Palladium-catalyzed allylic amination: a powerful tool for the enantioselective synthesis of acyclic nucleoside phosphonates†
Received
19th June 2017
, Accepted 7th August 2017
First published on 10th August 2017
Abstract
Acyclic nucleoside phosphonates have been prepared in a straightforward manner and in high yields by an enantioselective palladium-catalyzed allylic substitution involving nucleic bases as nucleophiles followed by cross-metathesis reaction with diethyl allylphosphonate.
Introduction
Acyclic nucleoside phosphonates (ANPs)1 belong to a family of modified nucleoside analogues, in which the sugar moiety has been replaced by a functionalized acyclic chain linking the nucleobase at one end and the phosphonic acid group at the other. Relevant ANPs that have been pursued as antiviral agents are cidofovir (HPMPC), adefovir (PMEA), and tenofovir2 (PMPA) (Fig. 1). Because of their limited oral bioavailability, the last two compounds have been converted to their oral prodrug forms, adefovir dipivoxil or bis(pivaloyloxymethyl)-PMEA [bis(POM)-PMEA] and tenofovir disoproxil or bis(isopropyloxycarbonyloxymethyl)-PMPA [bis(POC)-PMPA], respectively. Many analogues of these compounds have been prepared incorporating modifications in the base, alkyl chain or in the phosphonate moiety. Examples of modification in the alkyl chain are vinyl and allyl ANPs, where a CH2 group replaces the oxygen of the ether function in the alkyl chain (Fig. 1).3
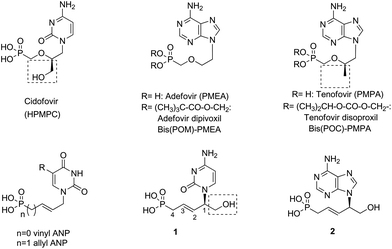 |
| Fig. 1 Relevant reported ANPs and products 1 and 2 described in this paper. | |
Acyclic nucleosides are commonly synthesized from nucleic bases and chiral starting materials by alkylation, Mitsunobu reaction, epoxide opening,4 and by silver-catalyzed addition of different nucleophiles to 9-allenyl-9H-purines.5 The reported asymmetric synthesis of acyclic nucleosides involves the transition metal-6 or organocatalyzed7 aza-Michael reaction of purinic bases to acrylates and asymmetric hydrogenation of purine-substituted acrylates.2
Allylic substitution has also been used in the synthesis of acyclic nucleosides to provide compounds with the nucleic base linked to the terminal carbon.8 Hartwig recently reported an enantioselective allylic amination using an iridium catalyst to afford acyclic nucleosides where the nucleic base is bonded to a stereocenter.9 Trost described the desymmetrization of meso 1,4-cyclopentenyl-dibenzoate derivatives using palladium-DACH catalysts in a straightforward synthesis of carbocyclic nucleosides.10 He found that the use of nucleobases as nucleophiles has a remarkable effect on the catalytic turnover and the enantioselectivity of desymmetrization reactions.9 Furthermore, Pd-catalyzed DYKAT of vinyl epoxide 4 is an efficient procedure for preparing 2-amino-3-buten-1-ol derivatives,11 which are attractive intermediates for synthesizing acyclic nucleosides, although for this purpose it will be necessary to explore the behavior of the different nucleic bases in the reaction. Recently, we have demonstrated that allyl carbonate 5, substituted with a hydroxymethyl group, is an appropriate substrate for the Pd-catalyzed asymmetric allylic amination reaction (Pd-AAA), affording the branched derivative with high regio- and enantioselectivities.12 In this regard, quite a striking different regioselectivity in the reaction of substrates 4 and 5 with amines was observed, with epoxide 4 furnishing equimolar mixtures of linear and branched aminated products. In this work, we report a comparative study of the Pd-catalyzed asymmetric allylic amination reaction starting from vinylepoxide 4 and carbonate 5 with different nucleic bases, and we show that the resulting derivatives 3 can be transformed into novel ANP nucleosides (1, 2), structurally related to cidofovir, tenofovir and to allyl ANP (Scheme 1). We have also extended this synthesis to the guanine precursor 10 (see Scheme 2).
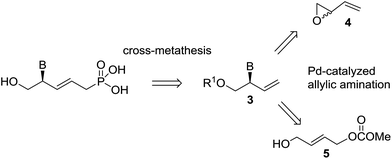 |
| Scheme 1 Retrosynthesis of ANPs 1 and 2. | |
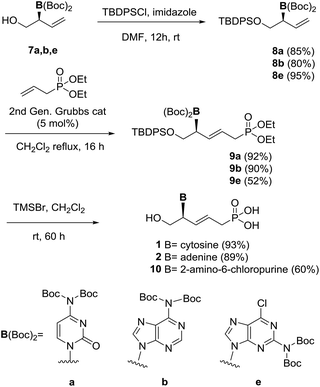 |
| Scheme 2 | |
Results and discussion
Preliminary assays starting from vinyl epoxide 4 and acetylcytosine under reaction conditions optimized in a previous study12 (6 mol% (R,R)-DACH-naphthyl Trost ligand and 2 mol% [Pd(η3-C3H5)Cl]2 as a catalytic system in CH2Cl2, at room temperature), led to the formation of a bis-allylation product containing the 3-buten-1-ol-2-yl units at position 1 and at the exocyclic NH group. This compound was obtained even by reducing the amount of acetylcytosine to 1.1 equivalents. We therefore turned our attention towards di-Boc-cytosine (6a).13 When the reaction was conducted under similar conditions using epoxide 4 and di-Boc-cytosine (6a), branched product 7a was obtained in 81% yield and 62% ee (Table 1, entry 1). Decreasing the temperature to 0 °C did not practically improve the enantioselectivity, while conducting the reaction at 35 °C eroded the enantioselectivity significantly (Table 1, entries 2 and 3). The analogous reaction of carbonate 5 with di-Boc-cytosine (6a) using the (S,S) ligand (Table 1, entry 4) furnished the enantiomer ent-7a in 92% yield and 73% ee. Interesting enough, all reactions from both 4 and 5 were highly regioselective affording exclusively the branched isomer. The use of BSA-KOAc did not improve the results. Lowering the temperature to 0 °C did not improve the enantioselectivity (Table 1, entry 5). Further optimization was focused on the precatalyst loading: a reduction from 2 mol% to 1 mol% produced a drastic decrease in the enantioselectivity (Table 1, entry 6), while an increase in the precatalyst loading to 3 mol% did not provide significant improvement (result not shown in the table). In this context, we selected room temperature and 2 mol% of the palladium precursor as standard reaction conditions.
Table 1 Allylic amination of epoxide 4 or carbonate 5 with cytosine derivative 6a affording 7a using Pd/DACH-naphthyl ligand as catalytic systema
It can be concluded from Table 1 that, in analogy to reaction from vinyl epoxide 4, Pd-AAA from carbonate 5 with di-Boc-cytosine (6a) using the [Pd(η3-C3H5)Cl]2/DACH-naphthyl ligand as the catalytic system provides good yields of the branched aminated product 7a/ent-7a in complete regioselectivity and moderate enantioselectivity. We therefore decided to extend this comparative study to other purinic bases. The results are collected in Table 2.
Table 2 Palladium-catalyzed allylic amination reaction of epoxide 4 or carbonate 5 with pyrimidinic and purinic bases 6b–ea
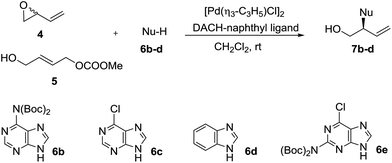
|
Entry |
Starting material |
Nucleic base |
Productb |
Conv.c (yield)d |
eee (%) |
Conditions: [Pd(η3-C3H5)Cl]2 (2 mol%), DACH-naphthyl ligand (6 mol%), substrate (1 equiv.), nucleophile (2 equiv.), rt, 16 h. Substrate concentration = 0.02 M.
The absolute configurations of the products were established by analogy with those obtained by Trost using phthalimide as analogous soft N-nucleophiles to nucleic bases (ref. 11a).
A branched/linear ratio >98 : <2 was observed in all cases by 1H NMR spectroscopy of the crude products.
Isolated yield of the branched regioisomer.
Determined by HPLC on a DAICEL CHIRALCEL OD-H column.
(R,R)-DACH-naphthyl ligand was used.
(S,S)-DACH-naphthyl ligand was used.
Using [Pd(η3-C3H5)Cl]2 (2 mol%), DACH-naphthyl ligand (6 mol%), substrate (2 equiv.), nucleophile (1 equiv.), rt, 16 h. Substrate concentration = 0.04 M.
|
1f |
4
|
6b
|
7b
|
>98 (92) |
92 |
2g |
5
|
6b
|
ent-
7b
|
>98 (92) |
88 |
3g |
4
|
6c
|
ent-
7c
|
>98 (89) |
91.4 |
4g |
5
|
6c
|
ent-
7c
|
>98 (96) |
91 |
5g |
4
|
6d
|
ent-
7d
|
>98 (89) |
88 |
6g |
5
|
6d
|
ent-
7d
|
>98 (92) |
88 |
7f |
4
|
6e
|
7e
|
31 (19) |
90 |
8f |
4
|
6e
|
7e
|
>98 (64) |
90 |
9f |
5
|
6e
|
7e
|
72 (43) |
92 |
Di-Boc-adenosine (6b) reacted with epoxide 4 in the presence of the (R,R)-DACH-naphthyl ligand to provide 7b in an excellent yield of 92% and 92% ee (entry 2). When the reaction was conducted with carbonate 5 using the (S,S)-DACH-naphthyl ligand, ent-7b was obtained in an excellent yield of 92% and 88% ee (entry 2).
6-Chloropurine (6c) is a versatile starting material in nucleoside synthesis since it allows a set of useful and well-known transformations for the synthesis of nucleoside derivatives. When carbonate 5 was treated with 6c in the presence of the (S,S)-DACH-naphthyl ligand under the standard conditions, compound ent-7c was obtained in very good 91% enantioselectivity, slightly higher than that obtained from 6b (Table 2, entry 2 vs. 4). Pd-AAA of 6c from epoxide 4 furnished the allylated purine ent-7c with almost the same enantioselectivity of that obtained from 6b (Table 2, entry 1 vs. 3).
Furthermore, the reaction of epoxide 4 and carbonate 5 with benzimidazole (6d) as a nucleophile using the (S,S)-DACH-naphthyl ligand, afforded compound ent-7d in good yields and the same enantioselectivity, (88% ee) (Table 2, entries 5 and 6).
Di-Boc-2-amino-6-chloropurine 6e reacted with epoxide 4 and carbonate 5 under standard conditions to provide 7e with high enantioselectivity but with low to moderate yields (Table 2, entries 7 and 9). However, on inverting the reaction stoichiometry a substantial improvement in the yield was achieved (64%) with the same enantio-induction (Table 2, entry 8).
Contrary to their complementary behavior in terms of regioselectivity observed with hard amine nucleophiles,12 both epoxide 4 and carbonate 5 afforded valuable branched products with high degrees of enantioselectivity when reacting with nucleobases. The regioselectivity observed in the reactions of epoxide 4 with nucleobases is comparable to that already observed with other soft nitrogen nucleophiles, such as imides or imidocarboxylates, as a result of a dynamic kinetic asymmetric transformation. As for carbonate 5, the branched regioisomer is always observed as a major product, regardless of the nitrogen nucleophile used, due to an enantioselective Pd-allylic amination, where hydrogen bonding interactions play an important role both in the ionization and the nucleophilic attack steps.12
Having explored the feasibility of using nucleic bases as nucleophiles for the synthesis of chiral branched heterocycles via a Pd-AAA with the DACH-naphthyl Trost ligand, we selected compounds 7a, 7b and 7e for synthesizing model acyclic pyrimidine and purine nucleosides.
For this purpose, the primary hydroxyl group was initially protected by reaction with tert-butyldiphenylsilyl chloride in DMF in the presence of imidazole to afford compounds 8a,b,e in excellent yields (Scheme 2). According to the retrosynthetic scheme and following previous studies in the field,14 compounds 8a,b were then treated with diethyl allylphosphonate15,16 in the presence of Grubb–Nolan's catalyst to afford compounds 9a,b in excellent 92% and 90% yields, respectively, as a result of a cross-metathesis reaction. The reaction of 8e under similar conditions afforded 9e in a moderate 52% yield. Compounds with E configuration were exclusively obtained. Removal of the protecting groups15 in the nucleic base, the phosphonate moiety and the hydroxyl group, was carried out by treatment of compounds 9a,b with TMSBr15 in dichloromethane to afford the target acyclic nucleosides 1 and 2 in excellent yields. Additionally, compound 9e was treated under the deprotection conditions to afford the 2-amino-6-chloro-9H-purine derivative 10 in good yield.
The saturated nucleoside 11, with an increased conformational mobility, was prepared by the reduction of the double bond in 9a (Scheme 3). Attempts to reduce the double bond under 1 bar of hydrogen pressure and using palladium on charcoal17 as catalysts led to no conversion. At higher hydrogen pressure (10 bar) the presence of two products was observed by 1H NMR, corresponding to the expected compound 11 and to a secondary product resulting from the reduction of the double bond and partial reduction of the cytosine. Optimal conditions were found driving the reaction at room temperature and using 3 bar of hydrogen pressure. In this way, full conversion and 87% yield of compound 11 was obtained. Removal of protecting groups using TMSBr afforded acyclic nucleoside phosphonate 12 in 80% yield.
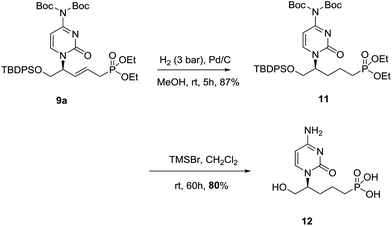 |
| Scheme 3 | |
In conclusion, acyclic nucleosides 1, 2, 10 and 12 were successfully prepared in high yields by palladium-catalyzed allylic amination from compounds 4 and 5, using pyrimidinic and purinic bases as nucleophiles, followed by ruthenium-catalyzed cross-metathesis with diethyl allylphosphonate and removal of protecting groups. Excellent enantioselectivity was obtained for the purinic derivatives 2 and 10.
Experimental section
General methods
All chemicals used were reagent grade and used as supplied unless otherwise specified. HPLC grade dichloromethane (CH2Cl2), tetrahydrofuran (THF) and dimethylformamide (DMF) were dried using a solvent purification system (Pure-Solv 400). Toluene was purified using standard procedures.181H and 13C NMR spectra were recorded on a Varian® Mercury VX 400 (400 MHz and 100.6 MHz respectively) or Varian 400-MR spectrometer in CDCl3 as a solvent, with chemical shifts (δ) referenced to the internal standard CDCl3 (7.26 ppm 1H, 77.23 ppm 13C) or Me4Si as an internal reference (0.00 ppm). 2D correlation spectra (gCOSY, NOESY, gHSQC, gHMBC) were visualized using a VNMR program (Varian®). ESI MS were run on an Agilent® 1100 Series LC/MSD instrument. Optical rotations were measured at room temperature in a PerkinElmer® 241 MC apparatus with 10 cm cells. IR spectra were recorded on a JASCO FT/IR-600 Plus Fourier transform infrared spectrometer ATR Specac Golden Gate. Optical rotations were measured at 598 nm on a Jasco DIP-370 digital polarimeter using a 100 mm cell. The enantiomeric excess was determined by an HPLC analysis using a DAICEL CHIRALCEL OD-H column. Reactions were monitored by TLC carried out on 0.25 mm E. Merck® silica gel 60F254 glass or aluminium plates. Developed TLC plates were visualized under a short-wave UV lamp (250 nm) and by heating plates that were dipped in ethanol/H2SO4 (15
:
1) and a basic solution of potassium permanganate. Flash column chromatography was carried out using forced flow of the indicated solvent on Fluka® or Merck® silica gel 60 (230–400 mesh). Radial chromatography was performed on 1 or 2 mm plates of Kieselgel 60 PF254 silica gel, depending on the amount of the product. Flash column chromatography (FCC) was performed using flash silica gel (32–63 μm) and employed a solvent polarity correlated with TLC mobility.
General procedure 1. Palladium-catalyzed allylic amination from butadiene monoepoxide (4).
In a Schlenk tube, [Pd(η3-C3H5)Cl]2 (2 mol%), the DACH-naphthyl ligand (see tables) (6 mol%), the nucleophile (2.0 eq.) and CH2Cl2 (c = 0.02 M) were introduced under an argon atmosphere. The resulting solution was stirred for 20 minutes. Butadiene monoepoxide (4) (1.0 eq.) was then added in one portion. The mixture was stirred at room temperature for 18 h. The reaction mixture was then diluted with H2O. The phases were separated and the aqueous phase was extracted with CH2Cl2. The combined organic phases were dried over MgSO4, filtered, and concentrated under vacuum. The resulting crude was purified by flash chromatography to afford a pure product.
General procedure 2. Palladium-catalyzed allylic amination from (E)-4-hydroxybut-2-en-1-yl methyl carbonate (5).
In a Schlenk tube, [Pd(η3-C3H5)Cl]2 (2 mol%), the (S,S)-DACH-naphthyl ligand (6 mol%) and CH2Cl2 (c = 0.02 M) were introduced under an argon atmosphere. The resulting mixture was stirred for 20 minutes. Carbonate 5 (1.0 eq.) and nucleophile (1.1 eq.) were then successively introduced. The mixture was stirred at room temperature for 18 h. The reaction mixture was then diluted with H2O. The phases were separated and the aqueous phase was extracted with CH2Cl2. The combined organic phases were dried over MgSO4, filtered, and concentrated under vacuum. The resulting crude was purified by flash chromatography to afford a pure product.
(R)-N,N-Bis(tert-butoxycarbonyl)-1-(1-hydroxybut-3-en-2-yl)-cytosine (ent-7a).
Following the general procedure 2, compound ent-7a was prepared from carbonate 5 (17 mg, 0.116 mmol), compound 6a (72 mg, 0.23 mmol), [Pd(η3-C3H5)Cl]2 (1 mg, 0.002 mmol) and (S,S)-DACH-naphthyl ligand (5.5 mg, 0.008 mmol) in dichloromethane (12 mL) at −10 °C. Purification by silica chromatography (hexanes/EtOAc 10
:
2) provided 41 mg (90%) of compound ent-7a as a yellow syrup and 75% ee (determined by chiral HPLC (Daicel Chiralcel OD-H, n-hexane–iPrOH, 90
:
10, flow = 0.5 mL min−1, detection, UV 210 nm; retention times (min), 9.79, 11.42, tR(R) = 9.79 min and tR(S) = 11.42 min)). [α]25D +7.13 (c 1.06, CHCl3). FTIR-ATR (cm−1): 2918, 2849, 1775, 1741, 1665, 1460, 1369, 1313, 1253, 1154, 1131, 788. 1H NMR (CDCl3, 400 MHz,): δ (ppm) 7.67 (d, 1H, J = 7.5 Hz), 7.05 (d, 1H, J = 7.5 Hz), 6.00 (ddd, 1H, J = 17.0, 10.6, 5.9 Hz), 5.42 (dd, 1H, J = 10.6, 1.5 Hz), 5.30 (dd, 1H, J = 17.0, 1.5 Hz), 5.28–5.23 (m, 1H), 4.00–3.94 (m, 2H), 3.15 (bs, 1H), 1.55 (s, 18H). 13C NMR (CDCl3, 100.6 MHz): δ (ppm) 161.9, 155.8, 149.7, 147.2, 147.0, 132.7, 120.5, 96.2, 85.1, 62.7, 61.2, 27.8. HRMS (ESI-TOF) m/z: [M + H]+ calcd for C18H28N3O6: 382.1973, found: 382.1964.
(S)-N,N-Bis(tert-butoxycarbonyl)-9-(1-hydroxybut-3-en-2-yl) adenine (7b).
Following the general procedure 1, compound 7b was prepared from butadiene monoepoxide (4) (0.02 mL, 0.25 mmol), compound 6b (100 mg, 0.3 mmol), [Pd(η3-C3H5)Cl]2 (2 mg, 0.0054 mmol) and (R,R)-DACH-naphthyl ligand (13 mg, 0.016 mmol) in dichloromethane (27 mL). Purification by silica gel chromatography (hexanes/EtOAc 1
:
1) provided 93 mg (92%) of product 7b as a yellow syrup and 92% ee (determined by chiral HPLC (Daicel Chiralcel OD-H, n-hexane–iPrOH 85
:
15, flow = 0.5 mL min−1, detection, UV 254 nm; retention times (min), 11.77, 13.47, tR(R) = 11.77 min and tR(S) = 13.47 min)). [α]25D −15.80 (c 0.92, CHCl3). FTIR-ATR (cm−1): 3347, 2979, 2926, 1787, 1600, 1107. 1H NMR (CDCl3, 400 MHz): δ (ppm) 8.84 (s, 1H), 8.17 (s, 1H), 6.20 (ddd, 1H, J = 17.1, 10.5, 6.4 Hz), 5.40 (d, 1H, J = 10.5 Hz), 5.30–5.18 (m, 1H), 5.14 (d, 1H, J = 17.1 Hz), 4.26–4.10 (m, 2H), 3.80 (bs, 1H), 1.47 (s, 18H). 13C NMR (CDCl3, 100.6 MHz): δ (ppm) 153.0, 151.7, 150.8, 150.6, 145.1, 132.7, 129.1, 119.7, 84.1, 63.8, 61.3, 27.9. HRMS (ESI-TOF) m/z: [M + H]+ calcd for C18H28N5O5: 406.2085, found: 406.2073.
(R)-6-Chloro-9-(1-hydroxybut-3-en-2-yl)-9H-purine (ent-7c).
Following the general procedure 1, compound ent-7c was prepared from butadiene monoepoxide (4) (0.024 mL, 0.3 mmol), compound 6d (50 mg, 0.32 mmol), [Pd(η3-C3H5)Cl]2 (2 mg, 0.006 mmol) and (S,S)-DACH-naphthyl ligand (14 mg, 0.017 mmol) in dichloromethane (30 mL) at rt. Purification by silica gel chromatography (hexanes/EtOAc 1
:
1) provided 60 mg (89%) product ent-7c as a colourless oil and 91.4% ee (determined by chiral HPLC (Daicel Chiralcel OD-H, n-hexane–iPrOH, 90
:
10, flow = 1 mL min−1, detection, UV 210 nm; retention times (min), 17.12, 18.36, tR(R) = 18.36 min and tR(S) = 17.12 min)). [α]25D +10.8 (c 1.23, CHCl3). FTIR-ATR (cm−1): 3347, 2927, 1591, 1561, 1337. 1H NMR (CDCl3, 400 MHz): δ(ppm) 8.70 (s, 1H), 8.23 (s, 1H), 6.21 (ddd, 1H, J = 17.0, 10.4, 6.4 Hz), 5.43 (dd, 1H, J = 10.4, 1.4 Hz), 5.30–5.25 (m, 1H), 5.24 (dd, 1H, J = 17.0, 1.4 Hz), 4.28–4.22 (m, 1H), 4.21–4.15 (m, 1H), 3.86 (t, 1H, J = 6.3 Hz). 13C NMR (CDCl3, 100.6 MHz): 151.8, 151.5, 151.4, 145.4, 132.2, 131.8, 120.4, 63.7, 61.0. HRMS (ESI-TOF) m/z: [M + H]+ calcd for C9H10ClN4O: 225.0538, found: 225.0532.
(R)-1-(1-Hydroxybut-3-en-2-yl)-1H-benzo[d]imidazole (ent-7d).
Following the general procedure 2, compound ent-7d was prepared from carbonate 5 (17 mg, 0.116 mmol), compound 6d (72 mg, 0.23 mmol), (η3C3H5PdCl)2 (1 mg, 0.002 mmol) and (S,S)-DACH-naphthyl ligand (5.5 mg, 0.008 mmol) in dichloromethane (12 mL). Purification by silica gel chromatography (hexanes/EtOAc 1
:
1) provided 20 mg (92%) of product ent-7d as a colourless oil and 88% ee (determined by chiral HPLC (Daicel Chiralcel OD-H, n-hexane–iPrOH, 92
:
08, flow = 1.0 mL min−1, detection, UV 220 nm; retention times (min), 17.84, 25.57, tR(R) = 17.84 min and tR(S) = 25.57 min)). [α]25D +26.0 (c 0.80, CHCl3). FTIR-ATR (cm−1): 3089, 2922, 1492, 1457. 1H NMR (CDCl3, 400 MHz): δ (ppm) 7.84 (s, 1H), 7.53 (d, 1H, J = 7.7 Hz), 7.36 (d, 1H, J = 7.7 Hz), 7.21 (t, 1H, J = 7.7 Hz), 7.14 (t, 1H, J = 7.7 Hz), 6.11 (ddd, 1H, J = 17.0, 10.8, 5.7 Hz), 5.37 (d, 1H, J = 10.8 Hz), 5.20 (d, 1H, J = 17.0 Hz), 5.05–4.97 (m, 1H), 4.22–4.10 (m, 2H). 13C NMR (CDCl3, 100.6 MHz): δ (ppm) 143.2, 142.2, 140.8, 132.9, 123.0, 122.5, 119.8, 119.4, 110.7, 63.3, 60.6. HRMS (ESI-TOF) m/z: [M + H]+ calcd for C11H13N2O: 189.1022, found: 189.1017.
(S)-2-Bis-tert-butoxycarbonyl-amino-6-chloro-9-(1-hydroxybut-3-en-2-yl)-9H-purine (7e).
Following the general procedure 2, compound 7e was prepared from carbonate 5 (17 mg, 0.116 mmol), compound 6e (85 mg, 0.23 mmol), [Pd(η3-C3H5)Cl]2 (1 mg, 0.002 mmol) and (R,R)-DACH-naphthyl ligand (5.5 mg, 0.008 mmol) in dichloromethane (6 mL) at room temperature. Purification by silica chromatography (hexanes/EtOAc 4
:
6) provided 22 mg (43%) of compound 7e as a yellow syrup and 92% ee (determined by chiral HPLC (Daicel Chiralcel OD-H, n-hexane–iPrOH, 90
:
10, flow = 0.6 mL min−1, detection, UV 230 nm; retention times (min), 13.5, 15.1, tR(R) = 13.5 min and tR(S) = 15.1 min)). [α]25D −34.0 (c 0.1, CHCl3). 1H NMR (CDCl3, 400 MHz): δ (ppm) 8.25 (s, 1H), 6.17 (ddd, J = 16.9, 10.5, 6.3 Hz, 1H), 5.40 (d, J = 10.4 Hz, 1H), 5.24–5.16 (m, 2H), 4.22 (dd, J = 12, 5.9 Hz, 1H), 4.11 (dd, J = 12.0, 3.0 Hz, 1H), 3.27 (br s, 1H), 1.44 (s, 18H). 13C NMR (CDCl3, 100.6 MHz,): δ (ppm) 152.2, 151.5, 151.3, 150.4, 146.0, 132.2, 129.9, 120.0, 83.9, 63.4, 60.9, 27.8. HRMS (ESI-TOF) m/z: [M + Na]+ calcd for C19H26ClN5O5Na: 462.1520, found: 462.1524.
(S)-N,N-Bis(tert-butoxycarbonyl)-1-[1-(tert-butyldiphenyl-silyloxy) but-3-en-2-yl]cytosine (8a).
Compound 7a (100 mg, 0.26 mmol) was dissolved in dry DMF (3 mL), treated under argon with tert-butyldiphenylchlorosilane (0.08 mL, 0.3 mmol) and imidazole (40 mg, 0.6 mmol), and the reaction mixture was stirred for 16 h at room temperature. The solution was then diluted with water and extracted with Et2O, and the organic layer was dried and evaporated. The residue was purified by column chromatography on silica gel (hexanes/EtOAc 10
:
2) to provide 137 mg (85%) of compound 8a. [α]25D −13.5 (c 1.27, CHCl3). FTIR-ATR (cm−1): 2931, 2857, 1742, 1671, 1524, 1455, 1370, 1319, 1256, 1137, 1110, 784, 701. 1H NMR (CDCl3, 400 MHz): δ (ppm) 7.75 (d, 1H, J = 7.5 Hz), 7.57 (d, 2H, J = 6.4 Hz), 7.48 (d, 2H, J = 6.4 Hz), 7.49–7.30 (m, 6H), 7.98 (d, 1H, J = 7.5 Hz), 6.01 (ddd, 1H, J = 17.6, 10.4, 6.0 Hz), 5.38–5.34 (m, 2H), 5.32 (d, 1H, J = 17.6 Hz), 3.97 (d, 2H, J = 3.6 Hz), 1.57 (s, 18H), 1.02 (s, 9H). 13C NMR (CDCl3, 100.6 MHz): δ (ppm) 161.9, 155.0, 149.8, 147.1, 135.7, 135.5, 133, 132.6, 130.1, 128.0, 120.6, 95.6, 84.9, 63.9, 59.6, 27.9, 26.7, 19.3. HRMS (ESI-TOF) m/z: [M + H]+ calcd for C34H46N3O6Si 620.3156, found 620.3171.
(S)-N,N-Bis(tert-butoxycarbonyl)-9-[1-(tert-butyldiphenylsilyloxy)but-3-en-2-yl]adenine (8b).
Compound 7b (250 mg, 0.6 mmol) was dissolved in dry DMF (7 mL) and treated under argon with tert-butyldiphenylchlorosilane (0.18 mL, 0.66 mmol) and imidazole (90 mg, 1.32 mmol), and the mixture was then stirred for 16 h at room temperature. The solution was then diluted with water and extracted with Et2O, and the organic layer was dried and evaporated. The residue was purified by column chromatography on silica gel (hexanes/EtOAc 10
:
2) to provide 319 mg (80%) of compound 8b as a colourless oil. [α]25D −10.93 (c 1.13, CHCl3). FTIR-ATR (cm−1): 2987, 2362, 1733, 1716, 1558, 1540, 1507, 1456, 1395, 1259, 1066, 749. 1H NMR (CDCl3, 400 MHz): δ (ppm) 8.79 (s, 1H), 8.26 (s, 1H), 7.50–7.25 (m, 10H), 6.02 (ddd, 1H, J = 17.2, 10.4, 6.4 Hz), 5.34 (dd, 1H, J = 11.6, 0.8 Hz), 5.30–5.20 (m, 1H), 5.17 (dd, 1H, J = 17.2, 0.8 Hz), 4.16 (dd, 1H, J = 11.2, 6.8 Hz), 4.05 (dd, 1H, J = 10.8, 4 Hz), 1.43 (s, 18H), 0.94 (s, 9H). 13C NMR (CDCl3, 100.6 MHz): δ (ppm) 153.3, 151.8, 150.5, 150.3, 144.5, 135.5, 135.4, 132.5, 130.1, 128.9, 127.9, 119.8, 83.7, 64.8, 59.4, 27.9, 26.8, 19.1. HRMS (ESI-TOF) m/z: [M + Na]+ calcd for C35H45N5NaO5Si 666.3088, found 666.3073.
(S)-2-Bis-tert-butoxycarbonyl-amino-6-chloro-9-[1-(tert-butyl diphenylsilyloxy)-but-3-en-2-yl]-9H-purine (8e).
Compound 7e (113 mg, 0.257 mmol) was dissolved in dry DMF (3 mL) and treated under argon with tert-butyldiphenylchlorosilane (80.1 μL, 0.308 mmol) and imidazole (40 mg, 0.59 mmol), and the mixture was then stirred for 16 h at room temperature. The solution was then diluted with water and extracted with Et2O, and the organic layer was dried and evaporated. The residue was purified by column chromatography on silica gel (hexanes/EtOAc 10
:
2) to provide 244 mg (95%) of compound 8e as a colourless oil. [α]25D −11.0 (c 0.3, CHCl3). 1H NMR (400 MHz, CDCl3) δ 8.33 (s, 1H), 7.55–7.51 (m, 2H), 7.49–7.46 (m, 2H), 7.45–7.30 (m, 6H), 6.16 (ddd, J = 17.0, 10.5, 6.3 Hz, 1H), 5.34 (dd, J = 10.5, 0.8 Hz, 1H), 5.27–5.19 (m, 1H), 5.18–5.09 (m, 1H), 4.13 (dd, J = 11.1, 5.6 Hz, 1H), 4.01 (dd, J = 11.1, 3.8 Hz, 1H), 1.39 (s, 18H), 1.01–0.95 (m, 9H). 13C NMR (100 MHz, CDCl3) δ 152.4, 151.7, 150.9, 150.4, 145.7, 135.5, 135.3, 132.3, 132.2, 132.1, 130.1, 130.0, 129.7, 127.9, 119.9, 83.4, 64.7, 59.2, 27.8, 26.7, 19.1. HRMS (ESI-TOF) m/z: [M + Na]+ calcd for C35H44ClN5O5SiNa: 700.2698, found: 700.2700.
Diethyl (2E,4S)-N,N-bis(tert-butoxycarbonyl)-1-[1-(tert-butyl-diphenylsilyloxy)-5-phosphonopent-3-en-2-yl]cytosine (9a).
To a solution of product 8a (50 mg, 0.08 mmol) and II generation Grubbs catalyst (4 mg, 0.004 mmol) in dichloromethane (4 mL) was added diethylallylphosphonate (0.06 mL, 0.32 mmol) and the reaction mixture was heated to reflux for 16 h. The solvent was then evaporated and the reaction crude was purified by silica gel chromatography (hexanes/EtOAc 1
:
2) to provide 57 mg (92%) of compound 9a as a yellow liquid. [α]25D −21.4 (c 1.2, CHCl3). FTIR-ATR (cm−1): 2969, 2931, 1741, 1671, 1455, 1370, 1319, 1255, 1111, 1024, 735, 701. 1H NMR (CDCl3, 400 MHz): δ (ppm) 7.68 (d, 1H, J = 7.6 Hz), 7.56 (d, 2H, J = 6.4 Hz), 7.45 (d, 2H, J = 6.4 Hz), 7.42–7.30 (m, 6H), 6.97 (d, 1H, J = 7.6 Hz), 5.87–5.79 (m, 2H), 5.29 (brs, 1H), 4.15–4.02 (m, 4H), 3.96 (d, 2H, J = 4.0 Hz), 2.60 (dd, 2H, J = 21.0, 6.4 Hz), 1.57 (s, 18H), 1.27 (t, 6H, J = 6.8 Hz), 1.03 (s, 9H). 13C NMR (CDCl3, 100.6 MHz): δ (ppm) 161.7, 154.6, 149.5, 146.9, 135.5, 135.3, 132.5, 132.4, 130, 129.9, 129.5, 129.4, 128.5, 127.8, 126.5, 126.3, 95.4, 84.7, 63.6, 62, 59.4, 30.6 (J = 140 Hz) 27.7, 26.8, 19.1, 16.4. HRMS (ESI-TOF) m/z: [M + H]+ calcd for C39H57N3O9PSi 770.3602, found 770.3628.
Diethyl (2E,4S)-N,N-bis(tert-butoxycarbonyl)-1-[1-(tert-butyl-diphenylsilyloxy)-5-phosphonopent-3-en-2-yl]adenine (9b).
To a solution of product 8b (140 mg, 0.22 mmol) and II generation Grubbs catalyst (9 mg, 0.011 mmol) in dichloromethane (11 mL) was added diethylallylphosphonate (0.16 mL, 0.88 mmol) and the reaction mixture was heated to reflux for 16 h. The solvent was then evaporated and the reaction crude was purified by silica gel chromatography (hexanes/EtOAc 2
:
1) to provide 157 mg (90%) of product 9b as a green liquid. [α]25D −14.6 (c 1.36, CHCl3). FTIR-ATR (cm−1): 2929, 2856, 1788, 1599, 1452, 1369, 1252, 1139, 1111, 1026, 704. 1H NMR (CDCl3, 400 MHz): δ (ppm) 8.77 (s, 1H), 8.20 (s, 1H), 7.42–7.29 (m, 10H), 6.15–6.02 (m, 1H), 5.76 (ddd, 1H, J = 15.6, 10.4, 7.6 Hz), 5.25 (brs, 1H), 4.16 (dd, 2H, J = 10.4, 6.4 Hz), 4.07–3.95 (m, 4H), 2.56 (dd, 1H, J = 21.0, 7.6 Hz), 1.43 (s, 18H), 1.22 (t, 6H, J = 6.8 Hz), 0.93 (s, 9H). 13C NMR (CDCl3, 100.6 MHz): δ (ppm) 153.2, 151.9, 150.6, 150.4, 144.5, 135.2, 132.4, 130.1, 129.1, 129.0, 128.0, 126.2, 83.8, 64.8, 62.2, 62.1, 59.2, 30.6 (J = 140 Hz), 28.0, 26.9, 19.2, 16.6. HRMS (ESI-TOF) m/z: [M + H]+ calcd for C40H57N5O8PSi 794.3714, found 794.3694.
Diethyl (2E,4S) 2-bis-tert-butoxycarbonyl-amino-6-chloro-9-[1-(tert-butyl-diphenylsilyloxy)-5-phosphonopent-3-en-2-yl]-9H-purine (9e).
To a solution of product 8e (134 mg, 0.197 mmol) and II generation Grubbs catalyst (8 mg, 0.009 mmol) in dichloromethane (8 mL) was added diethylallylphosphonate (0.134 mL, 0.788 mmol) and the reaction mixture was heated to reflux for 16 h. The solvent was then evaporated and the reaction crude was purified by silica gel chromatography (hexanes/EtOAc 1
:
2) to provide 86 mg (52%) of compound 9e as a colourless oil. [α]25D −2.4 (c 0.4, CHCl3). 1H NMR (400 MHz, CDCl3) δ 8.22 (s, 1H), 7.55–7.26 (m, 10H), 6.09–5.98 (m, 1H), 5.75 (dt, J = 14.6, 7.0 Hz, 1H), 5.18 (brs, 1H), 4.10 (dd, J = 11.0, 5.6 Hz, 2H), 4.03 (ddd, J = 14.3, 9.3, 5.3 Hz, 2H), 3.96 (dd, J = 11.0, 3.8 Hz, 2H), 2.61 (d, J = 7.4 Hz, 1H), 2.55 (d, J = 7.4 Hz, 1H), 1.40 (s, 18H), 1.24 (dd, J = 12.8, 7.0 Hz, 6H), 0.97 (s, 9H). 13C NMR (100 MHz, CDCl3) δ 152.1, 151.6, 150.9, 150.5, 145.5, 135.4, 135.3, 132.1, 130.1, 130.0, 129.8, 128.6, 128.5, 127.9, 127.8, 126.5, 126.4, 83.5, 64.7, 62.1, 61.9, 58.7, 31.2, 29.8, 27.9, 26.7, 19.0, 16.5, 16.4, 16.3. HRMS (ESI-TOF) m/z: [M + H]+ calcd for C40H56ClN5O8PSi: 828.3324, found 828.3364.
(2E,4S)-1-[5-Phosphono-1-hydroxypent-3-en-2-yl]cytosine (1).
TMSBr (0.08 mL, 0.6 mmol) was added to a solution of compound 9a (80 mg, 0.10 mmol) in CH2Cl2 (7 mL), and the mixture was stirred for 60 h at room temperature under positive pressure of dry Ar. The reaction was quenched by adding MeOH (3 mL) and the reaction mixture was then evaporated by heating at 60 °C. This process was repeated three times. The resulting residue was extracted with H2O and CH2Cl2, and the aqueous phase was evaporated to dryness to afford compound 1 (25 mg, 93%) as a yellow liquid. [α]25D −11.2 (c 2.89, MeOH). FTIR-ATR (cm−1): 2969, 1715, 1669, 1540, 1394, 1043, 973, 872, 793, 762, 748, 702. 1H NMR (D2O, 400 MHz): δ (ppm) 7.85 (d, 1H, J = 7.8 Hz), 6.16 (d, 1H, J = 7.8 Hz), 5.75–5.56 (m, 2H), 5.16 (bs, 1H), 3.88 (d, 2H, J = 6.0 Hz), 2.45 (d, 2H, J = 21.0, 7.0 Hz). 13C NMR (D2O, 100.6 MHz): δ (ppm) 158.8, 149.0, 147.0, 127.5, 127.4, 94.6, 60.9, 59.8, 31.8 (d, J = 130 Hz). HRMS (ESI-TOF) m/z: [M + H]+ calcd for C9H14N3O5P 276.0749, found 276.0719.
(2E,4S)-1-[5-Phosphono-1-hydroxypent-3-en-2-yl]adenine (2).
TMSBr (0.64 mL, 0.36 mmol) was added to a solution of compound 9b (50 mg, 0.06 mmol) in CH2Cl2 (4 mL), and the mixture was stirred for 60 h at room temperature under positive pressure of dry Ar. The reaction was quenched by adding MeOH (3 mL) and the reaction mixture was then evaporated by heating at 60 °C. This process was repeated three times. The resulting residue was extracted with H2O and CH2Cl2, and the aqueous phase was evaporated to dryness to afford compound 2 (16 mg, 89%) as a yellow liquid. [α]25D −12.4 (c 1.93, MeOH). FTIR-ATR (cm−1): 3070, 2325, 1691, 1609, 1531, 1496, 1425, 1387, 1224, 1107, 937, 770. 1H NMR (D2O, 400 MHz): δ (ppm) 8.44 (s, 1H), 8.40 (d, 1H), 6.07–5.97 (m, 1H), 5.84–5.69 (m, 1H), 5.38–5.31 (m, 1H), 4.12 (dd, 1H, J = 12.0, 8.4 Hz), 4.06 (dd, 1H, J = 12.0, 5.2 Hz), 2.62 (dd, 2H, J = 21.0, 7.0 Hz). 13C NMR (D2O, 100.6 MHz): δ (ppm) 149.6, 148.3, 143.9, 143.6, 127.8, 127.6, 118.1, 62.3, 59.2, 32.4 (d, J = 130 Hz). HRMS (ESI-TOF) m/z: [M + Na]+ calcd for C10H15N5NaO4P 323.0759, found 323.0769.
(2E,4S)-2-Amino-6-chloro-9-(5-phosphono-1-hydroxypent-3-en-2-yl)-9H-purine (10).
TMSBr (0.75 mL, 0.572 mmol) was added to a solution of compound 9e (79 mg, 0.095 mmol) in CH2Cl2 (7 mL), and the mixture was stirred for 60 h at room temperature under positive pressure of dry Ar. The reaction was quenched by adding MeOH (3 mL) and the reaction mixture was then evaporated by heating at 60 °C. This process was repeated three times. The resulting residue was extracted with H2O and CH2Cl2, and the aqueous phase was evaporated to dryness to afford compound 10 (19 mg, 60%) as a brown oil. [α]25D +6.22 (c 0.9, MeOH). 1H NMR (500 MHz, D2O) δ 8.92 (s, 1H), 5.98–5.88 (m, 1H), 5.79 (td, J = 14.4, 7.0 Hz, 1H), 5.23 (br s, 1H), 4.03 (dd, J = 12.2, 6.5 Hz, 1H), 3.97 (dd, J = 12.2, 4.1 Hz, 1H), 2.64 (d, J = 7.3 Hz, 1H), 2.59 (d, J = 7.4 Hz, 1H). 13C NMR (126 MHz, D2O) δ 155.2, 149.9, 137.5, 136.7, 135.6, 128.0, 107.7, 61.8, 60.9 (m), 31.2 (d, J = 132.1 Hz). HRMS (ESI-TOF) m/z: [M + Na]+ calcd for C10H13ClN5NaO4P 356.0291, found 356.0381.
Diethyl (S)-N,N-bis(tert-butoxycarbonyl)-1-[1-(tert-butyldiphenyl-silyloxy)-5-phosphonopent-2-yl]cytosine (11).
To a solution of 9a (150 mg, 0.2 mmol) in MeOH (6 mL), 5% palladium/carbon was added. The mixture was stirred under a hydrogen pressure (3 bar) at room temperature for 5 h. The reaction mixture was then filtered through Celite, washed with MeOH and concentrated under reduced pressure. The crude was purified by silica gel column chromatography (EtOAc) to give the compound 11 as a colourless oil (0.134 g, 87%). [α]25D −16.0 (c 2.00, CHCl3). FTIR-ATR (neat) 2930, 1743, 1669, 1456, 1320, 1264, 1137, 1113, 733, 702 cm−1. 1H NMR (CDCl3, 400 MHz): δ (ppm) 7.72 (d, 1H, J = 7.2 Hz), 7.55 (d, 2H, J = 7.6 Hz), 7.47 (d, 2H, J = 7.6 Hz), 7.40–7.30 (m, 6H), 6.99 (d, 1H, J = 7.2 Hz), 4.80 (brs, 1H), 4.12–3.93 (m, 4H), 3.84 (dd, 1H, J = 3.6, 11.2 Hz), 3.80 (dd, 1H, J = 3.2, 11.2 Hz), 1.95–1.86 (m, 2H), 1.77–1.63 (m, 4H), 1.55 (s, 18H), 1.26 (t, 6H, J = 6.8 Hz), 1.01 (s, 9H). 13C NMR (CDCl3, 100.6 MHz): δ (ppm) 161.8, 155.3, 149.8, 146.2, 135.7, 132.7, 130.2, 128.0, 95.7, 85, 64.4, 61.8, 61.7, 29.8, 27.9, 26.5 (J = 140 Hz), 24.5, 19.3, 16.7, 16.6. HRMS (ESI-TOF) m/z: [M + H]+ calcd for C39H59N3O9PSi 772.3758, found: 772.3733.
(S)-1-[5-Phosphono-1-hydroxypent-2-yl]cytosine (12).
TMSBr (0.15 ml, 0.9 mmol) was added to a solution of compound 11 (120 mg, 0.15 mmol) in CH2Cl2 (12 mL) and the mixture was stirred for 60 h at room temperature under positive argon pressure. Then MeOH (3 mL) was added and evaporated with heating (ca. 60 °C). This process was repeated three times. The residue was extracted with H2O and CH2Cl2, and the aqueous phase was evaporated. The crude product was purified by reverse phase chromatography on Silica C18 using (H2O, MeOH) as the eluent to give 33 mg (80%) of compound 12 as a white foam. [α]25D −12.0 (c 0.20, MeOH). FTIR-ATR (neat, cm−1): 3327, 2918, 1668, 1540, 1456, 1387, 1148, 1050, 920, 785 cm−1. 1H NMR (D2O, 400 MHz): δ (ppm) 7.87 (d, 1H, J = 8.0 Hz), 6.19 (d, 1H, J = 8.0 Hz), 4.67–4.62 (m, 1H), 3.76–3.70 (m, 2H), 1.81–1.75 (m, 4H), 1.55–1.48 (m, 2H). 13C NMR (D2O, 100.6 MHz): δ (ppm) 158.7, 149.4, 146.2, 94.2, 61.7, 58.5, 28.8, 25.4 (J = 130 Hz), 18.3. HRMS (ESI-TOF) m/z: [M + Na]+ calcd for C9H16N3NaO5P: 300.0725, found: 300.0733.
Conflicts of interest
There are no conflicts to declare.
Acknowledgements
The authors thank the Ministerio de Economía y Competitividad, Spain (grant DGI CTQ2014-58664-R). M. A. thanks AECI and SS thanks URV for a fellowship. MEC thanks to CONACyT-México for a post-doctoral grant.
Notes and references
-
(a) E. De Clercq, Collect. Czech. Chem. Commun., 2011, 76, 480 CrossRef
;
(b)
M. Krecmerova, in Herpesviridae—A Look into This Unique Family of Viruses, ed. G. D. Magel and S. Tyring, INTECH, 2012, ch. 11, p. 245 Search PubMed
;
(c) E. De Clercq, Antiviral Res., 2007, 75, 1 CrossRef CAS PubMed
;
(d) E. De Clercq and A. Holy, Nat. Rev. Drug Discovery, 2005, 4, 928 CrossRef CAS PubMed
;
(e) A. Holy, Curr. Pharm. Des., 2003, 9, 2567 CrossRef CAS PubMed
;
(f)
A. Holy, in Current Protocols in Nucleic Acid Chemistry, John Wiley and Sons, Unit 14.2, 2005 Search PubMed
.
-
(a) H.-L. Sun, F. Chen, M.-S. Xie, H.-M. Guo, G.-R. Qu, Y.-M. He and Q.-H. Fan, Org. Lett., 2016, 18, 2260 CrossRef CAS PubMed
;
(b) H.-L. Sun, F. Chen, M.-S. Xie, H.-M. Guo, G.-R. Qu, Y.-M. He and Q.-H. Fan, Org. Lett., 2014, 16, 2014 CrossRef PubMed
.
-
(a) K. A. Cruickshank, J. Jiricny and C. B. Reese, Tetrahedron Lett., 1984, 25, 681 CrossRef CAS
;
(b) H. Tanaka, H. Hayakawa, K. Obi and T. Miyasaka, Tetrahedron, 1986, 42, 4187 CrossRef CAS
;
(c) L. A. Agrofoglio, I. Gillaizeau and Y. Saito, Chem. Rev., 2003, 103, 1875 CrossRef CAS PubMed
;
(d) M. Hamada, V. Roy, T. R. McBrayer, T. Whitaker, C. Urbina-Blanco, S. P. Nolan, J. Balzarini, R. Snoeck, G. Andrei, R. F. Chinazi and L. A. Agrofolio, Eur. J. Med. Chem., 2013, 67, 398 CrossRef CAS PubMed
.
- For a recent review about chemical synthesis of acyclic nucleosides see:
(a) M.-S. Xie, H.-Y. Niu, G.-R. Qu and H.-M. Guo, Tetrahedron Lett., 2014, 55, 7156 CrossRef CAS
;
(b)
H.-M. Guo, S. Wu, H.-Y. Niu, G. Song and G.-R. Qu, in Chemical Synthesis of Nucleoside Analogues, ed. P. Merino, John Wiley and Sons, 2013, p. 103 Search PubMed
.
- T. Wei, M.-S. Xie, G.-R. Qu, H.-Y. Niu and H.-M. Gou, Org. Lett., 2014, 16, 900 CrossRef CAS PubMed
.
- M. Gandelman and E. N. Jacobsen, Angew. Chem., Int. Ed., 2005, 44, 2393 CrossRef CAS PubMed
.
-
(a) H.-M. Guo, T.-F. Yuan, H.-Y. Niu, J.-Y. Liu, R.-Z. Mao, D.-Y. Li and G.-R. Qu, Chem. – Eur. J., 2011, 17, 4095 CrossRef CAS PubMed
;
(b) H. Wu, Z. Q. Tian, L. L. Zhang, Y. D. Huang and Y. M. Wang, Adv. Synth. Catal., 2012, 354, 2977 CrossRef CAS
.
-
(a) H.-Y. Niu, C. Du, M.-S. Xie, Y. Wang, Q. Zhang, G.-R. Qu and H.-M. Guo, Chem. Commun., 2015, 51, 3328 RSC
;
(b) F. Amblard, S. P. Nolan, I. Gillaizeau and L. A. Agrofoglio, Tetrahedron Lett., 2003, 44, 9177 CrossRef CAS
.
- L. M. Stanley and J. F. Hartwig, J. Am. Chem. Soc., 2009, 131, 8971 CrossRef CAS PubMed
.
-
(a) B. M. Trost, R. Madsen, S. G. Guile and B. Brown, J. Am. Chem. Soc., 2000, 122, 5947–5956 CrossRef CAS
;
(b) B. M. Trost, R. Madsen, S. G. Guile and A. E. H. Elia, Angew. Chem., Int. Ed. Engl., 1996, 35, 1569 CrossRef CAS
.
-
(a) B. M. Trost, D. B. Home and M. J. Weltering, Chem. – Eur. J., 2006, 12, 6607 CrossRef CAS PubMed
;
(b) J. Llaveria, Y. Díaz, M. I. Matheu and S. Castillon, Org. Lett., 2009, 11, 205–208 CrossRef CAS PubMed
;
(c) J. Llaveria, Y. Díaz, M. I. Matheu and S. Castillon, Eur. J. Org. Chem., 2011, 1514 CrossRef CAS
;
(d) S. Soriano, M. Azzouz, J. Llaveria, P. Marcé, M. I. Matheu, Y. Díaz and S. Castillon, J. Org. Chem., 2016, 81, 5217 CrossRef CAS PubMed
.
- S. Soriano, M. I. Matheu, Y. Díaz and S. Castillon, Adv. Synth. Catal., 2016, 358, 4057 CrossRef CAS
.
- P. Andrea, G. Giampaolo, P. Ivana, C. Mariolino and N. Giammario, Eur. J. Org. Chem., 2008, 5786 Search PubMed
.
-
(a) P. R. Hanson and D. S. Stoianova, Tetrahedron Lett., 1998, 39, 3939 CrossRef CAS
;
(b) P. R. Hanson and D. S. Stoianova, Tetrahedron Lett., 1999, 40, 3297 CrossRef CAS
;
(c) M. Bujard, V. Gouverneur and C. Mioskowski, J. Org. Chem., 1999, 64, 2119 CrossRef CAS PubMed
;
(d) M. Schuman, M. Trevitt, A. Redd and V. Gouverneur, Angew. Chem., Int. Ed., 2000, 39, 2491 CrossRef CAS
.
- K. Hiroki, D. Topalis, J. Broggi, U. Pradere, V. Roy, S. Berteina-Raboin, P. S. Nolan, D. Deville-Bonne, G. Andrei, R. Snoeck, D. Garin, J. M. Crance and L. A. Agrofolio, Tetrahedron, 2008, 64, 3517 CrossRef
.
-
(a) U. Pradère, H. Clavier, V. Roy, S. P. Nolan and L. A. Agrofoglio, Eur. J. Org. Chem., 2011, 7324–7330 CrossRef
;
(b) A. Montagu, U. Pradére, V. Roy, S. P. Nolan and L. A. Agrofoglio, Tetrahedron, 2001, 67, 5319 CrossRef
.
- T. Tomioka, Y. Yabe, T. Takahashi and T. K. Simmons, J. Org. Chem., 2011, 76, 4669 CrossRef CAS PubMed
.
-
D. D. Perrin and W. L. F. Armarego, Purification of Laboratory Chemicals, Pergamon Press, Oxford, 3rd edn, 1989 Search PubMed
.
Footnote |
† Electronic supplementary information (ESI) available: 1H and 13C NMR and HPLC traces of reported products. See DOI: 10.1039/c7ob01478c |
|
This journal is © The Royal Society of Chemistry 2017 |
Click here to see how this site uses Cookies. View our privacy policy here.