DOI:
10.1039/C7RA01108C
(Paper)
RSC Adv., 2017,
7, 22640-22648
Evaluation of the protective effects of 13 traditional Chinese medicine compounds on ionizing radiation injury: bupleurum, shenmai, and breviscapine as candidate radioprotectors
Received
25th January 2017
, Accepted 15th April 2017
First published on 26th April 2017
Abstract
Background: Drugs are being sought that are effective as radioprotection for use in both planned and unplanned radiation exposure. Traditional Chinese medicines have been used successfully for the treatment of free radical-mediated diseases and therefore we expected that exploring such agents could yield an effective radioprotector. We therefore evaluated the radioprotective effects of 13 traditional Chinese medicine compounds (TCMCs) against ionizing radiation damage. Materials and methods: TCMCs were tested in vitro on human kidney 293T cells treated with 0, 2, 4, 6, 8 or 10 Gy irradiation for their ability to promote cell survival. Mice were subjected to a sub-lethal (4 Gy), lethal (7 Gy) or supra-lethal (13 Gy) dose of total-body irradiation (TBI) and TCMCs were tested for their ability to influence survival and hemopoietic function. Results: Of the 13 TCMCs tested, five including bupleurum, shenmai, and breviscapine, promoted survival of 293T cells. bupleurum, shenmai, and breviscapine also prolonged survival times of mice treated with supra-lethal and lethal TBI doses. As expected of an effective radioprotector, they also ameliorated bone marrow suppression, reducing the decrease in bone marrow cells after sub-lethal TBI, and they attenuated the TBI-induced reduction in granulocyte-macrophage colony-forming units. Conclusion: This investigation of TCMCs in vitro and in vivo identified bupleurum, shenmai, and breviscapine as having characteristics consistent with radioprotective agents. Further investigation to identify the bioactive components responsible for the radioprotective effect of these compounds is now required.
1. Introduction
Over the past decades, scientists worldwide have been searching for drugs that are effective in radioprotection and in radiorecovery for potential application during both planned (e.g., radiotherapy) and unplanned (e.g., nuclear accident, warfare, occupational) radiation exposure.1 An ideal radioprotector needs to meet prerequisite characteristics, such as having no cumulative or irreversible toxicity, an effective and definitive effect, stability (a 2–5 year shelf-life), and an easy administration route.2 So far, no single agent meets all the requirements. Amifostine (trade name Ethyol) is the only radioprotector that has been approved for use clinically, for the radiotherapy of head and neck cancer, despite its drawbacks of short term, and severe side effects (e.g., nausea, vomiting, diarrhea, hypotension, hypo-calcemia, nephro- and neuro-toxicity). Thus, there is an urgent need in radiation medicine for radioprotective agents with less toxicity and greater efficacy, and the quest to find them is ongoing.
A number of traditional Chinese medicines (TCMs) have been used successfully for the treatment of free radical-mediated diseases such as rheumatoid arthritis, atherosclerosis, inflammatory diseases, and ischemia/reperfusion damage.3,4 It is therefore reasonable to expect that there is a potential single or combination of different radiomodifiers that would be successful against ionizing radiation injury. The present study attempts to evaluate the potential radioprotective efficacy of 13 traditional Chinese medicine compounds (TCMCs) via cell culture, and animal models of total-body irradiation (TBI) in the sub-to supra-lethal range.
2. Materials and methods
2.1 Experimental materials
Detailed information on the 13 TCMCs are listed in Table 1. All are herbal products exhibiting anti-inflammatory properties or anti-microbial effects. Drug dosages for use in the experiments were selected according to those already used in clinical applications; the human to mouse equivalent dose rate was calculated according to body surface area.5 Medicines with single active ingredient compound use doses in mg per kg per day and others with complex active ingredients in mL per kg per day. Different doses of TCMCs dissolved in cell culture medium (for use in vitro) or saline (for use in vivo) were freshly prepared prior to each experiment. Unless otherwise specified, all of the chemicals used in this study were obtained from Sigma Aldrich (St Louis, MO, USA). Prior to use, stock solutions were stored at 4 °C in the dark. Radiation exposure was performed using a Gammacell-40 137Cs irradiator (Atomic Energy of Canada Ltd., Ottawa, ON, Canada) delivering a dose rate of 0.78 Gy min−1.
Table 1 Attributes of tested traditional Chinese medicine compounds
No. |
Generic drug name |
Derived from |
Manufacturer |
Drug approval number (state medical permitment no.) |
Product batch number |
Linear regression equationa |
IC10 for 293T (μL mL−1 medium) |
Note x, y values respectively stands for log(concentration, expressed as μl ml−1 medium) and cell survival rate. |
1 |
Bupleurum injection |
Bupleurum chinense DC. |
Henan Province Kang Hua Pharmaceutical Co., Ltd |
Z41021736 |
13102201 |
y = −0.4412x + 1.159, R2 = 0.9595 |
3.86 |
2 |
Danshen injection |
Radix Salvia miltiorrhiza |
Yibin Wuliangye Group Yibin Pharmaceutical Co., Ltd |
Z51020167 |
14030411 |
y = −0.5642x + 1.1331, R2 = 0.9841 |
2.65 |
3 |
Shenmai injection |
Red ginseng, Liriope (plant) |
Hebei Shenwei Pharmaceutical Co., Ltd |
Z13020888 |
13041222 |
y = −0.4951x + 1.1226, R2 = 0.9735 |
4.61 |
4 |
Herba Houttuyniae injection |
Houttuynia cordata |
Henan Province Kang Hua Pharmaceutical Co., Ltd |
Z41021744 |
13022801 |
y = −0.5734x + 1.0431, R2 = 0.9248 |
1.78 |
5 |
Xiangdan injection |
Radix salvia miltiorrhiza, Dalbergia odorifera |
Kang pharmaceutical Jiangsu Co., Ltd. |
Z32020680 |
131222 |
y = −0.512x + 1.0513, R2 = 0.9899 |
1.97 |
6 |
Radix isatidis injection |
Isatis tinctoria |
Shanxi Tianyuan Pharmaceutical Co., Ltd. |
Z14020774 |
1308211 |
y = −0.5475x + 0.9707, R2 = 0.96 |
1.35 |
7 |
Breviscapine injection |
Breviscapine |
Shiyao Yinhu Pharmaceutical Co., Ltd |
Z14021942 |
1011402102 |
y = −0.4701x + 1.1951, R2 = 0.9503 |
4.24 |
8 |
Chuanxinlian injection |
Andrographis paniculata |
Henan Province Kang Hua Pharmaceutical Co., Ltd |
Z41021725 |
13121702 |
y = −0.5393x + 1.0271, R2 = 0.9817 |
1.72 |
9 |
Shuanghuanglian injection |
Lonicera japonica, Scutellaria baicalensis Georgi, Forsythia suspensa (Thunb.) Vahl |
Henan Fussen Pharmaceutical Co. Ltd. |
Z41020750 |
13122111 |
y = −0.4668x + 0.834, R2 = 0.956 |
0.72 |
10 |
Safflower injection |
Flos carthami |
Shineway Pharmaceutical Group Co. Ltd. |
Z13020782 |
130531A2 |
y = −0.6026x + 1.1405, R2 = 0.9814 |
2.51 |
11 |
Anti-hepatitis-jaundice injection |
Capillary artemisia, Cape jasmine fruit, Baicalin, Honey suchle Flowers extract |
Shineway Pharmaceutical Group Co. Ltd. |
Z13020772 |
12121544 |
y = −0.5462x + 1.1316, R2 = 0.9917 |
2.65 |
12 |
Salvianolate injection |
Salvianolate |
Shanghai Green Valley Pharmaceutical Co., Ltd. |
Z20050247 |
13021628 |
y = −0.471x + 1.16222.24, R2 = 0.9924 |
3.60 |
13 |
Sulfotanshinone sodium injection |
Sulfotanshinone sodium |
SPH No.1 Biochemical & Pharmaceutical Co., Ltd. |
H31022558 |
130805 |
y = −0.5314x + 1.086, R2 = 0.9693 |
2.24 |
2.2 Cell culture and MTT assay
The human embryonic kidney cell-derived cell line 293T was obtained from the American Type Culture Collection (Rockville, MD, USA). Cells were cultured in basic DMEM supplemented with 10% fetal bovine serum and 100 units per mL penicillin and streptomycin antibiotic mixture at 37 °C in a humidified 5% CO2 atmosphere. Cell growth inhibition was determined using the colorimetric 3-(4,5-dimethyl-2-thiazolyl)-2,5-diphenyl-2-H-tetrazolium bromide (MTT) assay, which relies on the change from yellow MTT to purple formazan crystals that occurs in the presence of living cells. The assay was conducted in a 96-well plate on 8 × 103 cells per 200 μL per well. Cells were incubated for 24 h, after which the medium was removed and replaced with fresh medium containing the test compound, TCMCs were serially diluted with cell culture medium, followed by an incubation period of 24 or 48 h. Cells were then incubated with MTT solution (0.5 mg mL−1) for 4 h, carefully remove the supernatant with a pipette leaving behind the precipitate, and then 150 μL of dimethyl sulfoxide was added to each well to dissolve formazan precipitate. The absorbance (A) of each well (six parallel wells for each sample) was then measured at 570 nm using a Synergy HT Multi-Mode Microplate Reader (BioTek, Winooski, VT, USA). The percentage of cells surviving was calculated using the following formula: cell survival rate (%) = [(Atest − Ablank/Anegative control − Ablank)] × 100. These experiments were conducted in triplicate. A graph was constructed by linear regression analysis of the cell survival rate against the log concentration (expressed as μL mL−1 medium) of the tested compound, which was used to determine the concentration of compound that inhibits 10% of cells (IC10; data not shown). Drug concentration at an inhibition rate of 10%, which was considered not to affect cell proliferation and growth, we selected the half IC10 concentration considering its toxicity and negative effects on cells were negligible. TCMC was subsequently used as the single dose for further experiments on 293T cells detailed below.
2.3 Colony formation assay
Colony formation assays were conducted with half IC10 concentrations of each TCMC to test the protective effect of each compound. Cells were seeded into 12-well plates at a density of 1000 cells/well/2 mL cultured for 24 h to allow them to stabilize, then TCMCs were added separately followed by 12 h culture. Cells were then treated with a range of ionizing irradiation (IR) doses (0, 2, 4, 6, 8 or 10 Gy). After incubating these cells at 37 °C for 14 days, they were washed twice with phosphate buffered saline (PBS) and stained with 0.005% (w/v) crystal violet in 25% (v/v) methanol resulted in best staining/background. Using an inverted light microscope to examine the plate wells, the number of colonies containing ≥50 cells were counted, and the number used to calculate: the plating efficiency (PE)
=
(colonies counted/cells seeded) × 100% and the survival fraction (SF)= (colonies counted)/((cells seeded) × (PE/100)). GraphPad Prism 5 (GraphPad Software, LaJolla, CA, USA) was used to fit the cell survival curve to a standard linear-quadratic (LQ) model.6 The linear-quadratic (LQ) model of radiation effect was used to provides a satisfactory and practically useful explanation of fractionation and dose – rate effects, which can be applied to both tumor and normal tissues. Thereafter, we obtained the values of the LD50 (median lethal dose) of each compound according to this model.
2.4 Animal grouping, modeling, and administration
Male C57/BL mice were obtained from the Beijing Vital River Laboratory Animal Technology Co., Ltd. and housed in the certified animal facility at the Institute of Radiation Medicine of the Chinese Academy of Medical Sciences (CAMS) in a specific-pathogen-free grade animal facility under standard conditions (temperature of 22 ± 2 °C, 12/12 h light/dark cycle, and humidity of 50 ± 10%) with free access to water. Mice (10–12 weeks old; weighing 22–25 g) were divided randomly into three groups (n = 10 in each group): (a) sham-irradiated controls (SI control), receive no saline or TCMC, were placed in identical containers as the TBI groups for the same period without irradiation (b) TBI + saline, and (c) TBI + TCMC. The mice in the TBI + TCMC group were injected intraperitoneally with different doses (see Table 3) of TCMC 30 min before and immediately after IR, and were then maintained on the same dose once per day for seven days after IR. The TBI + saline group received the same volume of physiological saline instead of TCMC at the same frequency and duration as the TBI + TCMC group. In the survival assays, mice exposed to a supra-lethal dose of 13 Gy or a lethal dose of 7 Gy TBI were returned to the animal facility for daily observation and treatment as indicated. Treated mice were observed twice or three times daily for a period of 30 days and their body weight and survival condition recorded. The mice that received a sub-lethal dose of 4 Gy TBI were sacrificed two days after the seven-day treatment period and specimens were collected. All experiments were performed in compliance with Guidelines of Animal Experiments from Medical Ethics Committee, National Health Department of China, and were approved by the Animal Use Ethics Committee of CAMS.
Table 2 Nonlinear regression parameters according to linear-quadratic modela
Group |
IR |
IR + 1 |
IR + 2 |
IR + 3 |
IR + 4 |
IR + 5 |
IR + 6 |
IR + 7 |
IR + 8 |
IR + 9 |
IR + 10 |
IR + 11 |
IR + 12 |
IR + 13 |
Note: SF = exp(−αx − βx^2) according to linear-quadratic model, SF means survival fraction; x means radiation dose; α/β has positive correlation with radiosensitivity. Lower radiosensitivity compared with IR group. |
Best-fit values |
α |
0.1041 |
0.0555 |
0.2270 |
0.0598 |
0.0506 |
0.2621 |
0.4416 |
0.0750 |
0.4015 |
0.3142 |
0.3051 |
0.3736 |
0.2519 |
0.0543 |
β |
0.0163 |
0.0132 |
0.0181 |
0.0132 |
0.0143 |
0.0248 |
0.0095 |
0.0127 |
0.0004 |
0.0183 |
0.0159 |
0.0056 |
0.0183 |
0.0202 |
R2 |
0.9862 |
0.9951 |
0.9944 |
0.948 |
0.9825 |
0.9984 |
0.997 |
0.9754 |
0.9932 |
0.9967 |
0.994 |
0.9994 |
0.9961 |
0.9841 |
α/β |
6.40 |
4.22b |
12.52 |
4.53b |
3.55b |
10.58 |
46.49 |
5.90b |
1120.88 |
17.22 |
19.15 |
66.98 |
13.79 |
2.69b |
LD50 (Gy) |
4.07 |
5.45b |
2.54 |
5.33b |
5.42b |
2.19 |
1.52 |
5.00b |
1.72 |
1.98 |
2.05 |
1.81 |
2.35 |
4.67b |
Table 3 Drug doses applied in each TBI experiments
Generic drug name |
4 Gy TBI |
7 Gy TBI |
13 Gy TBI |
Dose 1 |
Dose 2 |
Bupleurum injection |
200 mg per kg per day |
400 mg per kg per day |
200 mg per kg per day |
200 mg per kg per day |
Shenmai injection |
10 mL per kg per day |
20 mL per kg per day |
10 mL per kg per day |
10 mL per kg per day |
Herba houttuyniae injection |
NA |
NA |
0.9 mL per kg per day |
0.9 mL per kg per day |
Breviscapine injection |
6 mg per kg per day |
12 mg per kg per day |
6 mg per kg per day |
6 mg per kg per day |
Sulfotanshinone sodium injection |
NA |
NA |
2.4 mL per kg per day |
2.4 mL per kg per day |
2.5 Specimen collection
Nine days after sub-lethal TBI, peripheral blood (100 μL) was obtained from the orbital sinus using a micro-pipette coated with K3EDTA anticoagulant. A Celltac Es hemocytometer (Nihon Kohden, Japan) was used to count white blood cells (WBCs) red blood cells (RBCs) and platelets (PLT), and measure hemoglobin (HGB) and RBC-specific volume (HCT), in each peripheral blood sample. Bone marrow (BM) cells were flushed from the femurs of the sacrificed mice with PBS, and bone marrow mononucleated cells (BMMNCs) were counted using the Celltac Es hemocytometer and expressed as number of cells per femur.
2.6 Hematopoietic colony-forming unit assay
Hematopoietic colony-forming unit assays were conducted by culturing BMMNCs in MethoCult GF M3534 methylcellulose medium (StemCell Technologies, Vancouver, BC, Canada) for 5–7 days; colonies of granulocyte-macrophage cells (CFU-GM) with >30 cells were counted.
2.7 Statistical analysis
Data are presented as the mean ± standard error of the mean (SEM). Survival for each group was estimated using Kaplan–Meier survival curves and differences in the survival curves among different groups were evaluated using the log-rank test. Differences in blood cell counts between groups were analyzed using one-way ANOVA and the Mann–Whitney U test. A p-value of <0.05 was considered statistically significant. All tests were two-tailed. All analyses were performed using GraphPad Prism Software and IBM SPSS Statistics version 22.0.
3. Results
3.1 TCMC IC10 values for 293T cells
The toxicity of the 13 TCMCs was determined in 293T cells by analyzing the IC10 values, the experimental standard of no-observed-effect concentration. Linear regression analysis was performed to estimate the relationship between the cell survival rate (y-value), and the log concentration of the tested compound (x-value); the goodness-of-fit values (R2) ranged from 0.92–0.99, and are shown with the linear regression equations for each compound in Table 1. The resulting equations were used to determine the IC10 values, which ranged from 0.72–4.61 μL mL−1 medium, with the highest and lowest toxicity being caused by Shuanghuanglian (no. 9) and shenmai (no. 3), respectively (Table 1).
3.2 293T cell colony formation
To investigate the influence of TCMC treatment on cell radiosensitivity, 293T cells were treated with TCMC at the half IC10 concentration followed by 0, 2, 4, 6, 8 or 10 Gy IR and then cultured for 14 days. The SF data were fitted to a LQ model6 using the formula SF = exp(−αx − βx^2), where the x-value is the radiation dose. The parameters α and β are the best-fit values and were calculated by non-linear regression analysis; the α/β ratio positively correlates with radiosensitivity. A significantly lower α/β ratio than that resulting from IR alone was evident for cells treated with bupleurum (no.1), shenmai (no. 3), herba houttuyniae (no. 4), breviscapine (no. 7), and sulfotanshinone sodium (no. 13; Table 2). The non-linear regression curve (according to LQ model) of the SF at each IR dose in the presence of each TCMC, and the survival curve for cells treated with the five potentially radioprotective TCMC at each dose of IR are shown in Fig. 1a and b, respectively.
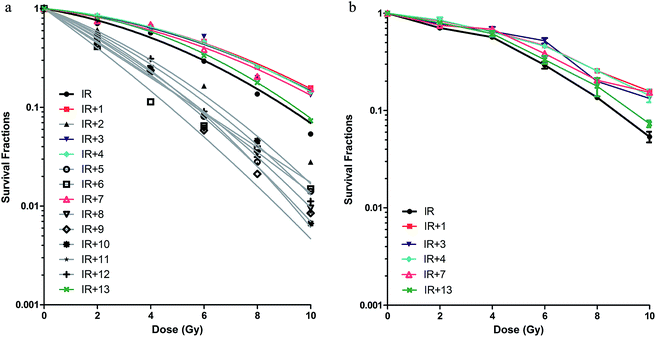 |
| Fig. 1 1 (Bupleurum injection), 3 (shenmai Injection), 4 (herba Houttuyniae injection), 7 (breviscapine injection), or 13 (sulfotanshinone sodium injection) treatment promotes colony formation ability of 293T cells after ionzing radiation. (a): nonlinear regression curve of 293T cells survival fractions treated with 0, 2, 4, 6, 8 or 10 Gy of gamma-ray according to Linear-quadratic model; (b): 1 (bupleurum injection), 3 (shenmai injection), 4 (herba houttuyniae injection), 7 (breviscapine injection), 13 (sulfotanshinone sodium injection) promotes survival fractions curve of radiated 293T cells. | |
3.3 Effect of TCMCs on survival of mice after lethal and supra-lethal TBI
To determine whether TCMCs had any radioprotective effect against supra-lethal and lethal doses of TBI in vivo, mice in the TCMC test groups were given TCMC (or TBI + saline) before, immediately after a supra-lethal dose of 13 Gy TBI, and for seven days afterwards, and the survival duration of the mice was monitored. Only data from TCMCs showing any positive effect are presented. Statistical differences between survival times of the TCMC-treated groups and the TBI + saline group were calculated using the log-rank test. The median survival times was 6 in the groups of mice treated with TBI + saline (control), while 8, 8, 7, 7.5 and 6 days in TBI + bupleurum (p = 0.0007), TBI + shenmai (p = 0.034), TBI + herba houttuyniae (p = 0.062), TBI + breviscapine (p = 0.032) and TBI + sulfotanshinone sodium (p = 0.543) groups, respectively. Next, we performed the same protocol but with a lethal dose of 7 Gy TBI and monitored the mice for up to 30 days after the end of the seven-day treatment period. The median survival times were 16.5, 24.5, 24.5, 24, 24.5 and 16.5 days with 30 day survival rates of 0, 50%, 50%, 50%, 50% and 20% in the TBI + saline (control), TBI + bupleurum (p = 0.036), TBI + shenmai (p = 0.024), TBI + herba houttuyniae (p = 0.064), TBI + breviscapine (p = 0.048), TBI + sulfotanshinone sodium (p = 0.55) groups, respectively (Fig. 2 and Tables 4 and 5).
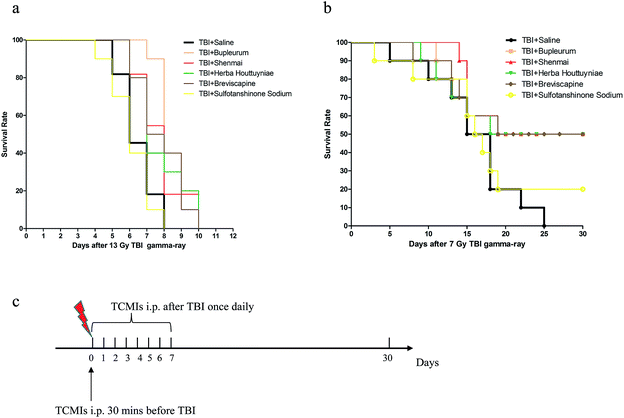 |
| Fig. 2 Survival curves after supralethal dose and lethal dose TBI. 1 (bupleurum injection), 3 (shenmai injection), 7 (breviscapine injection) prolonged survival time of mice exposured to supralethal (13.0 Gy) dose TBI ((a), p = 0.0007, 0.034, 0.062), increased the 30 day survival rate of mice exposured to lethal (7.0 Gy) dose TBI ((b), p = 0.036, 0.024, 0.048); (c) TBI and drug treatment. | |
Table 4 Comparison of survival curves after lethal dose 13 Gy TBI (n = 10)
|
Log-rank (Mantel–Cox) test |
sig difference? (p < 0.05 as significance) |
Chi square |
df |
p value |
Control vs. bupleurum |
11.410 |
1 |
0.0007 |
Yes |
Control vs. shenmai |
4.520 |
1 |
0.034 |
Yes |
Control vs. herba houttuyniae |
3.473 |
1 |
0.062 |
No |
Control vs. breviscapine |
4.620 |
1 |
0.032 |
Yes |
Control vs. sulfotanshinone sodium |
0.3702 |
1 |
0.543 |
No |
Table 5 Comparison of 30 day survival curves after 7 Gy TBI (n = 10)
|
Log-rank (Mantel–Cox) test |
Sig difference ? (p < 0.05 as significance) |
Chi square |
df |
p value |
Control vs. bupleurum |
4.405 |
1 |
0.036 |
Yes |
Control vs. shenmai |
5.086 |
1 |
0.024 |
Yes |
Control vs. herba houttuyniae |
3.440 |
1 |
0.064 |
No |
Control vs. breviscapine |
3.913 |
1 |
0.048 |
Yes |
Control vs. sulfotanshinone sodium |
0.353 |
1 |
0.55 |
No |
3.4 Effect of TCMCs on sub-lethal TBI-induced BM suppression in mice
Exposure to TBI usually induces peripheral blood count inhibition and BM damage.7,8 An effective radioprotector will rescue these parameters. Using the same treatment protocol as detailed for the supra-lethal TBI dose, we therefore evaluated the effects of TCMCs on hematopoietic parameters after exposure to a sub-lethal dose of 4 Gy TBI. Based on the results of the lethal and supra-lethal TBI experiments, we selected the top 3 positive drugs of the TCMCs with two doses of each (doses applied see Table 3) to evaluate their affection on BM suppression in mice. As shown in Table 5, levels of WBCs, RBCs, HGB, HCT and PLTs in the TBI + saline group were significantly lower than in the SI control group (p < 0.001). With TCMC treatment, WBCs, RBCs, HGB and HCT levels were increased but still remained lower than those of the SI controls. Compared to that of the TBI + saline group, the number of BMMNCs was significantly increased in the TBI + bupleurum (200 mg kg−1; p = 0.0012), TBI + shenmai (10 mL kg−1 and 20 mL kg−1; p = 0.049, p = 0.0038, respectively), and TBI + breviscapine (12 mg kg−1; p = 0.039) groups (Table 6, Fig. 3a).
Table 6 Peripheral blood cells and bone marrow mononucleated cells (BMMNCs) counts 9 days after 4.0 Gy TBIa
Group |
WBCs (109 L−1) |
RBCs (1012 L−1) |
HGB (g L−1) |
HCT (%) |
PLT (109 L−1) |
BMMNCs |
Note: ***p < 0.001 vs. the control group; **#p < 0.01 vs. the saline + TBI group; *#p < 0.05 vs. the saline + TBI group; datas are expressed as the means ± SEM (n = 10 for each group). |
Control |
8.55 ± 0.32 |
9.11 ± 0.16 |
129.40 ± 2.68 |
31.48 ± 0.58 |
549.00 ± 26.54 |
26.93 ± 1.11 |
TBI + saline |
0.61 ± 0.06*** |
7.09 ± 0.16*** |
102.67 ± 2.30*** |
24.23 ± 0.53*** |
108.67 ± 15.65*** |
7.55 ± 0.53*** |
TBI + Bupleurum 200 mg per kg per day |
0.94 ± 0.07*# |
6.03 ± 0.3**# |
85.20 ± 4.90**# |
20.92 ± 1.01*# |
134.40 ± 22.66 |
15.68 ± 0.67**# |
TBI + Bupleurum 400 mg per kg per day |
0.62 ± 0.05 |
6.82 ± 0.26**# |
98.40 ± 3.33 |
23.46 ± 0.71*# |
122.00 ± 22.79 |
8.72 ± 0.74 |
TBI + shenmai 10 mL per kg per day |
0.58 ± 0.07 |
7.21 ± 0.16 |
103.40 ± 2.42 |
24.38 ± 0.58 |
171.20 ± 29.24 |
10.24 ± 1.46*# |
TBI + shenmai 20 mL per kg per day |
1.00 ± 0.14*# |
8.98 ± 0.72*# |
100.80 ± 3.83 |
23.50 ± 0.62 |
208.80 ± 21.08**# |
12.84 ± 1.01**# |
TBI + breviscapine 6 mg per kg per day |
0.40 ± 0.05*# |
6.74 ± 0.22 |
96.60 ± 3.03 |
22.66 ± 0.67 |
77.20 ± 8.92 |
8.08 ± 1.23 |
TBI + breviscapine 12 mg per kg per day |
0.52 ± 0.10 |
6.90 ± 0.24 |
95.60 ± 3.20 |
22.88 ± 0.67 |
82.20 ± 11.43 |
9.68 ± 0.83*# |
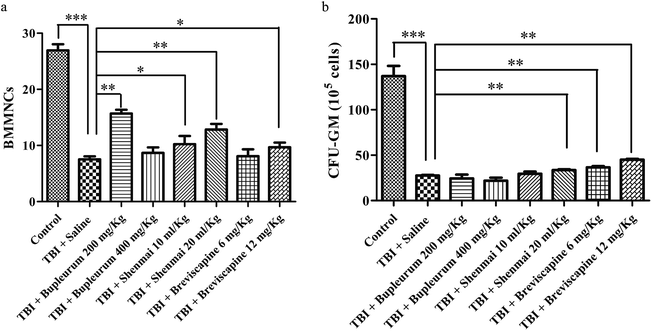 |
| Fig. 3 Bupleurum injection, shenmai injection, and breviscapine injection attenuate low dose TBI-induced BM suppression. (a) 1 (bupleurum injection, p = 0.0012, 0.27), 3 (shenmai injection, p = 0.049, 0.0038), 7 (breviscapine injection, p = 0.96, 0.039) ameliorates sublethal dose TBI-induced BMMNCs decrease. (b) 3 (shenmai injection, p = 0.89, 0.0026), 4 (herba houttuyniae injection, p = 0.0018, 0.0018) attenuated TBI-induced CFU-GM reduction. | |
Irradiation not only induces a reduction in hematopoietic cell number, but also causes functional inhibition of cells.7–9 As a measure of hematopoietic function, we therefore evaluated the effects of TCMC on CFU-GM. In comparison with the TBI + saline group, the TBI + shenmai (20 mL kg−1; p = 0.0026) and TBI + breviscapine (6 mg kg−1 and 12 mg kg−1; p = 0.0018, p = 0.0018, respectively) groups showed a significant increase in CFU-GMs (Fig. 3b).
These results suggest that the hematopoietic cell functions affected by IR can be partially restored by treatment with bupleurum, shenmai, or breviscapine.
4. Discussion
TCM has been used in China for more than two thousand years in the clinical treatment of a wide range of diseases including cerebrovascular diseases, stroke, amnesia, and dementia. In recent years China, several new compounds have been developed based on classical TCM formulae,3,10 for the treatment of, for example, stroke and inflammation.11,12 In addition, Chinese materia medica as well as active ingredients and components included in TCMCs, are being increasingly investigated,13–15 including for radioprotective effects.16 In the present study, the radioprotective activity of 13 selected TCMCs were evaluated, the TCMCs had been used in clinical practice for the treatment of influenza and malaria (bupleurum), heart disease (Danshen, Shenmai, Xiangdan, Salvianolate, Sulfotanshinone sodium), tumors (shenmai, synergistically with chemotherapy), stroke (breviscapine), and cerebrovascular disease (safflower), and as anti-oxidants and anti-inflammatory agents (Radix isatidis, Chuanxinlian, Shuanghuanglian, Anti-hepatitis-jaundice compound). Clinical trials of the TCMCs have shown that they are active pharmacologically as anti-oxidants, anti-inflammatory agents, and anti-microbials, and that they can act in isolation as well as in combination with other constituents.17–21 Our study, to the best of our knowledge, is the first to examine the therapeutic effects of these TCMCs on ionizing radiation damage in animal models.
4.1 Five TCMCs demonstrated radioprotective effect with no cytotoxicity
We first evaluated the cytotoxicity of TCMCs on 293T cells (Table 1) to determine the IC10 values and chose half IC10 as the appropriate concentration to investigate the cell response to different doses of IR exposure (Fig. 1). bupleurum injection, shenmai injection, herba houttuyniae injection, breviscapine injection, sulfotanshinone sodium injection demonstrated strong radioprotective effect without cytotoxicity. These five TCMCs have been used widely in clinical practice, bupleurum exhibits evident anti-inflammatory, antitumor, antiviral, anti-allergic, immunoregulation, and neuroregulation activities.22 Shenmai injection has been widely applied as an organ protector for cardio/cerebrovascular diseases, viral myocarditis, tumor chemotherapy, and adverse drug reactions.23 Although with some known adverse drug events and adverse drug reactions,24 herba houttuyniae has been proven to have heat-clearing, detoxifying and neuroprotective effects.25 Breviscapine injection has been extensively used in China since the 1970s for the treatment of ischemic cardiovascular and cerebrovascular diseases, such as angina pectoris, myocardial infarction and focal cerebral infarction.26 Sulfotanshinone sodium injection has been widely applied for its protective effect against angina pectoris27 and atherosclerotic lesions.28
4.2 TBI animal model
TBI animal model was established to further assess the radioprotective effect of the five TCMCs to assess radioprotective effect of these five TCMCs, TBI animal model was established, as described by Rosen et al.,29 TBI affects multiple organ systems or causes death, mainly via two mechanisms, gastrointestinal (GI) syndrome and hematopoietic syndrome. An effective radioprotector/mitigator should improve post-IR 30 day survival rates in rodents or humans by protecting against GI syndrome, hematopoietic syndrome, or both. The results from the present study showed that bupleurum, shenmai, and breviscapine effectively prolonged survival time of mice exposed to a supra-lethal (13 Gy) TBI dose, and improved the 30 day survival of mice exposed to a lethal (7 Gy) dose. The administration of each also attenuated sub-lethal TBI (4 Gy)-induced BM suppression, which was consistent with the results of the 293T cell colony formation assay. Evidence of hematopoietic protection was revealed by analysis of peripheral blood parameters after sub-lethal TBI. A significant increase in WBCs, RBCs, HGB and HCT was seen in bupleurum (200 mg kg−1)-treated mice compared with those treated with saline, and treatment with shenmai (20 mL kg−1) similarly affected WBCs, RBCs and HCT. While, compared to treatment with saline, shenmai and breviscapine both had significant positive effects on CFU-GM formation after sub-lethal IR.
4.3 Bupleurum, shenmai and breviscapine could be potentially used as potential radioprotectants
These data indicate that bupleurum, shenmai and breviscapine can act as effective radioprotectants, but the underlying mechanisms of their possible roles in the pathogenesis of TBI-induced injury and the active components involved remain to be determined. Furthermore, in the context of cancer treatment, other specific aspects of TCMC use will require evaluation, including the fact that protectants can often selectively target normal rather than tumor tissue,29–31 and the possible synergistic effects with tumor radiotherapy. Elucidating the mechanisms of action of TCMCs remains a great challenge due to the lack of knowledge of the active components and the possibility of synergistic actions of multiple components with one compound. In the present study, we found that some TCMCs were apparently cytotoxic or even had an IR sensitization effect, however, this requires confirmation.
Another unexplored area is the longer-term whole body effects of these radioprotector candidates on, for example, the skin, esophagus, lung and kidney, which are more radiosensitive tissues than BM and intestine. An additional consideration is how long after exposure to radiation would the TCMCs be effective as there is usually a “window” of time to administer protection prior to or after radiation;29 obviously, an agent with a wide window would be most valuable.
5. Conclusion
Bupleurum injection, shenmai injection, and breviscapine injection can be used as an efficacious radiation damage rescuer, further studies are necessary to confirm the bioactive compounds responsible for the radioprotective effect.
Conflict of interest
The authors declare that there is no conflict of interests regarding the publication of this paper.
List of abbreviations
BMMNCs | Bone marrow mononucleated cells |
CAMS | Chinese Academy of Medical Sciences |
CFU-GM | Colony-forming units-granulocyte/macrophage |
HCT | Red blood cell-specific volume |
HGB | Hemoglobin |
IC10 | 10 percent inhibitory concentration |
IR | Irradiation |
LD50 | Median lethal dose |
MTT | 3-(4,5-Dimethyl-2-thiazolyl)-2,5-diphenyl-2-H-tetrazolium bromide |
PE | Plating efficiency |
PLT | Platelets |
RBCs | Red blood cells |
SF | Survival fraction |
SI | Sham-irradiated |
TBI | Total-body irradiation |
TCM | Traditional Chinese medicine |
TCMC | Traditional Chinese medicine compound |
WBCs | White blood cells. |
Acknowledgements
This paper was supported by National Natural Science Foundation of China (No. 81402541, No. 81403059), the PUMC Youth Fund (3332016144) and the Fundamental Research Funds for CAMS (No. 1608).
References
- M. Z. Kamran, A. Ranjan, N. Kaur, S. Sur and V. Tandon, Med. Res. Rev., 2016, 36, 461–493 Search PubMed.
- C. N. Coleman, B. W. Fau, J. R. Fike, T. J. MacVittie and R. S. Wong, Radiat. Res., 2003, 159, 812–834 CrossRef CAS PubMed.
- K. Sun, J. Fan and J. Han, Acta Pharm. Sin. B, 2015, 5, 8–24 CrossRef PubMed.
- K. J. Krager, E. N. Pineda, S. V. Kharade, M. Kordsmeier, L. Howard, P. J. Breen, C. M. Compadre, M. Hauer-Jensen and N. Aykin-Burns, Evid. base. Compl. Alternative Med., 2015, 2015, 148791 Search PubMed.
- S. Reagan-Shaw, M. Nihal and N. Ahmad, FASEB J., 2008, 22, 659–661 CrossRef CAS PubMed.
- D. J. Brenner, Semin Radiat Oncol, 2008, 18, 234–239 CrossRef PubMed.
- A. Meng, Y. Wang, G. Van Zant and D. Zhou, Cancer Res., 2003, 63, 5414–5419 CAS.
- Y. Wang, B. A. Schulte, A. C. LaRue, M. Ogawa and D. Zhou, Blood, 2006, 107, 358–366 CrossRef CAS PubMed.
- P. Mauch, L. Constine, J. Greenberger, W. Knospe, J. Sullivan, J. L. Liesveld and H. J. Deeg, Int. J. Radiat. Oncol., Biol., Phys., 1995, 31, 1319–1339 CrossRef CAS.
- X. Meng, M. Wang, X. Wang, G. Sun, J. Ye, H. Xu and X. Sun, Free Radical Res., 2014, 48, 823–838 CrossRef CAS PubMed.
- J. Li, J. Xu, W. Xu, Y. Qi, Y. Lu, L. Qiu, Z. Hu, Z. Chu, Y. Chai and J. Zhang, Int. J. Mol. Sci., 2015, 16, 18938–18955 CrossRef CAS PubMed.
- D. Li, L. Lu, J. Zhang, X. Wang, Y. Xing, H. Wu, X. Yang, Z. Shi, M. Zhao, S. Fan and A. Meng, Int. J. Mol. Sci., 2014, 15, 10541–10553 CrossRef CAS PubMed.
- V. Veerapur, K. Prabhakar, V. Parihar, M. Kandadi, S. Ramakrishana, B. Mishra, B. Satish Rao, K. Srinivasan, K. Priyadarsini and M. Unnikrishnan, Evid. base. Compl. Alternative Med., 2009, 6, 317–324 CrossRef CAS PubMed.
- A. Montoro, J. F. Barquinero, M. Almonacid, A. Montoro, N. Sebastia, G. Verdu, V. Sahuquillo, J. Serrano, M. Saiz, J. I. Villaescusa and J. M. Soriano, Evid. base. Compl. Alternative Med., 2011, 2011, 174853 CAS.
- M. Bala, M. Gupta, M. Saini, M. Z. Abdin and J. Prasad, Evid. base. Compl. Alternative Med., 2015, 2015, 765705 Search PubMed.
- L. He, J. Wang, B. Sun, J. Zhao, L. Li, T. Xu, H. Li, J. Sun, J. Ren, R. Liu, Q. Chen, Z. Zhang and Y. Li, J. Nutr. Biochem., 2017, 40, 53–61 CrossRef CAS PubMed.
- X. H. Zhu, S. J. Li, H. H. Hu, L. R. Sun, M. Das and T. M. Gao, J. Ethnopharmacol., 2010, 127, 38–46 CrossRef PubMed.
- L. Li, J. S. Wang and L. Kong, Chin. J. Nat. Med., 2013, 11, 222–230 CrossRef PubMed.
- X. S. Xu, Z. Z. Ma, F. Wang, B. H. Hu, C. S. Wang, Y. Y. Liu, X. R. Zhao, L. H. An, X. Chang, F. L. Liao, J. Y. Fan, H. Niimi and J. Y. Han, Shock, 2009, 32, 201–209 CrossRef PubMed.
- W. Ding, J. Gu, L. Cao, N. Li, G. Ding, Z. Wang, L. Chen, X. Xu and W. Xiao, J. Ethnopharmacol., 2014, 155, 589–598 CrossRef CAS PubMed.
- P. Lam, F. Cheung, H. Y. Tan, N. Wang, M. F. Yuen and Y. Feng, Int. J. Mol. Sci., 2016, 17, 465 CrossRef PubMed.
- B. Yuan, R. Yang, Y. Ma, S. Zhou, X. Zhang and Y. Liu, Pharm. Biol., 2017, 55, 620–635 CrossRef CAS PubMed.
- L. Y. Lu, G. A.-O. Zheng and Y. Wang, Evid base Compl Alternative Med, 2014, 2014, 840650 Search PubMed.
- Z. X. Bian, L. Gao, H. C. Shang, T. X. Wu, Y. P. Li, B. L. Zhang and L. Song, J. Evid. Base. Med., 2010, 3, 5–10 CrossRef PubMed.
- H. G. Kim, H. U. Jeong, S. I. Hong and M. S. Oh, Planta Med., 2015, 81, 1697–1704 CrossRef CAS PubMed.
- X. Liu, L. Yao, D. Sun, X. Zhu, Q. Liu, T. Xu and L. Wang, Exp. Ther. Med., 2016, 12, 1383–1397 Search PubMed.
- X. Qiu, X. Jiang, X. Sun and N. Yang, Evid. base. Compl. Alternative Med., 2012, 2012, 715790 Search PubMed.
- W. Zhang, J. Liu, J. Wang, S. Zhang, S. Zhang and Z. Wu, Biomaterials, 2013, 34, 306–319 CrossRef CAS PubMed.
- E. M. Rosen, R. Day and V. K. Singh, Front. Oncol., 2014, 4, 381 Search PubMed.
- S. Fan, Q. Meng, J. Xu, Y. Jiao, L. Zhao, X. Zhang, F. H. Sarkar, M. L. Brown, A. Dritschilo and E. M. Rosen, Proc. Natl. Acad. Sci. U. S. A., 2013, 110, 18650–18655 CrossRef CAS PubMed.
- R. M. Day, T. A. Davis, M. Barshishat-Kupper, E. A. McCart, A. J. Tipton and M. R. Landauer, Int. Immunopharmacol., 2013, 15, 348–356 CrossRef CAS PubMed.
|
This journal is © The Royal Society of Chemistry 2017 |