DOI:
10.1039/C7RA00472A
(Paper)
RSC Adv., 2017,
7, 12871-12875
Ir-catalyzed asymmetric hydrogenation of β-keto esters with chiral ferrocenyl P,N,N-ligands†
Received
12th January 2017
, Accepted 19th February 2017
First published on 24th February 2017
Abstract
The Ir-catalyzed asymmetric hydrogenation of β-keto esters with chiral ferrocenyl P,N,N-ligands has been developed. Under the optimized conditions, a wide range of β-keto esters were hydrogenated to afford the corresponding β-hydroxy esters in good to excellent enantioselectivities (up to 95% ee).
Introduction
Transition-metal catalyzed asymmetric synthesis represents one of the most important developments in modern chemistry over the past several decades. Among the numerous catalytic methods, asymmetric hydrogenation of unsaturated olefins, ketones and imines is one of the most direct and convenient methods for the synthesis of enantiomerically pure compounds.1 In this regard, the hydrogenation of β-keto esters yield chiral β-hydroxy esters, which are important building blocks for the synthesis of chiral drugs and nature products.2 In 1987, Noyori3 reported the first ruthenium catalyzed enantioselective hydrogenation of β-keto esters using BINAP4 as a chiral ligand. Since this pioneering work, a large number of chiral ligands such as Synphos,5 difluorphos,6 Tunephos,7 Segphos,8 Solphos,9 P-Phos,10 PQ-Phos,11 MeO-Naphephos,12 Taniaphos,13 ClickFerrophos14 and t-Bu-QuinoxP*15 have been developed and successfully applied to the ruthenium catalyzed enantioselective hydrogenation of β-keto esters. Despite these developments, most of the chiral ligands are used in combination with ruthenium as the transition metal. In contrast to chiral ruthenium catalyst, iridium catalyzed asymmetric hydrogenation of β-keto esters remains less explored, although chiral iridium complexes are among the most commonly used catalysts in asymmetric hydrogenation of olefins, ketones and imines.16
Recently, Zhou17 reported an iridium catalyzed enantioselective hydrogenation of β-keto esters using chiral spiro P,N,N-ligands, SpiroPAP (Fig. 1). Inspired by SpiroPAP and our recent success in the development of chiral P,N,N-ligands for asymmetric catalysis,18 we have developed a new class of ferrocenyl P,N,N-ligands with two carbon chiral centers for iridium catalyzed asymmetric hydrogenation of α-alkyl-β-keto esters.19 The success of this work is due to the stereo control of dynamic kinetic resolution (DKR). Considering the structural similarity, we believe that this newly developed P,N,N-ligands should be also applicable to the iridium catalyzed enantioselective hydrogenation of simple β-keto esters without DKR, as the resulting chiral β-hydroxy esters are important organic intermediates. As a result, herein, we report our studies in detail on the iridium catalyzed asymmetric hydrogenation of β-keto esters with chiral P,N,N-ligands, which provides the chiral β-hydroxy esters with good to excellent enantioselectivity (up to 95% ee) under mild conditions (Scheme 1).
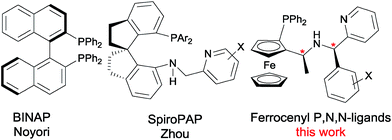 |
| Fig. 1 Examples of efficient chiral ligands in asymmetric hydrogenation of β-keto esters. | |
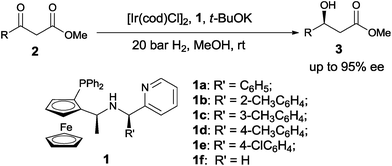 |
| Scheme 1 Ir-catalyzed asymmetric hydrogenation of β-keto esters 2 with chiral ferrocenyl P,N,N-ligands 1. | |
Results and discussion
As we have reported, the chiral ferrocenyl P,N,N-ligands 1 can be easily prepared from (Sc,Rp)-PPFNH2 through a modular procedure.19 With these ligands in hand, we then examined their efficiency in the iridium catalyzed asymmetric hydrogenation of β-keto esters. Methyl 3-oxo-3-phenylpropanoate (2a) was selected as standard substrate and the hydrogenation was carried in MeOH under 20 bar H2 in the presence of 0.1 mol% catalyst prepared in situ from [Ir(cod)Cl]2 and 1. As we can see from Table 1, the iridium complex of ferrocenyl P,N,N-ligands displayed good performance, affording (S)-methyl 3-hydroxy-3-phenylpropanoate (3a) in full conversion and with excellent enantioselectivities (92–94% ee) (entries 1–5). Ligand (Sc,Rp,Rc)-1c bearing 3-methyl phenyl group at the pyridinylmethyl position gave the best enantioselectivity of 94% ee (entry 3). In sharp contrast, ligand (Sc,Rp)-1f with only one chiral carbon center gave disappointing enantioselectivity (entry 6), similar as the result observed in the Ir-catalyzed asymmetric hydrogenation of simple ketones.20 Further optimization of the reaction conditions by screening different bases didn't improve the hydrogenation outcome. All of the bases tested such as t-BuOK, t-BuONa, KOH, NaOH and K2CO3 showed similar results (entries 6–10). The solvent effect was next investigated and an obvious solvent dependence was observed. The alcohol solvent was proved to be suitable solvent and MeOH was the best choice, while other solvents such as CH2Cl2 and toluene showed very low enantioselectivities (entries 11–14). When the catalyst loading was lowered to 0.02 mol% (S/C = 5000, S/C means substrate/catalyst) and 0.01 mol% (S/C = 10
000), the hydrogenation product 3a was still obtained in full conversion and with 89% and 85% ee, respectively (entries 15–16).
Table 1 Asymmetric hydrogenation of methyl 3-oxo-3-phenylpropanoate (2a): optimizing reaction conditionsa

|
Entry |
Ligand |
Base |
Solvent |
Conv.b (%) |
eec (%) |
Hydrogenation was carried out with 0.05 mol% of [Ir(cod)Cl]2, 0.11 mol% of chiral P,N,N-ligands and 5 mol% of bases under 20 bar of H2 at room temperature for 12 h, unless otherwise stated. Conversions were determined by 1H NMR analysis of the crude products. Enantiomeric excesses were determined by HPLC using a chiral stationary phase. The corresponding ethyl ester was obtained. The corresponding isopropyl ester was obtained. S/C = 5000. S/C = 10 000. |
1 |
(Sc,Rp,Rc)-1a |
t-BuOK |
MeOH |
100 |
92 |
2 |
(Sc,Rp,Rc)-1b |
t-BuOK |
MeOH |
100 |
93 |
3 |
(Sc,Rp,Rc)-1c |
t-BuOK |
MeOH |
100 |
94 |
4 |
(Sc,Rp,Rc)-1d |
t-BuOK |
MeOH |
100 |
93 |
5 |
(Sc,Rp,Rc)-1e |
t-BuOK |
MeOH |
100 |
91 |
6 |
(Sc,Rp)-1f |
t-BuOK |
MeOH |
76 |
3 |
7 |
(Sc,Rp,Rc)-1c |
t-BuONa |
MeOH |
100 |
93 |
8 |
(Sc,Rp,Rc)-1c |
KOH |
MeOH |
100 |
93 |
9 |
(Sc,Rp,Rc)-1c |
NaOH |
MeOH |
100 |
94 |
10 |
(Sc,Rp,Rc)-1c |
K2CO3 |
MeOH |
100 |
93 |
11d |
(Sc,Rp,Rc)-1c |
t-BuOK |
EtOH |
100 |
93 |
12e |
(Sc,Rp,Rc)-1c |
t-BuOK |
i-PrOH |
100 |
85 |
13 |
(Sc,Rp,Rc)-1c |
t-BuOK |
Toluene |
100 |
32 |
14 |
(Sc,Rp,Rc)-1c |
t-BuOK |
CH2Cl2 |
100 |
35 |
15f |
(Sc,Rp,Rc)-1c |
t-BuOK |
MeOH |
100 |
89 |
16g |
(Sc,Rp,Rc)-1c |
t-BuOK |
MeOH |
100 |
85 |
Under the optimal reaction conditions, the scope of β-keto esters for this hydrogenation was investigated, and the results are shown in Table 2. The results disclosed that a wide range of β-aryl-β-keto esters 2 can be hydrogenated smoothly and afford the corresponding chiral β-hydroxy esters 3 in high yields and good to excellent enantioselectivities (85–95% ee) (entries 1–11). Although the results are not super than the best ruthenium catalysts reported, it represents a new catalyst system.3–15 It is noteworthy that the steric and electronic effects of the group on the phenyl ring had important influence on the enantioselectivities.17a,21 The substrate 2b with an ortho-Cl in the phenyl ring tended to be hydrogenated with higher enantioselectivity than those with a meta- (2c) or para-Cl (2d) group (entries 2–4). It appeared that the electronic properties of the group at para position of the phenyl ring had some effect on the enantioselectivities, and the substrates with an electron-withdrawing group gave lower enantioselectivities than those with an electron-donating group (entries 4–8). Meanwhile, 2-naphthyl substituted substrate 2i worked well for this reaction and gave the hydrogenation product 3i in 92% yield and with 90% ee (entry 9). Furthermore, heteroaromatic β-keto esters 2j and 2k were also suitable substrates, providing the hydrogenation products 3j and 3k in 90% and 91% ee, respectively (entries 10 and 11). However, this catalyst system was less efficient for the hydrogenation of β-alkyl-β-keto esters such as β-isopropyl-β-keto esters 2l and β-methyl-β-keto esters 2m (entries 12–13).
Table 2 Asymmetric hydrogenation of β-keto esters 2 with Ir/(Sc,Rp,Rc)-1ca

|
Entry |
Substrate |
R |
Yieldb (%) |
eec (%) |
Hydrogenation was carried out with 0.05 mol% of [Ir(cod)Cl]2, 0.11 mol% of (Sc,Rp,Rc)-1c and 5 mol% of t-BuOK under 20 bar of H2 at room temperature for 12 h. Isolated yields. Enantiomeric excesses were determined by HPLC using a chiral stationary phase. |
1 |
2a |
C6H5 |
96 |
94 |
2 |
2b |
2-ClC6H4 |
95 |
95 |
3 |
2c |
3-ClC6H4 |
95 |
88 |
4 |
2d |
4-ClC6H4 |
94 |
87 |
5 |
2e |
4-BrC6H4 |
96 |
85 |
6 |
2f |
4-FC6H4 |
93 |
91 |
7 |
2g |
4-MeC6H4 |
95 |
93 |
8 |
2h |
4-MeOC6H4 |
95 |
93 |
9 |
2i |
2-Naphthyl |
92 |
90 |
10 |
2j |
2-Furyl |
93 |
90 |
11 |
2k |
2-Thienyl |
93 |
91 |
12 |
2l |
i-Pr |
90 |
64 |
13 |
2m |
Me |
90 |
8 |
Conclusion
In conclusion, we have developed a highly efficient iridium catalyzed asymmetric hydrogenation of β-keto esters with chiral ferrocenyl P,N,N-ligands. The results suggest that a wide range of β-keto esters can be hydrogenated with good to excellent enantioselectivities under the optimized conditions. The reaction provides a readily accessible method for the synthesis of β-hydroxy esters in excellent enantioselectivities. Further application of these ferrocenyl P,N,N-ligands in asymmetric hydrogenation is still in progress.
Experimental section
General method
All reactions were carried out under N2 atmosphere. Hydrogenation reaction was carried out in glove-box by use of a stainless steel autoclave. Solvents were purified by standard procedure. Commercial reagents were used without further purification. 1H NMR spectra were recorded on a Bruker 400 MHz spectrometer. Chemical shifts are expressed in δ value (ppm) using tetramethylsilane (TMS) as an internal standard. HPLC analysis was performed on an Agilent 1100 series instrument with a chiralpak AS-H, chiralcel OD-H, chiralcel AD-H or chiralcel OJ-H column. Optical rotations were recorded on a JASCO P-1020 polarimeter. The absolute configurations of the products were determined by comparing optical rotation with the reported data.
General procedure for asymmetric hydrogenation of β-keto esters
In a nitrogen-filled glovebox, a stainless steel autoclave was charged with [Ir(COD)Cl]2 (0.34 mg, 0.005 mmol) and (Sc,Rp,Rc)-L1c (0.66 mg, 0.011 mmol) in 1.0 mL of dry MeOH. After stirring for 1 h at room temperature, a solution of the substrates 2 (1.0 mmol) and t-BuOK (5.6 mg, 0.05 mmol) in 2.0 mL of MeOH was added to the reaction mixture, and then the hydrogenation was performed at room temperature under an H2 pressure of 20 bar for 12 h. The solvent was then evaporated and the residue was purified by flash column chromatography to give the corresponding hydrogenation product which was analyzed by chiral HPLC to determine the enantiomeric excesses.
(S)-Methyl 3-hydroxy-3-phenylpropanoate 3a22,23. Colorless oil was obtained in 96% yield. 94% ee was determined by chiral HPLC (chiralcel OD-H, n-hexane/i-PrOH = 90/10, 0.8 mL min−1, 210 nm, 40 °C): tR (S) = 11.5 min, tR (R) = 16.8 min. [α]28D = −45.5 (c 1.01, CHCl3). 1H NMR (400 MHz, CDCl3) δ 7.44–7.27 (m, 5H), 5.14 (dd, J = 8.8, 4.0 Hz, 1H), 3.72 (s, 3H), 2.97–2.67 (m, 3H).
(S)-Methyl 3-(2-chlorophenyl)-3-hydroxypropanoate 3b4b. Colorless oil was obtained in 95% yield. 95% ee was determined by chiral HPLC (chiralcel OJ-H, n-hexane/i-PrOH = 90/10, 0.8 mL min−1, 210 nm, 40 °C): tR (R) = 9.4 min, tR (S) = 10.7 min. [α]28D = −79.6 (c 1.03, CHCl3). 1H NMR (400 MHz, CDCl3) δ 7.63 (d, J = 7.6 Hz, 1H), 7.38–7.28 (m, 2H), 7.27–7.20 (m, 1H), 5.50 (d, J = 9.6 Hz, 1H), 3.75 (s, 3H), 2.92–2.83 (m, 1H), 2.65–2.55 (m, 1H).
(S)-Methyl 3-(3-chlorophenyl)-3-hydroxypropanoate 3c22. Colorless oil was obtained in 95% yield. 88% ee was determined by chiral HPLC (chiralcel AD-H, n-hexane/i-PrOH = 90/10, 0.8 mL min−1, 210 nm, 40 °C): tR (R) = 9.8 min, tR (S) = 10.8 min. [α]28D = −38.4 (c 1.05, CHCl3). 1H NMR (400 MHz, CDCl3) δ 7.43–7.37 (m, 1H), 7.29–7.23 (m, 3H), 5.11 (dd, J = 7.0, 5.8 Hz, 1H), 3.73 (s, 3H), 2.75–2.69 (m, 2H).
(S)-Methyl 3-(4-chlorophenyl)-3-hydroxypropanoate 3d22,23. Colorless oil was obtained in 94% yield. 87% ee was determined by chiral HPLC (chiralpak AS-H, n-hexane/i-PrOH = 95/5, 0.8 mL min−1, 210 nm, 40 °C): tR (R) = 18.4 min, tR (S) = 20.2 min. [α]28D = −37.6 (c 1.02, CHCl3). 1H NMR (400 MHz, CDCl3) δ 7.35–7.28 (m, 4H), 5.11 (dd, J = 8.1, 4.7 Hz, 1H), 3.73 (s, 3H), 2.75–2.67 (m, 2H).
(S)-Methyl 3-(4-bromophenyl)-3-hydroxypropanoate 3e22,23. Colorless oil was obtained in 96% yield. 85% ee was determined by chiral HPLC (chiralpak AS-H, n-hexane/i-PrOH = 90/10, 0.8 mL min−1, 210 nm, 40 °C): tR (R) = 12.1 min, tR (S) = 13.0 min. [α]28D = −31.7 (c 1.08, CHCl3). 1H NMR (400 MHz, CDCl3) δ 7.48 (d, J = 8.4 Hz, 2H), 7.25 (d, J = 8.4 Hz, 2H), 5.09 (dd, J = 7.9, 4.8 Hz, 1H), 3.72 (s, 3H), 2.78–2.63 (m, 2H).
(S)-Methyl 3-(4-fluorophenyl)-3-hydroxypropanoate 3f22. Colorless oil was obtained in 93% yield. 91% ee was determined by chiral HPLC (chiralpak AS-H, n-hexane/i-PrOH = 90/10, 0.8 mL min−1, 210 nm, 40 °C): tR (R) = 11.6 min, tR (S) = 12.7 min. [α]28D = −42.6 (c 1.03, CHCl3). 1H NMR (400 MHz, CDCl3) δ 7.40–7.30 (m, 2H), 7.10–6.98 (m, 2H), 5.12 (dd, J = 8.7, 4.1 Hz, 1H), 3.73 (s, 3H), 2.80–2.66 (m, 2H).
(S)-Methyl 3-hydroxy-3-(p-tolyl)propanoate 3g21a. Colorless oil was obtained in 95% yield. 93% ee was determined after conversion into the corresponding benzoate by chiral HPLC (chiralcel OD-H, n-hexane/i-PrOH = 90/10, 0.8 mL min−1, 210 nm, 40 °C): tR (R) = 9.0 min, tR (S) = 9.8 min. [α]28D = −44.7 (c 1.04, CHCl3). 1H NMR (400 MHz, CDCl3) δ 7.30–7.23 (m, 2H), 7.16 (d, J = 7.9 Hz, 2H), 5.09 (dd, J = 9.2, 3.8 Hz, 1H), 3.70 (s, 3H), 2.87–2.62 (m, 2H), 2.40 (br, 1H), 2.34 (s, 3H).
(S)-Methyl 3-(4-methoxyphenyl)-3-hydroxypropanoate 3h22. Colorless oil was obtained in 95% yield. 93% ee was by chiral HPLC (chiralcel AD-H, n-hexane/i-PrOH = 95/5, 0.8 mL min−1, 210 nm, 40 °C): tR (R) = 27.0 min, tR (S) = 28.5 min. [α]28D = −38.5 (c 1.00, CHCl3). 1H NMR (400 MHz, CDCl3) δ 7.30 (d, J = 8.6 Hz, 2H), 6.89 (d, J = 8.6 Hz, 2H), 5.09 (dd, J = 9.2, 3.6 Hz, 1H), 3.80 (s, 3H), 3.72 (s, 3H), 3.10 (br, 1H), 2.84–2.64 (m, 2H).
(S)-Methyl 3-hydroxy-3-(naphthalen-2-yl)propanoate 3i21a. Colorless oil was obtained in 92% yield. 90% ee was by chiral HPLC (chiralpak AS-H, n-hexane/i-PrOH = 90/10, 0.8 mL min−1, 210 nm, 40 °C): tR (R) = 13.3 min, tR (S) = 14.6 min. [α]28D = −38.6 (c 1.05, CHCl3). 1H NMR (400 MHz, CDCl3) δ 7.88–7.77 (m, 4H), 7.54–7.42 (m, 3H), 5.30 (dd, J = 8.6, 4.1 Hz, 1H), 3.72 (s, 3H), 3.05 (br, 1H), 2.90–2.75 (m, 2H).
(S)-Methyl 3-(furan-2-yl)-3-hydroxypropanoate 3j23. Colorless oil was obtained in 93% yield. 90% ee was by chiral HPLC (chiralcel OD-H, n-hexane/i-PrOH = 50/50, 0.8 mL min−1, 210 nm, 40 °C): tR (S) = 5.4 min, tR (R) = 8.0 min. [α]28D = −23.4 (c 1.01, CHCl3). 1H NMR (400 MHz, CDCl3) δ 7.28–7.23 (m, 1H), 7.02–6.94 (m, 2H), 5.38 (dd, J = 8.0, 4.6 Hz, 1H), 3.74 (s, 3H), 2.92–2.83 (m, 2H).
(S)-Methyl 3-hydroxy-3-(thiophen-2-yl)propanoate 3k23. Colorless oil was obtained in 93% yield. 91% ee was by chiral HPLC (chiralcel OD-H, n-hexane/i-PrOH = 50/50, 0.8 mL min−1, 210 nm, 40 °C): tR (S) = 5.4 min, tR (R) = 8.0 min. [α]28D = −24.9 (c 1.05, CHCl3). 1H NMR (400 MHz, CDCl3) δ 7.29–7.25 (m, 1H), 7.04–6.93 (m, 2H), 5.38 (dd, J = 8.0, 4.6 Hz, 1H), 3.74 (s, 3H), 2.93–2.83 (m, 2H).
(S)-Methyl 3-hydroxy-4-methylpentanoate 3l24. Colorless oil was obtained in 90% yield. 64% ee was determined after conversion into the corresponding benzoate by chiral HPLC (chiralcel AD-H, n-hexane/i-PrOH = 95/5, 0.8 mL min−1, 210 nm, 40 °C): tR (R) = 7.8 min, tR (S) = 9.0 min. [α]28D = −22.0 (c 1.18, CHCl3). 1H NMR (400 MHz, CDCl3) δ 3.82–3.75 (m, 1H), 3.72 (s, 3H), 2.69–2.37 (m, 3H), 1.78–1.65 (m, 1H), 0.94 (dd, J = 11.2, 6.8 Hz, 6H).
(S)-Methyl 3-hydroxybutanoate 3m7a. Colorless oil was obtained in 90% yield. 8% ee was determined after conversion into the corresponding benzoate by chiral HPLC (chiralcel AD-H, n-hexane/i-PrOH = 95/5, 0.8 mL min−1, 210 nm, 40 °C): tR (S) = 8.1 min, tR (R) = 8.8 min. [α]18D = +14.9 (c 1.05, CHCl3). 1H NMR (400 MHz, CDCl3) δ 4.28–4.14 (m, 1H), 3.72 (s, 3H), 2.87 (br, 1H), 2.53–2.38 (m, 2H), 1.24 (d, J = 6.3 Hz, 3H).
Acknowledgements
The authors are grateful for the financial support from the National Natural Science Foundation of China (No. 21403022, 21572226), Natural Science Foundation of Liaoning Province of China (No. 2015020194), and program of Star of Dalian Youth Science and Technology (No. 2016RQ062). The authors also thank Professor Hongchao Guo in CAU for providing several phosphines (synthesized in the National S&T Pillar Program of China, 2015BAK45B01).
Notes and references
- For some reviews, see:
(a) Handbook of Homogeneous Hydrogenation, ed. J. G. de Vries, C. J. Elsevier, Wiley-VCH, Weinheim, 2007 Search PubMed;
(b) J.-H. Xie, S.-F. Zhu and Q.-L. Zhou, Chem. Rev., 2011, 111, 1713–1760 CrossRef CAS PubMed;
(c) D.-S. Wang, Q.-A. Chen, S.-M. Lu and Y.-G. Zhou, Chem. Rev., 2012, 112, 2557–2590 CrossRef CAS PubMed;
(d) J. J. Verendel, O. Pamies, M. Dieguez and P. Andersson, Chem. Rev., 2013, 113, 2130–2169 Search PubMed.
- N. Andrushko and V. Andrushko, in Stereoselective Synthesis of Drugs and Natural Products, ed. V. Andrushko, Wiley, Hoboken, 2013, pp. 909–959 Search PubMed.
- R. Noyori, T. Ohkuma and M. Kitamura, J. Am. Chem. Soc., 1987, 109, 5856–5858 CrossRef CAS.
- For other examples of BINAP derivative ligands in Ru-catalyzed asymmetric hydrogenation of β-keto esters, see:
(a) A. Hu, H. L. Ngo and W. Lin, Angew. Chem., Int. Ed., 2003, 42, 6000–6003 CrossRef CAS PubMed;
(b) A. Hu, H. L. Ngo and W. Lin, Angew. Chem., Int. Ed., 2004, 43, 2501–2504 CrossRef CAS PubMed;
(c) B. Ma, T. Miao, Y. Sun, Y. He, J. Liu, Y. Feng, H. Chen and Q.-H. Fan, Chem.–Eur. J., 2004, 20, 9969–9978 CrossRef PubMed;
(d) Y.-Y. Huang, Y.-M. He, H.-F. Zhou, L. Wu, B.-L. Li and Q.-H. Fan, J. Org. Chem., 2006, 71, 2874–2877 CrossRef CAS PubMed.
-
(a) S. D. de Paule, S. Jeulin, V. Ratovelomanana-Vidal, J.-P. Genet, N. Champion and P. Dellis, Eur. J. Org. Chem., 2003, 1931–1941 CrossRef;
(b) S. D. de Paule, S. Jeulin, V. Ratovelomanana-Vidal, J.-P. Genet, N. Champion and P. Dellis, Proc. Natl. Acad. Sci. U. S. A., 2004, 101, 5799–5804 CrossRef PubMed.
- S. D. de Paule, S. Jeulin, V. Ratovelomanana-Vidal, J.-P. Genet, N. Champion and P. Dellis, Angew. Chem., Int. Ed., 2004, 43, 320–325 CrossRef PubMed.
-
(a) X. Sun, W. Li, G. Hou, L. Zhou and X. Zhang, Adv. Synth. Catal., 2009, 351, 2553–2557 CrossRef CAS;
(b) C.-J. Wang, C.-B. Wang, D. Chen, D. C. Yang, Z. Wu and X. Zhang, Tetrahedron Lett., 2009, 50, 1038–1040 CrossRef CAS.
- X. Wan, Y. Sun, Y. Luo, D. Li and Z. Zhang, J. Org. Chem., 2005, 70, 1070–1072 CrossRef CAS PubMed.
-
(a) J. Wu, H. Chen, Z.-Y. Zhou, C. H. Yeung and A. S. C. Chan, Synthesis, 2001, 1050–1054 Search PubMed;
(b) J. Wu, H. Chen, W. H. Kwok, K. H. Lam, Z. Y. Zhou, C. H. Yeung and A. S. C. Chan, Tetrahedron Lett., 2002, 43, 1539–1543 CrossRef CAS.
- M. Kesselgruber, M. Lotz, P. Martin, G. Melone, M. Muller, B. Pugin, F. Naud, F. Spindler, M. Thommen, P. Zbinden and H.-U. Blaser, Chem.–Asian J., 2008, 3, 1384–1389 CrossRef CAS PubMed.
-
(a) L. Qiu, F. Y. Kwong, J. Wu, W. H. Lam, S. Chan, W.-Y. Yu, Y.-M. Li, R. Guo, Z. Zhou and A. S. C. Chan, J. Am. Chem. Soc., 2006, 128, 5955–5965 CrossRef CAS PubMed;
(b) L. Qiu, J. Wu, S. Chan, T. T.-L. Au-Yeung, J.-X. Ji, R. Guo, C.-C. Pai, Z. Zhou, X. Li, Q.-H. Fan and A. S. C. Chan, Proc. Natl. Acad. Sci. U. S. A., 2004, 101, 5815–5820 CrossRef CAS PubMed.
- J. Madec, G. Michaud, J.-P. Genet and A. Marinetti, Tetrahedron: Asymmetry, 2004, 15, 2253–2261 CrossRef CAS.
-
(a) T. Ireland, G. Grossheimann, C. Wieser-Jeunesse and P. Knochel, Angew. Chem., Int. Ed., 1998, 38, 3212–3215 CrossRef;
(b) M. Lotz, K. Polborn and P. Knochel, Angew. Chem., Int. Ed., 2002, 41, 4708–4711 CrossRef CAS PubMed;
(c) K. Tappe and P. A. Knochel, Tetrahedron: Asymmetry, 2004, 15, 91–102 CrossRef CAS.
-
(a) S.-i. Fukuzawa, H. Oki, M. Hosaka, J. Sugasawa and S. Kikuchi, Org. Lett., 2007, 9, 5557–5560 CrossRef CAS PubMed;
(b) H. Oki, I. Oura, T. Nakamura, K. Ogata and S.-i. Fukuzawa, Tetrahedron: Asymmetry, 2009, 20, 2185–2191 CrossRef CAS.
- T. Imamoto, M. Nishimura, A. Koide and K. Yoshida, J. Org. Chem., 2007, 72, 7413–7416 CrossRef CAS PubMed.
- For some reviews in Ir-catalyzed asymmetric hydrogenation, see:
(a) A. Pfaltz, J. Blankenstein, R. Hilgraf, E. Hormann, S. McIntyre, F. Menges, M. Schonleber, S. P. Smidt, B. Wustenberg and N. Zimmermann, Adv. Synth. Catal., 2003, 345, 33–43 CrossRef CAS;
(b) K. Kallstrom, I. Munslow and P. G. Andersson, Chem.–Eur. J., 2006, 12, 3194–3200 CrossRef PubMed;
(c) S. Roseblade and A. Pfaltz, Acc. Chem. Res., 2007, 40, 1402–1411 CrossRef CAS PubMed.
-
(a) J.-H. Xie, X.-Y. Liu, X.-H. Yang, J.-B. Xie, L.-X. Wang and Q.-L. Zhou, Angew. Chem., Int. Ed., 2012, 51, 201–203 CrossRef CAS PubMed;
(b) X.-H. Yang, J.-H. Xie, W.-P. Liu and Q.-L. Zhou, Angew. Chem., Int. Ed., 2013, 52, 7833–7836 CrossRef CAS PubMed;
(c) X.-H. Yang, J.-H. Xie and Q.-L. Zhou, Org. Chem. Front., 2014, 1, 190–193 RSC;
(d) X.-H. Yang, K. Wang, S.-F. Zhu, J.-H. Xie and Q.-L. Zhou, J. Am. Chem. Soc., 2014, 136, 17426–17429 CrossRef CAS PubMed;
(e) H. Lin, L.-J. Xiao, M.-J. Zhou, H.-M. Yu, J.-H. Xie and Q.-L. Zhou, Org. Lett., 2016, 18, 1434–1437 CrossRef CAS PubMed.
-
(a) C. Zhang, X.-H. Hu, Y.-H. Wang, Z. Zheng, J. Xu and X.-P. Hu, J. Am. Chem. Soc., 2012, 134, 9585–9588 CrossRef CAS PubMed;
(b) C. Zhang, Y.-H. Wang, X.-H. Hu, Z. Zheng, J. Xu and X.-P. Hu, Adv. Synth. Catal., 2012, 354, 2854–2858 CrossRef CAS;
(c) F.-L. Zhu, Y. Zou, D.-Y. Zhang, Y.-H. Wang, X.-H. Hu, S. Chen, J. Xu and X.-P. Hu, Angew. Chem., Int. Ed., 2014, 53, 1410–1414 CrossRef CAS PubMed;
(d) F.-L. Zhu, Y.-H. Wang, D.-Y. Zhang and X.-P. Hu, Angew. Chem., Int. Ed., 2014, 53, 10223–10227 CrossRef CAS PubMed;
(e) F.-Z. Han, F.-L. Zhu, Y.-H. Wang, Y. Zou, X.-H. Hu, S. Chen and X.-P. Hu, Org. Lett., 2014, 16, 588–591 CrossRef CAS PubMed;
(f) D.-Y. Zhang, F.-L. Zhu, Y.-H. Wang, X.-H. Hu, S. Chen, C.-J. Hou and X.-P. Hu, Chem. Commun., 2014, 50, 14459–14462 RSC;
(g) Y. Zou, F.-L. Zhu, Z.-C. Duan, Y.-H. Wang, D.-Y. Zhang, Z. Cao, Z. Zheng and X.-P. Hu, Tetrahedron Lett., 2014, 55, 2033–2036 CrossRef CAS;
(h) F.-L. Zhu, Y.-H. Wang, D.-Y. Zhang, X.-H. Hu, S. Chen, C.-J. Hou, J. Xu and X.-P. Hu, Adv. Synth. Catal., 2014, 356, 3231–3236 CrossRef CAS;
(i) F.-L. Zhu and X.-P. Hu, Chin. J. Catal., 2015, 36, 86–92 CrossRef CAS;
(j) D.-Y. Zhang, L. Shao, J. Xu and X.-P. Hu, ACS Catal., 2015, 5, 5026–5030 CrossRef CAS;
(k) L. Shao, Y.-H. Wang, D.-Y. Zhang, J. Xu and X.-P. Hu, Angew. Chem., Int. Ed., 2016, 55, 5014–5018 CrossRef CAS PubMed;
(l) Z.-T. Liu, Y.-H. Wang, F.-L. Zhu and X.-P. Hu, Org. Lett., 2016, 18, 1190–1193 CrossRef CAS PubMed;
(m) C. Zhang, Y.-Z. Hui, D.-Y. Zhang and X.-P. Hu, RSC Adv., 2016, 6, 14763–14767 RSC;
(n) L. Shao, D.-Y. Zhang, Y.-H. Wang and X.-P. Hu, Adv. Synth. Catal., 2016, 358, 2558–2563 CrossRef CAS;
(o) X.-S. Chen, C.-J. Hou, Q. Li, Y.-J. Liu, R.-F. Yang and X.-P. Hu, Chin. J. Catal., 2016, 37, 1389–1395 CrossRef CAS.
- C.-J. Hou and X.-P. Hu, Org. Lett., 2016, 18, 5592–5595 CrossRef CAS PubMed.
- H. Nie, G. Zhou, Q. Wang, W. Chen and S. Zhang, Tetrahedron: Asymmetry, 2013, 24, 1567–1571 CrossRef CAS.
-
(a) V. Ratovelomanana-Vidal, C. Girard, R. Touati, J. P. Tranchier, B. B. Hassine and J. P. Genet, Adv. Synth. Catal., 2003, 345, 261–274 CrossRef CAS;
(b) Y. Li, S. Yu, X. Wu, J. Xiao, W. Shen, Z. Dong and J. Gao, J. Am. Chem. Soc., 2014, 136, 4031–4039 CrossRef CAS PubMed.
- P. Borowiecki and M. Bretner, Tetrahedron: Asymmetry, 2013, 24, 925–936 CrossRef CAS.
- R. J. Kloetzing, T. Thaler and P. Knochel, Org. Lett., 2006, 8, 1125–1128 CrossRef CAS PubMed.
- D.-H. Bao, H.-L. Wu, C.-L. Liu, J.-H. Xie and Q.-L. Zhou, Angew. Chem., Int. Ed., 2015, 54, 8791–8794 CrossRef CAS PubMed.
Footnote |
† Electronic supplementary information (ESI) available: Copies of 1H NMR spectra and HPLC for ee analysis. See DOI: 10.1039/c7ra00472a |
|
This journal is © The Royal Society of Chemistry 2017 |
Click here to see how this site uses Cookies. View our privacy policy here.