DOI:
10.1039/C6RA25533G
(Paper)
RSC Adv., 2017,
7, 12977-12983
Laccase-mediated in situ oxidation of dopa for bio-inspired coloration of silk fabric
Received
20th October 2016
, Accepted 9th February 2017
First published on 24th February 2017
Abstract
Bio-inspired or bionic coloration of fiber assemblies based on chemical oxidation of phenolics has gained more and more attention in recent years. To overcome the challenges of protecting the ecology and environment in textile processes, a novel approach for the coloration of silk fabrics via laccase-mediated in situ oxidation of dopa (dihydroxy phenylalanine) has been investigated in this work. In view of the fact that laccase is able to catalyze the oxidation of benzenediol followed by polymerization to produce strongly colored phenolic polymers, two biological coloration processes of silk fabrics based on in situ oxidation of dopa using laccase were investigated: (i) the adsorption of silk fabrics with dopa, followed by further catalysis of laccase and (ii) the simultaneous laccase-mediated polymerization and coloration of silk fabrics. Dosages of laccase and incubation time were evaluated in order to increase the color depth and fastness of the silk fabrics. The increase in laccase dosage and reaction time allowed improvement in the depth of the final color. The properties of colored silk fabrics were evaluated in terms of UV-protection and color fastness. Finally, the binding of the polymers of dopa with silk fibers was verified by Fourier transform infrared spectroscopy (FT-IR). The silk fabrics treated with laccase/dopa by a one-step process obtained K/S values of 16.935, light fastness of 4–5 level, and UPF of 100+. SEM and AFM observations showed that the surface of silk samples treated with laccase/dopa had numerous small flakes, which can be regarded as the melanin particles of dopa molecules, attached by the grafting reaction. The FT-IR analysis showed that the functional groups on the surfaces of laccase/dopa-treated silk fabrics were changed with the introduction of carbonyl groups. The results of XPS showed that the elemental composition of the surface of laccase/dopa-treated silk fabrics was similar to oral melanin. This dyeing technique could also be extended to the treatments of other types of fibers in textile dyeing and finishing processes.
Introduction
In the last few decades, the main focus of textile industry was on environmental protection, since large volumes of wastewater are generated in some wet processes like dyeing procedures, causing pollution problems. Thus, ecological dyeing techniques have become hot topics of research in textile dyeing.1,2 Ecological dyeing techniques such as supercritical carbon dioxide dyeing3 and ultrasonic dyeing4 techniques were investigated. However, the industrial applications of these dyeing technologies were restricted, since they required huge investments and were expensive. In addition to this, there has been a renewed interest in the use of natural dyes for textile coloration. However, the inherent problems associated with these techniques include poor dyeing reproducibility, low dye stability and color fastness that needs to be resolved.5
Nowadays, bio-inspired techniques, such as bionic dyeing, have been applied to textile processes. Xin's group was the first to report that dopamine could be chemically oxidized and then subsequently self-polymerized to obtain polymers that could be used for coloring fibers materials.6,7 This bionic dyeing technique, inspired by the adhesive properties of marine mussels, was a new method and concept in fiber coloration, in contrast to conventional dyeing of textiles. Due to some inherent shortcomings of chemical oxidation methods such as complex interaction, low yield, and environmental pollution,8 enzymatic bio-inspired coloration has been regarded as an environmentally-benign alternative approach.
Enzymes like polyphenol oxidases have been used in textile dyeing, since they are highly efficient, highly specific, require mild processing conditions, and are eco-friendly.9 Laccase is one kind of phenoloxidases that catalyzes the oxidation of large number of phenolic and non-phenolic aromatic compounds.10,11 It should be noted that several aromatic amines and phenolic compounds can be catalyzed by laccase, to form polymeric structures with chromophores, for fabric dyeing. S. Kim et al. confirmed that laccase from Trametes hirsuta was capable of reacting with flavonoids and polymerizes them, resulting in a strongly colored polymeric solution, which can be applied for the coloration of flax fabrics.12 Blanco et al. studied the color strength and fixation in laccase-assisted coloration of cotton fabrics by means of controlled pre-functionalization of cellulose with 2,5-diaminobenzenesulfonic acid.13,14
Dopamine with a bisphenol structure is an appropriate substrate for laccase. The chemical structure of dopa is similar to dopamine. The advantage of using dopa is that it is easily obtainable and economical. Dopa is also an important substrate for laccase, which can be catalyzed to dopa-melanin. Melanin not only imparts dark brown or black color to the hair and skin but also absorbs UV light and serves as an antioxidant for eliminating free radicals and plays a protective role in organisms.15,16 Melanin is also a natural pigment and has wide range of applications in chemical industry, food, cosmetics, textile, etc.17,18 The chemical structure of dopa is shown in Scheme 1a. Traditionally, dopa is catalyzed and oxidized to form melanin by chemical oxidation.19–21 However, laccase, an environmentally-benign catalyst, which requires milder reaction conditions, has been used in this work for the oxidative polymerization of dopa in aqueous solutions and the mechanism is shown in Scheme 1b.22
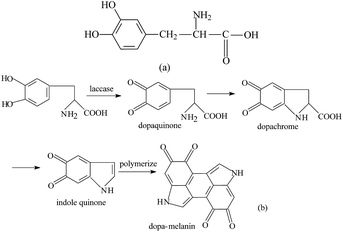 |
| Scheme 1 Structures of dopa (a) and laccase-mediated dopa (b). | |
In our present work, the biological coloration of silk fabric by in situ oxidation of dopa using laccase was studied, which combined the bio-inspired dyeing and enzymatic dyeing techniques of textiles. The effects of incubation time and laccase dosage on the polymerization of dopa, catalyzed by laccase, were studied in terms of the K/S values of silk fabrics. The surfaces of the dyed silk fabrics were characterized by FT-IR, X-ray photoelectron spectroscopy (XPS), atomic force microscopy (AFM), and scanning electron microscopy (SEM). The color fastness, binding force and anti-ultraviolet properties of the silk fabrics were also determined.
Experimental section
Materials and reagents
Laccase from Trametes versicolor (46.6 U g−1) was purchased from Novozymes (Shanghai, China). Silk fabrics were procured from Jiangsu Xinyuan Silk Textile Co. Ltd. (Nantong, China). Dopa (99% purity) was obtained from Aladdin Technology Co. Ltd. (Shanghai, China). All other chemicals used were commercially available and were of analytical grade.
Enzymatic grafting of dopa onto the surface of silk fabric
The silk fabrics were pretreated with distilled water for 10 min in order to improve wettability. Then the biological coloration of silk fabrics was carried out in the following steps. (i) Dopa treatment (Fig. 1a): the silk fabric (1 g) was immersed in 50 mL acetate buffer (pH 5) containing 0.1 g dopa. The reaction was allowed to proceed at 50 °C for 2 h in a shaking bath. Then the fabrics were dried in air for 24 h. (ii) Two-step method (Fig. 1b): the silk fabric (1 g) was immersed in 50 mL acetate buffer (pH 5) containing 0.1 g dopa. The reaction was allowed to proceed at 50 °C for 2 h in a shaking bath. Then the fabrics were immersed in 50 mL acetate buffer (pH 5) containing 1.5 U mL−1 laccase. The reaction was allowed to proceed at 50 °C for 24 h in a shaking bath. (iii) One-step method (Fig. 1c): the silk fabric (1 g) was immersed in 50 mL acetate buffer (pH 5) containing 2 g L−1 dopa and 1.5 U mL−1 laccase. The fabrics were washed twice with acetate buffer and then with distilled water for 20 min. The control and laccase-treated samples were treated similarly as mentioned above.
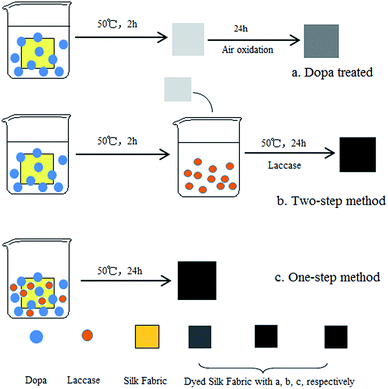 |
| Fig. 1 Schematic diagram of dyeing processes. | |
Color measurement
The color feature was tested using 650 Datacolor (Datacolor, USA). The range of wavelength was 360–740 nm.
Determination of levelness of coloration
The K/S values were measured using 650 Datacolor (Datacolor, USA) at eight different points on the dyed silk fabrics. The levelness of coloration in the fabrics was determined by calculating the mean K/S value and relative standard deviation Sr(λ). The smaller the deviation Sr(λ), the more is the evenness. |
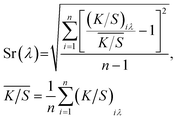 | (1) |
Color fastness test
The color fastness of the dyed fabrics was evaluated by the following test procedures: ISO 105-CO6:1998-color fastness to domestic and commercial washing (grey scale 1–5); ISO/DIS 105-X12:2001-color fastness to rubbing (grey scale 1–5).
UV-protection factor of colored silk fabric
Ultraviolet transmittance spectra were obtained using a UV-1000F Ultraviolet Transmittance Analyzer (Labsphere, USA). Each spectrum was an average of 10 measurements.
Binding force test
The silk fabric samples (1 g each) were treated with 25 mL DMF solution at 90 °C for 15 min. Then the fabrics were washed with distilled water for 10 min. The K/S values of the samples, before and after treatment, were determined.
SEM analysis
The silk fabric samples were scanned using a SU1510 scanning electron microscope (SEM, Hitachi, Japan) that operated at 5.00 kV and had a magnification of 1.00k.
AFM study
AFM is one of the most effective tools to examine the microstructure of the fiber. It can scan materials without any special preparation at normal temperature and pressure. AFM (CSPM 3300, Benyuan Company, China) was used in this study. The vertical resolution of the machine was 0.1 nm, while the horizontal resolution was 0.2 nm. The contact mode was chosen as the scanning mode in this study, and the size of the scan area was set as 5.0 μm × 5.0 μm.
FT-IR spectroscopy
The FT-IR spectroscopic analysis was carried out on a Nicolet iS10 FT-IR spectrometer (Thermo Fisher Scientific, USA). The spectra were recorded in the range of 4000–500 cm−1 at a resolution of 4 cm−1 and 16 scans per sample.
XPS analysis
The XPS analyses of silk fabrics were performed using a RBD upgraded PHI-5000C ESCA system (Perkin Elmer), with Mg Kα radiation (hν = 1253.6 eV). The X-ray anode was run at 250 W, and the high voltage was maintained at 14.0 kV with a detection angle of 54°. Both, the entire spectra (0–1100 eV) and the narrow spectra of carbon with significantly higher resolution were recorded using the RBD 147 interface (RBD Enterprises, USA) and the AugerScan 3.21 software. The binding energies were calibrated with the contaminant carbon (C1s = 284.5 eV). The spectral analysis including background subtraction, deconvolution, integration, and quantification was carried out using RBD AugerScan 3.21 software.
Results and discussion
Effects of process parameters on the dyeing of silk fabrics
The optimal reaction conditions for dyeing of silk fabric were determined in terms of the K/S values of the dyed fabrics. The effects of different process conditions on silk dyeing are shown in Table 1, Fig. 2.
Table 1 CIELAB coordinates of the dyed silk samples
Samples |
K/S |
L* |
a* |
b* |
c* |
h |
Untreated |
0.564 |
86.069 |
0.232 |
7.523 |
7.527 |
88.237 |
Dopa-treated |
7.517 |
63.270 |
7.965 |
24.562 |
25.685 |
74.641 |
Two-step method |
10.411 |
49.265 |
6.805 |
19.367 |
20.301 |
72.557 |
One-step method |
16.935 |
28.413 |
3.754 |
8.628 |
9.458 |
66.622 |
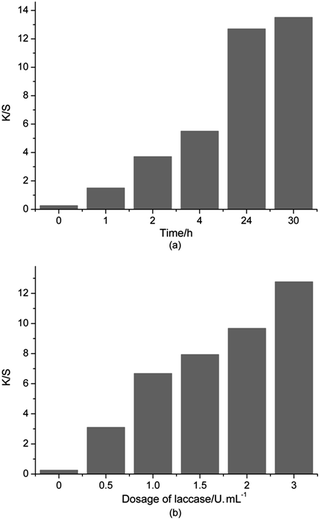 |
| Fig. 2 Effect of time (a) and laccase dosage (b) on the K/S values of silk fabrics. Conditions a: [dopa] = 2 g L−1, 1.5 U mL−1 laccase, 0–30 h at pH 5 and at 50 °C. Conditions b: [dopa] = 2 g L−1, 0–3 U mL−1 laccase, 24 h at pH 5 and at 50 °C. | |
Significant improvement in the K/S values of the silk samples was observed after enzymatic dyeing. The colorimetric results of the samples, dyed using different methods are summarized in Table 1.
The silk fabrics showed characteristic dark gray colors, due to fixation of the enzymatically polymerized dopa. As the table shows, the K/S value of the fabric, dyed using the two-step method, displayed higher color strength, compared to the dopa-treated fabric. This could be due to the fact that laccase played the role of an oxidation catalyst that accelerated the self-polymerization of dopa, as compared to air oxidation.
This also indicated that the measured K/S value of the fabric dyed using the one-step method displayed higher color strength, compared to the fabric dyed by the two-step method. One reason was that there was a difference in the order of adsorption and enzymatic polymerization of dopa in the dyeing process. These processes were simultaneous in the one-step method, whereas in the two-step method, dopa was first adsorbed on the silk fabric and then was oxidatively polymerized by laccase or air and was deposited on the surface of silk fabric.23 Another reason could be that dopa had less affinity for silk fibers compared to the oxidation products catalyzed by laccase, displaying lower K/S values.
The above experimental results showed that higher K/S values for silk fabrics could be obtained using the one-step method. Based on this, the effects of reaction time and amount of laccase on the one-step coloration process were further studied.
As shown in Fig. 2a, the K/S values of silk fabrics increased rapidly at first and then increased slowly after 24 h. Treatment for longer duration could improve the swelling of silk fibers that helped the silk surface to adsorb more color compounds. From these results, the optimal time for the coloration of silk fabrics with oxidized dopa was found to be 24 h.
Dosage of laccase is the most important factor that influences the enzymatic functions. Fig. 2b showed that the K/S values of silk fabrics gradually increased with an increase in the concentration of enzyme. The K/S value could barely be determined, when laccase was not used in the reaction. The increase of K/S value was determined at a laccase concentration of 0–1.0 U mL−1, due to the formation of a colored polymer and its deposition on the surface of the silk fabric. Furthermore, a slight increase in K/S value was observed when the laccase concentration was more than 1.0 U mL−1. This may be due to the saturation phenomenon during coloration. The above result implied that the adsorption of melanin on silk fabric was slightly affected by increase in the concentration of laccase above 1.0 U mL−1. Therefore, 1.0 U mL−1 was chosen as the lowest concentration of laccase required for coloration of silk fabrics for further dyeing experiments. These results showed that the rate of polymerization of dopa was consistent with the amount of laccase. A moderate increase of laccase dosage obviously speeded up the reaction rate.
Evaluation of levelness parameters
According to formula (1), the uniformities of the silk fabric dyed by the two-step and one-step methods were computed and their Sr(λ) values were 0.012532 and 0.018596, respectively. As lower values indicated better leveling property, the results showed the silk fabric colored by the two-step method had better color uniformity than the one colored by the one-step method. This could be due to the fact that dopa adhered evenly to the surface of the silk fabric initially and was then oxidized to form melanin particles in the two-step method. However, the processes of formation and adsorption of melanin in the one-step method were concurrent. As a result, many more melanin particles were generated and adsorbed onto the silk fabric, which prevented the even dyeing of the fabric.
Color fastness test
Table 2 shows the fastnesses of silk fabrics, dyed using the three different methods. The durability of the colored polymers attached to silk fabrics was tested in terms of washing, rubbing, and light fastnesses. The results showed that the samples had good dyeing fastness, especially light fastness. It further demonstrated that melanin formed by enzymatic oxidation, which had an affinity for silk fiber surface in acid solution, was acceptable for textile coloration.
Table 2 Washing, rubbing, and light fastnesses of samples dyed with dopa in the presence of laccase
Sample |
Staining level (rubbing fastness) |
Staining level (washing fastness) |
Fading level (light fastness) |
Dry |
Wet |
Fading |
Cotton staining |
Silk staining |
Dopa-treated |
4 |
3 |
4 |
4–5 |
5 |
4–5 |
Two-step |
4 |
3 |
4 |
4 |
4–5 |
5 |
One-step |
3–4 |
3 |
3–4 |
4 |
4–5 |
5 |
Nature of bonding between polyphenols and fibers
In order to identify the nature of bonding between the colored polymers and silk fibers, samples were treated with DMF and the K/S values were separately determined, before and after DMF treatment. The results are shown in Table 3. The K/S values of samples showed no significant differences before and after treatment. This proved that the color particles were bound to the silk fibers by not only hydrogen bonds and van der Waals forces, but also by covalent bonds. The possible reason could be the chemical reaction between the tyrosine residues of silk and dopa, which facilitated the stable coating of the oxidized derivatives of dopa with laccase on the surface of silk fabrics.
Table 3 K/S values (checksum: 360–700 nm) of the samples dyed using the two-step method and one-step method before and after DMF treatment
Samples |
K/S values |
Before DMF treatment |
After DMF treatment |
Dopa |
7.517 |
7.128 |
Two-step method |
10.411 |
10.146 |
One-step method |
16.935 |
16.286 |
Anti-ultraviolet properties
Improvements in UV-protection of fabrics have been a subject of great interest in textile industry.24 Fig. 3a shows ultraviolet resistance of untreated silk fabrics, and silk fabrics treated using two-step and one-step methods. In the range of 280–400 nm, the ultraviolet transmittance of uncolored silk fabric increased gradually, whereas the silk fabrics treated using the two-step and one-step methods showed lower values. Moreover, the UV transmittance of the silk fabric treated by the one-step method was much closer to zero than that treated by the two-step method. This proved that silk fabrics colored by dopa/laccase had excellent ultraviolet resistance. This could be explained by the fact that dopa was catalyzed and oxidized to form melanin by laccase, which was deposited on the silk fabric. The role of melanin in absorbing UV light and dissipating it as heat was extremely important. Uncolored silk fabric, silk fabrics colored using two-step and one-step methods showed different ultraviolet protection factors (UPF) of 12.48, 50+ and 100+ (Table 4). It was also evident that more melanin was deposited on the silk fabrics by the one-step method as compared to the two-step method.
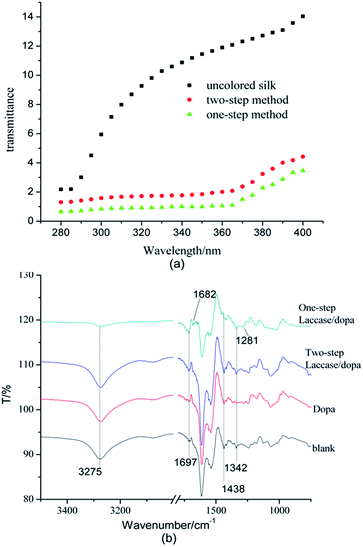 |
| Fig. 3 UV-protection (a), FT-IR spectra (b) of different silk samples. | |
Table 4 Parameters related to the UPF of silk fabric samples
Sample |
Transmittance/% |
UPF |
UVA |
UVB |
Untreated silk fabric |
11.61 |
5.21 |
12.48 |
Two-step method |
2.48 |
1.52 |
50+ |
One-step method |
1.59 |
0.79 |
100+ |
Surface morphology
Morphological changes in silk fabrics treated with dopa and dopa/laccase were studied by scanning electron microscopy, as shown in Fig. 4. The microscopic image of untreated sample was obtained at a magnification of 2000×, whereas the other treated samples were obtained at 1000× magnification. The surface of the untreated silk fiber was smoother compared to those of the treated silk fibers. A small number of solid particles were deposited on the surfaces of dopa treated silk fibers and silk fibers treated by the two-step method. This could be attributed to the affinity of dopa towards silk fibers. Furthermore, more particles were found adhered to the surfaces of silk fibers treated by one-step method.25 This suggested that more number of polymeric dye particles were formed in the one-step method and they were attached to the surfaces of the silk fibers. This result was consistent with the K/S results, mentioned above.
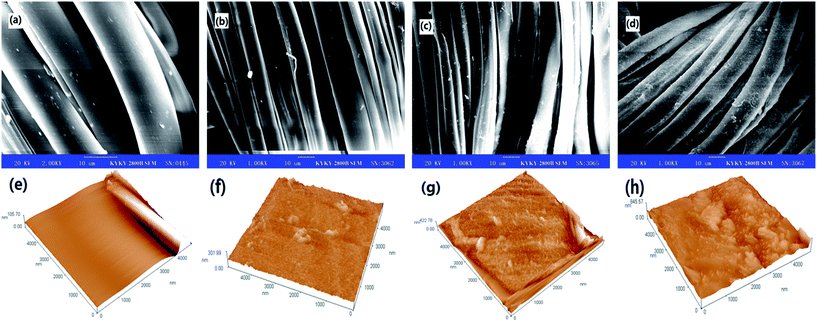 |
| Fig. 4 SEM and AFM images of control silk fabric (a and e) amplified at 2.00k, dopa-treated silk fabric (b and f), silk fabric treated with laccase/dopa by two-step method (c and g) and silk fabric treated with laccase/dopa by one-step method (d and h) amplified at 1.00k. | |
As shown in Fig. 4e, the untreated silk fibers appeared smooth and the height of the lines ranged from 0 to 105.7 nm. Aggregates were found adhering to the surfaces of the dopa-treated silk fabrics in Fig. 4f. From the AFM image, the sizes of aggregates were in the range of 0 to 301.99 nm. After the two-step treatment, the surface topography of the silk fibers with the aggregate sizes less than 422.78 nm, was close to that of the dopa-treated sample, as shown in Fig. 4g. Fig. 4h indicated that in the one-step treatment, more aggregates were deposited on the surface of silk fibers.26 It can be seen from Fig. 4h that almost the entire surface was covered with particles, which increased the height of the surface to 845.57 nm.
FT-IR of samples
The enzymatic coloration of silk fabrics by different methods was studied using FTIR spectroscopy. Fig. 3b shows that there was a huge difference in the peak at 3275 cm−1 between the blank and the sample treated using the one-step method. However, the differences in the 3275 cm−1 peak between untreated silk fabric and silk fabrics treated with dopa and by the two-step method were subtle. The changes in this peak could be attributed to the changes in the number of hydroxyl and amino groups present on the surface of the silk fabrics. Since the numbers of melanin granules formed and deposited on the silk fabrics by the one-step method were more, this sample showed weaker absorption peaks of the hydroxyl group, compared to the samples treated with dopa and by the two-step method. In contrast to this, the absorption peaks at 1697 cm−1, assigned to C
O, particularly the aryl ketones, gradually increased.27,28 The reason was that dopa was catalytically oxidized by laccase and polymerized to form melanin, which contained many carbonyl groups. Moreover, the absorption peaks of C
O in the sample treated by the one-step method shifted to lower wavenumbers, due to the formation of a conjugated structure. The absorption peaks at 1438 cm−1, 1342 cm−1, and 1281 cm−1 could be attributed to the C–H asymmetric vibrations, symmetric umbrella like vibrations, and rocking modes, respectively.29,30 The FT-IR results indicated that melanin was made from dopa through laccase catalysis and was deposited on the surface of silk fabric.
XPS analysis
Besides FT-IR spectroscopic analysis, XPS analysis was carried out to further understand the changes occurring on the surface of silk fabric, after the laccase/dopa treatment. The deconvolution of the C1s high-resolution signals in the XPS spectra was carried out by curve fitting to determine the bonding states of carbon on the silk surface.
The proportions and survey spectra of control and laccase/dopa-treated silk fabric are shown in Fig. 5a and b. The carbon content on the silk surface decreased from 77.26% to 72.86% and the oxygen content decreased from 18.87% to 8.00% after laccase treatment. An increase in nitrogen content from 3.87% (the control silk) to 19.14% (the laccase/dopa-treated silk) was evident, which could be attributed to the adhesion between silk surface and melanin. Furthermore, the carbon, nitrogen, and oxygen contents of laccase/dopa-treated silk fabric were 72.86%, 19.14%, and 8.00%, respectively. These contents were consistent with the molecular structure of melanin (Scheme 1b).
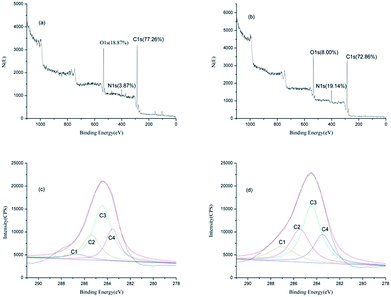 |
| Fig. 5 XPS survey spectra and deconvoluted C1s spectra of control (a and c) and laccase/dopa-treated (b and d) silk fabric. | |
Deconvolution of the C1s spectrum resulted in four peaks, C1, C2, C3 and C4, as shown in Fig. 5c and d. The C4 peak with the lowest binding energy, represented the carbon atom linked only to carbon or hydrogen atoms (–C–C).31,32 The C3 peak represented a carbon atom bound to a non-carbonyl oxygen atom (–C–O). The C2 peak represented a carbon atom bound to a nitrogen atom (–C–N). The C1 peak with the highest binding energy represented a carbon atom bound to one carbonyl oxygen atom (–C
O) or two non-carbonyl oxygen atoms (O–C–O).33,34
The C1 peak intensity on the silk surface increased after laccase treatment, since the attached melanin, on the surface of silk, was rich in –C
O groups. An increase in the C2 peak intensity after the laccase treatment was evident, which could be attributed to settling of melanin on the silk surface, leading to an increase in the number of –C–N groups. The changes in the proportions of C3 and C4 in the C1s spectra were very subtle.
Conclusions
This study demonstrated the grafting of dopa onto silk surface, mediated by laccase. The silk fabrics obtained black coloration and also excellent anti-UV properties after the two-step or one-step laccase/dopa treatments. The silk fabrics treated with laccase/dopa by one-step method showed K/S value of 16.935, light fastness of 5 level, and UPF of 100+, whereas the values for silk fabrics treated using the two-step process were K/S value of 10.411, light fastness of 5 level, and UPF of 50+ respectively. SEM showed numerous small flakes on the surface of the laccase/dopa-treated silk samples, which could be considered as melanin particles from dopa molecules, attached as a result of grafting reaction. AFM observations indicated that the particles gathered to form an even granular bed on the surface of the silk fibers. FT-IR analysis showed that the functional groups on the surfaces of laccase/dopa-treated silk fabrics changed, due to the introduction of carbonyl groups. XPS results showed that the contents of the elements on the surface of laccase/dopa-treated silk fabrics were similar to oral melanin. Consequently, dopa was oxidized by laccase to form dopa-melanin and was grafted onto the surfaces of silk fabrics. In conclusion, this eco-friendly enzymatic process provides an attractive alternative to the current methods for dyeing and functionalization of textiles.
Acknowledgements
This work was financially supported by National Natural Science Foundation of China (21274055, 51673087) and Program for Changjiang Scholars and Innovative Research Team in University (IRT_15R26) and Fundamental Research Funds for the Central Universities (JUSRP51717A).
Notes and references
- M. O. Bulut and E. Akar, J. Cleaner Prod., 2012, 32, 1–9 CrossRef CAS.
- F. Mahmoud and N. Hossein, Fibers Polym., 2014, 15, 2504–2514 CrossRef.
- H. D. Zheng, J. Zhang, J. Yan and L. J. Zheng, J CO2 UTil, 2016, 16, 272–281 CrossRef CAS.
- S. A. Larik, A. Khatri, S. Ali and S. H. Kim, Ultrason. Sonochem., 2015, 24, 178–183 CrossRef CAS PubMed.
- A. Ozan, C. Ali, G. Gorkem and Y. Arzu, Fibers Polym., 2013, 14, 866–873 CrossRef.
- L. He, V. LaiLaiSo and J. H. Xin, RSC Adv., 2014, 4, 20317–20322 RSC.
- V. LaiLaiSo, L. He and J. H. Xin, RSC Adv., 2014, 4, 41081–41086 RSC.
- J. W. Di, Y. F. Tu and F. Zhang, Spectrosc Spect Anal, 2004, 24, 835–837 Search PubMed.
- P. Jolanta, J. W. Anna, S. C. Agnieszka, W. Kamila, K. Magdalena, S. L. Jadwiga and L. O. Joanna, J. Cleaner Prod., 2016, 112, 4265–4272 CrossRef.
- R. Mustafa, L. Muniglia, B. Rovel and M. Girardin, Food Res. Int., 2005, 38, 995–1001 CrossRef CAS.
- L. F. Liu, L. D. Cheng, L. Q. Huang and J. Y. Yu, Fibers Polym., 2012, 13, 600–605 CrossRef CAS.
- S. Kim, C. López, G. Güebitz and A. Cavaco-Paulo, Eng. Life Sci., 2008, 8, 324–330 CrossRef CAS.
- C. D. Blanco, M. D. González, J. M. D. Monmany and T. Tzanov, Enzyme Microb. Technol., 2009, 44, 380–385 CrossRef CAS.
- Z. Y. Zhao, J. Liu, M. Hahn, S. Z. Qiao, A. P. J. Middelberg and L. Z. He, RSC Adv., 2013, 3, 22008–22013 RSC.
- G. M. Robinson and M. R. Smyth, Analyst, 1997, 122, 797–802 RSC.
- C. Blarxino, L. Mosca, C. Foppoli, R. Coccia, C. D. Marco and M. A. Rosei, Free Radicals Biol. Med., 1999, 26, 446–453 CrossRef.
- Z. L. Hegedus, Toxicology, 2000, 145, 85–101 CrossRef CAS PubMed.
- P. A. Riley, J. Biochem. Cell Biol., 1997, 29, 1235–1239 CrossRef CAS.
- Y. X. Tan, F. Zhang and J. W. Di, Chin. J. Spectrosc. Lab., 2002, 19, 585–588 CAS.
- J. W. Di and S. P. Bi, Spectrosc Spect Anal, 2005, 25, 84–86 Search PubMed.
- Y. Boyjoo, M. M. Wang, V. K. Pareek, J. Liu and M. Jaroniec, Chem. Soc. Rev., 2016, 45, 6013–6047 RSC.
- L. Jiang, Shengwuxue Tongbao, 1993, 28, 20–25 Search PubMed.
- L. Q. Zhang, K. Qian, X. P. Wang, F. Zhang, X. Shi, Y. J. Jiang, S. M. Liu, M. Jaroniec and J. Liu, Adv. Sci., 2016, 3, 1500363 CrossRef PubMed.
- T. T. Liu, L. L. Qu, K. Qian, J. Liu, Q. Zhang, L. H. Liu and S. M. Liu, Chem. Commun., 2016, 52, 1709–1712 RSC.
- S. Kim, C. López, G. Güebitz and A. Cavaco-Paulo, Eng. Life Sci., 2008, 8, 324–330 CrossRef CAS.
- R. Wang and Y. Q. Wang, Procedia Eng., 2011, 18, 307–311 CrossRef CAS.
- F. L. Huang, Q. F. Wei, X. Q. Wang and W. Z. Xu, Polym. Test., 2006, 25, 22–27 CrossRef CAS.
- V. Alessio, A. Annalisa, T. Claudio and F. Franco, Fibers Polym., 2006, 7, 105–111 CrossRef.
- M. Karabacak, D. Karagoz and M. Kurt, J. Mol. Struct., 2008, 892, 25–31 CrossRef CAS.
- A. B. Sheeraz and A. Shabbir, J. Mol. Struct., 2016, 1105, 169–177 CrossRef.
- M. Karabacak, L. Sinha, O. Prasad, A. M. Asiri, M. Cinar and V. K. Shukla, Spectrochim. Acta, Part A, 2014, 123, 352–362 CrossRef CAS PubMed.
- H. Tian, M. Saunders, A. Dodd, K. O'Donnell, M. Jaroniec, S. M. Liu and J. Liu, J. Mater. Chem. A, 2016, 4, 3721–3727 CAS.
- P. Nzokou and D. P. Kamdem, Surf. Interface Anal., 2005, 37, 689–694 CrossRef CAS.
- A. X. Dong, X. R. Fan, Q. Wang, Y. Y. Yu and A. Cavaco-Paulo, Int. J. Biol. Macromol., 2015, 79, 353–359 CrossRef CAS PubMed.
|
This journal is © The Royal Society of Chemistry 2017 |
Click here to see how this site uses Cookies. View our privacy policy here.