DOI:
10.1039/C6RA28009A
(Paper)
RSC Adv., 2017,
7, 14998-15004
Green synthesis of 1-phenyl-1-ortho-xylene ethane in IL and reaction mechanism†
Received
10th December 2016
, Accepted 23rd February 2017
First published on 7th March 2017
Abstract
1-Phenyl-1-ortho-xylene ethane (PXE) in IL was synthesized, and the catalysts used were AlCl3 in 1-butyl-3-methylimidazolium bromide ([BMIM][Br]) or 1-butyl-3-methylimidazolium chloride ([BMIM][Cl]), and H2SO4. When the concentrations of AlCl3 in IL, ortho-xylene and styrene were 0.35, 0.03 and 0.005 mol, respectively, it could be discovered that ([BMIM][Br]) was the best catalyst. When the mole fraction concentration of AlCl3/[BMIM][Br] was more than 0.40%, the reaction was able to achieve 100% conversion. When the reaction time was longer than 60 minutes, the percentage conversion of ortho-xylene attained was 100%. The mechanisms of the reaction were investigated by the DFT method at the B3LYP/6-311++G(d, p) level. The potential energy surface (PES) profile indicated that the energy of transition state was 22.59 kcal mol−1. This indicated that the activation energy of reaction was 28.24 kcal mol−1, which illustrated that the reaction occurred easily. Vibrational frequencies were observed in FT-IR spectra, the corresponding vibrational modes νs(H4–C3Al–H5) and νas(H4–C3Al–H5) indicated that an Al3+ ion was combined with a C3 atom. In addition, the repeated use of the catalysts was studied; the ionic liquids could still catalyze the reaction and the percentage yield of PXE was larger than 91% after 6 runs.
1. Introduction
1-Phenyl-1-ortho-xylene ethane (PXE) and bis(1-phenyl ethanyl) ortho-xylene (BPXE) are synthesis liquids with excellent performance because of their high boiling points, low viscosity, high solubility, low melting points, high-color printer's ability, high dielectric strengths and electrothermal stability, etc., and are widely used in pressure sensitive dye solvents of carbonless paper and electrical insulating oil. In addition, they can also be used as a plasticizer, transformer oil, and other high boiling point solvents. Current commercial alkylation processes are catalyzed primarily by concentrated sulfuric acid or hydrofluoric acid.1 The reaction equation is as follows:
The sulfuric acid process produces large amounts of spent acid and acid-soluble oils, which cause serious environmental problems. Anhydrous HF is highly toxic and its leakage produces dangerous stable aerosols at the ground level. In addition, equipment corrosion, transport, hazard management and environmental liability associated with the disposal of spent acids are disadvantages of both processes. To avoid pollution a more environmentally friendly catalyst is desired.2,3
Room temperature ionic liquid (IL) systems have many unique properties such as a very large liquid temperature range, and they can be made task-specific for certain applications,4 which include use as solvents in chemical synthesis and catalysis5–11 and separation technology,12,13 as electrolytes in electrochemical devices,14–16 and as lubricants or as heat-transfer fluids.17 In addition, there is a huge range of possible combinations of cations and anions, which contribute to the design of ILs.18 However, before these liquids can be used commercially on an industrial scale, the process variables need to be optimized in the laboratory, not only in batch and continuous reactor systems for maximum reactant conversion, but also the product yield and catalyst selectivity. Previous studies have demonstrated that the activity of such systems strongly depends on the molar ratio of AlCl3/IL. Usually, maximal activity can be obtained at a molar ratio of 1.5–2.0 of AlCl3 to IL.19,20
This study reports that 1-phenyl-1-ortho-xylene ethane (PXE) is synthesized in IL and the catalysts used are AlCl3 in 1-butyl-3-methylimidazolium bromide ([BMIM][Br]) or 1-butyl-3-methylimidazolium chloride ([BMIM][Cl]). The reaction mechanism presented in this work is drawn in the following scheme.
The catalytic activity of a Lewis acid-IL is studied under different experimental conditions, such as different catalysts, concentrations, temperatures and reaction times. The catalysts are facilely isolated from products and effectively recycled. The mechanism of the reaction is determined using quantum chemical calculations and vibrational frequencies are observed in FT-IR spectra. The repeated use of the catalysts is also investigated.
2. Experiments
2.1 Materials
High-purity ortho-xylene, styrene and anhydrous AlCl3 were used in this study and purchased from the Tianjin Damao Chemical Reagent Factory (Tianjin, China) and Chinese Medicine Group Chemical Reagent Co, Ltd (Beijing, China). [BMIM][Cl] (CAS no. 79
917-90-1) and [BMIM][Br] (CAS no. 85
100-77-2) were purchased from the Lanzhou (Lanzhou, China) Center for Green Chemistry with a purity of 99% and water mass fractions of less than 10−3 (g g−1). To remove traces of volatile compounds, the ILs were kept in a vacuum (about 10−6 bar) at 343 K for 24 h prior to use.
2.2 Apparatus and measurements
An Agilent6890 (Agitent Co. USA) gas chromatograph equipped with a flame ionization detector (FID) was employed for analysis. The detector temperature was maintained at 240 °C. The column was programmed with an initial temperature of 60 °C and this was increased thereafter to 220 °C at a rate of 15 °C min−1. The column used for this purpose was HP-1 with dimensions of 50 m × 0.25 mm × 0.50 μm.
To support the computational results, Fourier transform infrared (FT-IR) spectra of 2BP8HQ were recorded using a Nicolet iS5 FT-IR spectrometer (4000–525 cm−1; number of scans: 250; resolution: 1 cm−1) to study the variations of the IR spectra of ortho-xylene and styrene, and the reaction processes.
2.3 Computational methods
The reactant (R), intermediate (IM) and transition states (TS) and formed products (P) were fully optimized using the B3LYP/6-311++G(d, p) basis set, containing polarizable and diffuse functions, and this well described the weak interactions in the binary systems, especially for intermolecular H-bonded interactions. The interaction energy (DE) was estimated by employing the counterpoise (CP) method for the Gaussian basis set superposition error (BSSE).21 From intrinsic reaction coordinate (IRC) calculations and the potential surface (PES) energy profile for the transition state, two desired minima states of the mechanism were obtained. All the DFT calculations were carried out using the Gaussian09 package.22
3. Results and discussion
3.1 Solubility of AlCl3 in [BMIM][Cl] and [BMIM][Br]
A given amount of anhydrous AlCl3 is slowly added to 3.00 g of [BMIM][Cl] and [BMIM][Br] and the mixture is stirred at different temperatures until the catalyst is completely dissolved. AlCl3/[BMIM][Cl] and AlCl3/[BMIM][Br] ionic liquids are thus obtained. The solubility of AlCl3 in [BMIM][Cl] and [BMIM][Br] is shown in Table 1. It can be seen that the solubility of AlCl3 increased with the increase of temperature. The solubility of AlCl3 in [BMIM][Br] is higher than that in [BMIM][Cl]. The saturation points of AlCl3 in [BMIM][Cl] and [BMIM][Br] are around 50 °C and 80 °C, respectively. Experimental results also show that the solubility of AlCl3 in [BMIM][Cl] and [BMIM][Br] is larger in the latter than in the former. Since the catalytic properties of these binary mixtures depend on their acidities, we can conclude that the catalytic activity of the latter is stronger than the former, which can be adjusted by the molar ratio of AlCl3/IL.
Table 1 Solubility of AlCl3 in [BMIM][Cl] and [BMIM][Br]
Temp. (°C) |
[BMIM][Cl] |
[BMIM][Br] |
Solutiona |
Contentb |
Solubility (%) |
solutiona |
Contentb |
Solubility (%) |
The concentration is the mole concentration. Molar content of AlCl3 (X(AlCl3)) = ([AlCl3]/([AlCl3] + IL)). |
20 |
0.00056 |
0.029792 |
0.0330 |
0.00643 |
0.31936 |
0.4628 |
30 |
0.00061 |
0.032366 |
0.0350 |
0.00719 |
0.344118 |
0.5249 |
40 |
0.00068 |
0.035947 |
0.0400 |
0.00824 |
0.375501 |
0.6015 |
50 |
0.00075 |
0.039501 |
0.0430 |
0.00862 |
0.386132 |
0.6292 |
60 |
0.00078 |
0.041016 |
0.0450 |
0.00877 |
0.390229 |
0.6402 |
70 |
0.00078 |
0.041016 |
0.0450 |
0.00900 |
0.396406 |
0.6570 |
80 |
0.00078 |
0.041016 |
0.0450 |
0.00952 |
0.409921 |
0.6950 |
90 |
0.00078 |
0.041016 |
0.0450 |
0.00952 |
0.409921 |
0.6950 |
3.2 Effects of different catalysts on the reaction
Different catalysts were added to, under the conditions of stirring, solutions of styrene and ortho-xylene dropwise; the reaction is performed in an 80–85 °C oil bath. TLC (petroleum ether as a solvent) was used to monitor the progress of the reaction. After the completion of the reaction, the reaction liquid was left standing and allowed to cool; the lower layer was separated. The ionic liquid catalyst is rapidly added to small amounts of ortho-xylene for the next use. ortho-Xylene is distilled and recovered, and the distillation product is collected at 208–214 °C.
When the concentration of AlCl3 in IL, ortho-xylene and styrene are 0.35, 0.03 and 0.005 mol, respectively, the reactions are performed at 30 °C, the effects of different catalysts on the reaction are shown in Table 2. It can be seen that the orders of yield are AlCl3/[BMIM][Br] (99%) > AlCl3/[BMIM][Cl] (73%) > H2SO4 (56%), which indicated that the yields of AlCl3 in [BMIM][Cl] and [BMIM][Br] are larger than that of H2SO4, especially, the yield of AlCl3/[BMIM][Br] reaches 99%. This illustrates that AlCl3/[BMIM][Br] is the best catalyst for the alkylation reaction of ortho-xylene and styrene. Hence, AlCl3/[BMIM][Br] is used as the catalyst in the following experiments.
Table 2 Effects of different catalysts on the reactiona
Entry |
Catalyst |
Time (min) |
Conversion (%) |
Selectivity (%) |
Yieldb (%) |
PXE |
BPXE |
Reaction conditions: ortho-xylene (0.03 mol), styrene (0.005 mol) and catalyst (0.5 g) at 30 °C. GC yield was shown. The mole ratio of AlCl3 to IL is 0.35. |
1 |
H2SO4 |
60 |
45 |
47.6 |
9.5 |
56 |
2 |
AlCl3/[Bmim][Cl]c |
60 |
85 |
57 |
16 |
75 |
3 |
AlCl3/[Bmim][Br]c |
60 |
100 |
69 |
30 |
98 |
3.3 Effects of different mole fractions of AlCl3/[BMIM][Br] on the reaction
When the amount of ortho-xylene and styrene is 0.03 and 0.005 mol, respectively, the reactions are carried out at 30 °C, and the effect of the different mole fractions of AlCl3/[BMIM][Br] on the reaction is listed in Table 3. It can be seen that the percentage conversion of ortho-xylene increased with the increase of the mole fraction of AlCl3/[BMIM][Br], and when the mole fraction of AlCl3/[BMIM][Br] is larger than 0.40%, the percentage conversion of ortho-xylene attained was 100%. This shows that as long as the mole fraction concentration of AlCl3/[BMIM][Br] is more than 0.40%, the reaction will be able to achieve 100% conversion, and moreover, the rates of formation of PXE and BPXE increased with the increase of the mole fraction of AlCl3 to [BMIM][Br]. The 1H NMR spectrum for a mixture of PXE and BPEX with a 1
:
0.36 ratio is shown in Fig. S1 (ESI†). 1H NMR (500 MHz, CDCl3): δ 7.35–7.20 (m, 3 + 2.13H), 7.20–7.10 (m, 4 + 1.42H), 7.08–6.90 (m, 1 + 0.71H), 4.27 (q, J = 7.2 Hz, 1H), 4.12 (q, J = 7.2 Hz, 0.71H), 2.29 (s, 3H), 2.21 (s, 2.13H), 2.18 (s, 3H), 1.63 (d, J = 7.2 Hz, 2.13H), 1.58 (d, J = 7.2 Hz, 3H). The results are in agreement with the data in ref. 23.
Table 3 Effects of different mole fractions of AlCl3/[Bmim]Br on the reactiona
Entry |
AlCl3/[Bmim][Br] (mole ratio) |
Time (min) |
Conversion (%) |
Selectivity (%) |
Yieldb (%) |
PXE |
BPXE |
Reaction conditions: ortho-xylene (0.03 mol), styrene (0.005 mol) and catalyst (0.5 g) at 30 °C. GC yield is shown. |
1 |
0.28 |
60 |
24 |
24 |
1.3 |
17 |
2 |
0.32 |
60 |
26 |
28 |
1.6 |
21 |
3 |
0.35 |
60 |
30 |
35 |
3.5 |
25 |
4 |
0.38 |
60 |
84 |
59 |
6.9 |
70 |
5 |
0.39 |
60 |
97 |
67 |
21 |
92 |
6 |
0.40 |
60 |
100 |
70.6 |
24 |
98 |
3.4 Effects of different temperatures on the reaction
When the amount of ortho-xylene and styrene was 0.03 and 0.005 mol, respectively, the reaction time was 60 minutes and the mole fraction of AlCl3/[BMIM][Br] was 0.40%. The reactions were carried out, and the effects of different temperatures on the reaction are given in Table 4. It can be seen that the percentage conversion of ortho-xylene increased with the increase of temperature, and when the temperature is higher than 60 °C, the percentage conversion of ortho-xylene attained is 100%. This illustrates that 60 °C is enough for the reaction. At the same time, the rates of formation of PXE and BPXE increased with temperature, which do not vary when the temperature is 60 °C.
Table 4 Effects of different temperatures on the reactiona
Entry |
Temperature (°C) |
Conversion (%) |
Selectivity (%) |
Yieldb (%) |
PXE |
BPXE |
Reaction conditions: ortho-xylene (0.03 mol), styrene (0.005 mol) and AlCl3/[Bmim][Br] (0.5 g, mole ratio: 0.40) at the indicated temperature for 60 minutes. GC yield is shown. |
1 |
10 |
15 |
14 |
0.6 |
12 |
2 |
30 |
97 |
67 |
21 |
92 |
3 |
50 |
99 |
69 |
28 |
96 |
4 |
70 |
100 |
69 |
30 |
98.3 |
5 |
90 |
100 |
69 |
30 |
98.6 |
6 |
100 |
100 |
69 |
30 |
99 |
3.5 Effects of different reaction times on the reaction
When the amount of ortho-xylene and styrene is 0.03 and 0.005 mol, respectively, and the mole fraction of AlCl3/[BMIM][Br] is 0.40%, the reactions are carried out at 70 °C, and the effects of different reaction times on the reaction are shown in Table 5. It can be seen that the percentage conversion of ortho-xylene increased with reaction time, and when the reaction time is longer than 60 minutes, the percentage conversion of ortho-xylene attained is 100%. Consequently, according to the above results, the synthesis conditions for obtaining 1-phenyl-1-ortho-xylene ethane are that the amounts of ortho-xylene and styrene are 0.03 and 0.005 mol, respectively, the mole fraction of AlCl3/[BMIM][Br] is 0.40%, the reaction time is 60 minutes and the reactions are carried out at 70 °C.
Table 5 Effects of different reaction times on the reactiona
Entry |
Reaction time (min) |
Conversion (%) |
Selectivity (%) |
Yieldb (%) |
PXE |
BPXE |
Reaction conditions: ortho-xylene (0.03 mol), styrene (0.005 mol) and AlCl3/[Bmim][Br] (0.5 g, mole ratio: 0.40) at 70 °C. GC yield is shown. |
1 |
10 |
18 |
15 |
1.8 |
15 |
2 |
30 |
97 |
67 |
21 |
92 |
3 |
60 |
100 |
69 |
28 |
97.5 |
4 |
90 |
100 |
69 |
30 |
98.6 |
5 |
100 |
100 |
69 |
30 |
99 |
3.6 Reaction mechanism
3.6.1 Mechanism of the reaction. Quantum chemical calculations are a useful tool to predict the reaction mechanisms and kinetics of the alkylation reaction. Styrene (R1) and ortho-xylene (R2) have been fully optimized at the B3LYP/6-311++G(d, p) level, the geometrical parameters involving various species of R1, R2, IM, TS and P are shown in Fig. 1 and S2 (ESI†), and the important geometrical parameters are given in Table 6. It can be seen that styrene has a Π88 conjugated structure; for R, the bonds of C1–C2 and C2–C3 are averaged which are 1.390 and 1.486 (Å), respectively. When an Al3+ ion is combined with a C3 atom in styrene, the length of C3–Al3+ is 2.101 (Å), and the obtained IM joined with ortho-xylene. This illustrates that the Al3+ ion plays an important role in the alkylation processes of styrene and ortho-xylene. From IRC calculations for the transition state (TS), we obtain two desired minima states of the process. This indicates a transition state, and it has an imaginary frequency (−182.63 cm−1), the geometrical parameters of the TS are shown in Fig. 1. In the TS, a C2–H4–C7–H8 four-membered ring is formed, the distances of the bonds are 1.097, 2.562, 1.086 and 2.585 (Å), respectively, which provides helpful information on the formation of the C2–C7 bond. The bond lengths of C1–C2, C2–C3 and C2–C7 are 1.553, 1.576 and 1.600 (Å), respectively, which indicates that those bonds are stretched by steric effects of two benzene rings.
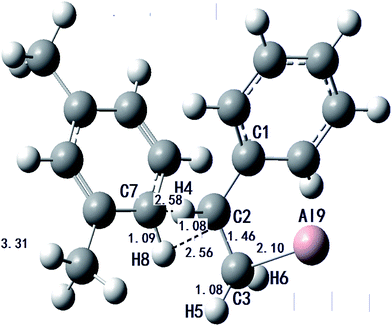 |
| Fig. 1 Geometrical parameters of the TS. | |
Table 6 Important geometrical parameters of the R, IM, TS and P (Å)
Bond length |
Reactant |
Intermediate |
Transition state |
Product |
C1–C2 |
1.390 |
1.395 |
1.473 |
1.553 |
C2–C3 |
1.486 |
1.460 |
1.460 |
1.576 |
C2–H4 |
1.090 |
1.098 |
1.097 |
1.091 |
C3–H5 |
1.100 |
1.100 |
1.097 |
1.091 |
C3–H6 |
1.081 |
1.087 |
1.088 |
1.097 |
C7–H8 |
1.087 |
1.087 |
1.086 |
1.110 |
C3–Al9 |
|
2.101 |
2.101 |
1.965 |
C2–C7 |
|
|
2.319 |
1.600 |
C2–H8 |
|
|
2.585 |
|
C7–H4 |
|
|
2.562 |
|
C10-A9 |
|
|
|
2.054 |
The potential energy surface (PES) profile is depicted in Fig. 2. The energy of the IM is lower than that of the reactant by 5.65 kcal mol−1, we can also find that the energy of the P is higher than that of the IM by 15.69 kcal mol−1. This result indicates that the IM is more stable than the P. The energy of the TS is 22.59 kcal mol−1, and this indicates that the activation energy of the alkylation reaction is 28.24 kcal mol−1. This illustrates that the reaction occurs easily, which is in agreement with the experimental results.
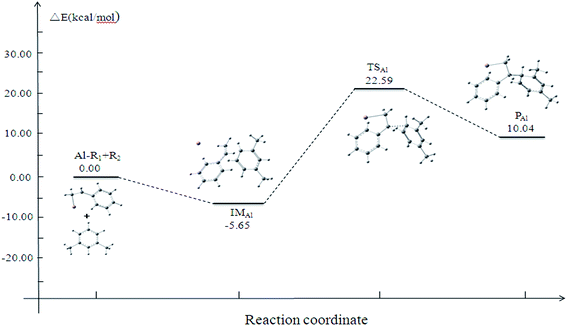 |
| Fig. 2 Energy profiles of the R, IM, TS and P. | |
3.6.2 Vibrational frequencies. Table 7 lists the main vibration variations of styrene, styrene + ortho-xylene + AlCl3 + [BMIM][Br] and PXE as well as the IR intensity observed in FT-IR spectra; the corresponding infrared spectra are shown in Fig. S3–S5.† It can be seen that the infrared peak appearing at 1710.33 cm−1 is assigned to the symmetrical stretching vibration of (C2–C3) in styrene. When AlCl3 in [BMIM][Br] is added to styrene and ortho-xylene, the symmetrical stretching vibration of (C2–C3) in styrene is red shifted from 1710.33 cm−1 to 1106.60 cm−1, which illustrates that the Al3+ ion combines with the C3 atom and decreases the C2–C3 bond strength. The infrared peaks 3165.10 and 3247.26 cm−1 are attributed to νs(H5–C3–H6) and νas(H4–C3–H5), respectively, which are also red shifted to 3026.38 and 3067.59 cm−1, the corresponding vibrational modes are νs(H4–C3Al–H5) and νas(H4–C3Al–H5), respectively. This indicates that the Al3+ ion joins to form a C3–Al3+ bond, which weakens the C3–H4 and C4–H5 bonds. In the product (PXE) the infrared vibrational frequencies 3045.83 and 3119.25 cm−1 correspond to the vibrational modes νs(H5–C3H7–H6) and νas(H5–C3H7–H6), respectively, and the blue shifts of these vibrational frequencies illustrate that Al3+ has been substituted for H7. The infrared peak at 991.48 cm−1 is the symmetrical stretching vibration of (C2–C3), which may be due to further weakening caused by product formation. The appearance of the vibrational frequency at 3053.79 cm−1, assigned to the symmetrical stretching vibration of (C2–C7), demonstrates a new bond formation between styrene and ortho-xylene.
Table 7 Main vibration spectrum variations of styrene, ortho-xylene, styrene + ortho-xylene + AlCl3 + [BMIM][Br] and PXE
Species |
Vibrational mode assignment |
Frequency (cm−1) |
IR intensity |
Styrene |
νs(C2–C3) |
1710 |
9.16 |
νs(H4–C2) |
3146 |
16.20 |
νs(H5–C3–H6) |
3165 |
2.99 |
νas(H4–C3–H5) |
3247 |
14.99 |
ortho-Xylene |
νs(C7–H8) |
3077 |
27.10 |
νs(C2–C3) |
1106 |
12.33 |
Styrene + ortho-xylene + AlCl3 + [BMIM][Br] |
νs(H4–C3Al–H5) |
3026 |
26.90 |
νas(H4–C3Al–H5) |
3067 |
2.28 |
νs(C7–H8) |
3161 |
9.30 |
νs(H4–C2) |
3190 |
7.86 |
νs(C2–C3) |
991 |
0.50 |
νs(C2–C7) |
1084 |
10.36 |
PXE |
νs(H5–C3H7–H6) |
3045 |
23.83 |
νs(H4–C2) |
3053 |
15.26 |
νas(H5–C3H7–H6) |
3119 |
29.02 |
3.7 Repeated use of the catalyst
When the amount of ortho-xylene and styrene is 0.03 and 0.005 mol, respectively, and the mole fraction of AlCl3/[Bmim][Br] is 0.40%, the reactions are carried out at 70 °C with a reaction time of 1.5 hours. After the reaction, the supernatant liquid is poured out. In order to remove ortho-xylene and volatile organic compounds from the ionic liquid, the reaction vessel was put under vacuum, and then the weight of the raw materials was added. The results are listed in Table 8. It can be seen that the ionic liquid can still catalyze the reaction and the percentage yield of PXE is larger than 91% after 6 runs. The activity of the catalyst decreased gradually after 6 runs.
Table 8 Catalyst performance in ionic liquid for repeated operations
Run |
Percentage conversion (%) |
Selectivity (%) |
Yield (%) |
PXE |
BPXE |
1 |
98.5 |
69.7 |
28.3 |
92.8 |
2 |
98.4 |
69.2 |
28.3 |
93.2 |
3 |
98.2 |
69.1 |
28.0 |
92.4 |
4 |
97.8 |
68.5 |
27.5 |
91.8 |
5 |
97.5 |
68.3 |
27.4 |
91.8 |
6 |
96.6 |
67.9 |
26.8 |
91.1 |
7 |
72.3 |
47.7 |
20.5 |
62.0 |
According to Table 8, the activity of the catalyst began to decrease after 3–4 cycles. The main explanations for this are leaching of AlCl3 from the binary mixture and catalyst poisoning by the reaction products and cokes. Measures can be taken to improve the catalytic activity, such as vacuum dehydration, solvent rinsing and the addition of AlCl3. Besides this, further study has been carried out by our group aiming to improve the catalytic efficiency.
4. Conclusions
In conclusion, green synthesis of 1-phenyl-1-ortho-xylene ethane in IL and the reaction mechanism are studied. The effects of different catalysts on the reaction are considered, when the amount of AlCl3 in IL, ortho-xylene and styrene are 0.35, 0.03 and 0.005 mol, respectively, the reactions are performed at 30 °C, and the order of the yield is AlCl3/[Bmim][Br] (99%) > AlCl3/[Bmim][Cl] (73%) > H2SO4 (56%). The effects of different mole fractions of AlCl3/[Bmim][Br] on the reaction are investigated; results show that as long as the mole fraction concentration of AlCl3/[Bmim][Br] is more than 0.40%, the reaction will be able to achieve 100% conversion. The effects of different temperatures on the reaction are studied; the percentage conversion of ortho-xylene was increased with the increase of temperature. When the temperature is higher than 60 °C, the percentage conversion of ortho-xylene attained is 100%. The effects of different reaction times on the reaction are investigated; when the reaction time is longer than 60 minutes, the percentage conversion of ortho-xylene attained is 100%. The mechanism of the reaction is discussed by quantum chemical calculations and vibrational frequencies are observed in FT-IR spectra. In addition, the repeated use of the catalyst is considered, the ionic liquid can still catalyze the reaction and the percentage yield of PXE is larger than 91% after 6 runs. The activity of the catalyst decreased gradually after 6 runs. The activity of the catalyst can be improved by vacuum dehydration, solvent rinsing and the addition of anhydrous AlCl3, which is being studied.
Acknowledgements
This work was supported by the National Natural Science Foundation of China (No. 21206085 and 21203250).
References
- G. A. Olah, Friedel-Crafts Chemistry, John Wiley and Sons, New York, 1973 Search PubMed
. - K. Qiao and Y. Deng, Alkylations of benzene in room temperature ionic liquids modified with HCl, J. Mol. Catal. A: Chem., 2001, 171, 81–84 CrossRef CAS
. - E. C. Polo, L. C. d. Silva-Filho, G. V. J. d. Silva and M. G. Constantino, Synthesis of 1-indanones through the intramolecular Friedel–Crafts acylation reaction using NbCl5 as Lewis acid, Quim. Nova, 2008, 31, 763–766 CrossRef CAS
. - T. Mu and B. Han, Structures and thermodynamic properties of ionic liquids, in Structures and interactions of ionic liquids, ed. S. Zhang, J. Wang, X. Lu and Q. Zhou, Springer, Berlin, 2014, vol. 151, pp. 107–139 Search PubMed
. - H. Sun, B. Cao, Q. Tian, S. Liu, D. Du, Z. Xue and H. Fu, A DFT study on the absorption mechanism of vinyl chloride by ionic liquids, J. Mol. Liq., 2016, 215, 496–502 CrossRef CAS
. - B. Cao, S. Liu, D. Du, Z. Xue, H. Fu and H. Sun, Experiment and DFT studies on radioiodine removal and storage mechanism by imidazolium-based ionic liquid, J. Mol. Graphics Modell., 2016, 64, 51–59 CrossRef CAS PubMed
. - G. Li, C. Yan, B. Cao, J. Jiang, W. Zhao, J. Wang and T. Mu, Highly efficient I2 capture by simple and low-cost deep eutectic solvents, Green Chem., 2016, 18, 2522–2527 RSC
. - B. Cao, J. Du, S. Liu, X. Zhu, X. Sun, H. Sun and H. Fu, Carbon dioxide capture by amino-functionalized ionic liquids: DFT based theoretical analysis substantiated by FT-IR investigation, RSC Adv., 2016, 6, 10462–10470 RSC
. - H.-P. Steinrück and P. Wasserscheid, Ionic liquids in catalysis, Catal. Lett., 2015, 145, 380–397 CrossRef
. - X. Sun, B. Cao, X. Zhou, S. Liu, X. Zhu and H. Fu, Theoretical and experimental studies on proton transfer in acetate-based protic ionic liquids, J. Mol. Liq., 2016, 221, 254–261 CrossRef CAS
. - X. Zhou, B. Cao, S. Liu, X. Sun, X. Zhu and H. Fu, Thermal reaction of the ionic liquid 1,2-dimethyl-(3-aminoethyl) imidazolium tetrafluoroborate: a kinetic and theoretical study, J. Mol. Model., 2016, 68, 87–94 CrossRef CAS PubMed
. - Q. Tian, S. Liu, X. Sun, H. Sun, Z. Xue and T. Mu, Theoretical studies on the dissolution of chitosan in acetate-based ionic liquids, Carbohydr. Res., 2015, 408, 107–113 CrossRef CAS PubMed
. - Q. Tian, R. Li, H. Sun, Z. Xue and T. Mu, Theoretical and experimental study on the interaction between 1-butyl-3-methylimidazolium acetate and CO2, J. Mol. Liq., 2015, 208, 259–268 CrossRef CAS
. - J. Jiang, W. Zhao, Z. Xue, Q. Li, C. Yan and T. Mu, PEGylated quasi-ionic liquid electrolytes: fundamental physiochemical properties and electrodeposition of aluminium, ACS Sustainable Chem. Eng., 2016, 4(10), 5814–5819 CrossRef CAS
. - S. Liu, Y. Chen, Y. Shi, H. Sun, Z. Zhou and T. Mu, Investigations on the thermal stability and decomposition mechanism of an amine–functionalized ionic liquid by TGA, NMR, TG-MS experiments and DFT calculations, J. Mol. Liq., 2015, 206, 95–102 CrossRef CAS
. - B. Cao, J. Du, D. Du, H. Sun, X. Zhu and H. Fu, Cellobiose as a model system to reveal cellulose dissolution mechanism in acetate-based ionic liquids: density functional theorystudy substantiated by NMR spectra, Carbohydr. Polym., 2016, 149, 348–356 CrossRef CAS PubMed
. - Z. Xue, X. Sun, Z. Li and T. Mu, CO2 as a regulator for controllable preparation of highly dispersed chitosan-supportedPd
catalysts in ionic liquid, Chem. Commun., 2015, 51, 10811–10814 RSC
. - X. Zhou, B. Cao, S. Liu, X. Sun, X. Zhu and H. Fu, Theoretical and experimental investigation on the capture of H2S in a series of ionic liquids, J. Mol. Graphics Modell., 2016, 68, 87–94 CrossRef CAS PubMed
. - Z. Zhao, Z. Li, G. Wang, W. Qiao and L. Cheng, Friedel–Crafts alkylation of 2-methylnaphthalene in room temperature ionic liquids, Appl. Catal., A, 2004, 262, 69–73 CrossRef CAS
. - M. Chen, D. Li, Y. Luo, M. He, J. Xie, H. Li and X. Yuan, Synthesis of 5-benzoylacenaphthene in the presence of Lewis acidic ionic liquids, J. Ind. Eng. Chem., 2011, 17, 14–17 CrossRef CAS
. - S. F. Boys and F. Bernardi, The calculation of small molecular interactions by the differences of separate total energies. Some procedures with reduced errors, Mol. Phys., 2002, 19(1), 553–566 Search PubMed
. - M. J. Frisch, G. W. Trucks, H. B. Schlegel, G. E. Scuseria, M. A. Robb, J. R. Cheeseman, G. Scalmani, V. Barone, B. Mennucci and G. A. Petersson, et al., Gaussian 09, Revision D.01, Gaussian Inc., Wallingford CT, 2009 Search PubMed
. - C. L. Ricardo, X. Mo, J. A. McCubbin and D. G. Hall, Surprising substituent effect provides a superior boronic acid catalyst for mild and metal-free direct Friedel–Crafts alkylations and prenylations of neutral arenes, Chem.–Eur. J., 2015, 21, 4218–4223 CrossRef CAS PubMed
.
Footnote |
† Electronic supplementary information (ESI) available. See DOI: 10.1039/c6ra28009a |
|
This journal is © The Royal Society of Chemistry 2017 |