viability was determined using an XTT assay. (C) Effects of compounds 2 and 9 on BMMCs degranulation. | |
TNF-α, produced by activated BMMCs, mediates many physiological processes of allergic inflammation; therefore, we determined the effect of isolated compounds on TNF-α expression. We incubated BMMCs with 0.5 μg mL−1 anti-DNP-IgE and 100 μM isolated compounds for 30 min and subsequently stimulated them with 0.1 μg mL−1 DNP-BSA for 24 h. Compounds 1–6 and 9 considerably inhibited TNF-α expression (Fig. 5). In particular, compounds 2 and 9 suppressed TNF-α expression by up to 55.9% and 68.1%, respectively. Thus, the present study determined the constituents responsible for the antiallergic effects of Xin-Yi.
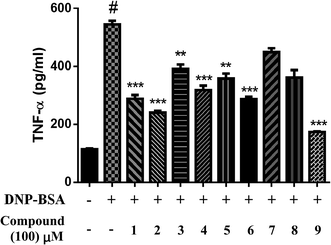 |
| Fig. 5 Effects of isolated compounds on TNF-α release. | |
On the basis of the antiallergic effects of compounds 1–6 and 9, we demonstrated the differences in the effects of the main active Frs. E5 and E6. The HPLC fingerprints (Fig. 1B) revealed that Fr. E5 mainly contains compounds 1–8, and Fr. E6 mainly contains compounds 7–9. In addition, compounds 2 and 9 exerted the strongest antiallergic effects among the nine compounds. Fr. E6 exerted stronger effects than did Fr. E5, because compound 9 was a main constituent in Fr. E6, and compound 2 was merely a microconstituent in Fr. E5. The agreement of results on the antiallergic effects between the fractions and purified compounds illustrates the feasibility of our bioassay-guided isolation procedure. Bisepoxylignans are present in large quantities (total content of compounds 3–5, 8, and 9 up to 5%) in Xin-Yi,24 indicating that they are the main bioactive constituents responsible for Xin-Yi's antiallergic efficacy; however, the intensity of these bisepoxylignans was moderate.
Finally, because of the similarity between the structures of compounds 1–9 and marked differences in their antiallergic effects, we can discuss the structure–activity relationship between these bisepoxylignans and antiallergic effects (Fig. 6). First, a greater number of methoxyl groups in the benzene ring was associated with a reduced antiallergic effect, as evidenced by the comparison of the antiallergic effects of compounds 2 and 3 with those of compounds 4, 5, 7, and 8. Second, compounds 7 and 8 exerted weaker antiallergic effects than did compounds 3 and 4, indicating that the methylenedioxy group reduced the activity. Third, substitution of the hydroxyl group with a methoxyl group in the benzene ring, such as in compounds 7 and 8, reduced the antiallergic effects compared with those of compound 2, suggesting that hydroxyl to methoxyl substitution reduces the antiallergic activity. Fourth, symmetrical bisepoxylignans, namely compounds 1, 3, 4, 5, 7, and 8, exerted substantially weaker antiallergic effects compared with those of the asymmetrical bisepoxylignans, namely compounds 6 and 9, indicating that the stereochemical structure of the compounds is crucial to their antiallergic activity. This finding is consistent with a previous study on the antiallergic activity of bisepoxylignans.15
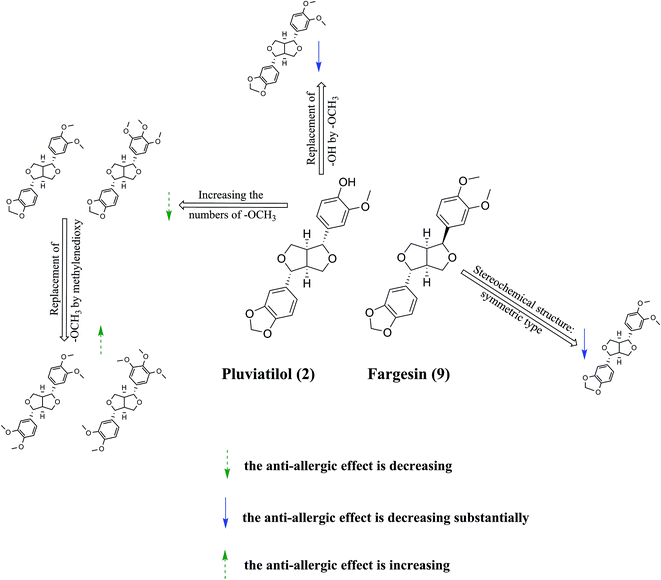 |
| Fig. 6 The structure–activity relationships of bisepoxylignans on the anti-allergic effect. | |
Conclusions
In conclusion, nine bisepoxylignans, including one novel compound, were obtained through antiallergic bioassay-guided isolation using in vitro and in vivo models. The proposed compound (isoeudesmin) and known compounds (pluviatilol, eudesmin, magnolin, lirioresinol-B dimethyl ether, epimagnolin B, and fargesin) obtained from Xin-Yi inhibited BMMC degranulation and TNF-α expression, contributing to Xin-Yi's antiallergic effect. Moreover, bisepoxylignans can be considered candidates for antiallergic agents derived from Xin-Yi.
Acknowledgements
This work was supported by the Excellent Academic Leaders Program of Shanghai (16XD1403500), the Eastern Scholar Tracking Program of Shanghai Municipal Education Commission (2012-90), the National Natural Science Foundation of China (81673570, 81261120567), and the program of Shanghai E-Research Institute of Bioactive Constituents in Traditional Chinese Medicine.
References
- D. L. Fu, The plant resources and new taxa and cultivars of yulania spach, The Doctoral Dissertation of Central South Forestry University. 2001, vol. 2, p. 88 Search PubMed.
- Y. H. Wang, F. Ye and X. H. Zhang, China Med. Her., 2012, 16, 12–14 Search PubMed.
- The Pharmacopoeia Commission of People's Republic of China, Pharmacopoeia of the People's Republic of China, Chemical Industry Press, Beijing, China, 2010, p. 169 Search PubMed.
- H. L. Wang, Z. H. Li and Y. T. Xu, Chin. Tradit. Herb. Drugs, 2015, 46, 1542–1555 CAS.
- H. Wei, R. S. Zhang, Z. X. Si and Y. F. Zhou, Chin. J. Exp. Tradit. Med. Formulae, 1997, 3, 21–23 Search PubMed.
- T. Ji and L. Y. Wang, China Pharm., 2010, 19, 27–28 Search PubMed.
- D. H. Deng, X. H. Shen and W. R. Zhu, Chin. J. Tradit. Med. Sci. Technol., 2007, 14, 318–319 Search PubMed.
- J. Jiang, X. S. Fan, N. Shang and Y. C. Liu, Journal of Nanjing TCM University, 2002, 18, 333–334 Search PubMed.
- Q. Song and N. H. Fischer, J. Mex. Chem. Soc., 1999, 43, 211–218 CAS.
- Y. Shen, C. G. Li, S. F. Zhou, E. C. K. Pang, D. F Story and C. C. Xue, Curr. Med. Chem., 2008, 15, 1616–1627 CrossRef CAS PubMed.
- G. P. Han and H. F. Zhou, Journal of Shanxi Normal University, 2005, 6, 142–144 Search PubMed.
- H. S. Chae, O. H. Kang, Y. C. Oh, J. G. Choi and J. H. Keum, Immunopharmacol. Immunotoxicol., 2011, 33, 709–771 CrossRef CAS PubMed.
- T. C. Moon, C. S. Seo, K. Haa, J. C. Kim, N. K. Hwang, T. G. Hong, J. H. Kim, D. H. Kim, J. K. Son and H. W. Chang, Arch. Pharmacal Res., 2008, 31, 606–610 CrossRef CAS PubMed.
- S. S. Hong, W. Jeong, J. K. Kim, J. G. Kwon, J. Y. Lee, E. K. Ahn, J. Oh, D. W. Seo and S. Oh, Fitoterapia, 2014, 99, 347–351 CrossRef CAS PubMed.
- H. G. Choi, Y. H. Choi, J. H. Kim, H. H. Kim, S. H. Kim, J. A. H. Kim, S. M. Lee, M. Na and S. H. Lee, Arch. Pharmacal Res., 2014, 37, 467–472 CrossRef CAS PubMed.
- R. Sun, X. L. Qian and L. L. Lv, J. Pharmacovigilance, 2013, 10, 71–73 Search PubMed.
- D. Wang, M. Zheng and Y. Qiu, J. Exp. Med., 2014, 13, 2635–2649 CrossRef PubMed.
- T. Iida, Y. Noro and K. Ito, Phytochemistry, 1983, 22, 211–213 CrossRef CAS.
- Y. W. Seo, Biotechnol. Bioprocess Eng., 2010, 15, 400–406 CrossRef CAS.
- H. W. Luo, Study on the chemical components and pharmacological activity of Magnolia denudate Desr, The Doctoral Dissertation of South-Central University for Nationalities, 2013 Search PubMed.
- Q. Guo, H. Fang and W. Su, Chin. Tradit. Herb. Drugs, 2004, 35, 849–852 CAS.
- T. J. Schmidt, S. Hemmati, E. Fuss and A. W. Alfermann, Phytochem. Anal., 2006, 17, 299–311 CrossRef CAS PubMed.
- H. Greger and O. Hofer, Tetrahedron, 1980, 36, 3551–3558 CrossRef CAS.
- P. M. Yu, Z. Y. Tian and Q. T. Xu, Lishizhen Medicine and Material Medica Research, 2005, vol. 16, pp. 652–653 Search PubMed.
Footnotes |
† Electronic supplementary information (ESI) available: Spectra of all compounds. See DOI: 10.1039/c7ra01476g |
‡ These authors contributed equally to this work. |
|
This journal is © The Royal Society of Chemistry 2017 |
Click here to see how this site uses Cookies. View our privacy policy here.