DOI:
10.1039/C6RA24704K
(Paper)
RSC Adv., 2017,
7, 2460-2465
A multifunctional Schiff base fluorescence sensor for Hg2+, Cu2+ and Co2+ ions†
Received
4th October 2016
, Accepted 30th November 2016
First published on 12th January 2017
Abstract
A multifunctional Schiff base fluorescence sensor (receptor L) was prepared and its metal ion sensing properties were investigated. Receptor L exhibited fluorescence and colorimetric responses toward Hg2+, Cu2+ and Co2+ with different color changes. Moreover, receptor L can be used for selective sensing of Hg2+, Cu2+ and Co2+ based on the distinct color changes in the real sample.
1. Introduction
Development of new receptors for the detection of different analytes simultaneously is emerging as an area of great interest,1–4 since such systems would lead to faster analytical processing and potential cost reductions. However most of the reported sensors are effective in selective recognition of only a particular analyte.5–11 Since the recognition units of the sensors are distinctive in sensing cation or anion behavior, thus, developing such sensors with multiple analyte recognition capability is a challenging task. In addition, multifunctional sensors that produce different fluorescence or color responses to different metal ions will have more potential in environmental applications. As a result, intense researches have been focused on the development of sensitive and selective receptors for the qualitative and quantitative recognition of multi metal ions. Interestingly, various sensors have been reported are quite specific, either for Cu2+ and Hg2+,12 or for Cu2+ and Co2+,13 or for Cu2+ and Zn2+ (ref. 14) or for Fe3+, Co2+ and Cu2+,15 but to the best of our knowledge, only a few fluorescent sensors for the simultaneous detection of Hg2+, Cu2+ and Co2+ was reported.16
Among various important analytes, Hg2+, Cu2+ and Co2+ are all significant environmental pollutants and also play a critical role in various biological processes. The accumulation of excess amounts of copper ions or their misregulation can cause a series of severe diseases such as Alzheimer's and Parkinson's diseases.17 In the marine environment, Hg2+ ion is converted by bacteria into toxic methylmercury, a highly potent neurotoxin, and is passed up the food chain to accumulate in humans.18 On the other hand, cobalt is another biologically important metal and is required by organisms as a micronutrient. At low content of cobalt is part of vitamin B12, which is one of the necessities of the body healthy. Accumulation of high levels of cobalt in humans results lung damage and heart disease.19,20 Due to the importance of cobalt, sensors are being developed for its detection, but only a few have been reported.
Receptor L has been reported by Wenhui Ma et al. recently;21 however, its metal ion-sensing properties have not yet been studied. Herein, we reported its application as a colorimetric and fluorescent sensor for selective sensing of Hg2+, Cu2+ and Co2+. Receptor L exhibits high fluorescence sensitivity and selectivity toward Hg2+ among a series of ions and show the naked-eyes detection toward Cu2+ and Co2+.
2. Materials and instrumental methods
2.1. Experimental section
All reagents were obtained from commercial suppliers and were used without further purification. Analytical thin-layer chromatography was performed using silica gel 60 F254 plates (Merck). The 1H NMR spectra were recorded with a Bruker AM 300 spectrometer. Chemical shifts are given in ppm with residual DMSO as reference. Mass spectra were recorded under electron impact (EI) or electron spray interface (ESI) conditions. UV-vis spectra were recorded by using Jasco V630 spectrophotometer with a diode array detector, and the resolution was set at 1 nm. Fluorescence spectra were recorded on a Jasco FP-8300 fluorescence spectrophotometer.
3. Results and discussion
3.1. The absorption and fluorescence studies of receptor L toward various metal ions
UV-vis and fluorescence spectra of receptor L were investigated in DMSO–H2O (9
:
1; v/v) by the presence of various metal ions (as perchlorate salts): Li+, Na+, K+, Ca2+, Mn2+, Hg2+, Fe2+, Fe3+, Co2+, Ni2+, Cu2+, Pb2+, Cd2+, Zn2+ and Al3+. Upon addition of Hg2+, Cu2+ and Co2+ ions to the solution of receptor L, a prominent change was observed in UV-vis absorption spectra and fluorescence spectra, as shown in Fig. 1 and 2, respectively. In the presence of Hg2+, the absorption spectra of receptor L showed a major band at 445 nm with a blue shift. In the presence of Cu2+ and Co2+, the absorption spectra of receptor L showed a major band at 481 nm and 499 nm with a red shift, respectively (Fig. 1). As shown in Fig. 2, upon addition of Cu2+ and Co2+ to the solution of receptor L, the fluorescence intensity of receptor L was strongly quenched. The solution of receptor L with Cu2+ showed a dramatic color change from yellow to orange. On the other hand, the solution of receptor L with Co2+ showed a dramatic color change from yellow to red which could easily be detected by the naked-eye, as shown in Fig. 3 and 4.
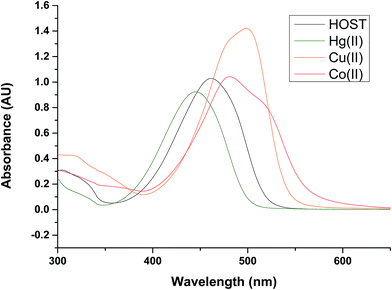 |
| Fig. 1 UV/vis spectra of receptor L (20 μM) recorded in H2O/DMSO = 1/9 (v/v) after addition of 5 equiv of Hg, Co, Cu Metal ion. | |
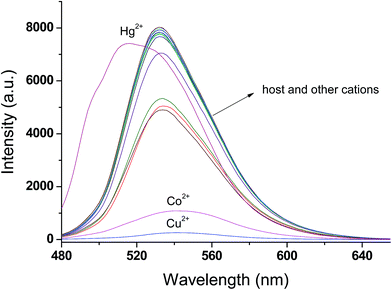 |
| Fig. 2 Fluorescence emission spectra (λex. = 460 nm) of receptor L (20 μM) in the presence of 5 equiv. of various cation in H2O/DMSO = 1/9 (v/v). | |
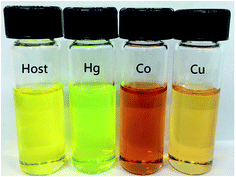 |
| Fig. 3 The color changes observed by naked eye of receptor (20 μM) upon addition of 5 equiv. of Hg2+, Co2+, Cu2+ in H2O/DMSO = 1/9 (v/v). | |
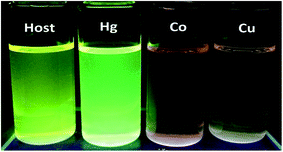 |
| Fig. 4 The color changes observed by UV light of receptor (20 μM) upon addition of 5 equiv. of Hg2+, Co2+ and Cu2+ in H2O/DMSO = 1/9 (v/v), respectively. | |
3.2. Fluorescence titration and binding studies
From the fluorescence titration profiles (Fig. 5), upon the addition of Hg2+, the fluorescence intensity of receptor L decreased at first and then increased. At a low concentration of Hg2+ (4–12 μM), the coordination of Hg2+ induced the reduction of the emission intensity. Along with the addition of Hg2+ (16–40 μM), the fluorescent emission band was gradually enhanced and simultaneously, was blue-shifted to appear at 514 nm wavelength. The result indicates a new species formation. Upon the more addition of Hg2+ (60–800 μM), the fluorescent emission band was gradually decreased. Meanwhile, the solution of receptor L showed a dramatic color change from yellow to light green which could easily be detected by the naked-eye (Fig. 3). The inconsistent fluorescence spectra with Hg2+ have suggested about the possibility of Hg2+-induced irreversible desulphurization chemical reaction leading to formation of urea derivative (X) by a complexation reaction with sulfur atom of thiourea subunit in the early stages. The structure of urea derivative (X) is similar with receptor L, therefore, the urea derivative (X) can also recognize Hg2+ leading to fluorescence quenching. Every mercury ion could generate the effective coordination with excessive receptor L at the low concentration of Hg2+. Along with the addition of a concentration, Hg2+ displays two functions; one is coordination role and the other is desulphurization. Therefore, we proposed a possible mechanism that the coordination and desulphurization of Hg2+, as shown in Scheme 1.
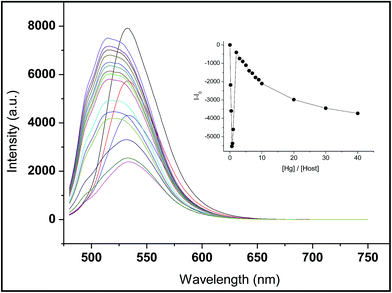 |
| Fig. 5 Fluorescence spectra of receptor L (20 μM) in H2O/DMSO = 1/9 (v/v) upon addition of increasing concentrations Hg2+ (0–800 μM). | |
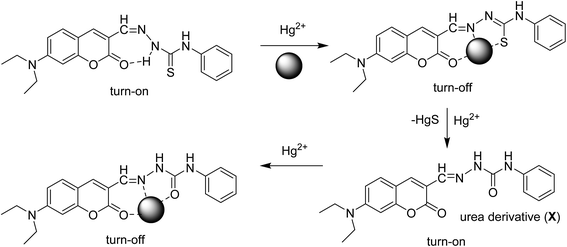 |
| Scheme 1 The proposed reaction for receptor L with Hg2+, Cu2+ and Co2+. | |
On the other hand, with the addition of increasing amounts of Cu2+ or Co2+ to a solution of receptor L, the maximum peak at 540 nm decreased gradually, as shown in Fig. S1 and S2,† respectively. There is obviously an intramolecular hydrogen bond present between carbonyl group and amine in the receptor L. The presence of Cu2+ or Co2+ might destroy the intramolecular hydrogen bond and led to fluorescence quenching. The possible mechanism is shown in Scheme 2. These results indicated that receptor L can be used for selective sensing of Hg2+, Cu2+ and Co2+ based on the distinct color changes in environmental analysis.
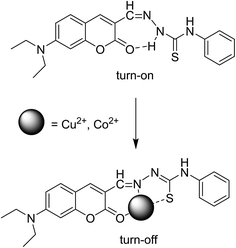 |
| Scheme 2 The proposed mechanism for receptor L with Cu2+ and Co2+. | |
From the fluorescence titration profiles, the association constants for receptor L–Hg2+, L–Cu2+ and L–Co2+ were determined as 1.85 × 104 M−1, 5.69 × 105 M−1 and 3.52 × 106 M−1, respectively, by the Stern–Volmer plot (Fig. S3 and S4†). A Job plot indicated a 1
:
1 stoichiometric complexation of receptor L with Hg2+, Cu2+ and Co2+ ions (Fig. S5–S7†). In addition, the formation of 1
:
1 complex between receptor L and Hg2+ was further confirmed by the appearance of a peak at m/z 596, assignable to [receptor L + Hg2+] (Fig. S8†). Similarly, the peak at m/z 458 and m/z 453 were assignable to [receptor L + Cu2+] (Fig. S9†) and [receptor L + Co2+] (Fig. S10†), respectively. The detection limit of receptor L for the analysis of Hg2+ ion was determined as 0.20 ppb, for Cu2+ ion as 0.29 ppb and for Co2+ it was determined as 0.007 ppb.
3.3. Application in real samples
The practical application of receptor L for selective sensing of Hg2+ in different source of water such as DI, tap, drink, ditch, lake and ground water has also been demonstrated. Hg2+, Cu2+ and Co2+ were dissolved in different source of water, respectively.
Addition of receptor L into each sample water with Hg2+ clearly showed changing color from bright yellow to bright green under UV light (Fig. 6). Addition of receptor L into each sample water with Cu2+ or Co2+ clearly showed changing color from bright yellow to orange and red by the naked-eye detection, respectively (Fig. 7). These results indicated that receptor L can be used for selective sensing of Hg2+, Cu2+ and Co2+ based on the distinct color changes in the real sample.
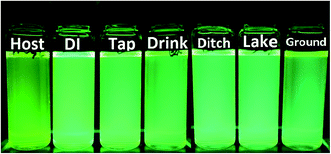 |
| Fig. 6 The color changes observed by UV light of receptor L upon addition of 5 equiv. of Hg2+ in different source of water. | |
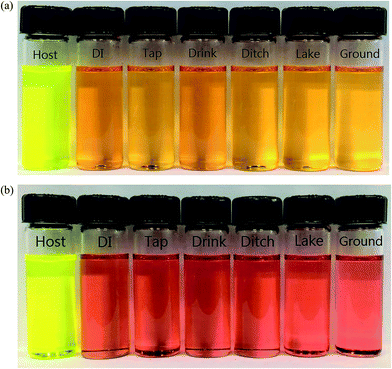 |
| Fig. 7 The color changes observed by naked eye in different source of water containing (a) Cu2+ (top) (b) Co2+ (bottom) respectively. | |
On the other hand, the indicator paper experiments were done using filter paper coated with the solution of receptor L. When this coated test paper was dropped in Hg2+, Cu2+ and Co2+ water solution, the color of the paper was changed from yellow to brown instantly under UV light, as shown in Fig. 8. This indicated that the change in color on the test paper was caused by the interaction of receptor L and Hg2+, Cu2+ and Co2+, implying that the visually test paper showed specific recognition to Hg2+, Cu2+ and Co2+.
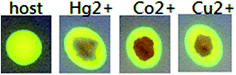 |
| Fig. 8 Photographs of test paper for detecting Hg2+, Cu2+ and Co2+ in water. | |
3.4. Reversible and competition experiments
Reversibility is a prerequisite in developing novel sensor for practical application. To examine the reversibility of receptor L toward Hg2+ in DMSO–H2O (9
:
1; v/v) solution of EDTA (20 μM), was added to the complex solution of receptor L and Hg2+. As expected, Hg2+-induced irreversible desulphurization chemical reaction leading to formation of urea/amide derivative (2), therefore fluorescence signal at 420 nm was not quenched (Fig. S11†). On the other hand, when solution of EDTA (40 μM) was added to the complex solution of receptor L and Cu2+, as expected, a fluorescence signal at 442 nm was completely quenched, which indicated the regeneration of the free receptor L (Fig. S12†). However, when the same experiment was performed for receptor L and Co2+, it showed irreversible character (Fig. S13†). This result indicates due to high binding constant of complex L–Co2+, cobalt ions are less likely to be replaced by EDTA.
The selectivity toward Hg2+, Cu2+ and Co2+ was further ascertained by the competition experiment. As shown in Fig. S14–S16,† receptor L was treated with Hg2+ or Cu2+ or Co2+ in the presence of other metal ions of the same concentration. Relatively low interference was observed for the detection of Hg2+ and Co2+ in the presence of other ions. For the detection of Cu2+, receptor L shows good selectivity in the presence of most metal ions, whereas quenching the fluorescence was observed in presence of Hg2+. The quenching is due to the displacement of Cu2+ by Hg2+ from receptor L–Cu2+. Although the Hg2+ replacement part of Cu2+ but still does not affect the detection of Cu2+. Thus receptor L can be used as a selective fluorescent sensor toward Hg2+, Cu2+ or Co2+ in the presence of most competing ions.
3.5. 1H-NMR titration experiments
To better understand the complexation behavior of receptor L with Hg2+ and Co2+, 1H NMR experiments were carried out in DMSO. The spectral differences for Co2+ and Hg2+ are depicted in Fig. 9 and 10, respectively. Upon addition of Co2+, no significant change was observed. Upon addition of 0.5 eq. Hg2+, dual peaks of quinoline ring and ethyl amines were appeared. These observations obviously indicated the presence of two isomers of receptor L. Upon the addition of 1.0 equiv. of Hg2+, the imine proton at 8.2 ppm was shifted upfield toward 8.0 ppm. The proton of quinoline ring at 8.7 ppm was shifted upfield toward 8.6 ppm. Meanwhile, most of the aromatic protons displayed downfield shift compared to those of receptor L alone. The result indicated that Hg2+-induced irreversible desulphurization chemical reaction leading to formation of urea/amide derivative (2).
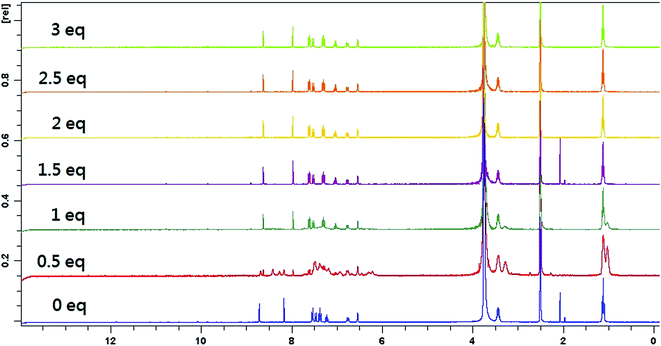 |
| Fig. 9 1H NMR titration plots of receptor L with Hg2+ in DMSO/D2O (9 : 1, v/v). | |
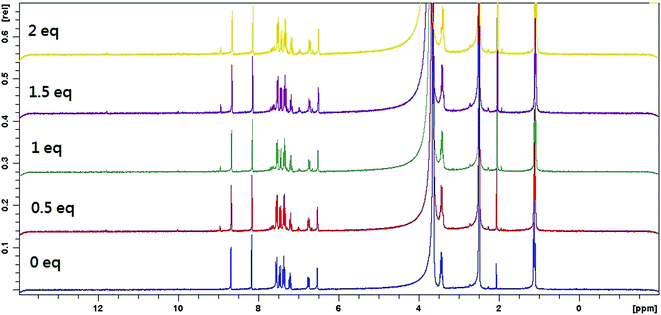 |
| Fig. 10 1H NMR titration plots of receptor L with Co2+ in DMSO/D2O (9 : 1, v/v). | |
In order to elucidate the formation of the L–Hg2+, we also performed the IR experiments of receptor L and receptor L–Hg2+ complex. The typical IR spectra are shown in Fig. S17.† The IR spectrum of the receptor L indicated a sharp peak at 1251 cm−1, which was assigned to C
S stretching. In addition, receptor L displayed a C
O stretching band in the 1715 cm−1 region. Interaction of L with Hg2+, the C
S stretching band was disappear, along with a shift in the N–H stretching vibration from 3475 cm−1 to 3500 cm−1 can be assigned to the formation of amide derivative as a consequence of Hg2+ ion induced irreversible desulphurization chemical reaction.
4. Conclusion
In summary, we have successfully designed and synthesized a simple, fluorescent and colorimetric receptor L, capable of recognizing Hg2+, Cu2+ and Co2+. Receptor L exhibited an excellent selectivity and sensitivity towards Hg2+ fluorescent intensity enhancement with blue shift. In addition, receptor L also showed a good selectivity towards Cu2+ and Co2+ by inducing a rapid color change from yellow to orange and red, respectively.
Acknowledgements
Ministry of Science and Technology, R. O. C. (grant numbers: 104-2113-M-018-002).
References
- A. Liu, L. Yang, Z. Zhang, Z. Zhang and D. Xu, Dyes Pigm., 2013, 99, 472–479 CrossRef CAS.
- Q. Zeng, P. Cai, Z. Li, J. Qin and B. Z. Tang, Chem. Commun., 2008, 1094–1096 RSC.
- V. K. Muthu, B. C. Denzil, S. Gandhi, S. Karuppannan and S. Subramanian, RSC Adv., 2015, 5, 94903–94908 RSC.
- S. Gandhi, A. Thangaraj and C. Duraisamy, Anal. Methods, 2014, 6, 2343–2348 RSC.
- S. Gandhi, V. Balasubramanian and C. Duraisamy, RSC Adv., 2014, 4, 30828–30831 RSC.
- S. Omprakash, K. Niranjan, G. M. Balaji, S. Subramanian and S. Gandhi, RSC Adv., 2016, 6, 656–660 RSC.
- H. Joydev, P. Suman and B. Subhajit, Tetrahedron Lett., 2014, 53, 4357–4360 Search PubMed.
- S. Gandhi, A. Thangaraj and C. Duraisamy, Analyst, 2012, 137, 5881–5884 RSC.
- A. Thangaraj, S. Gandhi, A. Palpandi, C. Duraisamy and G. Subbaiah, Tetrahedron Lett., 2014, 55, 671–675 CrossRef.
- A. Thangaraj, S. Gandh and C. Duraisamy, J. Photochem. Photobiol., A, 2014, 281, 47–52 CrossRef.
- C. Arivazhagan, B. Rosmita, R. Jagan and G. Sundargopal, Dalton Trans., 2016, 45, 5014–5020 RSC.
- L. Wang, J. X. Yan, W. Qin, W. Liu and R. Wang, Dyes Pigm., 2012, 92, 1083–1090 CrossRef CAS.
- L. Ding, S. Wang, Y. Liu, J. Cao and Y. Fang, J. Mater. Chem. A, 2013, 1, 8866–8875 CAS.
- S. Wang, G. Men, L. Zhao, Q. Hou and S. Jiang, Sens. Actuators, B, 2010, 145, 826–831 CrossRef CAS.
- K. D. Bhatt, H. S. Gupte, B. A. Makwana, D. J. Vyas, D. Maity and V. K. Jain, J. Fluoresc., 2012, 22, 1493–1500 CrossRef CAS PubMed.
- M. Saleem, C. H. Khang, M.-H. Kim and K. H. Lee, J. Fluoresc., 2016, 26, 11–22 CrossRef CAS PubMed.
- H. H. Wang, L. Xue, Z. J. Fang, G. P. Li and H. Jiang, New J. Chem., 2010, 34, 1239–1242 RSC.
- P. Grandjean, P. Weihe, R. F. White and F. Debes, Environ. Res., 1998, 77, 165–172 CrossRef CAS PubMed.
- E. Patel, C. Lynch, V. Ruff and M. Reynolds, Toxicol. Appl. Pharmacol., 2012, 258, 367–375 CrossRef CAS PubMed.
- D. C. Barceloux, Clin. Toxicol., 1999, 37, 201–208 CAS.
- W. Ma, W. Xia, Q. Xu, H. Han, B. Song, L. Sun and C. Liang, Acta Chim. Sin., 2012, 70, 917–920 CrossRef CAS.
Footnote |
† Electronic supplementary information (ESI) available. See DOI: 10.1039/c6ra24704k |
|
This journal is © The Royal Society of Chemistry 2017 |