DOI:
10.1039/C7QM00144D
(Chemistry Frontiers)
Mater. Chem. Front., 2017,
1, 1304-1309
The ‘folklore’ and reality of reticular chemistry
Received
29th March 2017
, Accepted 24th April 2017
First published on 5th May 2017
Abstract
Reticular chemistry, linking molecular building blocks together by strong bonds to make porous frameworks, is a rapidly expanding field of research, engaging laboratories worldwide. However, as with any emerging field, there exist ‘folklores’ permeating through the scientific discourse. These folklores do little in the way of advancing the field and, in no uncertain terms, negatively color our scientific pursuits. It is within this context that we seek to bring the folklores out into the open to provide the true realities of reticular chemistry.
In this special issue, we wish to share our views about the current state and future direction of reticular chemistry, which is the chemistry of linking molecular building blocks by strong bonds into crystalline extended structures, such as metal–organic frameworks (MOFs) and covalent organic frameworks (COFs)1–4 Since the measurement of the first gas adsorption isotherm for MOFs,5 the scope of their chemistry and applications has expanded to the point that this field has become one of the most actively researched in the chemical and materials sciences.6 Whether it is constructing the MOF/COF backbone or functionalizing the pores, reticular chemistry is practically becoming ‘covalent chemistry beyond molecules’.7,8 We use the term ‘folklore’ as it largely refers to the passing of traditional knowledge and practices primarily through oral communications among people who belong to the same community, tribe, or culture. Folklore is a common feature of art, literature, philosophy, religion, and mythology, yet it is, at least on the surface, avoided in science. This is understandable as science is based on observations and experiments to uncover the facts. However, in a sense, the field of reticular chemistry is not very much unlike other expanding fields of science in that folklore has found its way into the larger scientific discourse of the field. We must point out the fact that the main purpose of folklore is for entertainment as opposed to advancing scientific thought. Our concern is when folklore, even if it is in small part, affects reality and, therefore, the course of our experiments and scientific pursuits. This concern is the reason we choose to discuss the subject of folklore and reality in reticular chemistry. Many practitioners of this chemistry are bringing out its reality in their contributions, in spectacular ways not the least of which by combining the beauty of reticular structures with their substance and significance. This makes it all the more important to discuss the folklore and reality, such that emerging scholars in the field receive the message accurately and engage in productive scientific activities. There is a lot at stake for us to get it right!
Herein, we provide and detail three folklores that emerging scholars may encounter about MOFs/COFs, especially in institutions where MOF/COF chemistry is nascent. Although these folklores are beginning to subside (thankfully), we still believe it is useful to bring them out into the open and address them with the true reality. The importance of this discussion will allow students and researchers from around the world to have the freedom to imagine and create without the unnecessary distractions and corrosive indulgence in folklore.9
Folklore #1: “Applications are necessary for publishing MOF structures”
We know that for many scientific innovations, the applications were realized through adopting a basic science-approach towards understanding materials’ fundamental properties. Basic science and applications are central for making a meaningful contribution and building a field of research, as well as for turning that field into a scholarly discipline of teaching and learning. Without basic science, the engine of innovations will come to a halt bringing thriving technologies and knowledge-based economies to stagnation.
When a new MOF structure is made, it is incumbent upon the researcher to think deeply about what it is that he/she just made: examine the structure in detail, describe its chemical make-up and connectivity, and study it as if it were a new species. The beauty is in unveiling what the structure is ‘trying to tell us’. In other words, nature has revealed itself to us through that structure and provided us with the opportunity to recognize new facts about chemistry. If our thorough analysis and comprehensive understanding of the structure is performed in a setting absent of the confines of placing it within the current trend of applications, the likelihood of uncovering something unique is remarkably high (Fig. 1). This is the reality of reticular chemistry and, at present, remains a source of great inspiration in the literature.10–24 Furthermore, it is an aspect that leads us to understand our molecular creations more deeply, which inevitably moves us towards applications rather than away from them. Let us not forget that one of our most important products is to use research as a tool to elevate the minds of students and to teach them how to solve challenging problems and, in the meantime, discover the subtleties of how failure and success are intertwined.
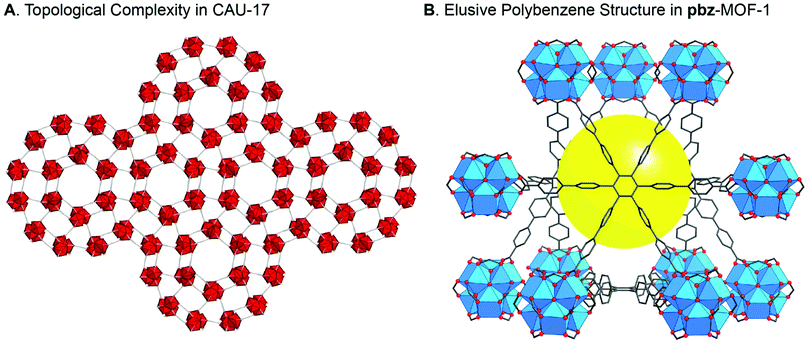 |
| Fig. 1 Reticular chemistry is based on a deep understanding of the unique characteristics of a new MOF structure, thus dispelling the folklore that “applications are needed to publish.” (A) Crystal structure of CAU-17 with one of the most complicated nets ever reported for a MOF structure.11 (B) The polybenzene or “cubic graphite” structure was proposed over 70 years ago, but only with the synthesis of pbz-MOF-1 was it realized.13 Atom colors: C, grey; O, red; Bi, red polyhedra; and Zr, blue polyhedra. H atoms are omitted for clarity and the yellow ball in pbz-MOF-1 represents the free space. | |
It goes without saying that each MOF/COF we discover is important. This is because by combining organic and inorganic units to make MOF crystals, we have combined into one field, two fields of study, organic and inorganic, which for many years were considered and taught as separate disciplines. Now, with MOFs/COFs, the chemistries of both fields are exploited with exceptional results. In the case of COFs, organic materials can be readily made as 2D and 3D crystalline, covalent frameworks, thereby extending organic chemistry beyond simple molecules and polymers.25 The resulting new MOF/COF materials have structures and properties unmatched by their constituent molecular building blocks. Thus, when we synthesize a new structure, we must develop its chemistry just like when an inorganic or organic chemist makes a new metal complex or molecule.
A final point to consider is that reticular chemistry as a discipline provides the intellectual framework for making completely new materials, such as MOFs, COFs, ZIFs (zeolitic imidazolate frameworks),26,27 CATs (metal-catecholate frameworks),28 woven frameworks,29 among others, and we expect many more to come. Researchers working on these materials seamlessly cross between molecular organic and inorganic, solid-state, topology, geometry and, more recently, engineering and biology disciplines. This is unprecedented in chemistry! Presently, one still finds that mainstream inorganic or organic textbooks taught in classrooms have very little (if any) solid-state chemistry and rarely discuss the molecular aspects of materials chemistry. Reticular chemistry offers a whole new world of beauty, relevance and impact, where the precision of molecular chemistry is the thread connecting basic science, properties, and applications of these new materials. Therefore, at this stage in the development of the field, the basic science is so intellectually rich that it can stand alone, and in many cases, it is so important that it does lead to desirable materials’ properties and applications.
Folklore #2: “MOFs are unstable, expensive, cannot be scaled to large quantities, and are yet to find commercialization”
This is the general view of reticular chemistry, to be fair, which is mostly held by scientists outside of the field. In reality, this view could not be farther from the truth. However, we think we understand why, despite the evidence to the contrary, that this folklore persists. Let us consider, for example, how our publications appear to others outside our field. They see regular reports detailing flexible MOFs and what do they think? Materials that are unstable, not robust, and prone to dynamic change. Of course, the reality is that these frameworks exhibit interesting gate-opening effects previously unseen in any other class of materials.30–33 They also see thermal gravimetric traces very clearly showing the decomposition of our materials, beyond 300 °C, to their primary oxides and they argue that after all: “MOFs are >90% organic, meaning that they cannot be stable” or “Since COFs are 100% organic, they stand no chance of having high thermal stability whatsoever.” They are also quick to point out that MOFs/COFs are made on milligram scales and believe that this is the maximum possible production scale. Furthermore, they see complicated organic linkers and, in some cases, exotic solvents of various kinds used in our syntheses, and when they pull up the prices for purchase, they can only conclude that MOFs/COFs are prohibitively expensive.
Because this is a folklore supported by what appears to be concrete evidence, its impact cuts deep and, thus, requires a direct response. The first thing to learn about MOFs/COFs is that no two materials are created equal in terms of their structure and properties. Therefore, it has been widely shown that they can be made with varying degrees of thermal and chemical stability. When considering stability (architectural, mechanical, thermal, or chemical), it is important to first determine what kind of use one is targeting for a specific reticular material. For example, uses in gas capture and release require much lower stability than high temperature catalysis. Nevertheless, significant advancements have been made in all aspects of framework stability, especially with respect to some Zr(IV)-based MOFs, amide COFs, and hydrophobic ZIFs.34–38
Many MOFs are now fabricated and properly shaped in ton quantities by BASF (Ludwigshafen) and have been deployed in various methane-fuelled automobile pilot programs as well as for other technologies.39–42 Their end price obviously depends on the types of organic linkers and metal ions employed, but the cost issue is heavily dependent on many other factors dealing with production scale, market value, and the specific application in question. It is a matter of fact that commercialization has less to do with the science we carry out in our laboratories and more to do with societal and economic factors. In the spirit of the foregoing discussion, let us pose this question: do other important scientific innovations, such as fullerene, graphene, or carbon nanotubes stand to the test of ton scale production and widespread commercialization? One can name many other fields of prominence in this context as well. The fact is that these discoveries and areas of research have first and foremost significantly enriched chemistry by opening our minds and providing new opportunities for research and development. Similarly, reticular chemistry has changed the way we approach making new materials and it has served to widen the scope of possible structures that we can imagine and make. The reality of reticular chemistry is that the number of MOF papers has exponentially grown since 1998.5 In fact, a recent study on the number of MOF structures deposited in the Cambridge Structural Database revealed the fact that over 69
000 MOFs have been reported.43 Similar trends are found when looking at the total number of patents awarded since 2005, which includes discoveries made by small companies and research programs at large chemical and automobile corporations. All of this bodes well for the establishment of an ever-increasing range of applications and ensures commercialization of MOFs as we are beginning to see with several start-up companies and BASF (Fig. 2).44–47
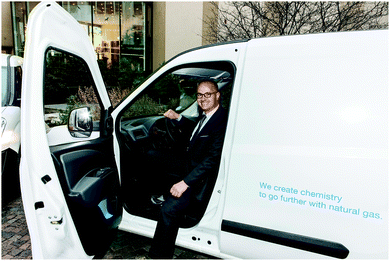 |
| Fig. 2 Using reticular chemistry, MOFs are being fabricated on the ton scale by BASF (Ludwigshafen) and several start-up companies, along with BASF, are beginning to commercialize these materials for various applications. Pictured above: Prof. Omar M. Yaghi in a pilot automobile with a MOF-filled natural gas tank. | |
Folklore #3: “All the low-hanging fruits in this field have been picked”
Nineteen years have passed since the first adsorption isotherm and proof of architectural stability and porosity,5 and the consequent emergence of MOFs as a new class of porous materials. Since then and with every major discovery, there are informal discussions that all of the interesting and ‘easy’ things have been accomplished in the field. In reality, this is not even remotely true and it is simply not the correct thinking!
We, as scientists, are not in the business of carrying out research on low hanging fruit. We must always choose scientific problems because they are challenging and intellectually stimulating. Are the problems we chose twenty years ago less or more difficult than those facing the field now? We believe that the problems are different and may very well be just as challenging. For example, the problem of establishing porosity and architectural robustness in extended structures was as difficult a challenge in the 1990s as any that we face now.
It is worth noting that in those early days, almost every aspiring scientist who was interested in developing new materials focused their attention towards creating research programs in the areas of nanoscience and nanotechnology. In the face of this, we sought something new and different by focusing our efforts on discovering and developing the building block approach for making new materials; this was a research approach that many considered to be a difficult, if not impossible, problem to solve. However, for those undeterred by this difficulty, it was rewarding to pursue and the field ultimately emerged as a strong attractor to graduate students, faculty positions, grants, industry involvement, and the like. The field rapidly continues to expand because the emphasis has always been placed on uncovering the basics of how to build structures from molecular building blocks – a zeitgeist, if you will, which is a result of the flexibility that materials can be tailor-made. Notwithstanding is the current state of being able to imagine a structure and to actually find a way to make it, characterize it, study its properties, and potential uses; all as part of one project.
Looking forward, let us consider the following: we have largely focused on carboxylate-based MOFs, but what about developing other linkages to the level of maturity that carboxylate-based MOFs have achieved? Would these new linkages provide new chemistries? What about building structures of extreme metrics for biological applications?48–50 What about constructing frameworks of varied composition (the so-called multivariate, MTV-MOFs)51–53 and finding ways of characterizing them? Can we build MOFs, in which the backbone is ordered and the functionalities are varied (i.e. achieve ‘heterogeneity (or diversity) within order’)?54 At a minimum, if we succeed in addressing the last question, we would have transferred a concept from biology and have created boundless opportunities to extend the realm of properties for truly achieving ‘sequence dependent materials’ (Fig. 3).55 If such aims are realized, a path is opened for: (a) designing materials with compartments that are open and connected to one another, yet function independently; (b) realizing materials that allow substrates to diffuse with definitive directionality and circuitry within the pores; and (c) constructing systems that can count, sort, and code chemical information in a similar fashion as to what we find in nature. All of these areas are within the realm of reticular chemistry and, indeed, various aspects of them are achievable objectives. Just these ideas alone fall within unchartered territory and, perhaps, are able to keep chemists busy for a while longer. Are these ideas easier or more difficult to accomplish than the original ideas pursued in the field 20 years ago? All of these questions and ideas are signs of a field with a lot of vigour and with much left to be explored. How the field of reticular chemistry grows and how the field is shaped in the future depends on the questions we ask and the creative way we go about answering them. Ultimately, the future depends on whether we set out to achieve high objectives or target the so-called low hanging fruit.
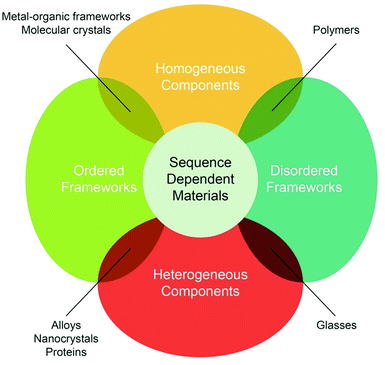 |
| Fig. 3 Studying the basics of how to construct extended frameworks from molecular building blocks is the reality of reticular chemistry. What happens when we increase the number of building blocks and introduce complexity previously only seen in nature? The future of this field is only limited by imagination and by pursuing challenging problems, such as realizing ‘sequence dependent materials’,55 as opposed to ‘low hanging fruit’. | |
Conclusions
We wish to conclude this contribution by remarking that this past year, 2016, marked the 100 year anniversary of Gilbert N. Lewis’ landmark paper on the nature of bonding in molecules.56 Since this original report, ‘The Atom and the Molecule’, chemists have built organic molecules with increasing complexity and precision, by what is referred to as total synthesis. The question of how molecules interact was not addressed until supramolecular chemistry. However, it was not until the advent of reticular chemistry that the interactions between molecules were perfected to make strongly bonded frameworks, which can withstand multiple organic reactions. It was only a result of reticular chemistry that we could begin to think of the framework as a molecule. In fact, the molecule fixes the atom in a specific geometry and spatial arrangement, and likewise, the framework fixes the molecule in a specific geometry and spatial arrangement. However, the exceptionality of the framework is that its chemistry is abundantly richer than its constituent molecules because it encompasses space into which matter can be further manipulated and controlled. The proven outcome of this has been architecturally and chemically robust frameworks. The fact that the framework is made from strong covalent bonds, and that organic and inorganic chemistry can be carried out on that framework without loss of order nor crystallinity, means that the framework is the logical progression of the molecule. What the field of reticular chemistry has accomplished and continues to do is develop the covalent chemistry of the framework. Is it not logical to conclude that we are writing the next chapter of chemistry? If we dare to think so, we most appropriately refer to this new chapter as ‘The atom, the molecule, and the framework’.2 Luckily, this is the reality and not the folklore of the reticular chemistry field!
Acknowledgements
We wish to thank the College of Chemistry at UC Berkeley and BASF (Ludwigshafen) for their contributions towards facilitating a productive atmosphere of freedom to explore and discover.
References
- O. M. Yaghi, M. O’Keeffe, N. W. Ockwig, H. K. Chae, M. Eddaoudi and J. Kim, Nature, 2003, 423, 705–714 CrossRef CAS PubMed.
- C. Diercks and O. M. Yaghi, Science, 2017, 355, 923 CrossRef CAS PubMed.
- A. P. Côté, A. I. Benin, N. W. Ockwig, M. O’Keeffe, A. J. Matzger and O. M. Yaghi, Science, 2005, 310, 1166–1170 CrossRef PubMed.
- H. M. El-Kaderi, J. R. Hunt, J. L. Mendoza-Cortés, A. P. Côté, R. E. Taylor, M. O’Keeffe and O. M. Yaghi, Science, 2007, 316, 268–272 CrossRef CAS PubMed.
- H. Li, M. Eddaoudi, T. L. Groy and O. M. Yaghi, J. Am. Chem. Soc., 1998, 120, 8571–8572 CrossRef CAS.
- H. Furukawa, K. E. Cordova, M. O’Keeffe and O. M. Yaghi, Science, 2013, 341, 1230444 CrossRef PubMed.
- O. M. Yaghi, J. Am. Chem. Soc., 2016, 138, 15507–15509 CrossRef PubMed.
- J. Jiang, Y. Zhao and O. M. Yaghi, J. Am. Chem. Soc., 2016, 138, 3255–3265 CrossRef CAS PubMed.
- K. E. Cordova, H. Furukawa and O. M. Yaghi, ACS Cent. Sci., 2015, 1, 18–23 CrossRef CAS PubMed.
- S. S. Park, C. H. Hendon, A. J. Fielding, A. Walsh, M. O’Keeffe and M. Dinca, J. Am. Chem. Soc., 2017, 139, 3619–3622 CrossRef CAS PubMed.
- A. K. Inge, M. Köppen, J. Su, M. Feyand, H. Xu, X. Zou, M. O’Keeffe and N. Stock, J. Am. Chem. Soc., 2016, 138, 1970–1976 CrossRef CAS PubMed.
- Z. Chen, L. J. Weselinski, K. Adil, Y. Belmabkhout, A. Shkurenko, H. Jiang, P. M. Bhatt, V. Guillerm, E. Dauzon, D.-X. Xue, M. O’Keeffe and M. Eddaoudi, J. Am. Chem. Soc., 2017, 139, 3265–3274 CrossRef CAS PubMed.
- D. Alezi, I. Spanopoulos, C. Tsangarakis, A. Shkurenko, K. Adil, Y. Belmabkhout, M. O’Keeffe, M. Eddaoudi and P. N. Trikalitis, J. Am. Chem. Soc., 2016, 138, 12767–12770 CrossRef CAS PubMed.
- O. K. Farha, I. Eryazici, N. C. Jeong, B. G. Hauser, C. E. Wilmer, A. A. Sarjean, R. Q. Snurr, S. T. Nguyen, A. O. Yazaydin and J. T. Hupp, J. Am. Chem. Soc., 2012, 134, 15016–15021 CrossRef CAS PubMed.
- V. Guillerm, L. J. Weselinski, Y. Belmabkhout, A. J. Cairns, V. D’Elia, L. Wojtas, K. Adil and M. Eddaoudi, Nat. Chem., 2014, 6, 673–680 CAS.
- J. An, O. K. Farha, J. T. Hupp, E. Pohl, J. I. Yeh and N. L. Rosi, Nat. Commun., 2012, 3, 604 CrossRef PubMed.
- N. Catarineu, A. Schoedel, P. Urban, M. Morla, C. Trickett and O. M. Yaghi, J. Am. Chem. Soc., 2016, 138, 10826–10829 CrossRef CAS PubMed.
- B. Tu, Q. Pang, E. Ning, W. Yan, Y. Qi, D. Wu and Q. Li, J. Am. Chem. Soc., 2015, 137, 13456–13459 CrossRef CAS PubMed.
- C. K. Brozek and M. Dinca, J. Am. Chem. Soc., 2013, 135, 12886–12891 CrossRef CAS PubMed.
- S. Kandambeth, A. Mallick, B. Lukose, M. V. Mane, T. Heine and R. Banerjee, J. Am. Chem. Soc., 2012, 134, 19524–19627 CrossRef CAS PubMed.
- K. T. Jackson, T. E. Reich and H. M. El-Kaderi, Chem. Commun., 2012, 48, 8823–8825 RSC.
- L. Ascherl, T. Sick, J. T. Margraf, S. H. Lapidus, M. Calik, C. Hettstedt, K. Karaghiosoff, M. Döblinger, T. Clark, K. W. Chapman, F. Auras and T. Bein, Nat. Chem., 2016, 8, 310–316 CrossRef CAS.
- S. Dalapati, M. Addicoat, S. Jin, T. Sakurai, J. Gao, H. Xu, S. Irle, S. Seki and D. Jiang, Nat. Commun., 2015, 6, 7786 CrossRef CAS PubMed.
- J. W. Colson, A. R. Woll, A. Mukherjee, M. P. Levendorf, E. L. Spitler, V. B. Shields, M. G. Spencer, J. Park and W. L. Dichtel, Science, 2011, 332, 228–231 CrossRef CAS PubMed.
- P. J. Waller, F. Gándara and O. M. Yaghi, Acc. Chem. Res., 2015, 48, 3053–3063 CrossRef CAS PubMed.
- K. S. Park, Z. Ni, A. P. Côté, J. Y. Choi, R. D. Huang, F. J. Uribe-Romo, H. K. Chae, M. O’Keeffe and O. M. Yaghi, Proc. Natl. Acad. Sci. U. S. A., 2006, 103, 10186–10191 CrossRef CAS PubMed.
- R. Banerjee, A. Phan, B. Wang, C. Knobler, H. Furukawa, M. O’Keeffe and O. M. Yaghi, Science, 2008, 319, 939–943 CrossRef CAS PubMed.
- N. T. T. Nguyen, H. Furukawa, F. Gándara, C. A. Trickett, H. M. Jeong, K. E. Cordova and O. M. Yaghi, J. Am. Chem. Soc., 2015, 137, 15394–15397 CrossRef CAS PubMed.
- Y. Liu, Y. Ma, Y. Zhao, X. Sun, F. Gándara, H. Furukawa, Z. Liu, H. Zhu, C. Zhu, K. Suenaga, P. Oleynikov, A. S. Alshammari, X. Zhang, O. Terasaki and O. M. Yaghi, Science, 2016, 351, 365–369 CrossRef CAS PubMed.
- S. Krause, V. Bon, E. Senkovska, U. Stoeck, D. Wallacher, D. M. Többens, S. Zander, R. S. Pillai, G. Maurin, F.-X. Coudert and S. Kaskel, Nature, 2016, 532, 348–352 CrossRef CAS PubMed.
- S. Kitagawa, R. Kitaura and S.-I. Noro, Angew. Chem., Int. Ed., 2004, 43, 2334–2375 CrossRef CAS PubMed.
- G. Férey and C. Serre, Chem. Soc. Rev., 2009, 38, 1380–1399 RSC.
- J. A. Mason, J. Oktawiec, M. K. Taylor, M. R. Hudson, J. Rodriguez, J. E. Bachman, M. I. Gonzalez, A. Cervellino, A. Guagliardi, C. M. Brown, P. L. Llewellyn, N. Masciocchi and J. R. Long, Nature, 2015, 527, 357–361 CrossRef CAS PubMed.
- J. H. Cavka, S. Jakobsen, U. Olsbye, N. Guillou, C. Lamberti, S. Bordiga and K. P. Lillerud, J. Am. Chem. Soc., 2008, 130, 13850–13851 CrossRef PubMed.
- P. J. Waller, S. Lyle, T. Osborn Popp, C. S. Diercks, J. A. Reimer and O. M. Yaghi, J. Am. Chem. Soc., 2016, 138, 15519–15522 CrossRef CAS PubMed.
- A. Phan, C. J. Doonan, F. J. Uribe-Romo, C. B. Knobler, M. O’Keeffe and O. M. Yaghi, Acc. Chem. Res., 2010, 43, 58–67 CrossRef CAS PubMed.
- N. T. T. Nguyen, H. Furukawa, F. Gándara, H. T. Nguyen, K. E. Cordova and O. M. Yaghi, Angew. Chem., Int. Ed., 2014, 53, 10645–10648 CrossRef CAS PubMed.
- A. J. Howarth, Y. Liu, P. Li, Z. Li, T. C. Wang, J. T. Hupp and O. K. Farha, Nat. Rev. Mater., 2016, 1, 15018 CrossRef CAS.
-
Chem. Soc. Rev., 2014, 43, 6173–6174 Search PubMed.
- M. Beckner and A. Dailly, Appl. Energy, 2016, 162, 506–514 CrossRef CAS.
- A. U. Czaja, N. Trukhan and U. Müller, Chem. Soc. Rev., 2009, 38, 1284–1293 RSC.
- Y. Chen, X. Huang, S. Zhang, S. Li, S. Cao, X. Pei, J. Zhou and B. Wang, J. Am. Chem. Soc., 2016, 138, 10810–10813 CrossRef CAS PubMed.
- P. Z. Moghadam, A. Li, S. B. Wiggin, A. Tao, A. G. P. Maloney, P. A. Wood, S. C. Ward and D. Fairen-Jimenez, Chem. Mater., 2017, 29, 2618–2625 CrossRef CAS.
-
Nat. Chem., 2016, 8, 987 Search PubMed.
- M. Peplow, Nature, 2015, 520, 148–150 CrossRef CAS PubMed.
- MOF Technologies, http://www.moftechnologies.com, accessed March 28, 2017.
- NuMat Technologies, http://numat-tech.com, accessed March 28, 2017.
- H. Deng, S. Grunder, K. E. Cordova, C. Valente, H. Furukawa, M. Hmadeh, F. Gándara, A. C. Whalley, Z. Liu, S. Asahina, H. Kazumori, M. O’Keeffe, O. Terasaki, J. F. Stoddart and O. M. Yaghi, Science, 2012, 336, 1018–1023 CrossRef CAS PubMed.
- P. A. Sontz, J. B. Bailer, S. Ahn and F. Akif Tezcan, J. Am. Chem. Soc., 2015, 137, 11598–11601 CrossRef CAS PubMed.
- Y. Suzuki, G. Cardone, D. Restrepo, P. D. Zavattieri, T. S. Baker and F. Akif Tezcan, Nature, 2016, 533, 369–373 CrossRef CAS PubMed.
- H. Deng, C. J. Doonan, H. Furukawa, R. B. Ferreira, J. Towne, C. B. Knobler, B. Wang and O. M. Yaghi, Science, 2010, 327, 846–850 CrossRef CAS PubMed.
- X. Kong, H. Deng, F. Yan, J. Kim, J. A. Swisher, B. Smit, O. M. Yaghi and J. A. Reimer, Science, 2013, 341, 882–885 CrossRef CAS PubMed.
- A. Helal, Z. H. Yamani, K. E. Cordova and O. M. Yaghi, Natl. Sci. Rev., 2017 DOI:10.1093/nsr/nwx013.
- H. Furukawa, U. Müller and O. M. Yaghi, Angew. Chem., Int. Ed., 2015, 54, 3417–3430 CrossRef CAS PubMed.
- T. M. Osborn Popp and O. M. Yaghi, Acc. Chem. Res., 2017, 50, 532–534 CrossRef CAS.
- G. N. Lewis, J. Am. Chem. Soc., 1916, 38, 762–785 CrossRef CAS.
|
This journal is © the Partner Organisations 2017 |
Click here to see how this site uses Cookies. View our privacy policy here.