DOI:
10.1039/C6RA28064A
(Paper)
RSC Adv., 2017,
7, 11796-11802
Design, synthesis, antibacterial and insecticidal activities of novel N-phenylpyrazole fraxinellone hybrid compounds†
Received
12th December 2016
, Accepted 13th February 2017
First published on 16th February 2017
Abstract
In continuation of our research aimed at the discovery and development of natural product-based antimicrobial and insecticidal agents, a series of 20 novel N-phenylpyrazole fraxinellone hybrid compounds were prepared and evaluated for their antibacterial and insecticidal activities. Two key steric configurations of compounds 4h and 4r were unambiguously determined by X-ray crystallography. Although only compound 4g showed better antibacterial activity against Bacillus subtilis with MIC value of 4 μg mL−1, than insecticidal activity, compounds 4n, 4o and 4t exhibited more promising insecticidal activity with final mortality rates (FMRs) of >60%, when compared with their precursor fraxinellone and the positive control toosendanin. This suggested that introducing polyhalogenated phenylpyrazole at C-4 and C-5 positions of fraxinellone could lead to more promising insecticidal derivatives than monohalogenated phenylpyrazole, especially introducing N-(2-chloro-4-fluoro-phenyl)pyrazole.
Introduction
Pyrazole, bearing two nitrogen atoms as a five membered heteroaromatic ring has attracted considerable attention in the development of medicinal chemistry and pesticide chemistry.1,2 It has won great popularity due to its manifold use. In the field of medicinal chemistry, pyrazole and its analogues represent one of the most important skeletons of pharmaceutically active compounds, which display a broad spectrum of medicinal activities like antibacterial, antifungal, anticancer, anti-inflammatory, anticonvulsant, antitubercular and so on.3 Whereas in pesticide chemistry, they also exhibit a wide range of agro-activities, such as antimicrobial,4 herbicidal,5 insecticidal,2 acaricidal,6 etc. Fipronil (I, Fig. 1) is one of the most representative N-phenylpyrazole insecticides with great insecticidal activity, discovered by Rhone Poulenc Inc., in 1987 and launched to the market in 1993.7 Ethiprole (II, Fig. 1) known as the second generation insecticide for the insect γ-aminobutyric acid (GABA) receptor, is a newer fipronil derivative, developed by Bayer CropScience AG and launched in 1996.8 Subsequently, many fipronil analogues have been reported, for example, pyriprole (III, Fig. 1) and pyrafluprole (IV, Fig. 1) with better biological activity than fipronil, were discovered by Mitsubishi Chemical in 2004.9 Although these fipronil analogues pesticides play important roles in agriculture, the overuse and improper application over years have resulted in the development of pest resistance and other undesirable side effects on non-target organisms and environment.10,11 Nowadays, owing to fipronil toxicity to other non-target organisms, it has been restricted and even banned for some application in Europe, the United States and China.9 And for ethiprole, it also has been found that the Laodelphax striatellus populations resistant to fipronil had cross-resistance to ethiprole.9 Great care should thus be taken in using these synthetic pesticides where they may affect other non-target organisms. During course of evolution, the selection pressure caused by herbivorous animals and pathogens has probably been resulted in a enormous chemical diversity in higher plants. Unlike compounds synthesized from industrial chemicals, secondary compounds from plants are virtually guaranteed to have biological activity for protecting the plant from herbivore, pathogen and competitor.12,13 Using natural products as the lead compounds for further modification to develop new drugs or pesticides with novel structures and mechanism has recently been a popular topic and one of the most effective drug design methods.14,15
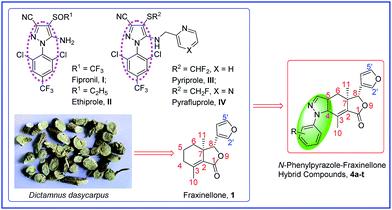 |
| Fig. 1 Structures of fipronil analogues, fraxinellone, and design of N-phenylpyrazole fraxinellone hybrid compounds. | |
Fraxinellone (1, Fig. 1) belonging to a small group of degraded limonoids, isolated from Dictamnus albus, Fagaropsis glabra, Melia azedarach and Dictamnus dasycarpus, has been found many interesting activities both in the fields of medicinal chemistry and agrochemistry such as anti-inflammatory, antimicrobial, insecticidal activity and so on.16–18 The acute toxicity of fraxinellone with LD50 value of 430 mg kg−1 for male mice by oral administration indicated that it also doesn't have high toxicity compared with those conventional synthetic chemical insecticides such as organophosphates, organochlorine pesticides etc.19 The structural modification of fraxinellone (1) was mainly reported by our research group,20–24 and we found that some of its derivatives exhibited higher insecticidal activity against M. separata than toosendanin, a commercial botanical insecticide. Inspired by the outstanding insecticidal activity of N-phenylpyrazole compounds mentioned above and characteristics of fraxinellone, and also in continuation our interest in developing novel natural products-based antimicrobial and insecticidal agents, herein we designed (Fig. 1) and prepared a series of novel N-phenylpyrazole fraxinellone hybrid compounds as antibacterial and insecticidal agents.
Experimental
Chemicals
Fraxinellone (1) was isolated from Dictamnus dasycarpus and intermediate 2 (Scheme 1) was synthesized as previously reported.20 Different substituted phenylhydrazine hydrochlorides were purchased from Macklin Biochemical Inc. (Shanghai, China). Toosendanin was obtained from Fortuneibo-tech Inc. (Shanghai, China). All reagents were of analytically grade and purchased from commercial resources. Anhydrous solvents were dried and purified according to standard methods before use. Analytical thin-layer chromatography (TLC) and preparative thin-layer chromatography (PTLC) were prepared by silica gel plates using silica gel GF254 (Qingdao Haiyang Chemical Co., Ltd., Qingdao, China).
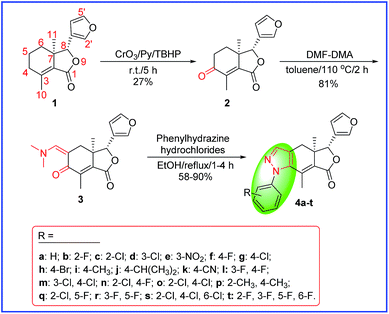 |
| Scheme 1 Synthetic route for the target compounds 4a–t. | |
Instrument
Melting points were determined on a digital melting-point apparatus and were uncorrected (Beijing Tech Instrument Co., Ltd., Beijing, China). Infrared spectra (IR) were recorded on a PE-1710 FT-IR spectrometer (Perkin-Elmer, Waltham, MA, USA). 1H/13C NMR spectra were carried out in CDCl3 on a Bruker Avance (400 MHz) spectrometer using tetramethylsilane (TMS) as the internal standard (Bruker, Bremerhaven, Germany). High-resolution mass spectra (HR-MS) were carried out with LTQ FT Ultra instrument (Thermo Fisher Scientific Inc., Waltham, MA, USA). Purity of all the target compounds was detected by a Waters e2695 HPLC Series System that had an automatic injector, a Waters 2489 UV/Vis detector and with Empower ChemStation software. (Waters, USA). Optical rotation was measured using an Autopol III automatic polarimeter (Rudolph Research Analytical, NJ, USA). X-ray crystallography was detected on a SMART APEX II equipment (Bruker, Karlsruhe, Germany).
General procedure for the synthesis of compound (3)
To a solution of fraxinellonone (2, Scheme 1) (1 mmol, 246 mg) in toluene was added dimethylformamide dimethyl acetal (DMF-DMA) [1.2 mmol, 143 mg] and the mixture was heated at 110 °C for 2.0 h. After cooling the mixture to RT, the crude product was concentrated in vacuo and purified by silica gel column chromatography eluting with petroleum ether/ethyl acetate (1
:
2, v/v) to afford compound 3 in 91% yield.
Data for 3. Orange solid, yield: 91%, mp: 66–68 °C; [α]20D = 88° (c 3.0 mg mL−1, CHCl3); IR cm−1 (KBr): 2963, 2919, 1757, 1717, 1639, 1547, 1208, 1025; 1H NMR (400 MHz, CDCl3) δ: 7.68 (s, 1H, H-2′), 7.49 (s, 1H, –CHN(CH3)), 7.46 (t, J = 1.6 Hz, 1H, H-5′), 6.35 (d, J = 1.2 Hz, 1H, H-4′), 5.11 (s, 1H, H-8), 3.12 (s, 6H, –CHN(CH3)), 2.97 (d, J = 13.2 Hz, 1H, H-6), 2.79 (d, J = 13.6 Hz, 1H, H-6), 2.25 (s, 3H, H-10), 0.93 (s, 3H, H-11); HRMS (ESI): calcd for C17H20O4N ([M + H]+), 302.1387; found, 302.1387.
General procedure for the synthesis of compounds (4a–t)
A mixture of compound 3 (0.15 mmol, 45.2 mg) and the corresponding substituted phenylhydrazine hydrochlorides (0.18 mmol) in ethanol was refluxed. When the reaction was complete according to the TLC analysis, the solvent was concentrated in vacuo and then purified by PTLC to afford the target compounds 4a–t in 58–90% yield. All the target compounds 4a–t were characterized by IR, 1H/13C NMR, HRMS and HPLC. Purity of all the target compounds 4a–t was >96%. Exemplary data of compounds 4a–d are shown as follows, others are included in the ESI.†
Data for 4a. Orange solid, yield: 83%, purity: 99%, mp: 85–87 °C; [α]20D = 4° (c 2.7 mg mL−1, CHCl3); IR cm−1 (KBr): 2961, 2923, 1736, 1629, 1502, 1164, 1024; 1H NMR (400 MHz, CDCl3) δ: 7.56 (s, 1H, –CH
N–), 7.52 (s, 1H, H-2′), 7.47–7.50 (m, 4H, –Ph and H-5′), 7.39–7.41 (m, 2H, –Ph), 6.39 (d, J = 1.2 Hz, 1H, H-4′), 5.19 (s, 1H, H-8), 2.80–2.89 (m, 2H, H-6), 2.05 (s, 3H, H-10), 0.91 (s, 3H, H-11); HRMS (ESI): calcd for C21H19O3N2 ([M + H]+), 347.1390; found, 347.1390.
Data for 4b. Pale yellow solid, yield: 70%, purity: 99%, mp: 204–206 °C; [α]20D = 25° (c 3.3 mg mL−1, CHCl3); IR cm−1 (KBr): 3132, 2962, 2925, 1741, 1631, 1512, 1217, 1041; 1H NMR (400 MHz, CDCl3) δ: 7.60–7.67 (m, 2H, –Ph and –CH
N–), 7.53 (s, 1H, H-2′), 7.45–7.52 (m, 2H, –Ph and H-5′), 7.31–7.35 (m, 1H, –Ph), 7.18–7.22 (m, 1H, –Ph), 6.39 (d, J = 0.8 Hz, 1H, H-4′), 5.19 (s, 1H, H-8), 2.79–2.92 (m, 2H, H-6), 2.07 (s, 3H, H-10), 0.90 (s, 3H, H-11); HRMS (ESI): calcd for C21H18O3N2F ([M + H]+), 365.1296; found, 365.1296.
Data for 4c. Yellow solid, yield: 73%, purity: 98%, mp: 160–162 °C; [α]20D = 11° (c 3.9 mg mL−1, CHCl3); IR cm−1 (KBr): 2967, 2926, 1741, 1631, 1498, 1162, 1024; 1H NMR (400 MHz, CDCl3) δ: 7.59–7.64 (m, 2H, –Ph and –CH
N–), 7.52 (s, 1H, H-2′), 7.45–7.50 (m, 4H, –Ph and H-5′), 6.39 (s, 1H, H-4′), 5.19 (s, 1H, H-8), 2.79–2.91 (m, 2H, H-6), 1.98 (s, 3H, H-10), 0.90 (s, 3H, H-11); HRMS (ESI): calcd for C21H18O3N2Cl ([M + H]+), 381.1000; found, 381.1000, C21H18O3N237Cl ([M + H]+), 383.0971; found, 383.0970.
Data for 4d. Yellow solid, yield: 65%, purity: 99%, mp: 167–169 °C; [α]20D = −14° (c 4.0 mg mL−1, CHCl3); IR cm−1 (KBr): 3114, 2970, 2926, 1741, 1592, 1160, 1025; 1H NMR (400 MHz, CDCl3) δ: 7.56 (s, 1H, –CH
N–), 7.53 (s, 1H, H-2′), 7.48 (t, J = 1.6 Hz, 1H, H-5′), 7.42–7.47 (m, 3H, –Ph), 7.28 (d, J = 7.6 Hz, 1H, –Ph), 6.39 (d, J = 0.8 Hz, 1H, H-4′), 5.19 (s, 1H, H-8), 2.80–2.88 (m, 2H, H-6), 2.10 (s, 3H, H-10), 0.92 (s, 3H, H-11); HRMS (ESI): calcd for C21H18O3N2Cl ([M + H]+), 381.1000; found, 381.0999, C21H18O3N237Cl ([M + H]+), 383.0971; found, 383.0971.
General procedure for the lactone hydrolysis of compounds (1, 4e, 4h and 4k)
To a solution of fraxinellone/4e/4h/4k (0.1 mmol, Fig. 9) in water/ethanol (3 mL, 2
:
1, v/v) and was added 0.1% aqueous NaOH (7 mL). The reaction mixture was stirred at room temperature. The reaction was complete until the lactone ring was opened and transformed into carboxylate according to TLC analysis.
Antibacterial assay
Minimal inhibitory concentrations (MICs) were determined by 2-fold serial dilution method in Mueller-Hinton (MH) broth (Beijing Aoboxing Bio-Tech Co., Ltd., Beijing, China) according to the guideline M07-A9 of the Clinical and Laboratory Standards Institute (CLSI).25 Bacterial strains were stored in 20% glycerol-Mueller-Hinton broth at −80 °C until further required for use. For MICs determination, a loopful of the stored bacterial fluid was directly streaked onto MH Agar. The plates were incubated at 37 °C for 12–16 h. A single colony was selected and transferred into a tube contained 1 mL MH broth, and then the broth culture was incubated at 37 °C with shaking at 200 rpm (Zhicheng shaker ZWY-1102C, Shanghai, China) until it achieves the turbidity of the 0.5 McFarland standard (usually 2–4 h). This results in a suspension containing approximately 1–2 × 108 colony-forming units (CFU) per mL. The colony forming units (CFU) were determined by the dropping method.26 Briefly, 10 μL appropriate dilutions of the bacterial suspension were dropped onto MH agar plate and then incubated at 37 °C overnight, after which the number of colonies on the plates is countable when 3–30 CFU in one drop. The bacterial suspension was adjusted with sterile saline to a concentration of 5 × 105 CFU mL−1 bacteria.27,28 The tested compounds 1–3, 4a–t and vancomycin (control drug) were dissolved in DMSO to prepare the stock solutions, then diluted in MH broth to obtain the required concentrations of 128, 64, 32, 16, 8, 4, 2, 1, 0.5 and 0.25 μg mL−1 in 96 well-plates. Two controls, one with bacterial suspension and DMSO at the same dilutions as used in the experiment, and the other with tested compounds solution with only medium, were made. The plates were incubated at 37 °C for 16–20 h. After incubation, optical density (OD) of the bacterial suspension was recorded using ELIASA at 600 nm (Synergy H1 Hybrid Microplate Reader, BioTek Instruments, Inc. VT, USA). Finally, the MIC value was recorded as the lowest concentration at which the OD values were found to be similar to that of only medium, and also at this concentration that visibly inhibited bacterial growth.
Insecticidal assay
Insecticidal activity of compounds 1, 3 and 4a–t against M. separata was evaluated by leaf-dipping method as described previously.20,23 For each compound, 30 pre-third-instar larvae (10 larvae per group) were used. Acetone solutions of fraxinellone (1), 3, 4a–t and toosendanin (used as a positive control) were prepared at the concentration of 1 mg mL−1. Fresh corn leaf dics (1 × 1 cm) were dipped into the corresponding solution for 3 s [a blank control group (CK) was treated with acetone alone], then taken out and dried in RT. Several treated leaf dics were kept in each dish. If the treated leaves were consumed, the corresponding ones were added to the dish. The experiment was carried out at [25 ± 2 °C; relative humidity (RH) 65–80%, and on 12 h/12 h (light/dark) photoperiod]. After 48 h, untreated fresh leaves were added to all dish until the adult emergence. The corrected mortality rate values of the tested compounds against the pre-third-instar larvae of M. separata was calculated by the following formula:
Corrected mortality rate (%) = (T − C) × 100/(1 − C) |
where T is the mortality rate in the treated group, and C is the mortality rate of the blank control group.
Result and discussion
Synthesis
The key intermediate enamino ketone 3 was obtained by the reaction of DMF-DMA with fraxinellonone (2), which was acquired as our reported procedure (Scheme 1).20 Then, the different substituent phenylhydrazine hydrochlorides was condensated with compound 3 in ethanol to provide the target compounds 4a–t. Their structures were determined by 1H/13C NMR, IR spectra, mp, optical rotation and HRMS. Additionally, the molecular structures of representative compounds 4h and 4r were confirmed unambiguously by X-ray crystallography (Fig. 2 and 3). Crystallographic data (excluding structure factors) of compounds 4h and 4r were deposited at the Cambridge Crystallographic Data Centre (CCDC) with publication no. CCDC 1483553 and 1483558, respectively. This suggested that we have successfully introduced the phenylpyrazole ring at C-4 and C-5 positions of fraxinellone and prepared a series of novel N-phenylpyrazole fraxinellone hybrid compounds 4a–t.
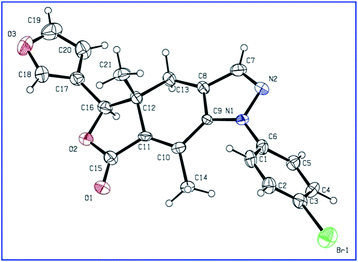 |
| Fig. 2 X-ray crystal structure of 4h. | |
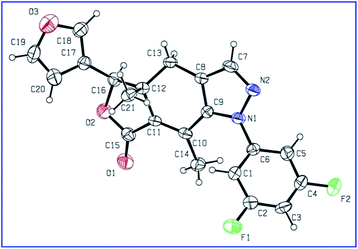 |
| Fig. 3 X-ray crystal structure of 4r. | |
Antibacterial activity
Currently, infectious diseases caused by drug-resistant Gram-positive/negative bacterial strains have been serious and growing threatens to human health.29 Based on the results depicted in Table 1, it was found that compound 4g with R = 4-Cl showed better activity against Gram-positive bacterial strain Bacillus subtilis with MIC value of 4 μg mL−1 among all the target compounds. Towards Bacillus subtilis, compounds 2, 4d, 4h and 4t also exhibited weak activity with MIC values of 64, 64, 32 and 64 μg mL−1, respectively. In addition, towards Gram-negative bacterial strains, all the N-phenylpyrazole fraxinellone hybrid compounds displayed poor activity with MIC values ≥128 μg mL−1 except compound 4b (MIC = 64 μg mL−1) showed weaker activity against Pseudomonas aeruginosa. This may be attributed to the probable prevention of these hybrid compounds to N-acetyl glucosamine (NAG) and N-acetyl muramic acid (NAM) from participating in the formation of peptidoglycan, which is the major cell wall components of Gram-positive bacterial strains30 (Fig. 4). In general, the antibacterial assay declared that most of the N-phenylpyrazole fraxinellone hybrid compounds exhibited less potent activity than vancomycin.
Table 1 Antibacterial activity (MIC, μg mL−1) of compounds 1–3 and 4a–t in vitroa
Compd |
Gram-positive bacterial strains |
Gram-negative bacterial strains |
S. a |
M. l |
B. s |
E. c |
S. m |
P. a |
S. a: Staphylococcus aureus; M. l: Micrococcus luteus; B. s: Bacillus subtilis; E. c: Escherichia coli; S. m: Stenotrophomonas maltophilia; P. a: Pseudomonas aeruginosa; VA: vancomycin (control drugs); MIC values determination was performed in triplicate. |
1 |
>128 |
>128 |
>128 |
>128 |
>128 |
>128 |
2 |
>128 |
>128 |
64 |
>128 |
>128 |
>128 |
3 |
>128 |
>128 |
>128 |
>128 |
>128 |
>128 |
4a |
>128 |
>128 |
>128 |
>128 |
>128 |
>128 |
4b |
64 |
>128 |
>128 |
128 |
>128 |
64 |
4c |
>128 |
>128 |
>128 |
>128 |
>128 |
128 |
4d |
>128 |
>128 |
64 |
>128 |
>128 |
>128 |
4e |
>128 |
>128 |
>128 |
>128 |
>128 |
>128 |
4f |
>128 |
>128 |
>128 |
>128 |
>128 |
>128 |
4g |
>128 |
>128 |
4 |
>128 |
>128 |
>128 |
4h |
>128 |
>128 |
32 |
>128 |
>128 |
>128 |
4i |
>128 |
>128 |
>128 |
>128 |
>128 |
>128 |
4j |
>128 |
>128 |
>128 |
>128 |
>128 |
>128 |
4k |
>128 |
>128 |
>128 |
>128 |
>128 |
>128 |
4l |
>128 |
>128 |
>128 |
>128 |
>128 |
>128 |
4m |
>128 |
>128 |
>128 |
>128 |
>128 |
>128 |
4n |
>128 |
>128 |
>128 |
>128 |
>128 |
128 |
4o |
>128 |
>128 |
>128 |
>128 |
>128 |
>128 |
4p |
>128 |
>128 |
>128 |
>128 |
>128 |
>128 |
4q |
>128 |
>128 |
>128 |
>128 |
>128 |
128 |
4r |
>128 |
>128 |
128 |
>128 |
>128 |
>128 |
4s |
>128 |
>128 |
>128 |
>128 |
>128 |
>128 |
4t |
128 |
128 |
64 |
>128 |
>128 |
128 |
VA |
2 |
0.5 |
0.25 |
64 |
64 |
128 |
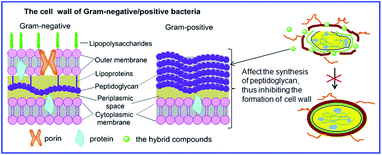 |
| Fig. 4 The possible antibacterial mechanism of the hybrid compounds. | |
Insecticidal activity
The insecticidal activity of compounds 1, 3 and 4a–t against the pre-third-instar M. separata, typical lepidopteran pest that can lead to widespread and extensive economic damage on food and fiber crop plants,31 was assessed as the final mortality rates (FMRs) at 1 mg mL−1 and outlined in Table 2. Among all the tested compounds, compounds 3, 4l, 4n, 4o, 4q, 4s, 4t showed more potent insecticidal activity than toosendanin, a commercial botanical pesticide derived from Melia azedarach. In addition, compounds 4k and 4r exhibited equivalent insecticidal potency to the positive control toosendanin (FMR = 51.7%), whereas which was higher than the parent compound 1 with FMR = 48.3%. What's more, compounds 4o and 4t displayed more potent insecticidal activity with FMRs >60%. Notably, compound 4n exhibited the most promising insecticidal activity with FMR of 75.9%, which was 24.2% higher than the toosendanin. On the other hand, as showed in Table 2, for most of compounds, rise in mortality rate during 10–20 days was higher than the first 10 days and 20–35 days. To further investigate this phenomenon, we collected the FMRs of compounds 3, 4k, 4l, 4n, 4o, 4q–t and toosendanin at three different growth stages of M. sepatata (Fig. 5), and found that the death of tested M. sepatata was mainly at the larval and pupation stage, and the percentage of which were about 30% and 50%, respectively. Due to feeding too much leaves carrying the tested compounds, part of larvaedied slowly with dehydration (Fig. 6). When the rest of larvae hit the pupation stage, many of them molt to malformed pupae with half larve and half pupae (Fig. 7), and these malformed pupae will finally die during the pupation stage. In the last adult stage, some moths have been malformed from eclosion and some moths appeared with shrinking or immature wings. All of them died to the end (Fig. 8). This suggested that these N-phenylpyrazole fraxinellone hybrid compounds probably affected the insect hormone. This phenomenon also was different with traditionally synthetic pesticides possessing the quick action and to kill the most of pests at the larval period,32 which may be more accordant with the concept of sustainable pest management (SPM) and friendly to ecosystem. Besides, the main skeleton of these hybrid compounds is fraxinellone, which belongs to the degrade limonoids that can be degraded by oxidation, hydrolysis and enzymolysis in nature.33 The 5-membered lactone and furyl-rings of limonoids can be opened by hydrolase or in alkaline environments,34,35 so we suppose that these hybrid compounds also could be degraded follow this principles. In order to verify one point of this hypothesis, we simulated the alkaline environments in nature by using 0.1% aqueous NaOH (7 mL) as base to hydrolyze the lactone rings of fraxinellone (1), compounds 4e, 4h and 4k in a solution of water/ethanol (3 mL, 2
:
1, v/v) under ambient temperature. The lactone rings of fraxinellone (1), compounds 4e, 4h and 4k were opened and transformed into the corresponding carboxylate after about 72–108 hours hydrolysis (Fig. 9), which can be easily dissolved in water. In addition, IR absorptions of compound 1′ exhibited the existence of OH group (3435 cm−1), whereas the fraxinellone (1) didn't showed the IR absorptions at 3435 cm−1 [(data for 1: IR cm−1 (KBr): 3148, 3130, 2930, 1741, 1671, 1607, 1202, 949) vs. 1′ (3435, 3150, 2941, 1700, 1667, 1414, 1204, 980)]. This proved that fraxinellone (1) was transformed into the corresponding carboxylate (1′). Inspired by these features, the natural products-based insecticidal agents may be attractive alternatives to those totally synthetic chemical insecticides for pest management in the future.
Table 2 Insecticidal activity of compounds 1, 3 and 4a–t against M. separata on leaves treated at a concentration of 1 mg mLa
Compd |
Corrected mortality rate (% ± SD) |
10 days |
20 days |
35 days |
Values are the mean ± SD of three replicates. |
1 |
16.7(±3.3) |
31.0(±3.3) |
48.3(±5.8) |
3 |
13.3(±3.3) |
41.4(±3.3) |
55.2(±3.3) |
4a |
10.0(±5.8) |
17.2(±5.8) |
34.5(±3.3) |
4b |
23.3(±3.3) |
41.4(±3.3) |
48.3(±5.8) |
4c |
10.0(±3.3) |
20.7(±3.3) |
44.8(±3.3) |
4d |
10.0(±0) |
27.6(±5.8) |
41.4(±3.3) |
4e |
10.0(±0) |
34.5(±3.3) |
34.5(±3.3) |
4f |
20.0(±0) |
34.5(±3.3) |
44.8(±6.7) |
4g |
6.7(±3.3) |
24.1(±3.3) |
37.9(±0) |
4h |
16.7(±3.3) |
24.1(±3.3) |
37.9(±0) |
4i |
6.7(±3.3) |
20.7(±3.3) |
27.6(±0) |
4j |
10.0(±0) |
24.1(±3.3) |
31.0(±3.3) |
4k |
20.0(±5.8) |
44.8(±3.3) |
51.7(±3.3) |
4l |
23.3(±3.3) |
41.4(±3.3) |
55.2(±3.3) |
4m |
20.0(±0) |
44.8(±3.3) |
48.3(±0) |
4n |
26.7(±3.3) |
62.1±(3.3) |
75.9 (±3.3) |
4o |
16.7(±3.3) |
44.8(±3.3) |
62.1(±3.3) |
4p |
6.7(±3.3) |
17.2(±0) |
24.1(±3.3) |
4q |
23.3(±3.3) |
44.8(±3.3) |
55.2(±3.3) |
4r |
16.7(±3.3) |
41.4(±3.3) |
51.7(±3.3) |
4s |
20.0(±0) |
44.8(±3.3) |
55.2(±6.7) |
4t |
23.3(±3.3) |
51.7(±3.3) |
65.5(±3.3) |
Toosendanin |
16.7(±3.3) |
41.4(±3.3) |
51.7(±3.3) |
Blank control |
0(±0) |
3.3.(±3.3) |
3.3(±3.3) |
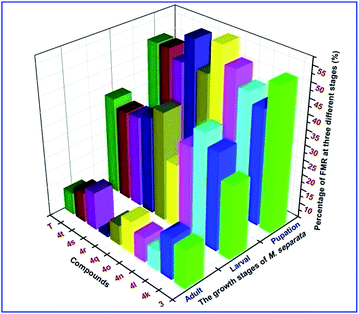 |
| Fig. 5 FMRs of compounds 3, 4k, 4l, 4n, 4o, 4q–4t and T (toosendanin) at three different growth stages of M. sepatata. | |
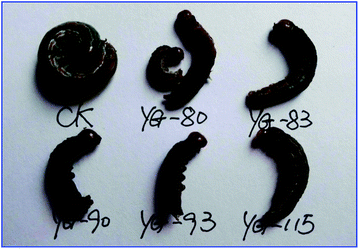 |
| Fig. 6 Representative abnormal larvae of M. separata. caused by compounds 4f (YG-80), 4n (YG-83), 4r (YG-90), 4s (YG-93) and 4k (YG-115) during the larval period (CK: blank control group). | |
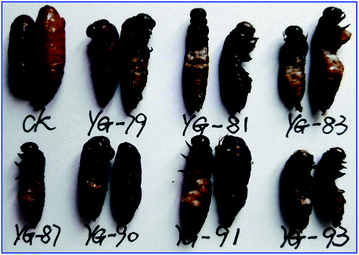 |
| Fig. 7 Representative malformed pupae of M. separata. caused by compounds 4b (YG-79), 4d (YG-81), 4n (YG-83), 4p (YG-87), 4r (YG-90), 4q (YG-91) and 4s (YG-93) during the pupation period (CK: blank control group). | |
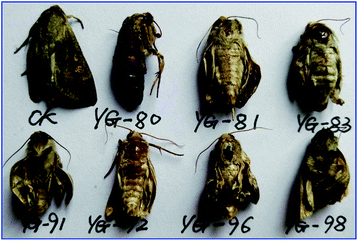 |
| Fig. 8 Representative malformed moth of M. separata. caused by compounds 4f (YG-80), 4d (YG-81), 4n (YG-83), 4q (YG-91), 4l (YG-92), 4t (YG-96) and 4h (YG-98) during the emergence period (CK: blank control group). | |
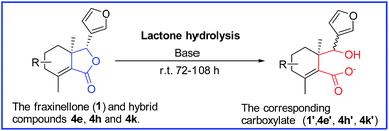 |
| Fig. 9 The possible pathway of lactone degradation of the fraxinellone-based derivatives. | |
Finally, on the basis of the results of insecticidal activity, the preliminary structure–activity relationships revealed that introduction of polyhalogenated phenylpyrazole at C-4 and C-5 positions of fraxinellone could lead more promising derivatives than monohalogenated phenylpyrazole. [e.g., FMRs: 4l (55.2%), 4r (51.7%) and 4t (65.5%) vs. 4b (48.3%) and 4f (44.8%); 4o (62.1%) and 4s (55.2%) vs. 4c (44.8%), 4d (41.4%) and 4g (37.9%)]. Interestingly, introduction of N-(2-chloro-4-fluoro-phenyl)pyrazole at C-4 and C-5 positions of fraxinellone showed much stronger insecticidal potency than that of N-(2-chloro-5-fluoro-phenyl)pyrazole (FMRs: 75.9% for 4n and 55.2% for 4q). To further study the structure–activity relationships of this series of novel fraxinellone derivatives, N-phenylpyrazole containing electron-donating and nitro groups were introduced at C-4 and C-5 positions of fraxinellone. However, the electron-donating and nitro groups are effective at declining the insecticidal activity (27.6% for 4i, 31.0% for 4j, 24.1% for 4p) in comparison with the parent compound 1.
Conclusions
In summary, a series of novel N-phenylpyrazole fraxinellone hybrid compounds modified at the C-4 and 5 positions of fraxinellone were synthesized and evaluated for their antibacterial and insecticidal activities. Two key steric configurations of compounds 4h and 4r were unambiguously determined by X-ray crystallography. Among all the derivatives, only compound 4g showed better activity against Bacillus subtilis with MIC value of 4 μg mL−1, the others showed poor antibacterial activity. Nevertheless, compounds 4n, 4o and 4t exhibited more promising insecticidal activity with the FMRs of >60%, when compared with their precursor fraxinellone and the positive control toosendanin. In addition, introduction of polyhalogenated phenylpyrazole at C-4 and C-5 positions of fraxinellone could lead more promising insecticidal derivatives than monohalogenated phenylpyrazole, especially introduction of N-(2-chloro-4-fluoro-phenyl)pyrazole. Current studies provide support for the application of fraxinellone derivatives as novel agrochemicals, and further studies on the development of novel fraxinellone derivatives containing nitrogen-bearing heterocyclic compounds are underway in our laboratories.
Acknowledgements
The present research was supported by National Natural Science Foundation of China (No. 21502176), the China Postdoctoral Science Foundation (No. 2015M582207), Postdoctoral Research Sponsorship in Henan Province (2015001) and Startup Research Fund of Zhengzhou University (145-51090119). We're very thankful to Dr Shangshang Qin (School of Pharmaceutical Sciences, Zhengzhou University) for his valuable advice in antibacterial activity.
Notes and references
- A. W. Brown, M. Fisher, G. M. Tozer, C. Kanthou and J. P. A. Harrity, J. Med. Chem., 2016, 59, 9473 CrossRef CAS PubMed.
- H. Song, Y. Liu, L. Xiong, Y. Li, N. Yang and Q. Wang, J. Agric. Food Chem., 2012, 60, 1470 CrossRef CAS PubMed.
- M. F. Khan, M. M. Alam, G. Verma, W. Akhtar and M. Akhter, Eur. J. Med. Chem., 2016, 120, 170 CrossRef CAS PubMed.
- D. Havrylyuk, O. Roman and R. Lesyk, Eur. J. Med. Chem., 2016, 113, 145 CrossRef CAS PubMed.
- T. W. Waldrep, J. R. Beck, M. P. Lynch and F. L. Wright, J. Agric. Food Chem., 1990, 38, 541 CrossRef CAS.
- H. Dai, G. Li, J. Chen, Y. Shi, S. Ge, C. Fan and H. He, Bioorg. Med. Chem. Lett., 2016, 26, 3818 CrossRef CAS PubMed.
- C. C. D. Tingle, J. A. Rother, C. F. Dewhurst, S. Lauer and W. J. King, Reviews of environmental contamination and toxicology, ed. W. W. George, Springer, New York, 2003 Search PubMed.
- P. Caboni, R. E. Sammelson and J. E. Casida, J. Agric. Food Chem., 2003, 51, 7055 CrossRef CAS PubMed.
- D. Jiang, X. Zheng, G. Shao, L. Zhang and H. Xu, J. Agric. Food Chem., 2014, 62, 3577 CrossRef CAS PubMed.
- M. Li, P. Li, L. Wang, M. Feng and L. Han, J. Agric. Food Chem., 2015, 63, 4435 CrossRef CAS PubMed.
- S. S. Walse, P. L. Pennington, G. I. Scott and J. L. Ferry, J. Environ. Monit., 2004, 6, 58 RSC.
- M. B. Isman, Annu. Rev. Entomol., 2006, 51, 45 CrossRef CAS PubMed.
- S. Miresmailli and M. B. Isman, Trends Plant Sci., 2014, 19, 29 CrossRef CAS PubMed.
- J. N. Seiber, J. Coats, S. O. Duke and A. D. Gross, J. Agric. Food Chem., 2014, 62, 11613 CrossRef CAS PubMed.
- I. Paterson and E. A. Anderson, Science, 2005, 310, 451 CrossRef PubMed.
- C. S. Lee, C. Won, H. Yoo, E. H. Yi, Y. Cho, J. W. Maeng, S. H. Sung, S. K. Ye and M. H. Chung, Biol. Pharm. Bull., 2009, 32, 1870 CAS.
- W. Zhao, J. L. Wolfender, K. Hostettmann, R. Xu and G. Qin, Phytochemistry, 1998, 47, 7 CrossRef CAS PubMed.
- Z. L. Liu, S. H. Ho and S. H. Goh, Insect Sci., 2009, 16, 147 CrossRef CAS.
- W. S. Woo, E. B. Lee, S. S. Kang, K. H. Shin and H. J. Chi, Planta Med., 1987, 53, 399 CrossRef CAS PubMed.
- Y. Guo, Y. Yan, X. Yu, Y. Wang, X. Y. Zhi, Y. Hu and H. Xu, J. Agric. Food Chem., 2012, 60, 7016 CrossRef CAS PubMed.
- Y. Guo, Y. Y. Yan, C. Yang, Y. Xiang, X. Y. Zhi and H. Xu, Bioorg. Med. Chem. Lett., 2012, 22, 5384 CrossRef CAS PubMed.
- Q. Li, X. Huang, S. Li, J. Ma, M. Lv and H. Xu, J. Agric. Food Chem., 2016, 64, 5472 CrossRef CAS PubMed.
- Y. Guo, H. Qu, X. Zhi, X. Yu, C. Yang and H. Xu, J. Agric. Food Chem., 2013, 61, 11937 CrossRef CAS PubMed.
- Y. Guo, R. Yang and H. Xu, Sci. Rep., 2016, 6, 35321 CrossRef PubMed.
- F. R. Cockerill, J. A. Hindler, J. B. Patel, J. Alder, M. Powell, M. N. Dudley, J. M. Swenson, G. M. Eliopoulos, R. B. Thomson, M. J. Ferraro, M. M. Traczewski, D. J. Hardy, J. D. Turnidge, D. W. Hecht, M. P. Weinstein and B. L. Zimmer, Methods for dilution antimicrobial susceptibility tests for bacteria that grow aerobically; approved standard–ninth edition: M07–A9, Clinical and Laboratory Standards Institute (CLSI), Wayne, PA, 2012 Search PubMed.
- B. Herigstad, M. Hamilton and J. Heersink, J. Microbiol. Methods, 2001, 44, 121 CrossRef CAS PubMed.
- K. Murakami, W. Minamide, K. Wada, E. Nakamura, H. Teraoka and S. Watanabe, J. Clin. Microbiol., 1991, 29, 2240 CAS.
- S. Ghosh, V. S. Goudar, K. G. Padmalekha, S. V. Bhat, S. S. Indic and H. N. Vasan, RSC Adv., 2012, 2, 930 RSC.
- A. J. Huh and Y. J. Kwon, J. Controlled Release, 2011, 156, 128 CrossRef CAS PubMed.
- K. Hiramatsu, Drug Resist. Updates, 1998, 1, 135 CrossRef CAS PubMed.
- E. Han and A. G. Gatehouse, Evolution, 1991, 45, 1502 CrossRef.
- D. J. Ecobichon, Neurotoxicology, 1999, 21, 211 Search PubMed.
- S. Hasegawa, J. Agric. Food Chem., 1976, 24, 24 CrossRef CAS PubMed.
- D. Lavie, E. C. Levy and M. K. Jain, Tetrahedron, 1971, 27, 3927 CrossRef CAS.
- S. Hasegawa and A. D. King Jr, J. Agric. Food Chem., 1983, 31, 807 CrossRef CAS.
Footnote |
† Electronic supplementary information (ESI) available. CCDC 1483553 and 1483558. For ESI and crystallographic data in CIF or other electronic format see DOI: 10.1039/c6ra28064a |
|
This journal is © The Royal Society of Chemistry 2017 |
Click here to see how this site uses Cookies. View our privacy policy here.