DOI:
10.1039/C6RA26877C
(Communication)
RSC Adv., 2017,
7, 256-259
Chiral carbon–sulfur center formation via Pd-catalyzed asymmetric allylic thioetherification: synthesis of allylic thioethers†
Received
16th November 2016
, Accepted 1st December 2016
First published on 23rd December 2016
Abstract
Pd-catalyzed asymmetric allylic thioetherification reaction of various sodium thiolates was realized, which gave the allylic thioethers in good to high yields with high enantioselectivities. The reaction results considerably depend on the substrates and the bulky sulfur nucleophile led to excellent enantioselectivity as well.
Approximately one-fifth of the 200 most-prescribed drugs in 2011 were organosulfur compounds.1 More interestingly, the optically active sulfur-containing compounds exhibit excellent biological activity.2 For example, the popular pharmaceutical products and naturally occurring compounds such as biotin,3 montelukast,4 eflucimibe,5 and mPEES-1 inhibitors contain a chiral center bearing a carbon–sulfur (C–S) bond (Fig. 1).6 Noticeably, a direct way for the construction of a C–S bond is by palladium-catalyzed allylic substitution.7 Pd-catalyzed asymmetric allylic substitution has become a powerful tool for the synthesis of chiral compounds.7,8 To this context, allylic thioetherification reactions have been less reported since sulfur nucleophiles can poison the transition-metal catalyst.9 Pd-catalyzed allylic substitutions of sulfur nucleophiles revealed that 4-chlorothiophenol,10 2-mercaptopyridine,10 2-mercaptopyrimidine,10 tert-butylthiol,10j tri-tert-butyl(tert-butylthio)silane,10j sulfinates,10e,10f,11 thiocarbamates,12 and thioacetates13 were suitable nucleophiles.
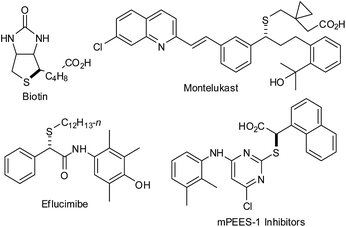 |
| Fig. 1 Four compounds with a chiral C–S center. | |
Iridium (Ir)-catalyzed asymmetric allylic substitutions of sulfur nucleophiles was contributed by our group14 and others.15 To the best of our knowledge, the C–S bond construction by Pd-catalyzed asymmetric allylations is a largely unexplored area in organic synthesis. In this paper, we report an enantioselective Pd-catalyzed allylic substitution of symmetrical allylic acetates with sodium thiolate (NaSR), which afford the allylic thioethers with high enantioselectivities.
An initial thioetherification reaction between (E)-1,3-bis(4-chlorophenyl)allyl acetate 1a and sodium prop-2-ene-1-thiolate 2a was explored with Pd-complex generated from Pd2(dba)3, Pd(OAc)2, and [Pd(C3H5)Cl]2 with (R)-BINAP L1 (ref. 16) at −10 °C, respectively (Table 1, entries 1–3). To our delight, the allylic thioether 3a was obtained with a 61% yield and 91% enantiomeric excesses (ee) when [Pd(C3H5)Cl]2/L1 was utilized (Table 1, entry 3). The remaining Pd salts such as Pd2(dba)3 and Pd(OAc)2 are ineffective for this reaction (entries 1 and 2). Solvent survey indicated that DCM is suitable (Table 1, entry 3) and the other solvents such as THF and toluene gave the poor yields with the moderate ee (Table 1, entries 4 and 5). In contrast, the reaction gave 3a in a 50% yield with 80% ee in the absence of base (Table 1, entry 6). These results illustrated that the nature of bases has a considerable influence on the enantioselectivities. Thus, a variety of bases including KOAc, NaOAc, KI, trimethylsilyl (E)-N-(trimethylsilyl)formimidate (BSA), DBACO, and KOAc/BSA was examined. A combination of KOAc and BSA gave rise to a superior result (Table 1, entry 11), whereas other bases led to fair yields with moderate to high ee (Table 1, entries 7–11). A slight excess 2a was employed and it gave the best result (Table 1, entry 11 vs. 12).
Table 1 Optimization of the reaction conditionsa

|
En |
Pd salt |
L |
Sol |
Base |
Yieldb [%] |
eec [%] |
Reaction conditions: 1a (0.20 mmol), 2a (1.0 equiv.), Pd salt (2.5 mmol%), L1–L5 (5 mmol%), base (1.0 equiv.), and solvent (2.0 mL) at −10 °C under argon. Isolated yield. Determined by HPLC. 2a = 1.2 equiv. At 0 °C. |
1 |
Pd2(dba)3 |
L1 |
DCM |
KOAc |
— |
— |
2 |
Pd(OAc)2 |
L1 |
DCM |
KOAc |
— |
— |
3 |
[Pd(C3H5)Cl]2 |
L1 |
DCM |
KOAc |
61 |
91 |
4 |
[Pd(C3H5)Cl]2 |
L1 |
THF |
KOAc |
30 |
85 |
5 |
[Pd(C3H5)Cl]2 |
L1 |
Toluene |
KOAc |
35 |
75 |
6 |
[Pd(C3H5)Cl]2 |
L1 |
DCM |
— |
50 |
80 |
7 |
[Pd(C3H5)Cl]2 |
L1 |
DCM |
NaOAc |
60 |
84 |
8 |
[Pd(C3H5)Cl]2 |
L1 |
DCM |
KI |
50 |
92 |
9 |
[Pd(C3H5)Cl]2 |
L1 |
DCM |
BSA |
61 |
92 |
10 |
[Pd(C3H5)Cl]2 |
L1 |
DCM |
DBACO |
60 |
90 |
11 |
[Pd(C3H5)Cl]2 |
L1 |
DCM |
KOAc/BSA |
73 |
92 |
12d |
[Pd(C3H5)Cl]2 |
L1 |
DCM |
KOAc/BSA |
81 |
95 |
13d |
[Pd(C3H5)Cl]2 |
L2 |
DCM |
KOAc/BSA |
Trace |
— |
14d |
[Pd(C3H5)Cl]2 |
L3 |
DCM |
KOAc/BSA |
— |
— |
15d |
[Pd(C3H5)Cl]2 |
L4 |
DCM |
KOAc/BSA |
— |
— |
16d |
[Pd(C3H5)Cl]2 |
L5 |
DCM |
KOAc/BSA |
— |
— |
17d,e |
[Pd(C3H5)Cl]2 |
L1 |
DCM |
KOAc/BSA |
68 |
83 |
The ligands are crucial to Pd-catalyzed asymmetric allylic substitutions.8 Therefore, the effect of ligands including (R)-Tol-BINAP L2,12 Trost L3,17 Josiphos L4,18 and PHOX L5 (ref. 19) (Fig. 2) on the allylic thioetherification was examined. The allylic thioetherification of 2a took place with the best enantioselectivity in the presence of the catalyst derived from (R)-BINAP L1 (Table 1, entry 12). Unexpectedly, L2 structurally similar to L1 only gave a trace amount of 3a (Table 1, entry 13). These outcomes indicated that the steric demand of the ligands has great influence on this reaction. Subsequent investigation indicated that the Pd complexes made from L3–L5 failed to promote this reaction (Table 1, entries 14–16). The reaction was carried out at 0 °C and it afforded a somewhat worse result than that at −10 °C (Table 1, entry 12 vs. 17).
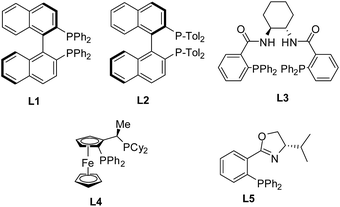 |
| Fig. 2 Chiral ligands L1–L5 used in this allylic sulfane. | |
Using this procedure, the scope of the thioetherification reactions of a range of allylic acetates 1 with sodium prop-2-ene-1-thiolate 2a was subsequently explored (Table 2). The allylic substrates 1a–f with electron-poor group (e.g., p-Cl, p-F, p-Br, m-Cl, m-F, and m-Br) on the phenyl ring gave the corresponding products 3a–f in moderate to high yields with the high level of the enantioselectivities.
Table 2 The scope of the allyl acetates 1 and sodium thiolates 2a,b,c,d

|
Reaction conditions: 1 (0.20 mmol), 2 (1.2 equiv.), [Pd(C3H5Cl)]2 (2.5 mmol), L1 (5 mmol), KOAc (1.0 equiv.), BSA (1.0 equiv.) and solvent (2.0 mL) at −10 °C under argon. Isolated yield. Determined by HPLC. At −15 °C. |
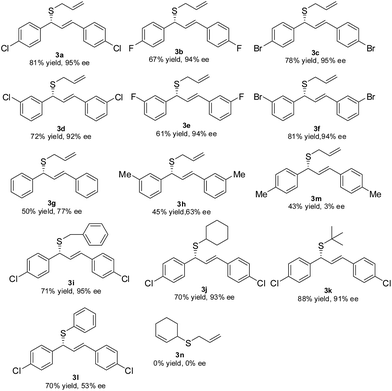 |
In addition, both (E)-1,3-diphenylallyl acetate 1g and the allylic substrate 1h with an electron-rich group (e.g., m-CH3) on the phenyl ring offered the allylic thioethers 3g and 3h in the acceptable yields with the moderate enantioselectivities. These results suggested that sodium prop-2-ene-1-thiolate 2a is a somewhat weak nucleophile and the reaction results considerably depend upon the allylic substrates in these cases. The steric demand and nature of sulfur nucleophiles 2 was also investigated. The representative sulfur nucleophiles such as sodium phenylmethanethiolate 2b, sodium cyclohexanethiolate 2c, sodium 2-methylpropane-2-thiolate 2d, and sodium benzenethiolate 2e were thus examined. 2b gave the results similar to that of 2a; and a bulky 2c resulted in the corresponding 3j in a 70% yield with 93% ee. Significantly, the more steric hindered 2d was utilized at −15 °C and it afforded the corresponding 3k in a 88% yield with 91% ee. Aromatic sulfur nucleophile 2e also gave rise to the corresponding 3l in a 70% yield with a lowering ee (Table 2). The allylic substrate 1i bearing an electron-donating group (e.g., p-CH3) on the phenyl ring provided 3m with a 43% yield with 3% ee. The aliphatic substrate such as cyclohex-2-enyl acetate 1j was also examined and the corresponding product 3n were not observed (Table 2).
A single-crystal X-ray diffraction analysis of 3c20 (Fig. 3) illustrates the absolute configuration of 3c as R (see ESI† for details).
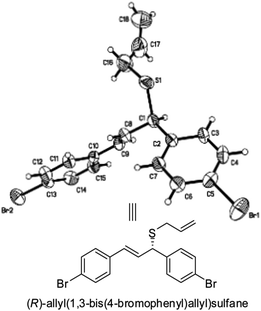 |
| Fig. 3 X-ray structure of (R)-3c. | |
Conclusions
In conclusion, we developed a practical method for the formation of C–S bond via Pd-catalyzed asymmetric allylic thioetherifications, which gives the allylic thioethers in good to high yields with high enantioselectivities. This method allows the use of bulky sulfur nucleophiles, illustrates good tolerance of the aryl-substituted allyl acetates, and offers a new way to chiral allylic thioethers.
Acknowledgements
We gratefully acknowledge the NSFC (21272175 and 20942003) for generous financial support.
Notes and references
- The table of Top 200 Pharmaceutical Products by Total US Prescriptions in 2011 is available via the Internet at http://www.pharmacytimes.com/publications/issue/2012/July2012/Top200-Drugs-of-2011.
-
(a) A. Nudelman, The Chemistry of Optically Active Sulfur Compounds, Gordon and Breach, New York, 1984 Search PubMed;
(b) Organosulfur Chemistry in Asymmetric Synthesis, ed. T. Toru and C. Bolm, Wiley-VCH, Weinheim, Germany, 2008 Search PubMed.
-
(a) A. K. Ghosh, W. J. Thompson, M. P. Munson, W. Liu and J. R. Huff, Bioorg. Med. Chem. Lett., 1995, 5, 83 Search PubMed;
(b) C. U. Kim, L. R. McGee, S. H. Krawczyk, E. Harwood, Y. Harada, S. Swaminathan, N. Bischofberger, M. S. Chen, J. M. Cherrington, S. F. Xiong, L. Griffin, K. C. Cundy, A. Lee, B. Yu, S. Gulnik and J. W. Erickson, J. Med. Chem., 1996, 39, 3431 CrossRef CAS PubMed;
(c) H. G. F. Richter, P. Angehrn, C. Hubschwerlen, M. Kania, M. G. P. Page, J.-L. Specklin and F. K. Winkler, J. Med. Chem., 1996, 39, 3712 CrossRef CAS PubMed;
(d) J. D. Buynak, L. Vogeti and H. Chen, Org. Lett., 2001, 3, 2953 CrossRef CAS PubMed;
(e) H. Li, L. Zu, H. Xie, J. Wang, W. Jiang and W. Wang, Org. Lett., 2007, 9, 1833 CrossRef CAS PubMed.
- A. Halama, J. Jirman, O. Boušková, P. Gibala and K. Jarrah, Org. Process Res. Dev., 2010, 14, 425 CrossRef CAS.
- M. Tisdale, S. D. Kemp, N. R. Parry and B. A. Larder, Proc. Natl. Acad. Sci. U. S. A., 1993, 90, 5653 CrossRef CAS.
-
(a) G. Sobal, E. J. Menzel and H. Sinzinger, Biochem. Pharmacol., 2001, 61, 373 CrossRef CAS PubMed;
(b) R. Suhas, S. Chandrashekar and D. C. Gowda, Eur. J. Med. Chem., 2012, 48, 179 CrossRef CAS PubMed.
- For a review, see: W. Liu and X. M. Zhao, Synthesis, 2013, 45, 2051 CrossRef CAS.
- For the reviews and selective papers, see:
(a) A. Saitoh, K. Achiwa, K. Tanaka and T. Morimoto, J. Org. Chem., 2000, 65, 4227 CrossRef CAS PubMed;
(b) Y. Tamaru, Eur. J. Org. Chem., 2005, 2647 CrossRef CAS;
(c) B. M. Trost and D. L. Van Vranken, Chem. Rev., 1996, 96, 395 CrossRef CAS PubMed;
(d) M. Johannsen and K. A. Jørgensen, Chem. Rev., 1998, 98, 1689 CrossRef CAS PubMed;
(e) T. Hayashi, J. Organomet. Chem., 1999, 576, 195 CrossRef CAS;
(f) G. Helmchen and A. Pfaltz, Acc. Chem. Res., 2000, 33, 336 CrossRef CAS PubMed;
(g) L.-X. Dai, T. Tu, S.-L. You, W.-P. Deng and X.-L. Hou, Acc. Chem. Res., 2003, 36, 659 CrossRef CAS PubMed;
(h) B. M. Trost and M. L. Crawley, Chem. Rev., 2003, 103, 2921 CrossRef CAS PubMed;
(i) B. M. Trost, J. Org. Chem., 2004, 69, 5813 CrossRef CAS PubMed;
(j) B. M. Trost, M. R. Machacek and A. Aponick, Acc. Chem. Res., 2006, 39, 747 CrossRef CAS PubMed;
(k) Z. Lu and S. Ma, Angew. Chem., Int. Ed., 2008, 47, 258 CrossRef CAS PubMed;
(l) M. Dieguez and O. Pamies, Acc. Chem. Res., 2010, 43, 312 CrossRef CAS PubMed.
-
(a) L. L. Hegedus and R. W. McCabe, Catalyst Poisoning, Marcel Dekker, New York, 1984 Search PubMed;
(b) A. T. Hutton, in Comprehensive Coordination Chemistry, ed. G. Wilkinson, R. D. Gillard and J. A. McCleverty, Pergamon: Oxford, U.K., 1984, vol. 5, p. 1151 Search PubMed.
- For Pd-catalyzed allylic substitutions of sulfur nucleophiles, see:
(a) C. Goux, P. Lhoste and D. Sinou, Tetrahedron Lett., 1992, 33, 8099 CrossRef CAS;
(b) C. Goux, P. Lhoste and D. Sinou, Tetrahedron, 1994, 50, 10321 CrossRef CAS;
(c) M. Moreno-Mañas, R. Pleixats and M. Villarroya, Tetrahedron, 1993, 49, 1457 CrossRef;
(d) N. Komine, A. Sako, S. Hirahara, Y. Hirano and M. Komiya, Chem. Lett., 2005, 34, 246 CrossRef CAS; For Pd-catalyzed asymmetrical allylic versions, see:
(e) H. Eichelmann and H. J. Gais, Tetrahedron: Asymmetry, 1995, 6, 643 CrossRef CAS;
(f) B. M. Trost, M. G. Organ and G. A. O'Doherty, J. Am. Chem. Soc., 1995, 117, 9662 CrossRef CAS;
(g) B. M. Trost, M. J. Krische, R. Radinov and G. Zanoni, J. Am. Chem. Soc., 1996, 118, 6297 CrossRef CAS;
(h) B. M. Trost, A. C. Krueger, R. C. Bunt and J. Zambrano, J. Am. Chem. Soc., 1996, 118, 6520 CrossRef CAS;
(i) B. M. Trost and R. Radinov, J. Am. Chem. Soc., 1997, 119, 5962 CrossRef CAS;
(j) M. Frank and H. J. Gais, Tetrahedron: Asymmetry, 1998, 9, 3353 CrossRef CAS;
(k) H. J. Gais, T. Jagusch, N. Spalthoff, F. Gerhards, M. Frank and G. Raabe, Chem.–Eur. J., 2003, 9, 4202 CrossRef CAS PubMed;
(l) W. Liu, X. M. Zhao, H. B. Zhang and L. Zhang, Chem. Commun., 2015, 51, 655 RSC.
-
(a) H. J. Gais, H. Eichelmann, N. Spalthoff, F. Gerhards, M. Frank and G. Raabe, Tetrahedron: Asymmetry, 1998, 9, 235 CrossRef CAS;
(b) B. M. Trost, M. L. Crawley and C. B. Lee, J. Am. Chem. Soc., 2000, 122, 6120 CrossRef CAS;
(c) Y. Uozumi and T. Suzuka, Synthesis, 2008, 1960 CrossRef CAS.
- L. E. Overman, S. W. Roberts and H. F. Sneddon, Org. Lett., 2008, 10, 1485 CrossRef CAS PubMed.
-
(a) D. Sinou, S. Divekar, M. Safi and M. Soufiaoui, Sulfur Lett., 1999, 22, 125 CAS;
(b) B. J. Lüssem and H. J. Gais, J. Org. Chem., 2004, 69, 4041 CrossRef PubMed.
-
(a) S. C. Zheng, N. Gao, W. Liu, D. G. Liu, X. M. Zhao and T. Cohen, Org. Lett., 2010, 12, 4454 CrossRef CAS PubMed;
(b) N. Gao, S. Zheng, W. Yang and X. Zhao, Org. Lett., 2011, 13, 1514 CrossRef CAS PubMed;
(c) W. Q. Huang, S. C. Zheng, J. L. Tang and X. M. Zhao, Org. Biomol. Chem., 2011, 9, 7897 RSC;
(d) S. C. Zheng, W. Q. Huang, N. Gao, R. M. Cui, M. Zhang and X. M. Zhao, Chem. Commun., 2011, 47, 6969 RSC;
(e) N. Gao, X. W. Guo, S. C. Zheng, W. K. Yang and X. M. Zhao, Tetrahedron, 2012, 68, 9413 CrossRef CAS;
(f) W. Liu, X. M. Zhao, H. B. Zhang, L. Zhang and M. Z. Zhao, Chem.–Eur. J., 2014, 20, 16873 CrossRef CAS PubMed.
-
(a) M. Ueda and J. F. Hartwig, Org. Lett., 2010, 12, 92 CrossRef CAS PubMed;
(b) M. Roggen and E. M. Carreira, Angew. Chem., Int. Ed., 2012, 51, 8652 CrossRef PubMed.
-
(a) H. Takaya, K. Mashima, K. Koyano, M. Yagi, H. Kumobayashi, T. Taketomi and S. Akutagawa, J. Org. Chem., 1986, 51, 629 CrossRef CAS;
(b) R. Noyori and H. Takaya, Acc. Chem. Res., 1990, 23, 345 CrossRef CAS;
(c) S. Akutagawa, Appl. Catal., A, 1995, 128, 171 CrossRef CAS;
(d) M. McCarthy and P. J. Guiry, Tetrahedron, 2001, 57, 3809 CrossRef CAS;
(e) H. Shimizu, I. Nagasaki and T. Saito, Tetrahedron, 2005, 61, 5405 CrossRef CAS.
- B. M. Trost, D. L. Van Vranken and C. Bingel, J. Am. Chem. Soc., 1992, 114, 9327 CrossRef CAS.
- H. C. L. Abbenhius, U. Burckhardt, V. Gramlich, C. Kollner, P. S. Pregosin, R. Salzman and A. Togni, Organometallics, 1995, 14, 759 CrossRef.
-
(a) P. V. Matt and A. Pfaltz, Angew. Chem., Int. Ed., 1993, 32, 566 CrossRef;
(b) J. Sprinz and G. Helmchen, Tetrahedron Lett., 1995, 34, 1769 CrossRef.
- ESI.‡.
Footnotes |
† Electronic supplementary information (ESI) available. CCDC 1485308. For ESI and crystallographic data in CIF or other electronic format see DOI: 10.1039/c6ra26877c |
‡ The authors wish it to be known that, in their opinion, the first two authors should be regarded as joint First Authors. |
|
This journal is © The Royal Society of Chemistry 2017 |
Click here to see how this site uses Cookies. View our privacy policy here.