DOI:
10.1039/C6RA27682B
(Paper)
RSC Adv., 2017,
7, 6685-6690
Superhydrophobic polysilsesquioxane/polystyrene microspheres with controllable morphology: from raspberry-like to flower-like structure†
Received
2nd December 2016
, Accepted 11th January 2017
First published on 20th January 2017
Abstract
Superhydrophobic polysilsesquioxane/polystyrene microspheres with raspberry-like to flower-like structure were fabricated via a cetyltrimethyl ammonium bromide (CTAB)-assisted sol–gel approach. The structure of nanoparticles on microcores could be controlled precisely by simply adjusting the content of the silane precursor: with increasing amount of silane precursor, the microspheres transformed from raspberry- to flower-like morphology. Besides the silane precursor, ammonia solution, CTAB and polyvinylpyrrolidone (PVP) also play essential roles in the formation of uniform and monodisperse hierarchical particles. The morphology, microstructure, and constitution of the microspheres were characterized by field-emission scanning electron microscopy (FE-SEM), transmission electron microscopy (TEM), Fourier transform infrared (FT-IR) spectroscopy, solid state 29Si magic angle spinning nuclear magnetic resonance (NMR) spectroscopy and X-ray diffraction (XRD). The formation mechanism of the hierarchical structure was also investigated in detail by time-dependent experiments. In addition, the particulate films, without any surface modification, showed superhydrophobic properties after simply assembling these hierarchical particles on glass substrates. The hierarchical morphology combined with the initial ethyl groups on the polysilsesquioxane materials were thought to be the main reason leading to this superhydrophobicity.
1 Introduction
Considerable attention has been attracted to the design and fabrication of materials with hierarchical structure, which could enable materials with enhanced physical and chemical properties, such as mechanical, thermal, optical, adsorption and catalytic properties.1–3 Hierarchical structures are defined as the structures with a morphology on different length scales. Varied hierarchical structured materials including honeycomb-like materials,4 mesoporous hollow spheres,5 snow-man like particles,6 raspberry-like materials,7 etc. have been developed. Among them, raspberry-like composite colloids have been fabricated for enhanced Raman scattering, separation, heterogeneous catalysts, drug delivery and fabrication of superhydrophobic materials.8–14 Raspberry-like particles possess a well-defined hierarchical structure, which consists of a microsized core decorated with nanoscaled particles on the surface. Due to the morphology with hierarchical micro-/nanostructures, the films obtained from these particles, after surface modification, display an extraordinary superhydrophobicity (defined with a water contact angle larger than 150°).15,16
Recently, various approaches including emulsion polymerization, layer-by-layer, templating and self-assembly method have been developed to construct these raspberry-like hierarchical structure with dual-sized surface roughness from the organic materials, inorganic materials and organic–inorganic hybrid materials.17–24 In a polymerization process, Chen et al. prepared a series of raspberry-like polymer/silica hybrid microspheres using a cationic monomer and nanosilica particles via electrostatic interaction.18 Through a sol–gel process, Ahmed et al. also prepared a raspberry-like silica particles using 3-mercaptopropyltrimethoxysilane as the sole precursor, which after calcination shows a high performance in separation field.25 However, the limitation is that the separation of the extra nanoparticles from the system is a complicated process and also the broad particle size distribution of the final materials from these methods limited their further applications.6,26
To precisely control the particle morphology and particle size distribution, templating or self-assembling method is commonly used to fabricate the raspberry-like spheres.2,27 Qian et al. prepared raspberry-like particles with hydrophilic polystyrene as template and tetraethyoxysilane (TEOS) as silica precursor.10 In a classical method, Ming et al. prepared amine- and epoxy-group modified silicas with different particle sizes and then simply assembled them into raspberry-like particles.28 Uniform-sized and monodisperse hierarchical spheres could be obtained by these methods. However, for applications in superhydrophobic coatings, surface modification with some low-surface-energy materials usually is a necessary step for the films prepared by these methods. For example, Puretskiy etc. grafted 2,3,4,5,6-pentafluorostyrene onto raspberry-like particles to make the superhydrophobic layers.29
Here, we demonstrate a facile synthetic route to fabricate hierarchical microspheres with controlled structure by hydrolysis–condensation process of silane precursor. Without surface modification, the film obtained from these particles displayed an ultrahydrophobic property due to the hierarchical micro-/nanostructures and hydrophobic organic groups initially on the materials surface.
Polysilsesquioxane (PSQ), one kind of molecular-level organic/inorganic hybrid materials, combined the mechanical merits of inorganic materials with functionalites of organic groups on the surface.30 Recently, we have developed a simple and environment-friendly approach to prepare organofunctionalized-PSQ hollow spheres for versatile applications.31–33
In this work, hierarchical polysilsesquioxane/polystyrene (HPSQ/PS) spheres with raspberry- to flower-like structures were generated by growing of PSQ particles on polymer latex cores. This procedure has a few advantages: firstly, the size of the nanoprotrusions can be precisely and simply controlled by adjusting reactant concentration; secondly, hierarchical microspheres are easily to be separated from the solvent system; thirdly, no complicated surface modification to the final product is needed for superhydrophobic property.
2 Experimental section
2.1 Fabrication of hierarchical PSQ/PS microspheres
Materials. Ethyltrimethoxysilane (ETMS, > 97%), cetyltrimethyl ammonium bromide (CTAB, ≥ 98%), polyvinylpyrrolidone (PVP, average mol wt 40
000) and styrene (St, ≥ 99%) were purchased from Sigma-Aldrich. 2,2′-Azobis(2-methylpropionitrile) (AIBN, ≥ 98%) and ammonia solution (28 wt%) were supplied by Junsei Chemical. All chemicals were used as received without any further purification. Distilled water (≈17 MΩ cm−1) from a Milli-Q water system was used throughout the experiment.
Preparation of hierarchical microspheres. Firstly, monodisperse polystyrene latexes with size of 1.1 μm were synthesized by dispersion polymerization according to reference with minor modification.34 In the first step, 20.0 g of St, 95 mL of ethanol, 3 mL of water, 1.5 g of PVP and 0.2 g of AIBN were charged into a 250 mL three-neck flask equipped with a mechanical stirrer in water bath. The solution was stirred and deoxygenated by bubbling N2 at room temperature for 30 min. Then, the mixture was slowly heated to 70 °C and kept reaction for 24 h. The PS particles were isolated by centrifugation at 8000 rpm and washing with ethanol, followed by freeze-drying process.For hierarchical microspheres, 0.1 g of CTAB, 0.25 g of PVP and 100 mg of PS particles were added into the mixture of water (6.6 mL) and methanol (8 mL). After sonication for 10 min, a milk-like homogenous suspension was obtained. To this mixture, 0.4 mL of ammonia solution was added, followed by adding 5 mmol of ethyltrimethoxysilane dropwise via syringe in one minute. The reaction was maintained for 12 hours with stirring at room temperature. The hierarchical microspheres (HPSQ/PS) were obtained by centrifugation at 8000 rpm for 5 min, washing with methanol for 3 times, followed by freeze-drying.
2.2 Characterization and apparatus
The morphology of the samples were observed by scanning electron microscopy (SEM) using a Hitachi S4800 FE-SEM system. Particle size distributions were obtained using dynamic light scattering (DLS) method with electrophonic light scattering spectrophotometer (ELS-8000, OTSUKA Electronics, Japan). Transmission electron microscopy (TEM) images were taken using a JEM-2011 electron microscope operating at 200 kV. Fourier transform infrared (FT-IR) spectra of KBr powder-pressed pellets were recorded on a Perkin-Elmer Spectrum GX-Spectrophotometer with a spectral resolution of 1 cm−1. 29Si magic angle spinning nuclear magnetic resonance (NMR) spectra were performed on a Bruker DSX400 spectrometer at frequencies of 400 MHz at room temperature. X-ray diffraction (XRD) experiments were performed on a Scintag XDS 2000 diffractometer by using a Cu-Kα radiation source, with a scanning speed of 1° min−1. The d-spacing were calculated according to the Bragg's equation. TGA analysis was performed using TG instrument Q500 from room temperature to 800 °C at a heating rate of 10 °C min−1 under N2 gas. Water contact angle measurements were performed on a Kruss DSA100 machine.
3 Results and discussion
3.1 Characterization of hierarchical structure
Well-defined hierarchical particles were prepared with nano-sized PSQ protrusions grown on micro-sized polystyrene latexes. Firstly, microspheres with uniform morphology were prepared by a dispersion polymerization procedure. Then, silane precursor was hydrolyzed and condensed on the polystyrene surface in present of surfactant (CTAB) and stabilizer (PVP).
As-prepared PS latex beads, as shown in SEM image of Fig. 1a, have a uniform diameter of approximate 1.1 μm, with a very narrow size distribution (Fig. 1b). Then, ethyl-functionalized polysilsesquioxane materials were formed on the PS microsphere surfaces with ammonia as catalyst. SEM images in Fig. 2 display the representative scanning electron micrographs of the as-prepared composite particles at different magnifications. Hierarchical morphology could be clearly observed from the magnified image of a single microsphere (Fig. 2a), whose surface is covered with a great number of nanoscale tubercles or protrusions. SEM image at low magnification (Fig. 2c) describes that the products are composed of homogeneous microspherical particles (∼1.4 μm) with a narrow size distribution as measured by DLS technique (Fig. 2d). Detailed structure revealed by TEM image (Fig. 2b) also clearly demonstrates the micro–nano hierarchical morphology with very uniform size and monodisperse property of the final products.
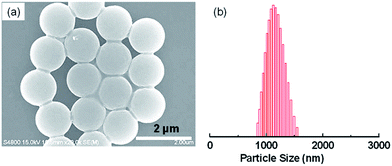 |
| Fig. 1 (a) SEM image of polystyrene latex beads with (b) the corresponding particle size distribution measured by DLS technique. | |
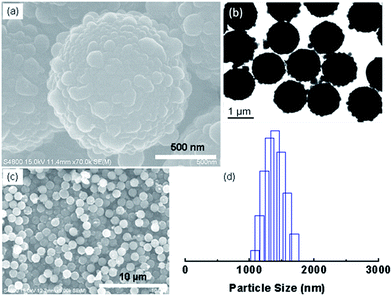 |
| Fig. 2 Typical SEM (a, c) and TEM (b) images of the hierarchical polysilsesquioxane/polystyrene microspheres (5 mmol of ETMS) with the corresponding size distribution (d) measured by DLS technique. | |
In FT-IR spectrum of HPSQ/PS (Fig. 3a), a sharp band at 1257.4 cm−1 for –CH2CH3 group stretch and two bands at 1123, 1006 cm−1 for Si–O–Si group stretch are found for the samples, which confirmed the existence of ethyl-functionalized PSQ materials. The microstructure the polysilsesquioxanes was investigated by solid-state 29Si NMR and X-ray diffraction techniques. Information about the condensation reaction can be obtained from the solid-state 29Si NMR spectrum (Fig. 3b), in which the characteristic peaks of monosubstituted silica (T) species were clearly visible. Intense and less intense peaks are observed at −66.0 ppm and −57.3 ppm that could be assigned to the T3 [RSi(OSi)3] and T2 [RSi(OSi)2OH] species, respectively. Fig. 3c shows the XRD pattern, in which two halos appeared at 9.6° and 23.1°, which attributed to the intermolecular and intramolecular spacing of the silsesquioxane components, respectively. TGA data (Fig. 3d) demonstrated the formation of PSQ materials on PS template and the PSQ content increased with the increasing of silane precursor content.
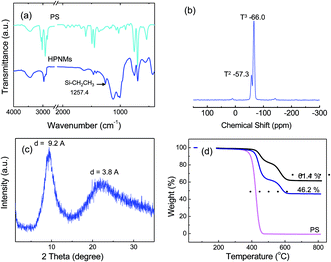 |
| Fig. 3 Characterizations of hierarchical polysilsesquioxane/polystyrene microspheres. (a) FTIR spectra of HPSQs and PS (b) 29Si NMR spectrum and (c) XRD pattern of HPSQ/PS microspheres (d) TGA data of HPSQs (upper and middle lines are for samples from 10 and 5 mmol of silane precursor, respectively) and TGA data of polystyrene. | |
3.2 Effect of silane precursor
Morphology controllable synthesis of the hierarchical structured spheres is one of the advantages of our fabrication process. By simply adjusting the silane precursor concentration, the morphology of the as-prepared samples could be transferred from raspberry-like to a flower-like structure. Different amounts of ethyltrimethoxysilane (1, 3, 5, 7, 10 mmol) were used with the other reactant kept constant (CTAB 0.1 g, PVP 0.25 g, NH3·H2O 0.4 mL). Fig. 4 shows the SEM images of the as-prepared HPSQ/PS materials at varied silane precursor amount. As seen from the enlarged images, all products show hierarchical morphology with microspheres coated by nanoprotrusions, whose size were clearly found increasing when precursor amount increased from 1 to 10 mmol, which also were confirmed by DLS technique (Fig. S1†). When 1 mmol of the silane precursors were used, ∼80 nm nanoparticles appeared on the microspheres (Fig. 4a and b). When the precursor amount increased to 3 and 5 mmol, the size of PSQ nanoparticles increased to 120, 140 nm, respectively, as shown in the SEM images (Fig. 4c and d and 2a). With further increasing of precursor amount to 7 mmol, the nanoparticles size increased to ∼220 nm and at the same time, the number of PSQ nanoparticles decreased. It seemed that the big PSQ nanoparticles were grown from some small nanoparticles. Finally, when the precursor amount increased to 10 mmol, the flower-like morphology appeared, since the nanoparticles have grown to be very big protrusions on the surface. Hence, the silane precursor concentration is an important factor to influence the hierarchical morphology of the PSQ/PS materials.
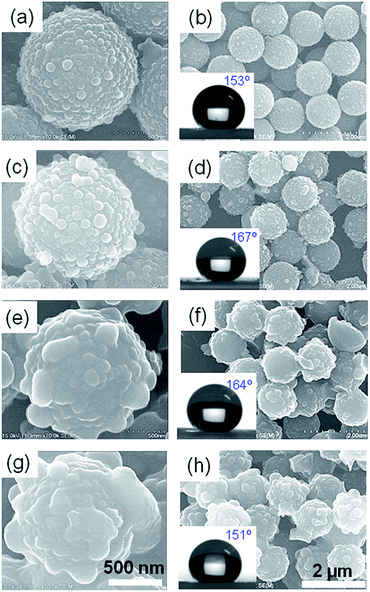 |
| Fig. 4 Effect of silane precursor on the hierarchical structure of HPSQ/PS materials. SEM images of HPSQs with different amount of ethyltrimethoxysilane: (a, b) 1 mmol, (c, d) 3 mmol, (e, f) 7 mmol, (g, h) 10 mmol. Insets are water droplet images on the corresponding surfaces. Scale bar of 500 nm is for (a, c, e, g), and scale bar of 2 μm is for (b, d, f, h). | |
When the hierarchical particles were deposited onto a glass substrate from ethanol suspension, a film with dual-size roughness could be obtained. This hierarchical surface is ideal building blocks for a superhydrophobic surface, because the rough surface endows the surface with a smaller contact area with water, as compared to smooth spheres, and results in a higher water contact angle.35
Also, as discussed in FTIR part, the surface of the raspberry-like spheres is covered by ethyl-functionalized polysilsesquioxane materials. It is widely accepted that the surface roughness combined with the initially hydrophobic surface chemistry makes it possible for the construction of superhydrophobic coatings.36 When these superhydrophobic coatings contact with water, air will be maintained at the solid–liquid interface, which will result in a high apparent water contact angles (by definition exceeding 150°). As expected, without any surface modifications, all as-prepared particulate films exhibit superhydrophobic property with water contact angle of 153°, 167°, 165°, 164°, 151° when the precursor amount are 1, 3, 5, 7, 10 mmol respectively. We believe that these superhydrophobic surfaces contain a unique combination of two fundamental properties: (1) surface roughness with raspberry to flower like morphology and (2) low surface energy from PSQ.
3.3 Effect of ammonia solution, CTAB and PVP
Another important synthetic factor that influences the surface topography is the concentration of ammonia solution, which worked as a catalyst to control the hydrolysis and condensation speed of the silane precursor. To investigate the effect of the catalyst on the hierarchical morphology, the amount of NH3·H2O was varied from 0.01 to 1 mL, with the other conditions kept constant (5 mmol of silane precursor, 0.1 g of CTAB and 0.25 g of PVP). The morphologies of the final products with different ammonia concentration were checked by SEM as shown in Fig. S2.† With small ammonia amount (0.01, 0.02 mL), the hydrolysis and condensation speed of silane precursor is very slow, which made the HPSQ/PS materials aggregated and generated connected microspheres. Increasing ammonia amount to 0.1 mL led to microspheres with much more uniform morphology, however, still some connected microspheres was observed. When ammonia amount increased to 0.4 mL, uniform and monodispersed spheres could be obtained as shown in Fig. 2. Further increasing ammonia concentration did not show obvious change on the sphere morphology. In summary, enough high concentration of ammonia solution is necessary for the formation of uniform and monodispersed microspheres with hierarchical structure.
CTAB and PVP also play very important roles in the formation of the hierarchical structure. Without using CTAB, only smooth spheres could be obtained as shown in SEM images of Fig. S3a and b.† Without using PVP, the hierarchical structure still could be observed (Fig. S3c and d†), and increase of CTAB amount (from 0.1 g to 0.25 g) could result in more clearly hierarchical structure, even though the morphology is not uniform. It is considered from these phenomena that CTAB plays a crucial role in the formation of hierarchical composite particles and PVP works as the stabilizer to ensure the uniform and monodispersed microspheres.
The polymer microspheres also play a significant role as the original seeds to induce the adsorption of hydrolyzed ETMS on them. When a control experiment without using any PS template was carried out, it was found that only macroscopic precipitate appeared and no regular sphere was formed (Fig. S4†).
3.4 Mechanism of hierarchical PSQ/PS forming process
To investigate the formation process of the hierarchical structure, evolution experiments were performed and the intermediate products at different stages (0.5 to 120 min) were studied by SEM techniques (Fig. 5). With reaction time for 0.5 min, no obvious nanoparticles were found on the surface of the microspheres (Fig. 5a). The particles are spherical, uniform in size and have a very smooth surface. When the reaction time increased to 20 min, several nanoscale protrusions are clearly observed on the smooth microspheres (Fig. 5b). Further prolonging the reaction time to 40 min, much more tiny nanoparticles appeared and adhered on the microspheres (Fig. 5c). For the reaction time of 60 min, much higher coverage of PSQ nanoparticles on the microsphere was obtained and the surface of microspheres became rough, as shown in Fig. 5d. Interestingly, the nanoscale protrusion could further grow up to a bigger size of nanoparticles with reaction time of 120 min, and the areas between the nanoparticles also were clearly covered by the PSQ materials, which means a well-formed hierarchical structure have been formed (Fig. 5e). Further increasing of the reaction time to 12 h did not affect the morphology much (Fig. 5f). Based on the time-dependent experiments above, it is clearly that several nanoparticles were firstly formed and attached on the microsphere, subsequently formed raspberry-like particles with further condensation process of the silane precursor (Scheme 1).
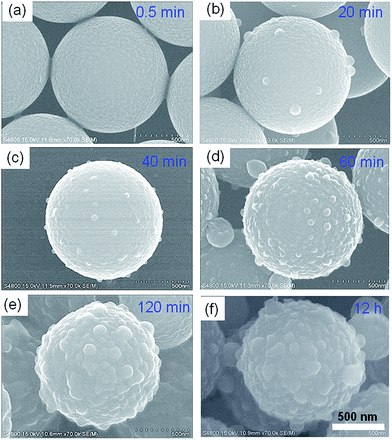 |
| Fig. 5 Formation process of the hierarchical microspheres. SEM images of the HPSQ/PS collected at different reaction time: (a) 0.5 min, (b) 20 min, (c) 40 min, (d) 60 min, (e) 120 min, (f) 12 h. | |
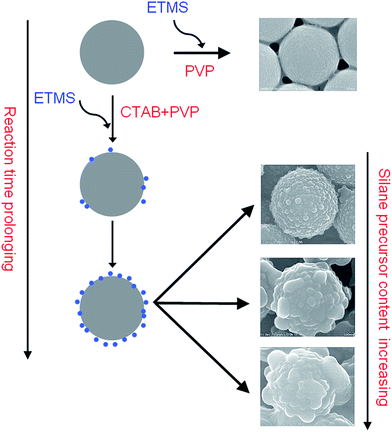 |
| Scheme 1 Schematic procedure for preparing hierarchical HPSQ/PS microspheres: with reaction time prolonging, PSQ nanoparticles formed, attached on microspheres and grown into raspberry- to flower-like spheres depending on the amount of silane precursor. Without using CTAB, just smooth microspheres were obtained. | |
4 Conclusions
In this work, a series of raspberry-like to flower-like particles were controllably fabricated through a PSQ materials formation, adhesion and growing on polystyrene cores. The diameter of nanoscale particles and the topography of the microspheres surface could be easily tailored by adjusting the silane precursor concentration. Other reactants such as ammonia solution, CTAB, and PVP in the system also play an essential roles for the formation of the raspberry-like structure. The hierarchical structure of the composite particles combined with the hydrophobic PSQ surface enables the as-prepared particulate film exhibit superhydrophobic property.
Acknowledgements
We thank the Education Department of Guizhou Province for the Youth Science and Technology Talent Growth Project. The work was also supported by the National Research Foundation of Korea (NRF) Grant funded by the Ministry of Science, ICT, and Future Planning, Korea {Acceleration Research Program (NRF-2014R1A2A1110054584); Brain Korea 21 Plus Program (21A2013800002)}.
References
- R. Lakes, Nature, 1993, 361, 511–515 CrossRef.
- W. Wei and Z. Z. Yang, Adv. Mater., 2008, 20, 2965–2969 CrossRef CAS.
- M. Agrawal, S. Gupta and M. Stamm, J. Mater. Chem., 2011, 21, 615–627 RSC.
- U. H. F. Bunz, Adv. Mater., 2006, 18, 973–989 CrossRef CAS.
- X. Du and J. H. He, Nanoscale, 2011, 3, 3984–4002 RSC.
- B. Zhao and M. M. Collinson, Chem. Mater., 2010, 22, 4312–4319 CrossRef CAS.
- M. Yu, H. Wang, X. Zhou, P. Yuan and C. Yu, J. Am. Chem. Soc., 2007, 129, 14576–14577 CrossRef CAS PubMed.
- D. Z. Xu, M. Z. Wang, X. W. Ge, M. H. W. Lam and X. P. Ge, J. Mater. Chem., 2012, 22, 5784–5791 RSC.
- M. D'Acunzi, L. Mammen, M. Singh, X. Deng, M. Roth, G. K. Auernhammer, H. J. Butt and D. Vollmer, Faraday Discuss., 2010, 146, 35–48 RSC.
- Z. Qian, Z. Zhang, L. Song and H. Liu, J. Mater. Chem., 2009, 19, 1297–1304 RSC.
- F. Caruso, Adv. Mater., 2001, 13, 11–22 CrossRef CAS.
- H. Wang and N. J. Halas, Adv. Mater., 2008, 20, 820–825 CrossRef CAS.
- U. Hasegawa, S. Sawada, T. Shimizu, T. Kishida, E. Otsuji, O. Mazda and K. Akiyoshi, J. Controlled Release, 2009, 140, 312–317 CrossRef CAS PubMed.
- B. N. Sahoo and B. Kandasubramanian, RSC Adv., 2014, 4, 22053–22093 RSC.
- Y. L. Zhang, H. Xia, E. Kim and H. B. Sun, Soft Matter, 2012, 8, 11217–11231 RSC.
- C.-H. Xue and J.-Z. Ma, J. Mater. Chem. A, 2013, 1, 4146–4161 CAS.
- H. Cheng, L. Zhang, J. He, W. Guo, Z. Zhou, X. Zhang, S. Nie and H. Wei, Anal. Chem., 2016, 88, 5489–5497 CrossRef CAS PubMed.
- M. Chen, S. Zhou, B. You and L. Wu, Macromolecules, 2005, 38, 6411–6417 CrossRef CAS.
- W. S. Choi, H. Y. Koo and W. T. S. Huck, J. Mater. Chem., 2007, 17, 4943–4946 RSC.
- K. Kulbaba, A. Cheng, A. Bartole, S. Greenberg, R. Resendes, N. Coombs, A. Safa-Sefat, J. E. Greedan, H. D. H. Stoever, G. A. Ozin and I. Manners, J. Am. Chem. Soc., 2002, 124, 12522–12534 CrossRef CAS PubMed.
- Y. Zhang, H. Chen, X. Shu, Q. Zou and M. Chen, Colloids Surf., A, 2009, 350, 26–32 CrossRef CAS.
- D. Nagao, T. Ueno, D. Oda and M. Konno, Colloid Polym. Sci., 2009, 287, 1051–1056 CAS.
- A. Perro, S. Reculusa, E. Bourgeat-Lami, E. Duguet and S. Ravaine, Colloids Surf., A, 2006, 284, 78–83 CrossRef.
- R. Guo, X. Chen, X. Zhu, A. Dong and J. Zhang, RSC Adv., 2016, 6, 40991–41001 RSC.
- A. Ahmed, H. Ritchie, P. Myers and H. F. Zhang, Adv. Mater., 2012, 24, 6042–6048 CrossRef CAS PubMed.
- X. Y. Li and J. H. He, ACS Appl. Mater. Interfaces, 2012, 4, 2204–2211 CAS.
- H.-J. Tsai and Y.-L. Lee, Langmuir, 2007, 23, 12687–12692 CrossRef CAS PubMed.
- W. Ming, D. Wu, R. van Benthem and G. de With, Nano Lett., 2005, 5, 2298–2301 CrossRef CAS PubMed.
- N. Puretskiy and L. Ionov, Langmuir, 2011, 27, 3006–3011 CrossRef CAS PubMed.
- R. M. Laine and M. F. Roll, Macromolecules, 2011, 44, 1073–1109 CrossRef CAS.
- F. P. Dong, W. P. Guo, S. W. Chu and C. S. Ha, Chem. Commun., 2010, 46, 7498–7500 RSC.
- F. Dong, W. Guo, S. S. Park and C. S. Ha, J. Mater. Chem., 2011, 21, 10744–10749 RSC.
- F. Dong, W. Guo, S. K. Park and C. S. Ha, Chem. Commun., 2012, 48, 1108–1110 RSC.
- S. H. Kim, A. D. Hollingsworth, S. Sacanna, S. J. Chang, G. Lee, D. J. Pine and G. R. Yi, J. Am. Chem. Soc., 2012, 134, 16115–16118 CrossRef CAS PubMed.
- N. Nuraje, W. S. Khan, Y. Lei, M. Ceylan and R. Asmatulu, J. Mater. Chem. A, 2013, 1, 1929–1946 CAS.
- W. Li and A. Amirfazli, Soft Matter, 2008, 4, 462–466 RSC.
Footnote |
† Electronic supplementary information (ESI) available: SEM images and particle size distribution by DLS. See DOI: 10.1039/c6ra27682b |
|
This journal is © The Royal Society of Chemistry 2017 |
Click here to see how this site uses Cookies. View our privacy policy here.