DOI:
10.1039/C7RA01186E
(Paper)
RSC Adv., 2017,
7, 11657-11664
Crown ether complexes of potassium quinolin-8-olates: synthesis, characterization and catalysis toward the ring-opening polymerization of rac-lactide†
Received
26th January 2017
, Accepted 9th February 2017
First published on 16th February 2017
Abstract
A series of crown ether complexes of potassium quinolin-8-olates were synthesized and characterized. Catalysis of these complexes towards the ring-opening polymerization of rac-lactide was evaluated. The crown ether complexes of potassium quinolin-8-olate and potassium 2-methylquinolin-8-olate exhibited high catalytic activity and good molecular weight control. The 18-crown-6 complex of potassium 5-chloroquinolin-8-olate showed lower catalytic activity and poor molecular weight control; whereas the 18-crown-6 complex of potassium 5,7-dichloroquinolin-8-olate was inactive. Among the complexes, 18-crown-6 complex of potassium quinolin-8-olate showed the best selectivity of isotacticity, the Pm value achieving 0.75 when the polymerization was performed in toluene at 0 °C.
Introduction
Polylactides (PLAs) as biodegradable and biocompatible polymers have found wide applications in medical, agricultural, and other fields. They are also considered as an alternative to petrochemical-derived polyolefin because the monomers can be obtained from annually renewable resources.1,2 The most efficient method for the production of PLAs is the ring-opening polymerization (ROP) of lactides (LA) catalyzed by metal complexes such as magnesium,3 calcium,4 aluminum,5 zinc,6 tin,7 titanium,8 zirconium9 and lanthanide complexes,10 which resulted in PLAs with high and controlled molecular weights, low polydispersity indices (PDIs), and specific stereo-microstructures. In recent years Li+, Na+ and K+ complexes also received considerable attention in catalyzing the ROP of LA because the alkali metal ions are nontoxic, cheap and easily available, and their complexes were often highly catalytically active.11,12 Various mono-, di-, and tridentate ligands were employed to stabilize the metal ions and tune catalytic properties of the complexes. For example, Cano et al. reported catalysis of Li, Na, and K phenoxo-imine complexes toward the ROP of rac-LA and demonstrated that the lithium complexes resulted in formation of heterorich-PLA (Pr up to 0.75) and the potassium complexes led to atactic PLA.11b Lin and Sun respectively reported catalysis of NNO-tridentate Schiff base-sodium complexes in the ROP of L-LA or rac-LA.11p,q In 2014 Wu and co-workers demonstrated that sodium and potassium complexes supported by a monophenoxy with one xanthenyl group at the ortho-position and 18-crown-6 or 15-crown-5 as an auxiliary ligand were highly active and isoselective catalysts for the ROP of rac-LA.12a Subsequently, several related monophenolato sodium and potassium complexes with crown ethers as auxiliary ligands were tested for the catalysis.12b–f In these examples, bulky phenoxy ligands are necessary for the stereoselective polymerization. In view of the work mentioned above, we attempted to study catalysis of crown ether potassium complexes bearing a didentate ligand. A chelate coordination mode will restrict rotation of K–O(quinolin-8-olate) single bond. This is probably helpful to improve the selectivity of the catalytic polymerization of rac-LA. For the purpose, we synthesized crown ether complexes of potassium quinolin-8-olates and studied their catalysis towards the ROP of rac-LA. Here we report the results.
Results and discussion
Synthesis of crown ether complexes of potassium quinolin-8-olates is presented in Scheme 1. In the presence of crown ether treatment of 8-hydroxyquinolines with KN(SiMe3)2 (KHMDS) in THF at room temperature generated corresponding crown ether complexes of potassium quinolin-8-olates 1–5. The complexes are soluble in DMSO, partly soluble in THF and almost insoluble in toluene. Elemental analyses demonstrated that composition of the complexes matched the respective formula of the expected molecules. 1H and 13C NMR spectroscopy were consistent with the respective molecular structure. Single crystal structural analysis of complex 1 (Fig. 1) showed that the central K+ cation is coordinated by quinolin-8-olate ligand through N,O-chelate mode and capped by 18-crown-6 through coordination of the six oxygen atoms. The K1–O1 distance of 2.728(2) Å is longer than the C–O distances in the potassium monophenolates reported by Wu et al. (2.444(5)–2.6141(14) Å).12b,c,e This may be caused by the difference of electron effects of phenyl and quinolyl groups. The distances between the potassium atom and the oxygen atoms of the crown ether vary in a range from 2.844(2) to 3.134(2) Å. Among them the K1–O2, K1–O3, and K1–O4 distances [2.8534(19), 2.9581(19) and 2.844(2) Å, respectively] are comparable to those in the crown ether potassium monophenolates reported by Wu et al. (2.6695(12)–2.9555(18) Å), whereas K1–O5, K(1)–O(6), and K(1)–O(7) distances (2.980(2), 3.046(2) and 3.134(2) Å, respectively) are longer than the above-mentioned ones.12b,c,e The K–N distance of 2.830(2) Å is within the normal range.13
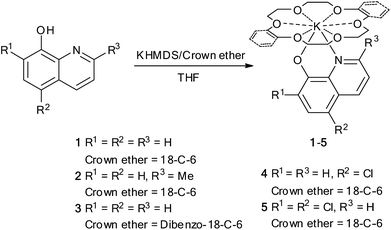 |
| Scheme 1 Synthesis of crown ether complexes of potassium quinolin-8-olates. | |
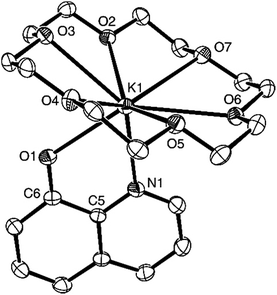 |
| Fig. 1 ORTEP drawing of complex 1. Selected bond lengths (Å) and angles (deg): K(1)–O(1) 2.728(2), K(1)–N(1) 2.830(2), K(1)–O(2) 2.8534(19), K(1)–O(3) 2.9581(19), K(1)–O(4) 2.844(2), K(1)–O(5) 2.980(2), K(1)–O(6) 3.046(2), K(1)–O(7) 3.134(2), O(1)–C(6) 1.291(3); O(1)–K(1)–N(1) 59.71(7). | |
Catalysis of complexes 1–5 towards the ROP of rac-LA was evaluated and the results are listed in Table 1. Complexes 1–3 exhibited good catalytic activity in toluene at room temperature. Complexes 1 and 2 can lead to almost complete monomer conversions in 10 min and 5 min, respectively, when using a ratio of 100
:
1
:
1 for [LA]0/[M]0/[BnOH]0 (Table 1, entries 1 and 2). Complex 3 led to 87% monomer conversion in 10 min under the same conditions (Table 1, entry 3). However, complex 4 exhibited lower catalytic activity compared with complexes 1–3. 4/BnOH-catalyzed polymerization of rac-LA required higher temperature (70 °C) when using a ratio of 100
:
1
:
1 for [LA]0/[M]0/[BnOH]0 and led to 71% monomer conversion in 60 min (Table 1, entry 4). Complex 5 was inactive even at 70 °C. It seems that the electron effects of the ligands markedly affect catalytic activity of the complexes. Electron-rich quinolinoxy ligands are beneficial to increase the catalytic activity of the potassium complexes. 18-Crown-6 complex of potassium quinolin-8-olate (1) exhibited a little higher activity than dibenzo-18-crown-6 complex of potassium quinolin-8-olate (3). But the latter showed better molecular weight control although both led to low PDIs of the polymers (Table 1, entries 1 and 3). The polymer formed by 2/BnOH catalysis showed a little higher PDI (PDI = 1.47), but the molecular weight determined by GPC approximately matched the calculated value. However, the polymer generated by 4/BnOH catalysis showed much lower molecular weight than the theoretical value (Table 1, entry 4). This may be due to high reaction temperature and long reaction time which caused side reactions such as transesterification and irreversible chain breaking reactions. The existence of transesterification was supported by the MALDI-TOF mass spectrum of the final polymer. The mass spectrum exhibited two main series of peaks. A series of peaks at 144m + 108 + 39, which can be assigned to m(C6H8O4) + BnOH + K+. Another series of peaks at 72m + 108 + 39, which can be ascribed to m(C3H4O2) + BnOH + K+ (Fig. S1 in ESI†). The series of peaks separated by m/z = 72 Da is indicative of transesterification occurring in the polymerization process.14 CH2Cl2 and THF were also used as solvents for the polymerization catalyzed by 1/BnOH. However, they were less effective than toluene. In these solvents the catalyst exhibited lower activity than in toluene and the molecular weight of the polymers obtained in these two solvents were also lower than the theoretical values (Table 1, entries 5 and 6). Catalysis of complex 1 was further tested for the polymerizations of lower and higher ratio of monomer to catalyst at room temperature (Table 1, entries 7–11). Polymerization of 20 equiv. of rac-LA was accomplished in 96% conversion within 2 min. When the ratio of monomer to catalyst was higher than 100, the polymerizations required longer time. The molecular weights of the polymers determined by GPC were lower than the calculated values. However, a linear relationship of molecular weights of the polymers to the ratio of monomer to catalyst was still remained (Fig. 2). The PDIs of the polymers were also low, ranging from 1.05 to 1.15. That is to say, the polymerizations are controlled. Complex 1 can also catalyze the immortal ROP of 500 equiv. of rac-LA in the presence of 10 equiv. of BnOH, resulting in polymer with controlled molecular weight and a relatively low PDI (Table 1, entry 12).
Table 1 ROP of rac-LA catalyzed by complexes 1–4a
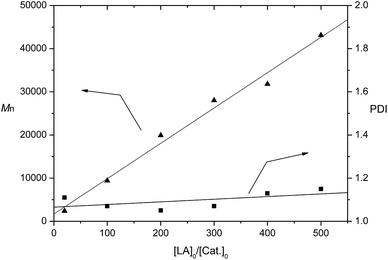 |
| Fig. 2 Polymerization of rac-LA catalyzed by 1/BnOH in toluene at room temperature. The relationships between Mn (▲), PDI (■) of the polymer and the initial mole ratios [LA]0/[BnOH]0 is shown (Table 1, entries 3 and 7–11). | |
The polymerizations catalyzed by complexes 1–4 in toluene were stereoselective, the Pm values of the formed polymers ranging from 0.62 to 0.72. Complex 1 led to the best selectivity, the Pm of the polymer achieving 0.72 at room temperature. However, the selectivity of complex 1 catalysis was lower when CH2Cl2 and THF were employed as the polymerization solvents (Table 1, entries 5 and 6). When the ratio of monomer to catalyst was higher than 100, the selectivity of polymers by complex 1 catalysis was approximately unchanged (Table 1, entries 8–11). In addition, reduction of polymerization temperature led to increase of selectivity. For example, polymerization of 100 equiv. of rac-LA catalyzed by complex 1 at 0 °C gave PLA with a Pm value of 0.75 (Table 1, entry 13 and Fig. 3). These selectivities are comparable to those of complexes 6 and 7 which involve bulky ortho-substituents on the aromatic rings (Chart 1).12b,c However, in our catalyst systems bulky substitutents on the phenoxy ligands are not necessary. This might be ascribed to rotation hindrance of the K–O bond due to O,N-chelate coordination of the ligand. The end group analysis of the PLA as shown in Fig. S2 (ESI†) proved that the polymer chain was capped with one benzyl ester end and one hydroxyl end, which are consistent with an insertion mechanism of a benzyloxy group into the lactide as supposed for most alkali metal phenoxides (Scheme 2).12
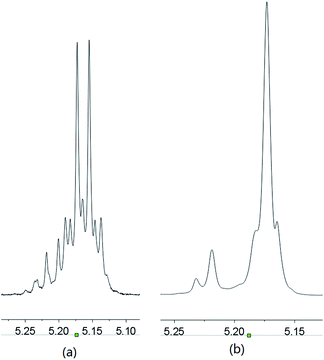 |
| Fig. 3 Methine region of the (a) normal 1H NMR spectrum and (b) homonuclear decoupled 1H NMR spectrum of the PLA prepared by complex 1/BnOH catalysis (Table 1, entry 13). | |
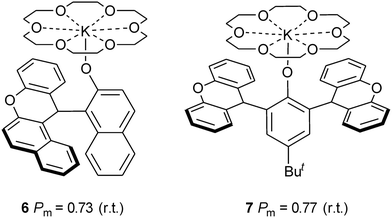 |
| Chart 1 | |
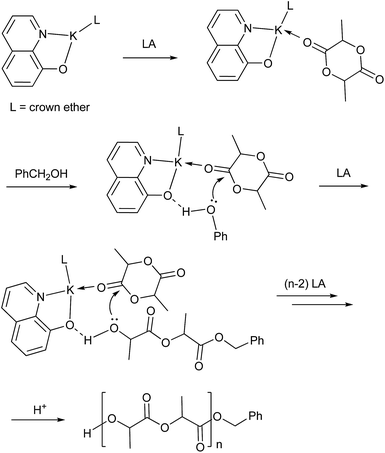 |
| Scheme 2 Proposed mechanism for the ROP of LA catalyzed by crown ether complex of potassium quinolin-8-olate. | |
Conclusions
We synthesized five crown ether complexes of potassium quinolin-8-olates and demonstrated four of them to be active catalysts for the ROP of rac-LA in the presence of BnOH. Electron effects of the quinolin-8-olate ligands strongly affect catalytic activity of the complexes. Electron-withdrawing substituted groups on the quinolyl ring caused reduction of catalytic activity or even inactivation of the complexes. Study using 1/BnOH in toluene also showed the catalytic polymerizations to be well controlled. Although quinolin-8-olate ligands have small steric hindrance, the complexes still led to stereoselective polymerization in toluene, the Pm ranging from 0.66 to 0.75 when the catalytic polymerization was carried out at room temperature or 0 °C. The rotation restriction of the O–K bond due to O,N-chelate coordination may play an important role in the selectivity besides the crown ether effect.
Experimental
All air or moisture sensitive manipulations were performed under dry N2 using standard Schlenk techniques. Solvents were distilled under nitrogen over sodium (toluene), sodium/benzophenone (tetrahydrofuran), or CaH2 (dichloromethane) and degassed prior to use. KN(SiMe3)2, crown ether and 8-hydroxyquinoline and its derivatives were purchased from local chemical companies and used as received. BnOH was distilled from CaO; rac-lactide was purchased from Daigang Biomaterial Co. and recrystallized three times from toluene prior to use. DMSO-d6, purchased from EMD Millipore Corporation, was degassed, and stored over 4 Å molecular sieves. CDCl3 was purchased from Cambridge Isotope Laboratories and used as received. NMR spectra were recorded on a Bruker AVANCE III 400 spectrometer at ambient temperature. The chemical shifts of 1H and 13C{1H} NMR spectra were referenced to TMS or internal solvent resonances. Elemental analyses were performed on an Elementar Vario EL III analyzer. Gel permeation chromatography (GPC) was recorded on a Waters 150C instrument equipped with UltraStyragel columns (103, 104, and 105 Å) and a 410 refractive index detector, using monodispersed polystyrene as the calibration standard. THF (HPLC grade) was used as an eluent at a flow rate of 1 cm3 min−1.
Synthesis of complex 1
To a stirred solution of 8-hydroxyquinoline (0.14 g, 1.00 mmol) and 18-crown-6 (0.27 g, 1.00 mmol) in THF (10 cm3) was slowly added KN(SiMe3)2 (1.1 cm3, 1 M solution in THF, 1.1 mmol) at room temperature. Then the mixture was stirred for 10 h and yellow precipitates were formed. The precipitates were collected by filtration, washed with THF, and dried in vacuo to give yellow solids (0.22 g, 47%). 1H NMR (400 MHz, DMSO-d6): δ 8.33 (dd, J = 3.9, 1.6 Hz, 1H), 7.78 (dd, J = 8.2, 1.6 Hz, 1H), 7.06 (dd, J = 8.2, 4.0 Hz, 1H), 6.97 (t, J = 7.8 Hz, 1H), 6.18 (d, J = 7.7 Hz, 1H), 6.14 (d, J = 8.0 Hz, 1H), 3.54 (s, 24H). 13C NMR (101 MHz, DMSO-d6): δ 170.25, 145.90, 142.54, 134.60, 131.50, 129.27, 119.44, 112.80, 101.44, 69.46. Anal. calcd for C21H30KNO7: C 56.36, H 6.76, N 3.13. Found: C 56.24, H 6.67, N 3.34.
Single crystals of complex 1 suitable for X-ray diffraction analysis were obtained from a saturated THF solution.
Synthesis of complex 2
To a stirred solution of 8-hydroxy-2-methylquinoline (0.16 g, 1.00 mmol) and 18-crown-6 (0.27 g, 1.00 mmol) in THF (10 cm3) was slowly added KN(SiMe3)2 (1.1 cm3, 1 M solution in THF, 1.1 mmol) at room temperature. The resultant mixture was stirred at room temperature for 10 h and yellow precipitates were formed. The precipitates were collected by filtration, washed with THF, and dried in vacuo to give yellow solids (0.19 g, 41%). 1H NMR (400 MHz, DMSO-d6): δ 7.68 (d, J = 8.3 Hz, 1H), 6.95 (d, J = 8.3 Hz, 1H), 6.89 (t, J = 7.8 Hz, 1H), 6.13 (d, J = 7.6 Hz, 1H), 6.09 (d, J = 7.9 Hz, 1H), 3.53 (s, 24H), 2.48 (s, 3H). 13C NMR (101 MHz, DMSO-d6): δ 169.85, 149.65, 145.06, 135.15, 129.44, 128.29, 119.79, 112.87, 101.34, 69.46, 24.58. Anal. calcd for C22H32KNO7: C 57.24, H 6.99, N 3.03. Found: C 57.06, H 6.92, N 3.05.
Synthesis of complex 3
To a solution of 8-hydroxyquinoline (0.14 g, 1.00 mmol) and dibenzo-18-crown-6 (0.36 g, 1.00 mmol) in THF (10 cm3) was slowly added KN(SiMe3)2 (1.1 cm3, 1 M solution in THF, 1.1 mmol) at room temperature with stirring. The resultant mixture was stirred at room temperature for 10 h. The yellow orange precipitates were collected by filtration, washed with THF, and dried in vacuo to give yellow orange solids (0.28 g, 52%). 1H NMR (400 MHz, DMSO-d6): δ 8.33 (dd, J = 3.9, 1.5 Hz, 1H), 7.84 (dd, J = 8.2, 1.3 Hz, 1H), 7.10 (dd, J = 8.2, 4.0 Hz, 1H), 7.05–6.94 (m, 5H), 6.93–6.86 (m, 4H), 6.29 (d, J = 7.7 Hz, 1H), 6.27 (d, J = 7.9 Hz, 1H), 4.22–4.02 (m, 8H), 3.99–3.78 (m, 8H), 3.64–3.55 (m, THF), 1.80–1.70 (m, THF). 13C NMR (101 MHz, DMSO-d6): δ 168.96, 147.26, 145.26, 143.14, 134.82, 131.20, 129.14, 120.84, 119.66, 112.66, 111.85, 103.03, 68.69, 67.14, 67.02, 25.13. Anal. calcd for C29H30KNO7: C 64.07, H 5.56, N 2.58. Found: C 63.93, H 5.76, N 2.54.
Synthesis of complex 4
To a solution of 5-chloro-8-hydroxyquinoline (0.18 g, 1.00 mmol) and 18-crown-6 (0.27 g, 1.00 mmol) in THF (10 cm3) was slowly added KN(SiMe3)2 (1.1 cm3, 1 M solution in THF, 1.1 mmol) at room temperature with stirring. The resultant mixture was stirred at room temperature for 10 h. The yellow precipitates were collected by filtration, washed with THF, and dried in vacuo to give yellow solids (0.25 g, 52%). 1H NMR (400 MHz, DMSO-d6): δ 8.39 (dd, J = 3.9, 1.5 Hz, 1H), 8.03 (dd, J = 8.4, 1.5 Hz, 1H), 7.26 (dd, J = 8.4, 4.0 Hz, 1H), 7.05 (d, J = 8.7 Hz, 1H), 6.08 (d, J = 8.7 Hz, 1H), 3.64–3.55 (m, THF), 3.54 (s, 24H), 1.81–1.69 (m, THF). 13C NMR (101 MHz, DMSO-d6): δ 169.90, 145.98, 142.87, 130.70, 129.34, 128.00, 120.62, 112.16, 100.65, 69.46, 67.03, 25.14. Anal. calcd for C21H29ClKNO7·0.55C4H8O: C 53.41, H 6.45, N 2.68. Found: C 53.47, H 6.38, N 2.60.
Synthesis of complex 5
To a stirred solution of 5,7-dichloro-8-hydroxyquinoline (0.21 g, 1.00 mmol) and 18-crown-6 (0.27 g, 1.00 mmol) in THF (10 cm3) was slowly added KN(SiMe3)2 (1.1 cm3, 1 M solution in THF 1.1 mmol) at room temperature. The resultant mixture was stirred at room temperature for 10 h. The yellow precipitates were collected by filtration, washed with THF, and dried in vacuo to give yellow solids (0.20 g, 39%). 1H NMR (400 MHz, DMSO-d6): δ 8.49 (dd, J = 3.9, 1.4 Hz, 1H), 8.07 (dd, J = 8.4, 1.6 Hz, 1H), 7.35–7.28 (m, 2H), 3.64–3.56 (m, THF), 3.54 (s, 24H), 1.79–1.71 (m, THF). 13C NMR (101 MHz, DMSO-d6): δ 163.61, 145.38, 144.33, 131.11, 128.40, 126.78, 120.76, 114.84, 100.58, 69.45, 67.01, 25.13. Anal. calcd for C21H28Cl2KNO7: C 48.84, H 5.46, N 2.71. Found: C 48.45, H 5.50, N 2.57.
X-ray crystallography
Single crystal of complex 1 was mounted in Lindemann capillaries under nitrogen. Diffraction data were collected at 298(2) K on a Bruker Smart CCD area detector with graphite-monochromated Mo Kα radiation (λ = 0.71073 Å). The structure was solved by direct methods using SHELXS-97 (ref. 16) and refined against F2 by full-matrix least squares using SHELXL-97.17 Hydrogen atoms were placed in calculated positions. Crystal data and experimental details of the structure determination are listed in Table 2.
Table 2 Details of the X-ray structure determinations of complex 1
Complex |
1·THF |
R1 = ∑||F0| − |Fc||/∑|F0|, wR2 = [∑w(F02 − Fc2)2/∑w(F04)]1/2. |
Empirical formula |
C25H38KNO8 |
fw |
519.66 |
Crystal system |
Monoclinic |
Space group |
P21/n |
a (Å) |
15.4725(12) |
b (Å) |
8.3765(7) |
c (Å) |
22.2908(19) |
β (deg) |
106.388(2) |
V (Å3) |
2771.6(4) |
Z |
4 |
Dcalcd (g cm−3) |
1.245 |
F(000) |
1112 |
μ (mm−1) |
0.237 |
θ range for data collecn (deg) |
2.61 to 25.02 |
No. of reflns collected |
13 547 |
No. of indep reflns (Rint) |
4867 (Rint = 0.0584) |
Restraints/params |
0/316 |
Goodness of fit on F2 |
1.078 |
Final R indicesa [I > 2σ(I)] |
R1 = 0.0490, wR2 = 0.0873 |
R Indices (all data) |
R1 = 0.1088, wR2 = 0.0961 |
Largest diff peak and hole [e Å−3] |
0.304 and −0.243 |
Polymerization of rac-LA
A typical polymerization procedure was exemplified using 1/BnOH as the catalyst. Complex 1 (8.94 mg, 0.02 mmol) and BnOH (0.20 cm3, 0.1 M in toluene, 0.02 mmol) were added in sequence to toluene (1.8 cm3). The resultant mixture was stirred at room temperature for 10 min and then added to a stirred mixture of rac-LA (0.2883 g, 2.00 mmol) and toluene (2.0 cm3) at the same temperature. The polymerization reaction was terminated after 10 min by adding a drop of water. White precipitates were filtered under reduced pressure and washed with hexane. Drying the wet cake under vacuum gave the polymer as white solid. For GPC analysis, the sample was dissolved in THF, passed through a short neutral aluminum oxide column, precipitated in methanol, and dried under vacuum.
Acknowledgements
We thank the Foundation for Talents of Anhui Province (Grant No. 2008Z011) for financial support. We also thank Professor D.-Q. Wang for the crystal structure determination.
Notes and references
-
(a) H. Seyednejad, A. H. Ghassemi, C. F. van Nostrum, T. Vermonden and W. E. Hennink, J. Controlled Release, 2011, 168, 152 Search PubMed
;
(b) A. Arbaoui and C. Redshaw, Polym. Chem., 2010, 1, 801 RSC
;
(c) N. Ajellal, J.-F. Carpentier, C. Guillaume, S. M. Guillaume, M. Helou, V. Poirier, Y. Sarazin and A. Trifonov, Dalton Trans., 2010, 39, 8363 RSC
;
(d) M. H. Chisholm, Pure Appl. Chem., 2010, 82, 1647 CrossRef CAS
;
(e) M. Labet and W. Thielemans, Chem. Soc. Rev., 2009, 38, 3484 RSC
;
(f) R. H. Platel, L. M. Hodgson and C. K. Williams, Polym. Rev., 2008, 48, 11 CrossRef CAS
;
(g) V. R. Sinha, K. Bansal, R. Kaushik, R. Kumria and A. Trehan, Int. J. Pharm., 2004, 278, 1 CrossRef CAS PubMed
;
(h) D. Mecerreyes, R. Jerôme and P. Dubois, Adv. Polym. Sci., 1999, 147, 1 CrossRef CAS
;
(i) B. J. O'Keefe, M. A. Hillmyer and W. B. Tolman, Dalton Trans., 2001, 2215 RSC
;
(j) K. M. Stridsberg, M. Ryner and A.-C. Albertsson, Adv. Polym. Sci., 2002, 157, 41 CrossRef CAS
;
(k) E. Chiellini and R. Solaro, Adv. Mater., 1996, 8, 305 CrossRef CAS
. -
(a) M. J.-L. Tschan, E. Brulé, P. Haquette and C. M. Thomas, Polym. Chem., 2012, 3, 836 RSC
;
(b) J. Wu, T.-L. Yu, C.-T. Chen and C.-C. Lin, Coord. Chem. Rev., 2006, 250, 602 CrossRef CAS
;
(c) C. A. Wheaton, P. G. Hayes and B. J. Ireland, Dalton Trans., 2009, 4832 RSC
. -
(a) H. B. Wang, J. Guo, Y. Yang and H. Ma, Dalton Trans., 2016, 45, 10942 RSC
;
(b) S. Ghosh, P. K. S. Antharjanam and D. Chakraborty, Polymer, 2015, 70, 38 CrossRef CAS
;
(c) S. Ghosh, D. Chakraborty and V. Ramkumar, J. Polym. Sci., Part A: Polym. Chem., 2015, 53, 1474 CrossRef CAS
;
(d) M. Honrado, A. Otero, J. Fernandez-Baeza, L. F. Sanchez-Barba, A. Garces, A. Lara-Sanchez, J. Martinez-Ferrer, S. Sobrino and A. M. Rodriguez, Organometallics, 2015, 34, 3196 CrossRef CAS
;
(e) Y. Sarazin, B. Liu, T. Roisnel, L. Maron and J.-F. Carpentier, J. Am. Chem. Soc., 2011, 133, 9069 CrossRef CAS PubMed
;
(f) M. H. Chisholm, K. Choojun, J. C. Gallucci and P. M. Wambua, Chem. Sci., 2012, 3, 3445 RSC
;
(g) C.-Y. Sung, C.-Y. Li, J.-K. Su, T.-Y. Chen, C.-H. Lin and B.-T. Ko, Dalton Trans., 2012, 41, 953 RSC
;
(h) S. Song, H. Ma and Y. Yang, Dalton Trans., 2013, 42, 14200 RSC
;
(i) R. A. Collins, J. Unruangsri and P. Mountford, Dalton Trans., 2013, 42, 759 RSC
;
(j) Y. Huang, W. Wang, C.-C. Lin, M. P. Blake, L. Clark, A. D. Schwarz and P. Mountford, Dalton Trans., 2013, 42, 9313 RSC
;
(k) Y. Gao, Z.-G. Dai, J.-J. Zhang, X.-X. Ma, N. Tang and J.-C. Wu, Inorg. Chem., 2014, 53, 716 CrossRef CAS PubMed
;
(l) B. Gao, D.-H. Zhao, X. Li, Y. Cui, R.-L. Duan and X. Pang, RSC Adv., 2015, 5, 440 RSC
;
(m) T. J. J. Whitehorne, B. Vabre and F. Schaper, Dalton Trans., 2014, 43, 6339 RSC
. -
(a) D. J. Darensbourg, W. Choi, O. Karroonnirun and N. Bhuvanesh, Macromolecules, 2008, 41, 3493 CrossRef CAS
;
(b) M.-W. Hsiao and C.-C. Lin, Dalton Trans., 2013, 42, 2041 RSC
;
(c) M. G. Cushion and P. Mountford, Chem. Commun., 2011, 47, 2276 RSC
;
(d) J. P. Davin, J. C. Buffet, T. P. Spaniol and J. Okuda, Dalton Trans., 2012, 41, 12612 RSC
. -
(a) P. McKeown, M. G. Davidson, G. Kociok-Köhnb and M. D. Jones, Chem. Commun., 2016, 52, 10431 RSC
;
(b) Z. Sun, R. Duan, J. Yang, H. Zhang, S. Li, X. Pang, W. Chen and X. Chen, RSC Adv., 2016, 6, 17531 RSC
;
(c) A. Pilone, N. D. Maio, K. Press, V. Venditto, D. Pappalardo, M. Mazzeo, C. Pellecchia, M. Kol and M. Lamberti, Dalton Trans., 2015, 44, 2157 RSC
;
(d) Y.-L. Hsieh, N. Huang, G.-H. Lee and C.-H. Peng, Polymer, 2015, 72, 281 CrossRef CAS
;
(e) H. Z. Du, A. H. Velders, P. J. Dijkstra, Z. Y. Zhong, X. S. Chen and J. Feijen, Macromolecules, 2009, 42, 1058 CrossRef CAS
;
(f) H.-L. Chen, S. Dutta, P.-Y. Huang and C.-C. Lin, Organometallics, 2012, 31, 2016 CrossRef CAS
;
(g) B. Gao, X. Li, R. L. Duan, Q. Duan, Y. H. Li, X. Pang, H. J. Zhuang and X.-S. Chen, RSC Adv., 2015, 5, 29412 RSC
;
(h) A. Pilone, K. Press, I. Goldberg, M. Kol, M. Mazzeo and M. Lamberti, J. Am. Chem. Soc., 2014, 136, 2940 CrossRef CAS PubMed
;
(i) Y. Cui, D. Li, B. Gao, Y. Zhou, L. Chen, B. Qiu, Y. Li, Q. Duan and N. Hu, J. Coord. Chem., 2016, 69, 4 Search PubMed
;
(j) B. Gao, D. Li, X. Li, R. Duan, X. Pang, Y. Cui, Q. Duan and X. Chen, Catal. Sci. Technol., 2015, 5, 4644 RSC
;
(k) B. Gao, R. Duan, X. Pang, X. Li, Z. Qu, Z. Tang, X. Zhuang and X. Chen, Organometallics, 2013, 32, 5435 CrossRef CAS
. -
(a) H. Wang, Y. Yang and H. Ma, Inorg. Chem., 2016, 55, 7356 CrossRef CAS PubMed
;
(b) M. J. Walton, S. J. Lancaster, J. A. Wright, M. R. J. Elsegood and C. Redshaw, Dalton Trans., 2014, 43, 18001 RSC
;
(c) M. Honrado, A. Otero, J. Fernández-Baeza, L. F. Sánchez-Barba, A. Garcés, A. Lara-Sáncheza and A. M. Rodrígueza, Dalton Trans., 2014, 43, 17090 RSC
;
(d) W.-A. Ma and Z.-X. Wang, Organometallics, 2011, 30, 4364 CrossRef CAS
;
(e) C. A. Wheaton and P. G. Hayes, Chem. Commun., 2010, 46, 8404 RSC
;
(f) P. L. Arnold, I. J. Casely, Z. R. Turner, R. Bellabarba and R. B. Tooze, Dalton Trans., 2009, 7236 RSC
;
(g) Y.-H. Tsai, C.-H. Lin, C.-C. Lin and B.-T. Ko, J. Polym. Sci., Part A: Polym. Chem., 2009, 47, 4927 CrossRef CAS
;
(h) C. K. Williams, L. E. Breyfogle, S. K. Choi, W. Nam, V. G. Young Jr, M. A. Hillmyer and W. B. Tolman, J. Am. Chem. Soc., 2003, 125, 11350 CrossRef CAS PubMed
;
(i) M. Cheng, A. B. Attygalle, E. B. Lobkowsky and G. W. Coates, J. Am. Chem. Soc., 1999, 121, 11583 CrossRef CAS
;
(j) B. M. Chamberlain, M. Cheng, D. R. Moore, T. M. Ovitt, E. B. Lobkovsky and G. W. Coates, J. Am. Chem. Soc., 2001, 123, 3229 CrossRef CAS PubMed
. -
(a) A. P. Dove, V. C. Gibson, E. L. Marshall, A. J. P. White and D. J. Williams, Chem. Commun., 2001, 283 RSC
;
(b) N. Nimitsiriwat, V. C. Gibson, E. L. Marshall and M. R. J. Elsegood, Inorg. Chem., 2008, 47, 5417 CrossRef CAS PubMed
;
(c) H. Y. Chen, H. J. Fang, Y. J. Chen, S. C. N. Hsu, Y. C. Lai, H. W. Ou, W. T. Peng, Y. J. Chang, Y. C. Tsou, T. Y. Wu, H. Chung, Y. Chen, T. C. Huang and B. S. Wu, J. Polym. Sci., Part A: Polym. Chem., 2012, 50, 3286 CrossRef CAS
;
(d) P. Degee, P. Dubois, S. Jacobsen, H. G. Fritz and R. Jerome, J. Polym. Sci., Part A: Polym. Chem., 1999, 37, 2413 CrossRef CAS
;
(e) M. Moller, R. Kange and J. L. Hedrick, J. Polym. Sci., Part A: Polym. Chem., 2000, 38, 2067 CrossRef CAS
;
(f) S. J. Moravek, J. M. Messman and R. F. Storey, J. Polym. Sci., Part A: Polym. Chem., 2009, 47, 797 CrossRef CAS
;
(g) X. C. Zhang, D. A. Macdonald, M. F. A. Goosen and K. B. McAuley, J. Polym. Sci., Part A: Polym. Chem., 1994, 32, 2965 CrossRef CAS
;
(h) A. P. Dove, V. C. Gibson, E. L. Marshall, H. S. Rzepa, A. J. P. White and D. J. Williams, J. Am. Chem. Soc., 2006, 128, 9834 CrossRef CAS PubMed
;
(i) N. Nimitsiriwat, E. L. Marshall, V. C. Gibson, M. R. J. Elsegood and S. H. Dale, J. Am. Chem. Soc., 2004, 126, 13598 CrossRef CAS PubMed
;
(j) K. B. Aubrecht, M. A. Hillmyer and W. B. Tolman, Macromolecules, 2002, 35, 644 CrossRef CAS
;
(k) M. Lahcini, P. M. Castro, M. Kalmi, M. Leskela and T. Repo, Organometallics, 2004, 23, 4547 CrossRef CAS
;
(l) V. Poirier, T. Roisnel, S. Sinbandhit, M. Bochmann, J. F. Carpentier and Y. Sarazin, Chem.–Eur. J., 2012, 18, 2998 CrossRef CAS PubMed
. -
(a) A. Sauer, A. Kapelski, C. Fliedel, S. Dagorne, M. Kol and J. Okuda, Dalton Trans., 2013, 42, 9007 RSC
;
(b) B. Gao, X. Li, R. L. Duan and X. Pang, New J. Chem., 2015, 39, 2404 RSC
;
(c) B. Gao, Q. Duan, Y. H. Li, D. N. Li, L. Q. Zhang, Y. Cui, N. H. Hu and X. Pang, RSC Adv., 2015, 5, 13437 RSC
. -
(a) M. D. Jones, S. L. Hancock, P. McKeown, P. M. Schäfer, A. Buchard, L. H. Thomas, M. F. Mahon and J. P. Lowe, Chem. Commun., 2014, 50, 15967 RSC
;
(b) A. Stopper, J. Okuda and M. Kol, Macromolecules, 2012, 45, 698 CrossRef CAS
. -
(a) M. Zhang, X. Ni and Z. Shen, Organometallics, 2014, 33, 6861 CrossRef CAS
;
(b) L. Clark, M. G. Cushion, H. E. Dyer, A. D. Schwarz, R. Duchateau and P. Mountford, Chem. Commun., 2010, 46, 273 RSC
;
(c) L. Clark, G. B. Deacon, C. M. Forsyth, P. C. Junk, P. Mountford, J. P. Townley and J. Wang, Dalton Trans., 2013, 42, 9294 RSC
;
(d) H. E. Dyer, S. Huijser, N. Susperregui, F. Bonnet, A. D. Schwarz, R. Duchateau, L. Maron and P. Mountford, Organometallics, 2010, 29, 3602 CrossRef CAS
;
(e) S. Yang, K. Nie, Y. Zhang, M.-Q. Xue, Y.-M. Yao and Q. Shen, Inorg. Chem., 2014, 53, 105 CrossRef CAS PubMed
;
(f) J.-S. Qiu, M. Lu, Y.-M. Yao, Y. Zhang, Y.-R. Wang and Q. Shen, Dalton Trans., 2013, 42, 10179 RSC
;
(g) X.-D. Shen, M.-Q. Xue, R. Jiao, Y. Ma, Y. Zhang and Q. Shen, Organometallics, 2012, 31, 6222 CrossRef CAS
;
(h) W.-J. Zhang, S.-F. Liu, W.-H. Yang, X. Hao, R. Glaser and W.-H. Sun, Organometallics, 2012, 31, 8178 CrossRef CAS
;
(i) W.-J. Zhang, S.-F. Liu, W.-H. Sun, X. Hao and C. Redshaw, New J. Chem., 2012, 36, 2392 RSC
;
(j) M. Bouyahyi, N. Ajellal, E. Kirillov, C. M. Thomas and J. F. Carpentier, Chem.–Eur. J., 2011, 17, 1872 CrossRef CAS PubMed
;
(k) E. Grunova, E. Kirillov, T. Roisnel and J. F. Carpentier, Dalton Trans., 2010, 39, 6739 RSC
;
(l) J. C. Buffet, A. Kapelski and J. Okuda, Macromolecules, 2010, 43, 10201 CrossRef CAS
;
(m) T. Cao, A. Buchard, X. F. Le Goff and C. K. Williams, Inorg. Chem., 2012, 51, 2157 CrossRef CAS PubMed
;
(n) C. Bakewell, T. Cao, N. Long, X. F. Le Goff, A. Auffrant and C. K. Williams, J. Am. Chem. Soc., 2012, 134, 20577 CrossRef CAS PubMed
;
(o) S. Sun, K. Nie, Y. F. Tan, B. Zhao, Y. Zhang, Q. Shen and Y.-M. Yao, Dalton Trans., 2013, 42, 2870 RSC
;
(p) Y. Cui, W. Gu, Y. Wang, B. Zhao, Y. Yao and Q. Shen, Catal. Sci. Technol., 2015, 5, 3302 RSC
;
(q) Y.-L. Duan, J.-X. He, W. Wang, J.-J. Zhou, Y. Huang and Y. Yang, Dalton Trans., 2016, 45, 10807 RSC
. -
(a) A. K. Sutar, T. Maharana, S. Dutta, C.-T. Chen and C.-C. Lin, Chem. Soc. Rev., 2010, 39, 1724 RSC
;
(b) F. M. García-Valle, R. Estivill, C. Gallegos, T. Cuenca, M. E. G. Mosquera, V. Tabernero and J. Cano, Organometallics, 2015, 34, 477 CrossRef
;
(c) E. Kober, R. Petrus, P. Kociecka, Z. Janas and P. Sobota, Polyhedron, 2015, 85, 814 CrossRef CAS
;
(d) Y. Huang, Y.-H. Tsai, W.-C. Hung, C.-S. Lin, W. Wang, J.-H. Huang, S. Dutta and C.-C. Lin, Inorg. Chem., 2010, 49, 9416 CrossRef CAS PubMed
;
(e) L. Wang, X. Pan, L. Yao, N. Tang and J. Wu, Eur. J. Inorg. Chem., 2011, 632 CrossRef
;
(f) B. Calvo, M. G. Davidson and D. García-Vivó, Inorg. Chem., 2011, 50, 3589 CrossRef CAS PubMed
;
(g) W.-Y. Lu, M.-W. Hsiao, S. C. N. Hsu, W.-T. Peng, Y.-J. Chang, Y.-C. Tsou, T.-Y. Wu, Y.-C. Lai, Y. Chen and H.-Y. Chen, Dalton Trans., 2012, 41, 3659 RSC
;
(h) J. Zhang, C. Jian, Y. Gao, L. Wang, N. Tang and J. Wu, Inorg. Chem., 2012, 51, 13380 CrossRef CAS PubMed
;
(i) H.-Y. Chen, L. Mialon, K. A. Abboud and S. A. Miller, Organometallics, 2012, 31, 5252 CrossRef CAS
;
(j) R. K. Dean, A. M. Reckling, H. Chen, L. N. Dawe, C. M. Schneider and C. M. Kozak, Dalton Trans., 2013, 42, 3504 RSC
;
(k) S.-C. Roşca, D.-A. Roşca, V. Dorcet, C. M. Kozak, F. M. Kerton, J.-F. Carpentier and Y. Sarazin, Dalton Trans., 2013, 42, 9361 RSC
;
(l) C. Thomas, A. Milet, F. Peruch and B. Bibal, Polym. Chem., 2013, 4, 3491 RSC
;
(m) D. Alhashmialameer, N. Ikpo, J. Collins, L. N. Dawe, K. Hattenhauera and F. M. Kerton, Dalton Trans., 2015, 44, 20216 RSC
;
(n) C. Gallegos, V. Tabernero, M. E. G. Mosquera, T. Cuenca and J. Cano, Eur. J. Inorg. Chem., 2015, 5124 CrossRef CAS
;
(o) F. M. García-Valle, R. Estivill, C. Gallegos, T. Cuenca, M. E. G. Mosquera, V. Tabernero and J. Cano, Organometallics, 2015, 34, 477 CrossRef
;
(p) H.-W. Ou, K.-H. Lo, W.-T. Du, W.-Y. Lu, W.-J. Chuang, B.-H. Huang, H.-Y. Chen and C.-C. Lin, Inorg. Chem., 2016, 55, 1423 CrossRef CAS PubMed
;
(q) Q. Zhang, W. Zhang, S. Wang, G. A. Solan, T. Liang, N. M. Rajendrana and W.-H. Sun, Inorg. Chem. Front., 2016, 3, 1178 RSC
;
(r) W.-J. Chuang, Y.-T. Huang, Y.-H. Chen, Y.-S. Lin, W.-Y. Lu, Y.-C. Lai, M. Y. Chiang, S. C. N. Hsu and H.-Y. Chen, RSC Adv., 2016, 6, 33014 RSC
. -
(a) J.-J. Zhang, J. Xiong, Y.-Y. Sun, N. Tang and J.-C. Wu, Macromolecules, 2014, 47, 7789 CrossRef CAS
;
(b) J. Xiong, J.-J. Zhang, Y.-Y. Sun, Z.-R. Dai, X.-B. Pan and J.-C. Wu, Inorg. Chem., 2015, 54, 1737 CrossRef CAS PubMed
;
(c) Z.-R. Dai, Y.-Y. Sun, J. Xiong, X.-B. Pan and J.-C. Wu, ACS Macro Lett., 2015, 4, 556 CrossRef CAS
;
(d) Z. R. Dai, Y.-Y. Sun, J. Xiong, X.-B. Pan, N. Tang and J.-C. Wu, Catal. Sci. Technol., 2016, 6, 515 RSC
;
(e) Y.-Y. Sun, J. Xiong, Z.-R. Dai, X.-B. Pan, N. Tang and J.-C. Wu, Inorg. Chem., 2016, 55, 136 CrossRef CAS PubMed
;
(f) Y.-Q. Cui, C.-J. Chen, Y.-Y. Sun, J.-C. Wu and X.-B. Pan, Inorg. Chem. Front., 10.1039/c6qi00449k;
(g) Y.-G. Li, H.-W. Zhao, X.-Y. Mao, X.-B. Pan and J.-C. Wu, Dalton Trans., 2016, 45, 9636 RSC
;
(h) C.-J. Chen, Y.-Q. Cui, X.-Y. Mao, X.-B. Pan and J.-C. Wu, Macromolecules, 2017, 50, 83 CrossRef CAS
. -
(a) D. L. Hughes and M. R. Truter, J. Chem. Soc., Dalton Trans., 1979, 520 RSC
;
(b) P. B. Hitchcock, M. F. Lappert and D.-S. Liu, J. Organomet. Chem., 1995, 488, 241 CrossRef CAS
;
(c) A. R. Kennedy, R. E. Mulvey, R. I. Urquhart and S. D. Robertson, Dalton Trans., 2014, 43, 14265 RSC
;
(d) R. Travieso-Puente, M.-C. Chang and E. Otten, Dalton Trans., 2014, 43, 18035 RSC
. - L. N. Saunders, L. N. Dawe and C. M. Kozak, J. Organomet. Chem., 2014, 749, 34 CrossRef CAS
. - M. Save, M. Schappacher and A. Soum, Macromol. Chem. Phys., 2002, 203, 889 CrossRef CAS
. - G. M. Sheldrick, Acta Crystallogr., Sect. A: Found. Crystallogr., 1990, 46, 467 CrossRef
. - G. M. Sheldrick, SHELXL97: Programs for Structure Refinement, Universität Göttingen, Göttingen, Germany, 1997 Search PubMed
.
Footnote |
† Electronic supplementary information (ESI) available: MALDI-TOF mass spectrum and 1H NMR spectrum of the polymer. CCDC 1509154. For ESI and crystallographic data in CIF or other electronic format see DOI: 10.1039/c7ra01186e |
|
This journal is © The Royal Society of Chemistry 2017 |
Click here to see how this site uses Cookies. View our privacy policy here.