DOI:
10.1039/C6OB02277D
(Communication)
Org. Biomol. Chem., 2017,
15, 56-60
1,6-Conjugate addition of zinc alkyls to para-quinone methides in a continuous-flow microreactor†
Received
18th October 2016
, Accepted 1st November 2016
First published on 1st November 2016
Abstract
An efficient method for the synthesis of alkyl diarylmethanes through the 1,6-conjugate addition of dialkylzinc reagents to para-quinone methides (p-QMs) has been developed under continuous flow conditions using a microreactor. This protocol allows to access unsymmetrical alkyl diarylmethanes in moderate to excellent yields using a wide range of p-QMs and dialkylzinc reagents. Interestingly, this transformation worked well without the requirement of a catalyst.
Microreaction technology is emerging as one of the imperative sub-areas in organic synthesis1 and its application has been realised from lab-scale syntheses to process industries.2 This technology involves processing continuous-flow reactions within structured microchannels having a diameter of less than 1 mm. Due to the high surface to volume ratio of the microchannels, mixing of components as well as the heat transfer occur efficiently and, as a result, the overall mass transfer in the reaction improves significantly. Microreaction technology is particularly useful in highly exothermic reactions, which involve the formation of explosive or hazardous intermediates or products.3 Moreover, the formation of unwanted by-products could be minimised in a microreactor as the reaction mixture does not stay in the microchannels for a long time. Due to these inherent advantages, the application of micro-flow reactors has been comprehended not only in simple two-component reactions,1 but also in multi-component,4 photochemical5 and enantioselective reactions.6 Microreaction technology has also been utilised in the multi-step synthesis of natural products7 as well as active pharmaceutical ingredients (APIs).8
The utility of zinc alkyls in carbon–carbon bond forming reactions has been well appraised. In particular, the enantioselective 1,2-addition of dialkylzinc reagents to carbonyl compounds and imines is very well explored.9 There are several reports documented for the non-enantioselective as well as enantioselective 1,4-conjugate addition of dialkylzinc reagents to enones.10 However, performing the 1,6-conjugate addition of organometallic reagents to dienone systems in a regiospecific or a highly regioselective manner is a challenging task as these types of reactions always produce 1,4- and/or 1,2-addition products along with the 1,6-product. Despite this limitation, many reports are available in the literature for the regioselective and enantioselective 1,6-conjugate addition of organometallic reagents to dienone systems.11 In fact, a few metal catalysed enantioselective 1,6-conjugate addition reactions of zinc alkyls to dienones are reported.12 While exploring para-quinone methide (p-QM) as an electrophile for carbon–carbon13 and carbon–heteroatom14 bond forming reactions, we believed that it could be possible to access alkyl diarylmethanes through the regioselective 1,6-conjugate addition of dialkylzinc reagents to p-QMs. It is worth mentioning that alkyl diarylmethanes are an important class of compounds in the area of drug discovery as many of them possess interesting medicinal properties (Fig. 1).15
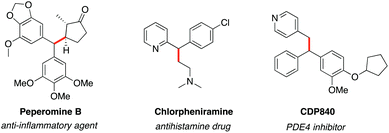 |
| Fig. 1 Some biologically active unsymmetrical alkyl diarylmethanes. | |
Herein, we report an effective and a direct method for the synthesis of unsymmetrical alkyl diarylmethanes through the regiospecific 1,6-conjugate addition of dialkylzinc reagents to p-QMs by taking advantage of the unique 1,6-reactivity of p-QMs.16 Since the continuous-flow method has many advantages over the conventional reactions carried out in the reaction flask, we decided to explore this methodology in a continuous-flow microreactor. Although there are a few reports available for the 1,2-addition of dialkylzinc reagents to aldehydes under continuous-flow,17,6c to the best of our knowledge, the 1,6-conjugate addition to p-QMs has not been reported so far either using conventional flask chemistry or under a continuous-flow method in a microreactor.
The optimisation studies were carried out with 1 (20 mg dissolved in 1 mL of solvent) and diethylzinc 2a (2 equiv. dissolved in solvent accordingly to make it to 1 mL) under various flow rates in a microreactor having a total volume of 100 μL (Table 1). To out delight, when the solutions of 1 & 2a in toluene were injected at a flow rate of 20 μL min−1 (residence time in the microreactor = 2.5 min) through the microreactor maintained at 25 °C, the expected product 3 was observed in 60% conversion (based on 1H NMR) [entry 1]. Since product 3 and p-QM 1 were observed at the same Rf value in the TLC plate, purification of 3 was found to be difficult, so the conversion in this case was calculated based on 1H NMR analysis of the crude mixture. An interesting thing is that this transformation worked without the requirement of any external catalyst. When the flow rates of 1 and 2a were decreased to 10 μL min−1 (residence time = 5 min), we observed that there was a significant improvement in the conversion of 1 to 3 (74%, based on 1H NMR) [entry 2]. From this observation, we supposed that it could be possible to maximise the conversion ratio by decreasing the flow rates further. Consequently, the reaction was performed at a flow rate of 5 μL min−1 (residence time = 10 min). In this case, complete consumption of 1 was observed (by 1H NMR), and product 3 was obtained in 92% isolated yield (entry 3). To find out the best suited solvent for this transformation, a few reactions were carried out in other solvents by keeping the flow rates constant (5 μL min−1). However, unfortunately, solvents such as n-hexane and diethylether were found to be less efficient, when compared to toluene, as product 3 was obtained only in 81 and 82% yield respectively (entries 4 & 5). The reaction was also carried out in a mixture of toluene and hexane (1
:
1) and, in this case, 3 was isolated in only 85% yield (entry 6). The alkyl diarylmethane 3 was obtained in a relatively low yield (85%), when the temperature of the microchannels was lowered to 0 °C (entry 7). To find out the optimal equivalents of 2a with respect to 1, another reaction was performed with 1.5 equivalents of 2a. In this case, the complete consumption of 1 was not observed and 3 was obtained in 78% yield (by 1H NMR) [entry 8]. So, it is clear that the use of 2 equivalents of 2a is necessary to drive the reaction to completion. To compare the efficiency of the continuous-flow reaction with conventional reaction flask chemistry, an experiment was performed in which, a solution of Et2Zn in toluene was added in a drop-wise manner to a solution of 1 in toluene in a 10 mL rb flask at 0 °C and the resulting mixture was stirred at rt for half an hour (entry 9). In this case, product 3 was obtained in 90% isolated yield, which clearly indicates that the yield of 3 in a microreactor (92%, entry 3) was marginally better when compared to that of the conventional reaction carried out in the reaction flask (entry 9).
Table 1 Optimisation studiesa
After finding the most effective reaction conditions (entry 3, Table 1), the substrate scope of this transformation was assessed using a range of p-QMs and zinc alkyls under these conditions. The reaction worked generally very well for most of the p-QMs, irrespective of the electronic nature of the aryl group attached to them (Table 2). In the cases of simple aryl substituted p-QMs (1a & 1b), the alkyl diarylmethanes 3a and 3b were obtained in 85 and 99% isolated yields respectively when the reaction was carried out with diethylzinc (2a). For p-QMs (1c–e), substituted with electron-rich arenes, the corresponding products (3c–e) were obtained in the range of 74–88% isolated yields. The alkyl diarylmethanes (3f–j) were obtained in good yields (78–91%) when p-QMs (1f–j), substituted with halogenated arenes, and were treated with 2a under standard conditions. This transformation was also found to be effective for p-QMs (1k & 1l), prepared from electron-poor aromatic aldehydes such as 3-trifluoromethyl benzaldehyde and 4-nitrobenzaldehyde, and the respective products (3k & 3l) were isolated in 64 and 91% yields respectively. However, in the case of 1m (substituted with 2-thiophenyl), the reaction was found to be very slow, therefore, the flow rates of 1m & 2a were reduced to 2 μL min−1 (instead 5 μL min−1) to obtain product 3m in 76% yield. This methodology was also elaborated on other zinc alkyls such as dicyclopentylzinc (2b) [0.4 M solution in Et2O] and dicyclohexylzinc (2c) [0.4 M solution in Et2O]. For example, the reaction of 2b with the p-QMs 3a and 3 gave the products 3n and 3o in 93 and 75% isolated yields respectively.
Reaction conditions: reactions were carried out with 20 mg of 1 at 25 °C. 1 was diluted with 1 mL of toluene. 2 was diluted with toluene accordingly to make it to 1 mL.
The flow rates of 1m and 2a = 2 μL min−1. Yields reported are isolated yields.
|
|
Similarly, the products 3p and 3q were obtained in good yields (98 and 89% respectively) when 2c was used as a nucleophile. Further extension in the substrate scope was carried out by using other substituted p-QMs such as 1r (derived from 1,6-diisopropyl phenol) and, in this case, the expected product 3r was obtained in 85% isolated yield.
To show the continuous production of 3 over a period of time, a reaction was performed with a relatively large scale of 1 (Scheme 1). In this experiment, 0.5 g of 1 (dissolved in 5 mL of toluene) and 2a (3.9 mL dissolved in 1.1 mL of toluene) were injected through a microreactor over a period of more than 16 h at a flow rate of 5 μL min−1 and 3 was obtained in 95% isolated yield.
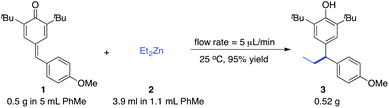 |
| Scheme 1 Continuous production of 3 over a period of time. | |
Another interesting thing is that one can visually monitor the progress of this particular reaction in a microreactor as the colours of the starting material (1) and the product (3) are different. In general, the p-QMs are deep yellow or orange in colour and most of the alkyl diarylmethanes are colourless. When the reaction between 1 and 2a was monitored carefully under the standard conditions, the colour change of the reaction mixture from yellow to colourless was observed during the course of the reaction. The actual photograph of the reaction set up is given in Fig. 2. As shown in Fig. 2, the colour of the reaction mixture in the first half of the microchannels was yellow and, as the reaction progressed, the reaction mixture became colourless in the later portion of the microchannels, which clearly indicates that the reaction was complete.
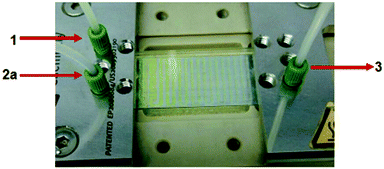 |
| Fig. 2 Actual photograph of the reaction mixture in a microreactor. | |
Conclusions
We have developed a continuous-flow method for the synthesis of unsymmetrical alkyl diarylmethanes through 1,6-conjugate addition of various zinc alkyls to p-quinone methides in a microreactor. This protocol worked generally well for a wide range of p-QMs as well as zinc alkyls. Since this transformation was proceeding well even at a sub-zero temperature without any catalyst, the scope of this transformation is limited to non-enantioselective version only.
Acknowledgements
We gratefully acknowledge IISER Mohali for providing financial support and infrastructure. ASJ thanks IISER Mohali for a research fellowship. The NMR and HRMS facilities at IISER Mohali are gratefully acknowledged.
Notes and references
- For selected reviews, see:
(a) K. Jähnisch, V. Hessel, H. Löwe and M. Baerns, Angew. Chem., Int. Ed., 2004, 43, 406–446 CrossRef PubMed;
(b) P. Watts and S. J. Haswell, Chem. Soc. Rev., 2005, 34, 235–246 RSC;
(c) K. Geyer, J. D. C. Codeé and P. H. Seeberger, Chem. – Eur. J., 2006, 12, 8434–8442 CrossRef CAS PubMed;
(d) J. Kobayashi, Y. Mori and S. Kobayashi, Chem. – Asian J., 2006, 1, 22–35 CrossRef CAS PubMed;
(e) B. P. Mason, K. E. Price, J. L. Steinbacher, A. R. Bogdan and D. T. McQuade, Chem. Rev., 2007, 107, 2300–2318 CrossRef CAS PubMed;
(f) P. Watts and C. Wiles, Chem. Commun., 2007, 443–467 RSC;
(g) B. Ahmed-Omer, J. C. Brandt and T. Wirth, Org. Biomol. Chem., 2007, 5, 733–740 RSC;
(h) C. Wiles and P. Watts, Eur. J. Org. Chem., 2008, 1655–1671 CrossRef CAS;
(i) T. Fukuyama, M. T. Rahman, M. Sato and I. Ruy, Synlett, 2008, 151–163 CAS;
(j) R. L. Hartman and K. F. Jensen, Lab Chip, 2009, 9, 2495–2507 RSC;
(k) K. Geyer, T. Gustafsson and P. H. Seeberger, Synlett, 2009, 2382–2391 CAS;
(l) T. Razzaq and C. O. Kappe, Chem. – Asian J., 2010, 5, 1274–1289 CAS;
(m) D. T. McQuade and P. H. Seeberger, J. Org. Chem., 2013, 78, 6384–6389 CrossRef CAS PubMed;
(n) K. S. Elvira, X. C. I. Solvas, R. C. R. Wootton and A. J. deMello, Nat. Chem., 2013, 5, 905–915 CrossRef CAS PubMed;
(o) H. P. L. Gemoets, Y. Su, M. Shang, V. Hessel, R. Luque and T. Noël, Chem. Soc. Rev., 2016, 45, 83–117 RSC;
(p) M. Movsisyan, E. I. P. Delbeke, J. K. E. T. Berton, C. Battilocchio, S. V. Ley and C. V. Stevens, Chem. Soc. Rev., 2016, 45, 4892–4928 RSC.
-
(a) X. Zhang, S. Stefanick and F. Villani, J. Org. Process Res. Dev., 2004, 8, 455 CrossRef CAS;
(b) D. M. Roberge, B. Zimmermann, F. Rainone, M. Gottsponer, M. Eyholzer and N. Kockmann, Org. Process Res. Dev., 2008, 12, 905–910 CrossRef CAS;
(c) A. Pohar and I. Plazl, Chem. Biochem. Eng. Q., 2009, 23, 537–544 CAS;
(d) P. Plouffle, A. Macchi and D. M. Roberge, Org. Process Res. Dev., 2014, 18, 1286–1294 CrossRef;
(e) P. L. Heider, S. C. Born, S. Basak, B. Benyahia, R. Lakerveld, H. Zhang, R. Hogan, L. Buchbinder, A. Wolfe, S. Mascia, J. M. B. Evans, T. F. Jamison and K. F. Jensen, Org. Process Res. Dev., 2014, 18, 402–409 CrossRef CAS.
- T. Westermann and L. Mleczko, Org. Process Res. Dev., 2016, 20, 487–494 CrossRef CAS.
-
(a) W. S. Bremner and M. G. Organ, J. Comb. Chem., 2007, 9, 14–16 CrossRef CAS PubMed;
(b) N. Pagano, A. Herath and N. D. P. Cosford, J. Flow Chem., 2011, 1, 28–31 CrossRef CAS PubMed;
(c) G. C. O. Silva, J. R. Correa, M. O. Rodrigues, H. G. O. Alwim, B. C. Guido, C. C. Gatto, K. A. Wanderley, M. Fioramonte, F. C. Gozzo, R. O. M. A. de Souza and B. A. D. Neto, RSC Adv., 2015, 5, 48506–48515 RSC.
- For recent reviews, see:
(a) E. E. Coyle and M. Oelgemöller, Photochem. Photobiol. Sci., 2008, 7, 1313–1322 RSC;
(b) M. Oelgemöller and O. Shvydkiv, Molecules, 2011, 16, 7522–7550 CrossRef PubMed;
(c) J. P. Knowles, L. D. Elliott and K. I. Booker-Milburn, Beilstein J. Org. Chem., 2012, 8, 2025–2052 CrossRef CAS PubMed;
(d) Y. Su, N. J. W. Straathof, V. Hessel and T. Noël, Chem. – Eur. J., 2014, 20, 10562–10589 CrossRef CAS PubMed;
(e) D. Cambie, C. Bottecchia, N. J. W. Straathof, V. Hessel and T. Noël, Chem. Rev., 2016, 116, 10276–10341 CrossRef CAS PubMed.
- For reviews, see:
(a) X. Y. Mak, P. Laurino and P. H. Seeberger, Beilstein J. Org. Chem., 2009, 5, 19 Search PubMed;
(b) D. Zhao and K. Ding, ACS Catal., 2013, 3, 928–944 CrossRef CAS;
(c) T. Tsubogo, T. Ishiwata and S. Kobayashi, Angew. Chem., Int. Ed., 2013, 52, 6590–6604 CrossRef CAS PubMed;
(d) R. Porta, M. Benagila, F. Coccia, S. Rossi and A. Puglisi, Symmetry, 2015, 7, 1395–1409 CrossRef and references cited therein.
-
(a) J. C. Pastre, D. L. Browne and S. V. Ley, Chem. Soc. Rev., 2013, 42, 8849–8869 RSC;
(b) P. Zhang, M. G. Russell and T. F. Jamison, Org. Process Res. Dev., 2014, 18, 1567–1570 CrossRef CAS;
(c) M. de Léséleuc, E. Godin, S. Parisien-Collette, A. Lévesque and S. K. Collins, J. Org. Chem., 2016, 81, 6750–6756 CrossRef PubMed.
- For reviews, see:
(a) D. Webb and T. F. Jamison, Chem. Sci., 2010, 1, 675–680 RSC;
(b) B. Gutmann, D. Cantillo and C. O. Kappe, Angew. Chem., Int. Ed., 2015, 54, 6688–6728 CrossRef CAS PubMed;
(c) M. Baumann and I. R. Baxendale, Beilstein J. Org. Chem., 2015, 11, 1194–1219 CrossRef CAS PubMed;
(d) R. Porta, M. Benaglia and A. Puglinsi, Org. Process Res. Dev., 2016, 20, 2–25 CrossRef CAS.
- For selected reviews, see:
(a) K. Soai and S. Niwa, Chem. Rev., 1992, 92, 833–856 CrossRef CAS;
(b) L. Pu and H.-B. Yu, Chem. Rev., 2001, 101, 757–824 CrossRef CAS PubMed;
(c) A. B. Charette, A. A. Boezio, A. Côté, E. Moreau, J. Pytkowicz, J.-N. Desrosiers and C. Legault, Pure Appl. Chem., 2005, 77, 1259–1267 CrossRef CAS;
(d) F. Schmidt, R. T. Stemmler, J. Rudolph and C. Bolm, Chem. Soc. Rev., 2006, 35, 454–470 CAS;
(e) K. Yamada and K. Tomioka, Chem. Rev., 2008, 108, 2874–2886 CrossRef CAS PubMed;
(f) A. Lemire, A. Côté, M. K. Janes and A. B. Charette, Aldrichimica Acta, 2009, 42, 71–83 CAS;
(g) S. Kobayashi, Y. Mori, J. S. Fossey and M. M. Salter, Chem. Rev., 2011, 111, 2626–2704 CrossRef CAS PubMed.
-
(a) B. E. Rossiter and N. M. Swingle, Chem. Rev., 1992, 92, 771–806 CrossRef CAS;
(b) T. Jerphagnon, M. G. Pizzuti, A. J. Minnaard and B. L. Feringa, Chem. Soc. Rev., 2009, 38, 1039–1075 RSC;
(c) J. H. Kim, Y. O. Ko, J. Bouffard and S. Lee, Chem. Soc. Rev., 2015, 44, 2489–2507 RSC.
-
(a) T. den Hartog, S. R. Harutyunyan, D. Font, A. J. Minnard and B. L. Feringa, Angew. Chem., Int. Ed., 2008, 47, 398–401 CrossRef CAS PubMed;
(b) T. Nishimura, Y. Yasuhara, T. Sawano and T. Hayashi, J. Am. Chem. Soc., 2010, 132, 7872–7873 CrossRef CAS PubMed;
(c) T. den Hartog, D. J. van Dijken, A. J. Minnard and B. L. Feringa, Tetrahedron: Asymmetry, 2010, 21, 1574–1584 CrossRef CAS;
(d) E. M. P. Silva and A. M. S. Silva, Synthesis, 2012, 3109–3128 CrossRef CAS;
(e) T. Sawano, A. Ashouri, T. Nishimura and T. Hayashi, J. Am. Chem. Soc., 2012, 134, 18936–18939 CrossRef CAS PubMed;
(f) T. Nishimura, A. Noishiki and T. Hayashi, Chem. Commun., 2012, 48, 973–975 RSC;
(g) M. Tissot, D. Poggiali, H. Hénon, D. Müller, L. Guénée, M. Mauduit and A. Alexakis, Chem. – Eur. J., 2012, 18, 8731–8747 CrossRef CAS PubMed;
(h) F. Meng, X. Li, S. Torker, Y. Shi, X. Shen and A. H. Hoveyda, Nature, 2016, 537, 387–393 CrossRef CAS PubMed.
- For selected examples, see:
(a) H. Hénon, M. Mauduit and A. Alexakis, Angew. Chem., Int. Ed., 2008, 47, 9122–9124 CrossRef PubMed;
(b) M. Magrez, J. Wencel-Delord, A. Alexakis, C. Crévisy and M. Mauduit, Org. Lett., 2012, 14, 3576–3579 CrossRef CAS PubMed;
(c) C. Jahier-Diallo, M. S. T. Morin, P. Queval, M. Rouen, I. Artur, P. Querard, L. Toupet, C. Crévisy, O. Baslé and M. Mauduit, Chem. – Eur. J., 2015, 21, 993–997 CrossRef CAS PubMed;
(d) M. S. T. Morin, T. Vives, O. Baslé, C. Crévisy, V. Ratovelomanana-Vidal and M. Mauduit, Synthesis, 2015, 2570–2577 CAS;
(e) T. E. Schmid, S. Drissi-Amraoui, C. Crévisy, O. Baslé and M. Mauduit, Beilstein J. Org. Chem., 2015, 11, 2418–2434 CrossRef CAS PubMed.
-
(a) V. Reddy and R. V. Anand, Org. Lett., 2015, 17, 3390–3393 CrossRef CAS PubMed;
(b) B. T. Ramanjaneyulu, S. Mahesh and R. V. Anand, Org. Lett., 2015, 17, 3952–3955 CrossRef CAS PubMed;
(c) P. Goswami and R. V. Anand, ChemistrySelect, 2016, 1, 2556–2559 CrossRef CAS;
(d) P. Arde and R. V. Anand, RSC Adv., 2016, 6, 77111–77115 RSC;
(e) S. Mahesh, G. Kant and R. V. Anand, RSC Adv., 2016, 6, 80718–80722 RSC.
- P. Arde and R. V. Anand, Org. Biomol. Chem., 2016, 14, 5550–5554 CAS.
-
(a) C. Tsutsui, Y. Yamada, M. Ando, D. Toyama, J. Wu, L. Wang, S. Taketani and T. Kataoka, Bioorg. Med. Chem. Lett., 2009, 19, 4084–4087 CrossRef CAS PubMed;
(b) R. Aslanian, M. wa Mutahi, N.-Y. Shih, J. J. Piwinski, R. West, S. M. Williams, S. She, R.-L. Wu and J. A. Hey, Bioorg. Med. Chem. Lett., 2003, 13, 1959–1961 CrossRef CAS PubMed;
(c) B. Hughes, D. Howat, H. Lisle, M. Holbrook, T. James, N. Gozzard, K. Blease, P. Hughes, R. Kingaby, G. Warrellow, R. Alexander, J. Head, E. Boyd, M. Eaton, M. Perry, M. Wales, B. Smith, R. Owens, C. Catterall, S. Lumb, A. Russell, R. Allen, M. Merriman, D. Bloxham and G. Higgs, Br. J. Pharm., 1996, 118, 1183–1191 CrossRef CAS.
- For recent examples involving p-QM as an electrophile, see:
(a) W. D. Chu, L. F. Zhang, X. Bao, X. H. Zhao, C. Zeng, J. Y. Du, G. B. Zhang, F. X. Wang, X. Y. Ma and C. A. Fan, Angew. Chem., Int. Ed., 2013, 52, 9229–9233 CrossRef CAS PubMed;
(b) L. Caruana, F. Kniep, T. K. Johansen, P. H. Poulsen and K. A. Jørgensen, J. Am. Chem. Soc., 2014, 136, 15929–15932 CrossRef CAS PubMed;
(c) K. Gai, X. Fang, X. Li, J. Xu, X. Wu, A. Lin and H. Yao, Chem. Commun., 2015, 51, 15831–15834 RSC;
(d) A. Lopez, A. Parra, C. Jarava-Barrera and M. Tortosa, Chem. Commun., 2015, 51, 17684–17687 RSC;
(e) Z. Yuan, X. Fang, X. Li, J. Wu, H. Yao and A. Lin, J. Org. Chem., 2015, 80, 11123–11130 CrossRef CAS PubMed;
(f) A. Parra and M. Tortosa, ChemCatChem, 2015, 7, 1524–1526 CrossRef CAS;
(g) Y. Lou, P. Cao, T. Jia, Y. Zhang, M. Wang and J. Liao, Angew. Chem., Int. Ed., 2015, 54, 12134–12138 CrossRef CAS PubMed;
(h) Z. Wang, Y. F. Wong and J. Sun, Angew. Chem., Int. Ed., 2015, 54, 13711–13714 CrossRef CAS PubMed;
(i) C. Jarava-Barrera, A. Parra, A. López, F. Cruz-Acosta, D. Collado-Sanz, D. J. Cárdenas and M. Tortosa, ACS Catal., 2016, 6, 442–446 CrossRef CAS PubMed;
(j) F. S. He, J. H. Jin, Z. T. Yang, X. Yu, J. S. Fossey and W. P. Deng, ACS Catal., 2016, 6, 652–656 CrossRef CAS;
(k) K. Zhao, Y. Zhi, A. Wang and D. Enders, ACS Catal., 2016, 6, 657–660 CrossRef CAS;
(l) Y. Shen, J. Qi, Z. Mao and S. Cui, Org. Lett., 2016, 18, 2722–2725 CrossRef CAS PubMed;
(m) S. Gao, X. Xu, Z. Yuan, H. Zhou, H. Yao and A. Lin, Eur. J. Org. Chem., 2016, 3006–3012 CrossRef CAS;
(n) Y. F. Wong, Z. Wang and J. Sun, J. Org. Biomol. Chem., 2016, 14, 5751–5754 RSC;
(o) X.-Z. Zhang, Y.-H. Deng, X. Yan, K.-Y. Yu, F.-X. Wang, X.-Y. Ma and C.-A. Fan, J. Org. Chem., 2016, 81, 5655–5662 CrossRef CAS PubMed;
(p) X.-Z. Zhang, J.-Y. Du, Y.-H. Deng, W.-D. Chu, X. Yan, K.-Y. Yu and C.-A. Fan, J. Org. Chem., 2016, 81, 2598–2606 CrossRef CAS PubMed;
(q) K. Zhao, Y. Zhi, T. Shu, A. Valkonen, K. Rissanen and D. Enders, Angew. Chem., Int. Ed., 2016, 55, 12104–12108 CrossRef CAS PubMed.
-
(a) P. Hodge, D. W. L. Sung and P. W. Stratford, J. Chem. Soc., Perkin Trans. 1, 1999, 2335 RSC;
(b) M. A. Pericàs, C. I. Herrerías and L. Solà, Adv. Synth. Catal., 2008, 350, 927–932 CrossRef;
(c) J. Rolland, X. C. Cambeiro, C. Rodríquez-Escrich and M. A. Pericàs, Beilstein J. Org. Chem., 2009, 5, 56 Search PubMed;
(d) L. Osorio-Planes, C. Rodríquez-Escrich and M. A. Pericàs, Org. Lett., 2012, 14, 1816–1819 CrossRef CAS PubMed.
Footnote |
† Electronic supplementary information (ESI) available. See DOI: 10.1039/c6ob02277d |
|
This journal is © The Royal Society of Chemistry 2017 |