DOI:
10.1039/C6RA28594E
(Paper)
RSC Adv., 2017,
7, 19223-19230
Photoluminescence tuning of Ca8−xSrxMgGd(PO4)7:Eu2+,yMn2+ phosphors for applications in white LEDs with excellent color rendering index
Received
23rd December 2016
, Accepted 24th March 2017
First published on 31st March 2017
Abstract
Two series of novel phosphors of green-yellow emitting Ca8−xSrxMgGd(PO4)7:Eu2+ and yellow-orange emitting Sr8MgGd(PO4)7:Eu2+,yMn2+ were successfully synthesized by a solid-state reaction. The crystal structures, photoluminescence (PL), PL excitation (PLE) spectra and decay times were investigated in detail. Upon excitation at 350 nm, the Ca4Sr4MgGd(PO4)7:Eu2+ phosphor showed strong green emission centered at 513 nm, and the Sr8MgGd(PO4)7:Eu2+ phosphor showed strong yellow emission centered at 513 nm and 593 nm, and Sr8MgGd(PO4)7:Eu2+,Mn2+ showed strong orange emission centered at 510 nm and 616 nm. In addition, a standard warm white-light emitting single-phased phosphor of Sr8MgGd(PO4)7:Eu2+,Mn2+ with chromaticity coordinates (x, y), correlated color temperature (CCT) and Commission Internationale de l'Eclairage (CIE) coordinates of (0.4497, 0.3929), 2705 K, and 89.7 was realized via energy transfer between Eu2+ and Mn2+. The energy transfer was demonstrated to be a resonant type dipole–quadrupole mechanism. Moreover, the obtained LED device exhibits an excellent color-rendering index (Ra = 97) at a correlated color temperature of 5651 K with the CIE coordinates (0.3290, 0.3410).
1. Introduction
In recent years, white lighting-emitting diodes (LEDs) have attracted much attention because of their applications in liquid crystal displays and solid-state lighting.1,2 The presently-commercialized white LEDs, fabricated by combining a blue-emitting LED chip with the yellow-emitting garnet phosphor (Y3Al5O12:Ce3+) have played an important role in solid-state lighting in recent years.3 They are characterized by cool white light with Commission Internationale de I'Eclairage (CIE) chromaticity coordinates of (0.292, 0.325), a correlated color temperature (CCT) of 7756 K, and poor color rendering indices (CRI, Ra) of 75.4,5 Trichromatic white LEDs are considered to be useful to improve the CRI of white LEDs for their high color-rendering properties.6 Nevertheless, different aging rate for each phosphor and the reabsorption of the blue light by red and green phosphors, will lead to low luminescence efficiency in such system.7 Recently, white LEDs fabricated using near-ultraviolet chips (360–420 nm) coupled with a blend of yellow- and blue-emitting phosphors have exhibited favorable properties, including tunable CCTs, tunable CIE chromaticity coordinates, and excellent Ra values. To realize full-spectrum LEDs, therefore, it is important to develop new green-yellow-emitting and yellow-orange-emitting phosphors for near-UV LED applications.
Recently, whitlockite-type hosts, for example, Ca9Lu(PO4)7:Eu2+,Mn2+,8 Ca8MgGd(PO4)7:Eu2+,9 Sr8MgGd(PO4)7:Eu2+,10 Sr9Mg1.5(PO4)7:Eu2+,11 and Ca8MgY(PO4)7:Eu2+,Mn2+ (ref. 12) have been reported for LED phosphors due to their outstanding luminescence properties as down-conversion phosphors.
To the best of our knowledge, the crystal structures and luminescence properties of color tunable Ca8−xSrxMgGd(PO4)7:Eu2+ green-yellow-emitting phosphor and Sr8MgGd(PO4)7:Eu2+,yMn2+ yellow-orange-emitting phosphor have not yet been reported in the literature. In this study, we investigated the luminescence properties of color tunable green-yellow-emitting Ca8−xSrxMgGd(PO4)7:Eu2+ and yellow-orange-emitting Sr8MgGd(PO4)7:Eu2+,Mn2+ phosphors. In addition, white-light near-UV LEDs possessing excellent CRI values and warm correlated color temperatures were fabricated using phosphor blends of blue-emitting Sr5(PO4)3Cl:Eu2+ and red-emitting Sr4Al14O25:Mn4+ with some green-emitting Ca4Sr4MgGd(PO4)7:0.02Eu2+ or yellow-emitting Sr8MgGd(PO4)7:0.02Eu2+ or orange-emitting Sr8MgGd(PO4)7:0.02Eu2+,0.25Mn2+ and their optical properties were investigated.
2. Experimental section
2.1 Materials and synthesis
Polycrystalline phosphors with composition of Ca8−xSrxMgGd(PO4)7:0.02Eu2+ and Sr7.98MgGd(PO4)7:0.02Eu2+,yMn2+ described in this work were prepared with a high-temperature solid-state reaction. Briefly, the constituent raw materials SrCO3 (A. R., 99.9%), CaCO3 (A. R., 99.9%), MgO (A. R., 99%), Gd2O3 (A. R., 99.99%), MnCO3 (A. R., 99.9%), NH4H2PO4 (A. R., 99%), and Eu2O3 (A. R., 99.99%) were weighed according to the stoichiometric ratio. Individual batches of 10 g were weighted according to the designed stoichiometry and mixed homogeneously with the same mass of absolute ethyl alcohol as the dispersant. After planetary ball-milling process, the obtained homogeneous slurry was placed in a Petri dish and dried in an oven. Then, the dried mixtures were put into a crucible with a lid and heated in a tubular furnace at 1250 °C for 6 hours under a reducing atmosphere of 5% H2/95% N2. When cooled down to room temperature, the prepared phosphors were crushed and ground for subsequent measurements.
2.2 Characterization
All crystal structure compositions were checked for phase formation by using powder X-ray diffraction (XRD) analysis with a Rigaku X-ray diffractometer (Tokyo, Japan) with a graphite monochromator using Cu Kα radiation (λ = 1.54056 Å), over the angular range 10 < 2θ < 80, operating at 40 kV and 40 mA. XRD Rietveld profile refinements of the structural models and textural analysis were performed with the use of TOPAS 4.2 software. The photoluminescence (PL) and photoluminescence excitation (PLE) spectra of the samples were analyzed by using a Hitachi F-7000 spectrophotometer (Tokyo, Japan) with a 150 W Xe lamp. The luminescence decay curve was obtained from a Lecroy Wave Runner 6100 digital oscilloscope (1 GHz) using a tunable laser (pulse width = 4 ns; gate = 50 ns) as the excitation source (Continuum Sunlite OPO). Optical properties such as luminescence spectra, correlated color temperature (CCT), color-rendering index, and the Commission International de I'Eclairage (CIE) color coordinates of the initial mixed phosphors and the white LEDs fabricated were characterized using a DARSA PRO 5100 PL system (PSI Trading Co. Ltd, Korea) and evaluated under a forward bias current of 60 mA at room temperature.
3. Results and discussion
3.1 Phase identification and crystal structure
Fig. 1(a) depicts powder XRD profiles of Ca8−xSrxMgGd(PO4)7:Eu2+ (x = 0, 1, 2, 3, 4, 5, 6, 7, and 8) samples along with the standard data Ca8MgGd(PO4)7 (JCPDS card no. 50-1766). From Fig. 1, we can clearly find that all the diffraction peaks of the samples can be well indexed to the standard data of Ca8MgGd(PO4)7, which proves that the phase of Ca8−xSrxMgGd(PO4)7:Eu2+ samples is pure. Ca8−xSrxMgGd(PO4)7:Eu2+ are isostructural with Ca8MgGd(PO4)7 and the doping of Eu2+ ions does not cause significant impurities to the crystal structure. Fig. 1(b) shows the zoomed powder XRD profiles in the range of 29–32 degree, which exhibits the linear shift of peaks with the Ca replaced the Sr in Ca8−xSrxMgGd(PO4)7:Eu2+ samples.
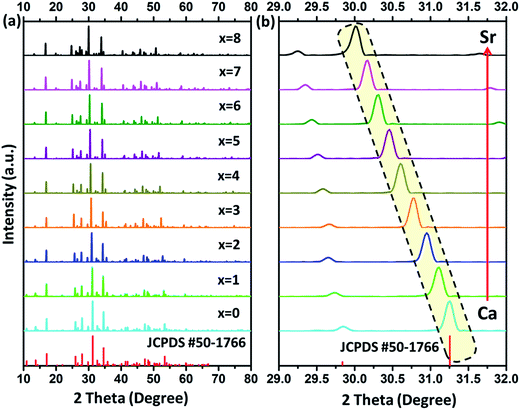 |
| Fig. 1 (a) Representative powder XRD profiles of Ca8−xSrxMgGd(PO4)7:Eu2+ (x = 0, 1, 2, 3, 4, 5, 6, 7, and 8) together with the standard data of Ca8MgGd(PO4)7 (JCPDS card no. 50-1766). (b) The zoomed powder XRD profiles in the range of 29–32 degree. | |
The lattice parameters from Rietveld refinements using TOPAS 4.2 are displayed in Fig. 2. The lattice parameters and cell volumes show a linear dependence on x, as can be anticipated from Vegard's law.13 Moreover; the substitution is accompanied by a increase of a, c and V. The corresponding equations of the cell parameters as a function of chemical composition, x, are presented in Fig. 2 and can be explained by the difference in ionic radii between the substituted and substituting ions. It can been seen that both the lattice constants and primitive volume increase linearly when x increases in Ca8−xSrxMgGd(PO4)7, which could be ascribed to the smaller ionic radius of Ca2+ ions (1.00 Å 1.12 Å and 1.18 Å when corresponding number is 6, 8 and 9, respectively) compared to that of Sr2+ ions (1.18 Å 1.26 Å and 1.31 Å when corresponding number is 6, 8 and 9, respectively).
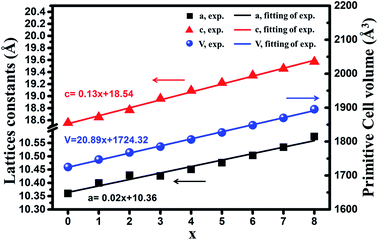 |
| Fig. 2 Dependence of the measured lattice constants (a and c) and primitive cell volume (V) on x of the compounds Ca8−xSrxMgGd(PO4)7 (x = 0, 1, 2, 3, 4, 5, 6, 7, 8). | |
To get the detailed crystal structure information on the obtained samples, we carried out Rietveld refinement of Sr8MgGd(PO4)7:0.02Eu2+ sample with the single crystal structure data of Sr9MgH(PO4)7 (ICSD no. 5113) as the initial model. Fig. 3 shows the observed (crosses) and calculated (red dots) XRD patterns together with their difference (blue) for the refinement of Sr8MgGd(PO4)7:0.02Eu2+ sample. The refinement results reveal that Sr8MgGd(PO4)7 has the trigonal structure with the space group of R3m, cell parameters of a = b = 10.569 Å, c = 19.57 Å, and cell volume of V = 1893.22 Å3. And the refinement finally converged to GOF = 2.37, Rp = 7.7%, and Rwp = 11.09% (Table 1), which again demonstrates that Sr8MgGd(PO4)7 is isotypic with Sr9MgH(PO4)7 and Eu2+ ions have been doped into the host lattice successfully.
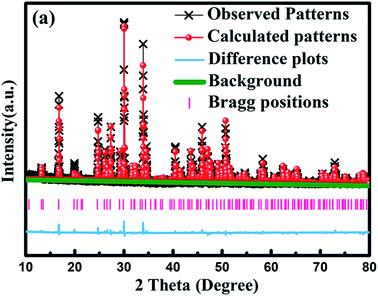 |
| Fig. 3 Observed (crosses) and calculated (red dots) powder XRD patterns of Sr8MgGd(PO4)7:0.02Eu2+ phosphor. The pink sticks stand for the positions of Bragg reflection and the blue line marks the difference between observed and calculated data. | |
Table 1 Rietveld refinement and crystallographic data of Sr8MgGd(PO4)7:0.02Eu2+ sample
Formula |
Sr8MgGd(PO4)7:0.02Eu2+ |
Space group |
R m (no. 166), trigonal |
a = b (Å) |
10.569 |
c (Å) |
19.57 |
α = β (deg) |
90 |
γ (deg) |
120 |
V (Å3) |
1893.22 |
Rp (%) |
7.7 |
Rwp (%) |
11.09 |
GOF |
2.37 |
The crystal structure model of Sr9MgH(PO4)7 is depicted in Fig. 4. In the unit cell of Sr8MgGd(PO4)7, there are three Sr crystallographic sites (Sr1, Sr2, and Sr3), two Mg sites (Mg1 and Mg2) and two P sites (P1 and P2). The coordinated polyhedrons of Sr1, Sr2 and Sr3 are also shown in Fig. 4.
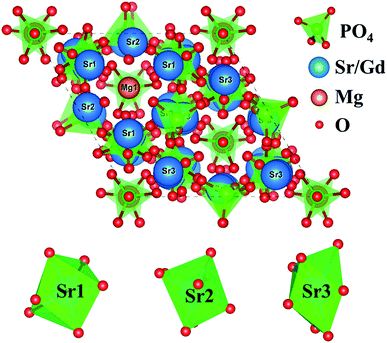 |
| Fig. 4 Crystal structure of Sr8MgGd(PO4)7 and the coordinated polyhedrons of Sr1, Sr2, Sr3. | |
3.2 Photoluminescence properties of Ca8−xSrxMgGd(PO4)7:0.02Eu2+ doped phosphors
Fig. 5 shows PLE spectra of Ca8−xSrxMgGd(PO4)7:0.02Eu2+ (x = 0, 1, 2, 3, 4, 5, 6, 7, 8). The excitation spectra monitored at 510 nm consist of broad bands ranging from 220–500 nm with a maximum at ∼350 nm, which are assigned to 4f7–4f65d1 transition of Eu2+ ions. The PLE spectra nearly cover the region from UV to blue. Moreover, the PL spectra, as shown in Fig. 6, are measured and studied to further analyze the Eu2+ emitting centers in Ca8−xSrxMgGd(PO4)7:0.02Eu2+. The luminous intensity of Ca8−xSrxMgGd(PO4)7:0.02Eu2+ is rather low when x ≤ 2 for the difference between radius of Ca2+ (1 Å when corresponding number is 6) and that of Eu2+ (1.17 Å when corresponding number is 6) is very large. Then the luminous intensity increased with more Sr2+ replacing Ca2+. The radius of Sr2+ (1.18 Å when corresponding number is 6) is very similar to the radius of Eu2+, which leads the Eu2+ is easier to replace Sr2+ than Ca2+. Interestingly, the two emission bands turn to one emission bands at green light region when x = 4, namely, Ca4Sr4MgGd(PO4)7:0.02Eu2+. Form Fig. 2, it is known that both the lattices constants and primitive volume increase linearly when x increases in Ca8−xSrxMgGd(PO4)7 which could be ascribed to the larger ionic radius of Sr2+ ions compared to that of Ca2+ ions. Eu2+ ions are easier to replace Sr2+ rather than Ca2+ because of their similar ionic radius. In Ca8MgGd(PO4)7 host, Eu2+ is very difficult to replace Ca2+, leading the weak emission intensity. Sr2+ makes the crystal lattice larger when Ca2+ is replaced by Sr2+, which leading that Eu2+ can be easily doped into host, then the emission intensity increased. However, Eu2+ has the tendency to replace the Sr2+ which has similar ion radius in different Sr sites. So there are significant spectral variations when Ca2+ is subtitled by Sr2+. When Sr2+ continues to replace Ca2+, the green emission band turns back into two emission bands, covered with green and yellow regions. The insets show the photographs of phosphors excited by 365 nm excitation. From Fig. 7(a), fitted curve (red dashed line) and deconvoluted Gaussian components (red, green and blue dashed lines for peak 1, 2 and 3 respectively) of Ca8−xSrxMgGd(PO4)7:0.02Eu2+ (λex = 350 nm) are shown in detail. It can be seen that there are three deconvoluted Gaussian peaks of Eu2+ corresponding to three types of Sr2+/Ca2+ sites in all samples. Peak 1 has a red shift before x = 4, while peak 2 and peak 3 have a blue shift before x = 4 with the increase replacement of Sr2+, which is shown in Fig. 7(b). However, the emission peak position would not change when x > 4, which means the luminescence of Eu2+ no longer depends strongly on the change of crystal field, even though the lattice parameters still increase. As depicted in Fig. 7(c), the luminous intensity increased and reached maximum at x = 4, and then decreased. After x = 6, the luminous intensity increased again and reached maximum at x = 8, that is Sr8MgGd(PO4)7:0.02Eu2+.
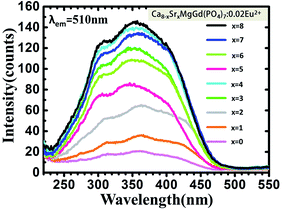 |
| Fig. 5 PLE spectra of Ca8−xSrxMgGd(PO4)7:0.02Eu2+ (x = 0, 1, 2, 3, 4, 5, 6, 7, 8). | |
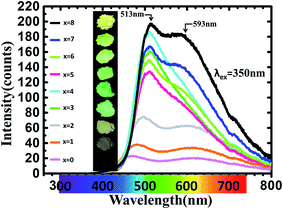 |
| Fig. 6 PL spectra of Ca8−xSrxMgGd(PO4)7:0.02Eu2+ (x = 0, 1, 2, 3, 4, 5, 6, 7, 8). The insets show photographs of phosphors excited by 365 nm excitation. | |
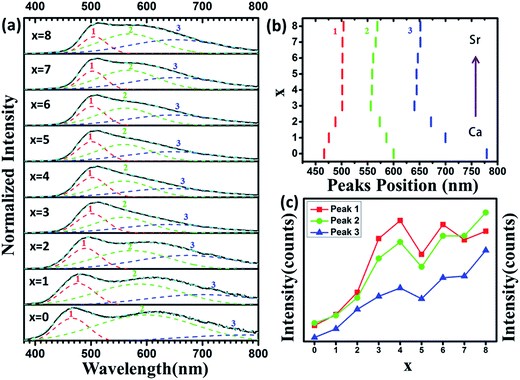 |
| Fig. 7 (a) Fitted curve (red dashed line) and deconvoluted Gaussian components (red, green, blue and cyan dashed lines) of Ca8−xSrxMgGd(PO4)7:0.02Eu2+ (λex = 350 nm). (b) The variation of peaks position with x increase in Ca8−xSrxMgGd(PO4)7:0.02Eu2+ (x = 0, 1, 2, 3, 4, 5, 6, 7, 8). (c) The comparison of peaks intensity. | |
3.3 Energy transfer properties in Sr8MgGd(PO4)7:Eu2+,Mn2+ phosphors
The PLE spectra (Fig. 8.) increase the intensity with increasing the concentration of doping Mn2+ ions when monitored at 600 nm. The broad excitation bands covered from 220 nm to 470 nm. Under the excitation of 350 nm, both the emission of Eu2+ and Mn2+ can be observed in the PL spectrum of the co-doped samples as shown in Fig. 9. The emission intensity for Eu2+ decreases with increasing Mn2+ concentration, whereas the emission intensity for Mn2+ increases with increasing the concentration. The insets show photographs of phosphors excited by 365 nm excitation. The color turns from yellow to orange-yellow with the increasing Mn2+ concentration. There is a concentration quenching about Mn2+ 616 nm emission peak when y > 0.25 in Sr8MgGd(PO4)7:0.02Eu2+,yMn2+.
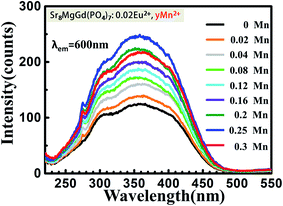 |
| Fig. 8 PLE spectra of Sr8MgGd(PO4)7:Eu2+,yMn2+ (y = 0, 0.02, 0.04, 0.08, 0.12, 0.16, 0.2, 0.25). | |
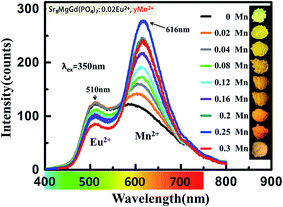 |
| Fig. 9 PL spectra of Sr8MgGd(PO4)7:0.02Eu2+,yMn2+ (y = 0, 0.02, 0.04, 0.08, 0.12, 0.16, 0.2, 0.25). The insets show photographs of phosphors excited by 365 nm excitation. | |
In order to well understand the energy transfer process, we measured the PL decay curves and then calculated the lifetimes as well as energy-transfer efficiencies. Fig. 10 shows the PL decay curves of the Eu2+ ions in Sr8MgGd(PO4)7:Eu2+,yMn2+, which were measured with excitation at 350 nm and monitored at 510 nm. One can see that the decay curve of the singly Eu2+ doped Sr8MgGd(PO4)7:Eu2+ sample can be well fitted into a single-exponential function with a decay time of 1.161 μs. This is the radiative decay time of the Eu2+ ions. For the Eu2+ and Mn2+ codoped samples, the doping of the Mn2+ ions significantly modifies the fluorescent dynamics of the Eu2+ ions. The results reveal that the fluorescence decays deviate slightly from a single exponential rule, indicating the presence of a nonradiative process. The effective lifetime is defined as
|
 | (1) |
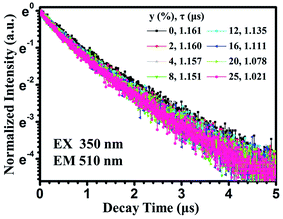 |
| Fig. 10 PL decay curves of Eu2+ in Sr8MgGd(PO4)7:Eu2+,yMn2+ (excited at 350 nm and monitored at 510 nm). | |
On the basis of eqn (1), the effective lifetime values were calculated to be 1.160, 1.157, 1.151, 1.135, 1.111, 1.078, and 1.021 μs for Sr8MgGd(PO4)7:Eu2+,yMn2+ with y = 0.02, 0.04, 0.08, 0.12, 0.16, 0.2, and 0.25, respectively. It can be seen that the decay lifetime of the Eu2+ ions decreases monotonically with an increase in the Mn2+ doping concentration, which strongly supports energy transfer from the Eu2+ to Mn2+ ions.
Based on the following equation,14 the energy transfer efficiency (ηT) from Eu2+ to Mn2+ can be evaluated:
|
 | (2) |
where
τs and
τso are the decay time of the Eu
2+ ions in the absence and presence of Mn
2+ ions.
The results are shown in Fig. 11. It can be observed that the ηT increases gradually with the increase of the Mn2+ doping concentration and finally reaches to 0.12 for y = 0.25.
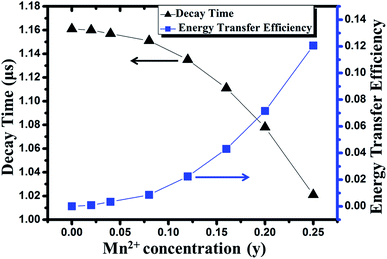 |
| Fig. 11 Dependence of decay time and energy transfer efficiency on the concentration of Mn2+ in Sr8MgGd(PO4)7:Eu2+,yMn2+ phosphor. | |
According to Dexter's theory,15 non-radiative energy transfer between sensitizer and activator ions can occur by exchange interaction, or multipolar interactions. The following formula will be fitted linearly if exchange interaction works:
|
 | (3) |
where
C is the total concentration of Eu
2+ and Mn
2+ ions,
ηso and
ηs are the luminescence quantum efficiencies of Eu
2+ in the absence and presence of Mn
2+, respectively. The value of
ηso/
ηs can be approximately estimated from the related decay time's ratio
τso/
τs. Thus,
eqn (3) can be represented by the following equation:
|
 | (4) |
As shown in Fig. 12(a), linear relation is not well obtained via exchange interaction (R2 = 0.858). Therefore, the energy of Eu2+ is transferred to Mn2+ by multiple–multiple interaction. For multipolar interactions, the following relation can be obtained:
|
 | (5) |
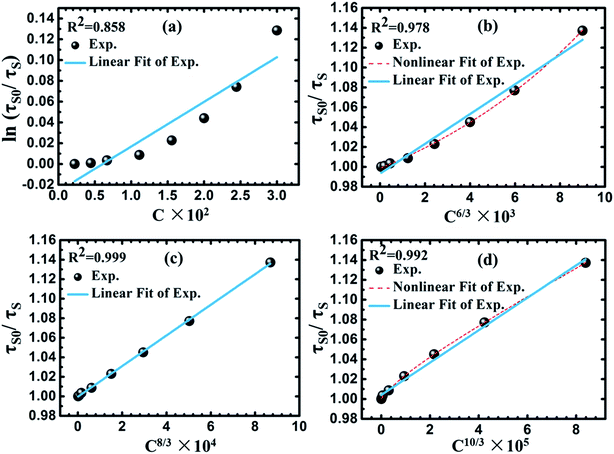 |
| Fig. 12 Dependence of ln(τso/τs) of Eu2+ on CEu+Mn (a), and τso/τs of Eu2+ on C6/3 (b), C8/3 (c) and C10/3 (d). | |
As mentioned above, eqn (5) can be also represented by the following equation:
|
 | (6) |
where
a = 6, 8 and 10 is corresponding to dipole–dipole, dipole–quadrupole and quadrupole–quadrupole interactions, respectively. In
Fig. 12(b)–(d), it can be observed that a well fitted linear relation was obtained when
a = 8, which indicates that the dominant interaction mechanism for energy transfer from Eu
2+ to Mn
2+ in Sr
8MgGd(PO
4)
7 is dipole–quadrupole interaction.
3.4 Temperature-dependent emission spectra, LED lamp fabrication and EL spectrum
To demonstrate the potential application of Ca8−xSrxMgGd(PO4)7:0.02Eu2+ and Sr8MgGd(PO4)7:0.02Eu2+,yMn2+ phosphor, as shown in Fig. 13, temperature-dependent integrated PL intensity of the phosphors have been obtained. These three types of phosphors have similar thermal stabilities. There is around 50% intensity attenuation when temperature is 100 °C. The internal quantum efficiency of Sr4Ca4Gd(PO4)7:0.02Eu2+, Sr8Gd(PO4)7:0.02Eu2+ and Sr8Gd(PO4)7:0.02Eu2+,0.25Mn2+ phosphors is 20.6%, 30.2% and 17.8%. As Fig. 14(a) shows, phosphor-converted LED was fabricated by combining a 365 nm UV-chip and driven by a forward-bias current of 60 mA. Point B, G, Y, O and R represent Sr5(PO4)3Cl:0.02Eu2+ blue phosphor, Ca4Sr4MgGd(PO4)7:0.02Eu2+ green phosphor, Sr8MgGd(PO4)7:0.02Eu2+ yellow phosphor, Sr8MgGd(PO4)7:0.02Eu2+,0.25Mn2+ orange phosphor and Sr4Al14O25:0.01Mn4+ red phosphor, respectively. All the possible that mixed kinds of phosphors are contained in the area of dashed triangle. BGR (or BYR) represents the mixture composed of Sr5(PO4)3Cl:0.02Eu2+ blue phosphor, Sr4Al14O25:0.01Mn4+ red phosphor and Ca4Sr4MgGd(PO4)7:0.02Eu2+ green phosphor (or Sr8MgGd(PO4)7:0.02Eu2+ yellow phosphor). A standard warm white-light emitting single-phased phosphor of Sr8MgGd(PO4)7:Eu2+,Mn2+ (point O) with chromaticity coordinates (x, y), correlated color temperature (CCT) and Commission Internationale de l'Eclairage (CIE) coordinates of ((0.4497, 0.3929), 2705 K, 89.7) is realized via energy transfer between Eu2+ and Mn2+. The insets show photographs of the LED lamp packages driven by 60 mA current. Fig. 14(b) and (c) show the electroluminescence (EL) spectra of the lamps. Three emission bands can be clearly seen in Fig. 14(b): 450 nm, attributed to Sr5(PO4)3Cl:0.02Eu2+ blue phosphor, the 510 and 668 nm, attributable to the Ca4Sr4MgGd(PO4)7:0.02Eu2+ green phosphor and Sr4Al14O25:0.01Mn4+ red phosphor, respectively. CRI value of BGR is 94.1 and CCT is 7556 K. Four emission bands can be clearly seen in Fig. 14(c), 450 nm, attributed to Sr5(PO4)3Cl:0.02Eu2+ blue phosphor, the 510 and 600 nm, attributable to the Sr8MgGd(PO4)7:0.02Eu2+ yellow phosphor, Sr4Al14O25:0.01Mn4+ red phosphor, respectively. CRI value of BYR reach up to 97 and CCT is 5651 K. The luminous efficiencies of BGR, BYR and O LED prototype under 60 mA are 23.67 lm W−1, 24.03 lm W−1 and 24.28 lm W−1, respectively. The low luminous efficiency contributes to our low efficient 365 nm UV-chips. The CIE chromaticity diagram, CIE chromaticity coordinates, CCT, and Ra were listed in Table 2. The results obtained for the LED package demonstrated that Ca8−xSrxMgGd(PO4)7:0.02Eu2+ has potential applications in the full-spectrum white-light NUV LEDs with excellent CRIs.
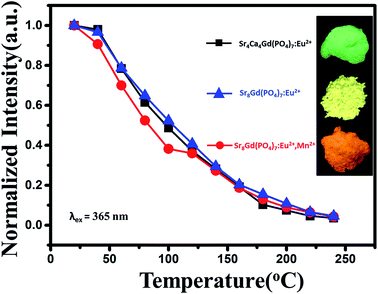 |
| Fig. 13 Temperature-dependent integrated PL intensity of Sr4Ca4Gd(PO4)7:0.02Eu2+, Sr8Gd(PO4)7:0.02Eu2+ and Sr8Gd(PO4)7:0.02Eu2+,0.25Mn2+ phosphors. Inset: photographs of the phosphors under 365 nm UV-light. | |
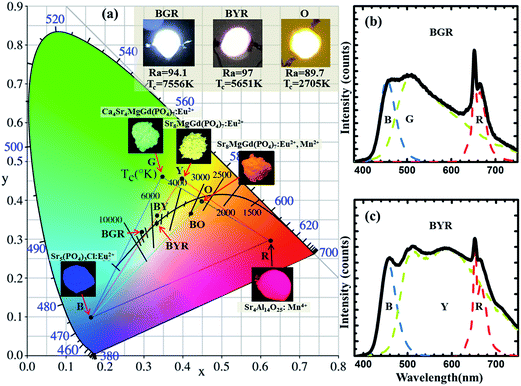 |
| Fig. 14 CIE chromaticity diagram of a mixing of Ca4Sr4MgGd(PO4)7:0.02Eu2+ green phosphors (point G), Sr8MgGd(PO4)7:0.02Eu2+ yellow phosphors (point Y), Sr8MgGd(PO4)7:0.02Eu2+,0.25Mn2+ orange phosphors (point O), Sr5(PO4)3Cl:0.02Eu2+ blue phosphors (point B) and Sr4Al14O25:0.01Mn4+ red phosphors (point R). The insets show photographs of LED package with 365 nm UV-chips driven by 60 mA current and phosphors excited by 365 nm excitation. | |
Table 2 CIE chromaticity coordinates, CCT, and Ra for marked points
Point |
Components |
(x, y) |
CCT (K) |
Ra |
B |
Sr5(PO4)3Cl:0.02Eu2+ |
(0.1641, 0.0994) |
— |
18.3 |
G |
Ca4Sr4MgGd(PO4)7:0.02Eu2+ |
(0.3492, 0.4599) |
5125 |
72.0 |
Y |
Sr8MgGd(PO4)7:0.02Eu2+ |
(0.3972, 0.4535) |
4080 |
82.0 |
O |
Sr8MgGd(PO4)7:0.02Eu2+,0.25Mn2+ |
(0.4497, 0.3929) |
2705 |
89.7 |
R |
Sr4Al14O25:0.01Mn4+ |
(0.6252, 0.2859) |
1001 |
20.6 |
BY |
B + Y |
(0.3316, 0.3719) |
5541 |
90.3 |
BO |
B + O |
(0.4155, 0.3548) |
2949 |
84.9 |
BGR |
B + G + R |
(0.2966, 0.3213) |
7556 |
94.1 |
BYR |
B + Y + R |
(0.3290, 0.3410) |
5651 |
97.0 |
4. Conclusions
In summary, we have synthesized two series of novel phosphors of Ca8−xSrxMgGd(PO4)7:Eu2+ and Sr8MgGd(PO4)7:Eu2+,yMn2+ by the high temperature solid-state reaction. A warm white-light emitting single-phased phosphor of Sr8MgGd(PO4)7:Eu2+,Mn2+ with chromaticity coordinates (x, y), correlated color temperature (CCT) and Commission Internationale de l'Eclairage (CIE) coordinates of ((0.4497, 0.3929), 2705 K, 89.7) is realized via energy transfer between Eu2+ and Mn2+. The energy transfer was demonstrated to be a resonant type dipole–quadrupole mechanism. A white LED with Ra = 97 (or Ra = 94.1) was successfully fabricated by coating Sr8MgGd(PO4)7:Eu2+ (or Ca4Sr4MgGd(PO4)7:Eu2+) with a blend of Sr5(PO4)3Cl:Eu2+ blue and Sr4Al14O25:Mn4+ red phosphor onto a near UV 365 nm chip.
Acknowledgements
This work was financially supported by the programs of National Natural Science Foundation of China (No. 51272282 & 51302311), and significant achievement transformation project of colleges and universities of the Central in Beijing (ZD20141000201), supported by Beijing Municipal Education Commission.
References
- S. Nakamura, T. Mukai and M. Senoh, Candela. Candela-class high-brightness InGaN/AlGaN double-heterostructure blue-light-emitting diodes, Appl. Phys. Lett., 1994, 64, 1687–1689 CrossRef CAS.
- P. Thiyagarajan, M. Kottaisamy and M. S. Ramachandra Rao, Luminescent properties of near UV excitable Ba2ZnS3:Mn red emitting phosphor blend for white LED and display applications, J. Phys. D: Appl. Phys., 2006, 39, 2701–2706 CrossRef CAS.
- V. Bachmann, C. Ronda and A. Meijerink, Temperature Quenching of Yellow Ce3+ Luminescence in YAG:Ce, Chem. Mater., 2009, 21, 2077–2084 CrossRef CAS.
- A. A. Setlur, W. J. Heward, Y. Gao, A. M. Srivastava, R. G. Chandran and M. V. Shankar, Crystal Chemistry and Luminescence of Ce3+-Doped Lu2CaMg2(Si,Ge)3O12 and Its Use in LED Based Lighting, Chem. Mater., 2006, 18, 3314–3322 CrossRef CAS.
- H. S. Jang, Y. H. Won and D. Y. Jeon, Improvement of Electroluminescent Property of Blue LED Coated with Highly Luminescent Yellow-Emitting Phosphors, Appl. Phys. B: Lasers Opt., 2009, 95, 715–720 CrossRef CAS.
- L. Huang, M. Guo, S. Zhao, D. Deng, H. Wang, Y. Hua, G. Jia and S. Xu, Luminescence of Ca2LiSiO4F:Ce3+,Tb3+ Phosphors, ECS J. Solid State Sci. Technol., 2013, 2, R3083–R3087 CrossRef CAS.
- A. A. Setlur, W. J. Heward, Y. Gao, A. M. Srivastava, R. G. Chandran and M. V. Shankar, Crystal Chemistry and Luminescence of Ce3+-Doped Lu2CaMg2(Si,Ge)3O12 and Its Use in LED Based Lighting, Chem. Mater., 2006, 18, 3314–3322 CrossRef CAS.
- N. Guo, Y. Huang, H. You, M. Yang, Y. Song, K. Liu and Y. Zheng, Ca9Lu(PO4)7:Eu2+,Mn2+: A Potential Single-Phased White-Light-Emitting Phosphor Suitable for White-Light-Emitting Diodes, Inorg. Chem., 2010, 49, 10907 CrossRef CAS PubMed.
- H. Yanlin, D. Haiyan, J. Kiwan, C. Eunjin, L. Ho, M. Jayasimhadri and Y. Soung-Soo, Luminescence properties of triple phosphate Ca8MgGd(PO4)7:Eu2+ for white light-emitting diodes, J. Phys. D: Appl. Phys., 2008, 41, 095110 CrossRef.
- C. H. Huang, D. Y. Wang, Y. C. Chiu, Y. T. Yeh and T. M. Chen, Sr8MgGd(PO4)7:Eu2+: yellow-emitting phosphor for application in near-ultraviolet-emitting diode based white-light LEDs, RSC Adv., 2012, 2, 9130–9134 RSC.
- W. Z. Sun, Y. L. Jia, R. Pang, H. F. Li, T. F. Ma, J. P. Fu, S. Zhang and L. H. Jiang, Sr9Mg1.5(PO4)7:Eu2+: A Novel Broadband Orange-Yellow-Emitting Phosphor for Blue Light-Excited Warm White LEDs, ACS Appl. Mater. Interfaces, 2015, 7, 25219–25226 CAS.
- D. Wen, Z. Dong, J. Shi, M. Gong and M. Wu, Standard White-Emitting Ca8MgY(PO4)7:Eu2+,Mn2+ Phosphor for White-Light-Emitting LEDs, ECS J. Solid State Sci. Technol., 2013, 2, R178–R185 CrossRef CAS.
- A. R. Denton and N. W. Ashcroft, Vegard's law, Phys. Rev. A: At., Mol., Opt. Phys., 1991, 43, 3161–3164 CrossRef CAS.
- P. I. Paulose, G. Jose, V. Thomas, N. V. Unnikrishnan and M. K. R. Warrier, Luminescence Properties of Mn2+-Doped Sol–Gel Glasses, J. Phys. Chem. Solids, 2003, 64, 841 CrossRef CAS.
- D. L. Dexter and J. A. Schulman, Theory of Concentration Quenching in Inorganic Phosphors, J. Chem. Phys., 1954, 22, 1063–1070 CrossRef CAS.
|
This journal is © The Royal Society of Chemistry 2017 |