DOI:
10.1039/C6RA27182K
(Paper)
RSC Adv., 2017,
7, 27121-27127
Enhancing the osteogenic capacity of MG63 cells through N-isopropylacrylamide-modified polyethylenimine-mediated oligodeoxynucleotide MT01 delivery
Received
23rd November 2016
, Accepted 9th April 2017
First published on 23rd May 2017
Abstract
An oligodeoxynucleotide (ODN), with its design based on human mitochondrial DNA (MT01), has been demonstrated to promote the proliferation and activation of osteoblasts. However, critical issues such as poor oligonucleotide stability in the presence of nucleases currently limit its broad application. This study evaluated whether N-isopropylacrylamide-modified polyethylenimine (PEN) nanoparticles could effectively deliver ODN MT01 locally with low or no toxicity and promote osteoblast differentiation in vitro. Our data demonstrate that at a w/w ratio of 6
:
1, PEN/MT01 nanoparticles were effectively transfected into MG63 cells without cytotoxicity, whereby they promoted cell proliferation in vitro. Quantitative PCR and western blotting analyses showed that the PEN/MT01 complexes enhanced mRNA and protein expression of osteoprotegerin, Sp7, and runt-related transcription factor 2, and decreased levels of receptor activator of nuclear factor kappa-B ligand, which are markers indicative of MG63 differentiation into preosteoblasts or mature osteoblasts. This approach demonstrates the potential of using PEN as a biocompatible gene therapy carrier to address regeneration of bone defects.
1. Introduction
Alveolar bone loss caused by periodontal disease can significantly affect patient quality of life: even to the extent of forming an edentulous jaw. The ultimate goal of periodontal therapy is to achieve complete regeneration of dental structures, especially the alveolar bone.1,2 With developments in alveolar surgery, molecular biology, and tissue engineering, several new strategies are routinely utilized in clinical practice, including distraction osteogenesis, guided bone regeneration membranes, and the application of growth factors. However, the outcomes of these treatments are limited by poor clinical predictability.3
In our previous studies, an oligodeoxynucleotide (ODN) that was designed based on human mitochondrial DNA (MT01), was demonstrated to promote the proliferation and activation of osteoblasts and stimulate the proliferation and differentiation of bone marrow mesenchymal stem cells (BMSCs) into osteoblasts.4–7 However, crucial problems such as poor oligonucleotide stability in the presence of nuclease activity in vitro and in vivo, and low intracellular penetration, would limit its wide application to enhance osteogenic capacity.8 To overcome these problems, chemical modifications of ODNs have been employed, mainly focusing on the phosphodiester backbone and/or the sugar moiety. For example replacement of the non-bridging oxygen of the phosphodiester backbone with sulfur results in a phosphorothioate with enhanced stability against enzymatic degradation. Although chemical modifications may improve ODN stability and cell penetration, they may also produce a variety of side effects. For example, repeated administration of backbone-modified ODNs has been shown to lead to reduced immune responses, lymphoid follicle destruction, and organ enlargement.9,10
To date, encapsulating ODNs in the form of nanoparticles (NPs) is an effective method to mediate their in vivo delivery: likely by increasing ODN stability, improving cell penetration, and avoiding non-specific aptameric effects.11,12
Poly(ethyleneimine) (PEI) is a well-known cationic polymer that is reported to result in high gene transfection efficiency both in vitro and in vivo.13–15 Compared with viral vectors however, PEI has a number of disadvantages, such as a relatively low efficiency in gene transfection, high cell toxicity, and as a non-degradable polymer.16,17 To achieve clinical applicability of PEI, it is therefore essential to improve its transfection efficiency and/or reduce its cytotoxicity.18 Tian et al. constructed a PEI derivative through modification of branched PEI by N-isopropylacrylamide using the Michael addition, named N-isopropylacrylamide-modified polyethylenimine (PEN).19 PEN exhibited improved transfection efficiency and decreased cytotoxicity. This approach did not reduce the content of protonated amines or influence the formation of stable nanocomplexes between the carrier and pDNA through electrostatic interaction. Furthermore, Zhang et al. demonstrated that PEN successfully mediated p53 gene delivery and up-regulated cellular p53 expression levels.20 These findings suggest that PEN could be used as a potential carrier to enable effective gene delivery for the treatment of many diseases.
In the present study, PEN was employed as a carrier for MT01 delivery, and was assessed using the MG63 osteoblast-like cell line as a model. The ability to promote MG63 proliferation and activation was assessed by the MTT assay and expression levels of alkaline phosphatase (ALP) were determined. Real-time PCR (qPCR) and western blotting analyses were used to determine the effects of PEN/MT01 on the expression levels of Sp7, Runx-2, collagen-I, OPG, and RANKL, to obtain deeper insight into the molecular mechanism of MT01-mediated proliferation and activation of osteoblasts.
2. Experimental section
2.1 Materials
Human osteoblast-like cell line MG 63 was obtained from American Type Culture Collection (ATCC, CRL-1427). MT01 and MT01s (MT01 modifed by sulfur) were synthesized by TaKaRa (Dalian, China). The sequence of MT01 is as follows: 5′-ACC CCC TCT ACC CCC TCT ACC CCC TCT-3′. Branched PEI-25K was purchased from Aldrich and used as received. 3-(4,5-Dimethylthiazol-2-yl)-2,5-diphenyltetrazolium bromide (MTT), the alkaline phosphatase (ALP) kit and the kit for a micro-BCA assay were obtained from Jiancheng Biological Reagent Co. (Nanjing, China). A real time PCR kit was purchased from TaKaRa (Tokyo, Japan). The monoclonal antibodies specific for β-actin, Sp7 and OPG were purchased from Santa Cruz Biotech. Co., and those specific for Runx-2 and RANKL were sourced from Abcam Co. (USA). Dulbecco's Modified Eagle's Medium (DMEM) and fetal bovine serum (FBS) were purchased from Gibco (Grand Island, USA). All other chemicals were of the highest reagent grade commercially available and used as received.
2.2 Preparation of PEN and PEN/MT01 complexes
PEN derivative was synthesized according to a previously published method.19 Briefly, 1 g of PEI-25K was dissolved in 5 mL of chloroform, followed by stirred addition of the desired amount of N-isopropylacrylamide based on the required molar ratios. The solution was incubated at 40 °C for 96 h. Upon completion of the reaction, chloroform was removed by rotary evaporation. The crude product was dissolved in 5 mL of distilled water, dialyzed against water, filtrated, and lyophilized. The PEN solution (adjusted to pH 7.0) and the MT01 solutions were freshly diluted in phosphate buffered saline (PBS) (pH 7.4) to obtain the desired concentrations. Complexes were prepared by gently mixing the carrier PEN and MT01 together at different mass ratios (0.25, 0.5, 1.0, 2.0, 4.0, 6.0, 8.0 w/w). Complexes were subsequently incubated at room temperature for 30 min. The final concentration of MT01 was 0.5 mg mL−1.
2.3 Gel retardation assay
The PEN/MT01 nanocomplexes were prepared by gently mixing the carrier PEN and MT01 together at different mass ratios (0.25, 0.5, 1.0, 2.0, 4.0, 6.0, 8.0, w/w) and incubated at room temperature for 30 min before use. The binding ability of PEN with MT01 was then assessed by 2% agarose gel electrophoresis in a tris-acetate-EDTA (TAE) buffer solution (120 V, 22 min).
2.4 Size distribution and zeta potential
The mean particle sizes and zeta potentials of the PEN/MT01 complexes were determined by a Malvern Nano ZS90 Zetasizer (Malvern, UK). Particle size was assessed from three cycles, and zeta potential was performed by three repeated cycles with 100 runs each. The morphologies of PEN/MT01 were measured by transmission electron microscopy (TEM) using FEI Tecnai G2 S-TWIN F20.
2.5 Cell viability assay
An evaluation of cell viability at 48 hours post-transfection of PEN/MT01 complexes (PEN/MT01 at w/w ratios of 2.0, 4.0, 6.0, 8.0) was performed with MTT. The MG63 cells were seeded onto 96-well plates at a density of 5.0 × 103 cells per well, pre-incubated in fresh serum-free DMEM medium for 24 h, and further incubated with different mass ratios of PEN/MT01 nanocomplexes at an MT01 concentration of 0.5 μg mL−1. After 48 h, 100 μL of MTT solution (1 mg mL−1 in PBS) was added to each well, and the plate was incubated for an additional 4 h. The MTT solution was then removed from each well, and 150 μL DMSO was added to dissolve the formazan crystals. The plate was incubated for an additional 10 min, and the absorbance at 492 nm was recorded using a GF-M3000 microplate reader (Shandong, China).
2.6 Transfection efficiency of PEN/MT01
The in vitro gene transfection efficacy of PEN was evaluated in MG63 cells. Briefly, MG63 cells were seeded into six-well plates at 3 × 105 cells per well and then cultured for 24 h prior to transfection. The medium was then removed from each well and the cells were washed once with 1 mL per well of PBS. The cells were then incubated in serum-free medium with PEN/MT01 polyplexes at different mass ratios for 4 h. The transfected cells were then washed once with PBS and analyzed using an Olympus IX71 fluorescence microscope (Osaka, Japan).
2.7 Cell cycle analysis after PEN/MT01 transfection
For cell cycle analysis, MG63 cells were seeded in a six-well plate at a density of 3 × 105 cells per well and incubated for 24 h before transfection. The transfection was performed using PEN/MT01 nanocomplexes (6
:
1 w/w, 1 μg MT01) in serum-free DMEM for 4 h. After incubating in DMEM containing 10% FBS for 48 h, the cells were collected, washed twice with PBS, suspended in 0.5 mL solution containing 10 μL RNase A (25 μg mL−1) and 10 μL PI (50 μg mL−1), and incubated at 37 °C for an additional 30 min in the dark. The cell cycle was monitored by analyzing 10
000 gated cells using a FACS caliber (BD Biosciences) and ModFITLT 2.0 (Verity Software House, Topsham, ME, USA).
2.8 Effect of PEN/MT01 on ALP activity
After incubation with MT01s, MT01, and PEN/MT01 nanocomplexes for 48 h and 72 h, cells were collected and lysed to determine ALP activity using an Alkaline Phosphatase Kit according to the manufacturer's instructions. Protein concentration of cell lysates was measured using a micro-BCA assay kit, and ALP activity was normalized to total protein concentration. Average values were obtained from triplicate measurements.
2.9 Real-time PCR assay
MG63 cells were seeded at a density of 3 × 105 cells per well in 6-well plates and incubated with PEN/MT01 nanocomplexes (6
:
1, w/w, 1 g MT01), MT01s or MT01 for 24, 48 and 72 h. The total RNA was extracted using RNAiso Plus as recommended by the manufacturer. For reverse transcription, first-strand cDNA was synthesizedfrom 1 g of total RNA, and the obtained cDNA was used as a template for real-time PCR amplifications as follows: 1 cycle at 94 °C for 2 min followed by 30 cycles at 94 °C for 30 s, 55 °C for 30 s and 72 °C for 1 min. The primers were as follows: GAPDH(F):5′-ATGGGGAAGGTGAAGGTC-3′/(R):5′-TTAGGATCCATCTGCGCTC-3′;OPG(F):5′-TTGCCCTGACCACTAC-3′/(R):5′-TTTGAGAAGAACCCATC-3′;RANKL(F):5′-GCCTCCCGCTCCATGTTC-3′/(R):5′-TTAGGATCCATCTGCGCT-3′;Runx-2(F):5′-GAGATCATCGCCGACCAC-3′/(R):5′-TACCTCTCCGAGGGCTACC-3′.
2.10 Westernblot analysis
The expression levels of proteins associated with osteoblast-related factors after PEN/MT01 transfection were evaluated by Western blotting analysis. MG63 cells were cultured in 10 cm double dishes and treated with PEN/MT01 nanocomplexes for a predetermined time. Then the cells were collected, washed twice with tris-buffered saline and centrifuged at 5000 × g for 5 min at 4 °C. Afterwards, the collected cells were suspended in lysis buffer (50 mM tris, pH 7.6, 0.01% EDTA, 1% Triton X-100, 1 mM PMSF, and 1 μg mL−1 leupeptin) on ice for 30 min, and centrifuged at 12
000 × g for 15 min at 4 °C. The protein concentration was measured using a BCA Protein Assay Reagent kit. The samples (40 μg protein) were separated by 12% SDS-PAGE and transferred to polyvinylidene difluoride (PVDF) membranes. The membranes were blocked with 5% skim milk in tris-buffered saline with 0.1% Tween (TBST) for 1 h at room temperature and followed by the incubation with the primary antibodies at 4 °C over night. After washing, the membranes were incubated with the secondary antibody at 20 °C for 2 h, and bound antibody was detected using an ECL chemiluminescent system.
2.11 Statistical analysis
All experiments were performed at least in triplicate for each assay. The results were presented as mean ± SD. Student's t-test was used to analyze the data pertaining to measuring differences in gene expression. One-way ANOVA was applied to analyze the data pertaining to cell proliferation and ALP activity (a probability level of 5%, P < 0.05 was considered statistically significant).
3. Results and discussion
3.1 Construction and characterization of PEN/MT01
The derivative PEN was synthesized through the modification of PEI-25K with N-isopropylacrylamide via Michael addition as previously reported.19 Based on the optimization of grafting ratio,20 PEN175 was selected and employed in the present research. The binding affinity of PEN to MT01 was examined by agarose gel retardation assay. As is shown in Fig. 1, the migration of MT01 was completely retarded by PEN when the PEN/MT01 w/w ratio was over 0.5
:
1. The ability to bind and condense DNA into nano-sized complexed particles is a necessary prerequisite for efficient gene delivery vectors. Our data suggest that neutral or positively charged complexes between PEN/MT01 were formed when the w/w ratio reached 0.5
:
1. Transport of the complexes into target cells requires passage across different barriers, ranging in sizes from micrometers to nanometer.21,22 A moderate positive surface charge and a proper particle size of carrier/DNA complexes are critical for efficient endocytosis and transfection. In this study, the particle size and zeta potential of PEN/MT01 at different mass ratios were therefore determined by a Malvern Nano ZS90 Zetasizer. As shown in Table 1, with an increasing ratio of polymer to MT01, the nanocomplexes could be formed ranging in size between 34.8 and 283.9 nm and the zeta potential increased from −24.4 at a ratio of 2
:
1 up to 25.7 at a ratio of 10
:
1. The TEM images of PEN/MT01 are presented in Fig. 2a and b. TEM images showed that PEN/MT01 polyplexes had a globular morphology. The average size of the PEN/MT01 polyplexes is about 200 nm.
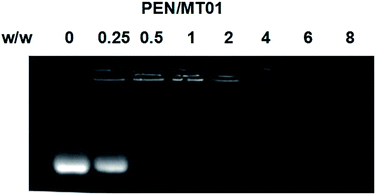 |
| Fig. 1 Agarose gel electrophoresis retardation assay of PEN/MT01 at various w/w ratios of 0, 0.25, 0.5, 1, 2, 4, 6, 8. | |
Table 1 The particle size and zeta potential of PEN/MT01 complexes at different ratios
Sample |
Particle size (nm) |
Zeta potential (mV) |
2 : 1 |
211 |
−24.4 |
4 : 1 |
283.9 |
0.6 |
6 : 1 |
258 |
8.04 |
8 : 1 |
34.8 |
21 |
10 : 1 |
52.58 |
25.7 |
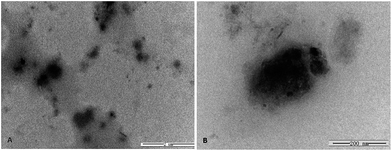 |
| Fig. 2 TEM micrographs of PEN/MT01 polyplexes with N/P ratio 6.0. | |
3.2 Cell viability assay
To determine the cytotoxicity of PEN/MT01 nanocomposites, MG63 cells were transfected with PEN/MT01 complexed at different w/w ratios. At 48 hours post-transfection, the cytotoxicity was examined by MTT assay. As shown in Fig. 2, the results revealed that while there was almost no cytotoxicity of PEN/MT01 at a w/w ratio of less than 8
:
1, there was significant toxicity of PEN/MT01 with a w/w ratio of 10
:
1. At a mass ratio of 6
:
1, PEN exhibited higher cell viability compared to other ratios (Fig. 3). Taken together, these characteristics qualify the derivative PEN as a potentially efficient carrier for MT01 delivery.
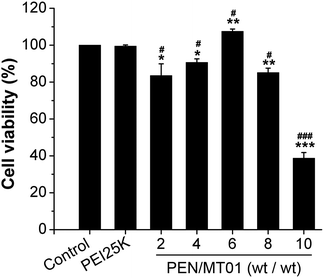 |
| Fig. 3 Cell viability of PEN/MT01 complexes transfected at various w/w ratios (2 : 1, 4 : 1, 6 : 1, 8 : 1, and 10 : 1) into MG63 cells. | |
3.3 Evaluation of transfection efficiency of PEN/MT01
Following co-culture of MG63 cells with PEN/MT01 that was labeled with Cy5, PEN/MT01 complexes could be visualized as shown in Fig. 4. The fluorescence intensity was observed to increase with increased ratio of polymer, consistent with quantitative analysis of the mean fluorescence intensity. Although PEN exhibited a slightly higher transfection efficiency at a mass ratio of 8
:
1 compared with that at 6
:
1, cell viability was enhanced at the latter ratio, therefore a mass ratio of 6
:
1 was employed for further delivery studies.
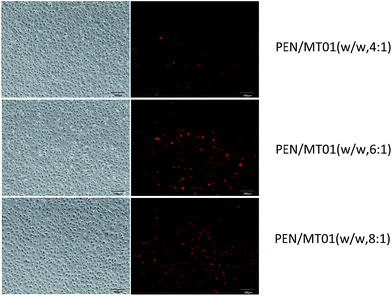 |
| Fig. 4 Fluorescence microscopic images of MG63 cells exposed to PEN/Cy5-labeled/MT01 at different mass ratio. | |
3.4 Cell cycle analysis after PEN/MT01 transfection
Flow cytometry revealed that in the cells treated with PEN/MT01 nanocomplexes, more cells were observed in S and G2 phases compared with the control group cells (Fig. 5). This finding indicated that PEN/MT01 nanocomplexes affected the cell cycle and promoted cell proliferation of MG63 cells by increasing the proportion of cells in the DNA synthesis phase.
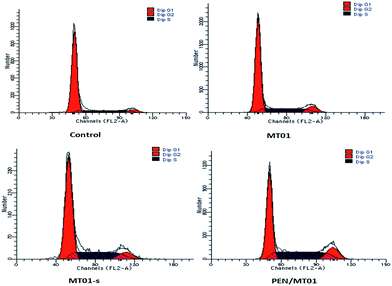 |
| Fig. 5 Induction of cell cycle arrest in MG63 cells 72 h post incubation with MT01 as assessed by FACS analysis. | |
3.5 ALP activity
ALP activity is an early marker of osteogenic differentiation, par-ticipating in the regulation of the mature bone matrix calcification process and affecting cell osteogenic mineralization ability.23 As shown in Fig. 6, MG63 cells treated with PEN/MT01 nanoparticles displayed more dark purple staining than cells of the control group. ALP expression levels in the PEN/MT01 group were found to be higher than levels in the control group at 24 h, 48 h and 72 h of treatment. Moreover, ALP activity was markedly enhanced in the PEN/MT01 group compared to the MT01 and MT01s treatment groups, being statistically significant different at 24 h, 48 h and 72 h (P < 0.05). This result demonstrated that PEN possesses a stable and efficient transfection capability.
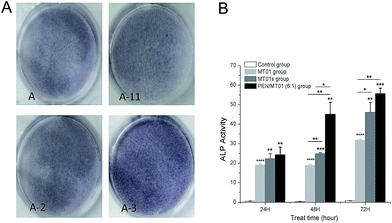 |
| Fig. 6 ALP staining following PEN/MT01 treatment (A) control. (A-1) MT01. (A-2) MT01-s. (A-3) PEN/MT01 (6 : 1, w/w). (B) Effect of PEN/MT01 treatment on ALP activity of MG63 cells, at different time intervals. | |
3.6 Real-time PCR assay
To evaluate the effect of PEN/MT01 nanocomplexes on the osteogenic differentiation of MG63 cells in vitro, mRNA expression levels of OPG, RANKL, and Runx-2 were determined at 24 h, 48 h, and 72 h (Fig. 7). OPG expression levels were significantly increased at 48 h and 72 h after PEN/MT01 treatment compared with the control. Conversely, RANKL mRNA expression levels were significantly lower at 48 h and 72 h after PEN/MT01 treatment compared with the control. Finally, Runx-2 mRNA expression levels were significantly increased at 72 h after PEN/MT01 treatment compared with the control. These results provide direct evidence for the upregulation of osteoblastic activity by PEN/MT01.
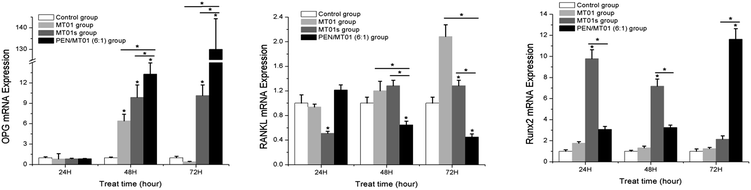 |
| Fig. 7 Expression of specific osteogenic genes (OPG, RANKL, Runx-2) in MG63 cells evaluated by qPCR at 24, 48, and 72 hours of treatment with MT01, MT01a or PEN/MT01. | |
3.7 Western blotting analysis
To obtain a further insight into the mechanism of PEN/MT01 induced osteogenic differentiation, MG63 cells were treated with MT01, MT01s or PEN/MT01 and the protein expression levels of Sp7, Runx-2, OPG and RANKL were measured by the Western blotting technique at different time points of treatment. As shown in Fig. 8, after MT01 transfection using PEN, the expression levels of Sp7, Runx-2 and OPG appeared to be increased compared to those of the control group and the free MT01 group. Notably, MT01s treatment also induced an increase in the levels of these proteins, consistent with previous research results.5 In contrast to MT01s, protein expression levels of OPG and Sp7 after PEN/MT01 treatment were higher at 24 h and 72 h, while for Runx-2, higher levels were observed from 48 h to 72 h after PEN/MT01 treatment. On the other hand, RANKL expression levels were decreased after MT01 transfection, and markedly down-regulated expression of RANKL was detected in the PEN/MT01 group.
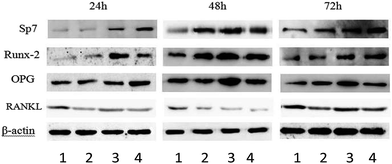 |
| Fig. 8 Western blotting analysis of expression levels of Sp7, Runx-2, OPG and RANKL in MG63 cells after transfection of free MT01 (lane 2), of MT01 modified by phosphorothioate (lane 3) or of MT01mediated by PEN (lane 4). Lane 1: control. | |
4. Discussion
Repair of large bone defects is a major challenge, requiring sustained stimulation to continually promote bone formation locally.24 Recent advances in cell and molecular biology have enabled researchers to integrate medical gene technology into the field of bone regeneration. Our previous studies have found that ODN MT01 could regulate the balance of bone formation and resorption, and that it therefore has a great potential in the rebuilding of alveolar bone.5–7 Here we applied PEN as a carrier for MT01 delivery with the aim to enhance its cellular uptake capability and its stability.
Different types of PEI are regarded as the most effective non-viral gene vectors due to their ability to reduce the negative charge of DNA and to partially condense its normally large hydrodynamic volume and the ability to escape from lysosomes.25 PEN exhibits a higher transfection efficiency and lower cytotoxicity than PEI25K which is achieved through modification of PEI25K with N-isopropylacrylamide via Michael addition. Compared with previous modification strategies, this approach did not reduce the content of protonated amines, and thus would not influence the formation of stable nanocomplexes between the carrier and pDNA through electrostatic interaction.19 In a previous study, PEN has been employed as a vector to accomplish p53 gene delivery. Compared with PEI25K and Lipofectamine2000, the derivative exhibited lower protein adsorption, but better or higher gene transfection efficiency.20 In this paper, we describe for the first time using PEN as ODN vector for bone defect treatment.
According to the reaction shown in Fig. 1, the complete MT01 retardation could be induced by PEN at a mass ratio of ≥0.5, indicating the formation of nanocomplex between PEN and MT01. Surface properties of nanoparticles, such as particle size and zeta potential, are important factors for a gene delivery vector. Based on the Table 1, the range actually was 34.8–283.9 nm in the mass ratios studied and the values of zeta potentials exhibited an increasing tendency with an increasing ratio of carrier. However, there was significant toxicity of PEN/MT01 when transfected into cells at a w/w ratio of 10
:
1. On the other hand, the particle size and zeta potential were measured to be 258 nm and +8.04 mV, respectively, at a mass ratio of 6
:
1, and transfection of cells with PEN/MT01 at this ratio even promoted both cell proliferation (Fig. 3).
Our data further demonstrated that at a w/w ratio of 6
:
1, PEN/MT01 enabled efficient transfection of MG63 cells (Fig. 4). In addition, qPCR and western blot analyses showed that the PEN/MT01 complex increased osteogenic gene expression (Fig. 7 and 8). Osteogenesis is tightly regulated by a diverse set of internal and external factors such as hormones, growth factors, transcriptional factors, and signaling pathways that result in the formation of mineralized bone.26 Runx-2 is a critical transcription factor in the process of osteogenesis and is responsible for the activation of osteoblast differentiation marker genes.26,27 In the current study, the mRNA expression level of Runx-2 was greatly influenced by MT01, with PEN/MT01 treatment resulting in a more profound effect compared with MT01-s and MT01 treatment at 48 h and 72 h. Similarly, the mRNA expression level of OPG was clearly increased after MT01 transfection, with significant up-regulation of OPG expression detected in the PEN/MT01 group. Not surprisingly, RANKL expression was decreased in the PEN/MT01 group. OPG/RANK/RANKL signaling is considered to be a key connection pathway between osteoblasts and osteoclastogenesis. RANKL is mainly synthesized by cells of the osteoblastic lineage and is essential for mediating bone resorption through mediating osteoclastogenesis and the activation of mature osteoclasts.26,28 In the present study, we found that MT01 transfection mediated by PEN or modified by sulfur led to the promotion of osteogenesis to a greater extent than free MT01. It was clear from our findings that PEN efficiently mediated the intracellular delivery of MT01 to increase OPG expression while decreasing RANKL expression. Thus, PEN has the potential to promote the osteogenic capacity of MT01. Compared to MT01-s, PEN/MT01 possessed remarkable superiority, indicating that PEN possessed stable and efficient transfection capability after modification. It should noted that the use of particulate carriers could avoid specific side effects caused by chemical modifications. Our results indicate that PEN can condense MT01 and protect it from being damaged during primary emulsion.
5. Conclusions
The delivery of MT01 into MG63 cells was successfully achieved using the PEN derivative. Following PEN-mediated MT01 transfection, osteoblastic differentiation of MG63 cells was efficiently promoted. Using non-chemical modification of ODN with PEN represents a simple and effective alternative approach, and PEN-mediated MT01 delivery has great potential for alveolar bone regeneration.
Acknowledgements
This research was supported by the Natural Science Foundation of China (No. 81371153 and 81600879), the Science Technology Program of Jilin Province (No. 20150101173JC) and the Medical Support Program of the Jilin university (No. 2013108031 and 470110000423). The PEN was provided by the Key Laboratory for Molecular Enzymology and Engineering of Ministry of Education, School of Life Sciences, Jilin University.
References
- A. Hernández, E. Sánchez and I. Soriano, Acta Biomater., 2012, 8, 781–791 CrossRef PubMed.
- C. Qiao, K. Zhang and H. Jin, Int. J. Nanomed., 2013, 8, 2985–2995 Search PubMed.
- U. Ripamonti and J. C. Petit, Cytokine Growth Factor Rev., 2009, 20, 489–499 CrossRef CAS PubMed.
- G. Yang, M. Wan and Y. Zhang, Immunology, 2011, 131, 501–512 CrossRef PubMed.
- Z. Feng, Y. Shen and L. Wang, Int. J. Mol. Sci., 2011, 12, 2543–2555 CrossRef CAS PubMed.
- Y. Shen, Z. Feng and C. Lin, Int. J. Mol. Sci., 2012, 13, 2877–2892 CrossRef CAS PubMed.
- X. Hou, Y. Shen and C. Zhang, Int. J. Mol. Sci., 2012, 7902–7914 CrossRef CAS PubMed.
- G. Lambert, E. Fattal and P. Couvreur, Adv. Drug Delivery Rev., 2001, 47, 99–112 CrossRef CAS PubMed.
- M. Heikenwalder, M. Polymenidou and T. Junt, Nat. Med., 2004, 10, 187–192 CrossRef CAS PubMed.
- N. Hanagata, Int. J. Nanomed., 2012, 7, 2181–2195 CrossRef CAS PubMed.
- F. Takeshita, C. A. Leifer and I. Gursel, J. Immunol., 2001, 167, 3555–3558 CrossRef CAS.
- S. C. Semple, S. K. Klimuk and T. O. Harasym, Methods Enzymol., 2000, 313, 322–341 CAS.
- W. T. Godbey, K. K. Wu and A. G. Mikos, Proc. Natl. Acad. Sci. U. S. A., 1999, 96, 5177–5181 CrossRef CAS.
- A. L. Gomes dos Santos, A. Bochot and N. Tsapis, Pharm. Res., 2006, 23, 770–781 CrossRef CAS PubMed.
- H. Tian, L. Lin and J. Chen, J. Controlled Release, 2011, 155, 47–53 CrossRef CAS PubMed.
- X. Zhao, Z. Li and H. Pan, Acta Biomater., 2013, 9, 6694–6703 CrossRef CAS PubMed.
- F. W. Huang, H. Y. Wang and C. Li, Acta Biomater., 2010, 6, 4285–4295 CrossRef CAS PubMed.
- M. L. Forrest, G. E. Meister and J. T. Koerber, Pharm. Res., 2004, 21, 365–371 CrossRef CAS.
- H. Tian, F. Li, J. Chen and Y. Huang, Macromol. Biosci., 2012, 12, 1680–1688 CrossRef CAS PubMed.
- J. Zhang, D. Wu and Z. Xing, Colloids Surf., B, 2015, 129, 54–62 CrossRef CAS PubMed.
- M. R. Park, K. O. Han and I. K. Han, J. Controlled Release, 2005, 105, 367–380 CrossRef CAS PubMed.
- Y. Liu and T. M. Reineke, J. Am. Chem. Soc., 2005, 127, 3004–3015 CrossRef CAS PubMed.
- Y. Takayama and K. Mizumachi, J. Biosci. Bioeng., 2009, 107, 191–195 CrossRef CAS PubMed.
- K. Andreas, R. Zehbe and M. Kazubek, Acta Biomater., 2011, 7, 1485–1495 CrossRef CAS PubMed.
- L. Zhao, K. Zhang and W. Bu, RSC Adv., 2016, 41, 34081–34089 RSC.
- G. Chen, C. Deng and Y. P. Li, Int. J. Biol. Sci., 2012, 8, 272–288 CrossRef CAS PubMed.
- S. Vimalraj, B. Arumugam and P. J. Miranda, Int. J. Biol. Macromol., 2015, 78, 202–208 CrossRef CAS PubMed.
- M. C. Walsh and Y. Choi, Front. Immunol., 2014, 5, 511 Search PubMed.
|
This journal is © The Royal Society of Chemistry 2017 |