DOI:
10.1039/C6OB02250B
(Communication)
Org. Biomol. Chem., 2017,
15, 69-75
Deconjugative alkylation/Heck reaction as a simple platform for dihydronaphthalene synthesis†
Received
14th October 2016
, Accepted 10th November 2016
First published on 14th November 2016
Abstract
A simple platform for carbocycle synthesis by Knoevenagel adduct deconjugative alkylation/Heck reaction is described. Deconjugative alkylation of Knoevenagels adducts is two-fold synthetically enabling because C–C bond formation is (1) operationally simple due to the ease of Knoevenagel adduct carbanion generation and (2) results in alkene migration, which poises the substrate for cyclization. Furthermore, the gem-dinitrile moiety serves as a functional group for synthetic manipulation.
Introduction
Knoevenagel adducts have attractive chemical attributes. They are prepared from abundant ketones and malonic acid derivatives by an eco-friendly condensation reaction.1 Of specific interest to this work, they have highly acidic γ-C–H bonds, which allow for operationally simple activation to their respective allyl carbanions for coupling reactions.2–4 Upon deconjugative alkylation (γ-deprotonation/α-alkylation),2 the alkylidene-olefin cannot re-conjugate unlike mono-electron-withdrawing group analogues (i.e. when E2 = H) (Scheme 1). A synthetic platform that takes advantage of the dual nature of deconjugative alkylation (C–C bond formation and olefin migration) for carbocyclization via a broadly defined “pairing” reaction has the potential to be a straightforward approach to the synthesis of useful chemical architectures. On this line, the malonic acid moiety, which is critical for implementing the chemistry, is situated within the carbocycle and would serve as a useful functional group for further manipulation to target structures.
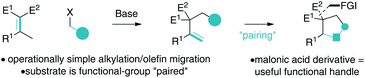 |
| Scheme 1 General strategy for carbocycle synthesis. | |
We4 recently disclosed that Knoevenagel adducts can behave as trimethylenemethane dipole surrogates for reaction with electrophiles and nucleophiles in a 3-component fashion. In continued studies, we reasoned that aryl acetone-derived Knoevenagel adducts 1 and o-bromo-benzylic electrophiles 2 could react as described in Scheme 1 to yield useful cyclic scaffolds. As described in Scheme 2, Knoevenagel adduct 1 could undergo directed deconjugative alkylation (by deprotonation of the most acidic γ-C–H as shown to Ia)2 with o-bromo-benzylic electrophiles 2 yielding 3. The deconjugative alkylation process directly pairs the substrate for intramolecular Heck cyclization, ideally favouring a less common 6-endo-trig5–7 reaction pathway (over 5-exo-trig) to yield aryl dihydronaphthalene 4 (Scheme 2). Notably, related aryltetralins and naphthalenes have significant biological activities and medicinal relevance.8–12 If achieved, the proposed tetralin scaffolds 4 will bear a gem-dinitrile moiety, which will allow for access to unexplored nitrile-containing aryl tetralin analogues 5 and 6 by utilization of reductive13,14 or dehydrocyanation protocols.15,16 From a medicinal chemistry perspective, nitrile inclusion would be beneficial for the following reasons: (a) a nitrile is sterically small (A value = 0.17), (b) has noted metabolic stability, (c) changes the lipophilicity of the molecule, and (d) is isosteric to a halogen.17
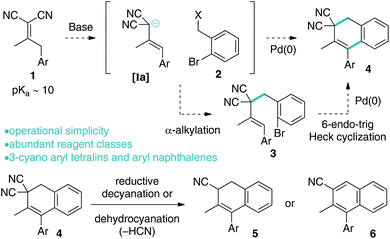 |
| Scheme 2 General hypothesis for carbocycle synthesis. | |
Related bicyclic scaffolds are commonly prepared by intramolecular Friedel–Crafts alkylation with a benzylic electrophile, or by a Diels–Alder cycloaddition of o-xylylenes generated from various precursors.18–20 The former route is considered biomimetic.19 Alternatively, 1-arylation of preformed bicycles (tetralins, naphthalenes, or related) is also common by organometallic addition, C–H arylation, or other methods.21 There are other unique cyclization strategies,22 however, of interest to the work herein, is aryl tetralin synthesis by intramolecular Heck cyclization,5d,7 which is poorly examined.
The proposed strategy is a unique platform for tetralin synthesis, utilizes abundant reagent classes as sole carbon sources (ketone + malononitrile = Knoevenagel adduct; benzylic electrophiles), has the potential to be operationally simple due to the ease of Knoevenagel adduct anion generation, and would result in structurally unique 3-cyano aryl dihydronaphthalenes 5 and aryl naphthalenes 6. For these reasons we wished to examine the possibility of this hypothesis.
Development and scope of the strategy
We chose to examine Knoevenagel adduct 1a, prepared from p-methoxyphenylacetone and malononitrile by the Cope–Knoevenagel procedure,1c and o-bromobenzyl iodide 2a, prepared from commercial 6-bromoveratraldehyde, as our initial reagents for formal [3 + 3]-cyclization (Scheme 3). To begin, we examined the deconjugative alkylation2 reaction and found the reaction was high yielding under standard alkylation conditions (NaH, DMF). Notably, the Knoevenagel adduct's aromatic group directs the deprotonation allowing for the desired styrene-moiety to be synthesized upon α-benzylation.
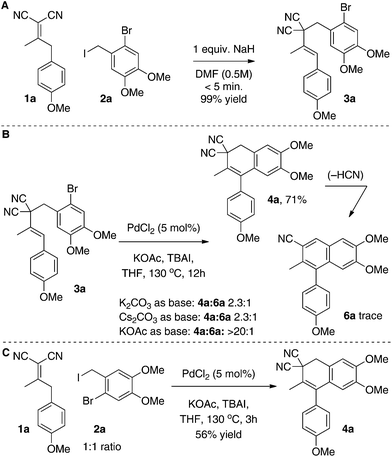 |
| Scheme 3 Development of the formal [3 + 3] annulation strategy. | |
We next examined the key intramolecular Heck cyclization and found that “Jeffery” conditions23,24 worked best with an optimized yield of 71% for dihydronaphthalene 4a (Scheme 3B). We were pleased with this result as relatively inexpensive PdCl2 (5 mol%) could be used as a catalyst in the presence of TBAI as an additive. That said, a variety of other conditions were attempted and are summarized in the ESI.† In brief, it appears that in our hands various Pd-phosphine catalyst-combinations were poorly reactive and only when ligandless conditions (“Jeffery conditions”) were employed did we begin to observe the desired product 4a. Interestingly, various bases were screened during the optimization and we observed byproduct cyanonaphthalene 6a in some cases. The byproduct is derived from the product 4a by a base-promoted dehydrocyanation.15,16 For example, when the more basic carbonate bases (H2O pKa ∼ 10) were used in lieu of KOAc (H2O pKa ∼ 4), a significant amount of 6a was observed (22% isolated yield). As the final point, we were also able to perform the γ-deprotonation/α-benzylation/Heck cyclization sequence in one-pot (56% yield from 1a and 2a). However, we ultimately decided to examine the scope over two steps as this yield was higher and isolation of benzylated intermediate 3 is simple.
We were pleased to find that a variety of substituted aryl tetralins 4a–4h could be prepared by the formal [3 + 3]-cyclization strategy (Scheme 4). Generally speaking the deconjugative benzylation reactions were excellent and the Heck reaction worked best when both aromatic rings were electron rich. For example, the yield was decreased for 4d due to significant formation of its naphthalene byproduct (via dehydrocyanation). To reiterate, the electronics of the tetralin play a key role in favouring either product 4 or byproduct 6 under the reaction conditions. The reaction was not limited to arylacetone-derived Knoevenagel adducts as we were able to utilize a 1,3-diphenyl-ketone Knoevenagel adduct to prepare the tetralin 4f. We also wished to explore the scalability. As is common with Jeffrey conditions, we were able to reduce the catalyst loading on the 5 gram scale when preparing tetralin 4h.24 Excitingly, the reaction works excellently on the large scale and ample material was prepared for further substrate processing.
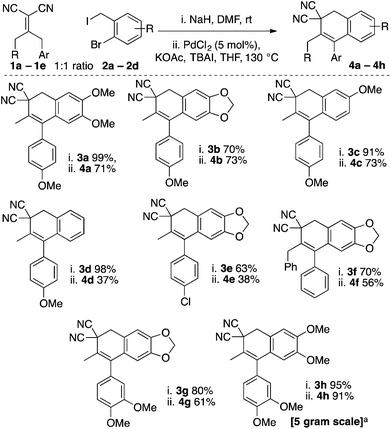 |
| Scheme 4 Scope of 1-aryl tetralin synthesis. aPdCl2 (2.5 mol%), KOAc (5 equiv.), TBAI (THF), THF (0.75 M), 130 °C, 5. | |
We also examined the cyclohexanone-derived substrate 3i (eqn (1)). Unfortunately, only decomposition was observed using the standard protocol. The acetophenone-derived molecule 3j was a competent coupling partner, albeit in modest yield under the conditions optimized for arylacetone-derived substrates (eqn (2)). Interestingly, the tetralin was not observed and we were only able to isolate the 2-phenylnaphthalene product 4j. If further examined this could be a powerful strategy to prepare highly substituted naphthalenes from acetophenones, malononitrile, and benzylic electrophiles.
Considering the results from our initial scope studies (Scheme 4) and the results in eqn (1) and (2), we wondered if the γ-deprotonation/α-alkylation could be directed by sterics to prepare other uniquely substituted tetralin cores (Scheme 5). In this regard we found that a variety of γ,γ-disubstituted Knoevenagel adducts could afford the desired tetralin products 4k–4q in modest to good yields. For example, products 4k–4l are derived from isopropyl methyl ketone, 4m–4p are derived pyruvaldehyde dimethyl acetal, and 4q is derived from acetoin.
| 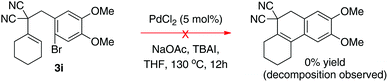 | (1) |
| 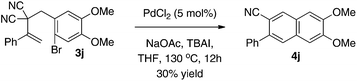 | (2) |
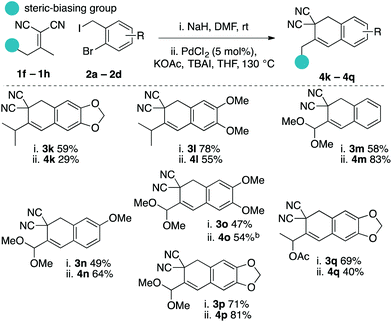 |
| Scheme 5 Sterically-biased formal [3 + 3]-cyclizations. | |
Having established that a variety of tetralin cores bearing a gem-dicyano group can be prepared, we next examined two different strategies to mono-decyanate the scaffolds; reductive decyanation13,14 and base-promoted dehydrocyanation (E2 reaction) (Schemes 6 and 7, respectively).15,16 We were able to realize a reductive decyanation reaction yielding 3-cyano aryl tetralins when using either lithium naphthalenide14c (LN) or samarium(II) iodide14e (SmI2) (Scheme 6). Using LN, mono-decyanation was observed on a variety of scaffolds (4b, 4c, 4e) preparing cyanotetralins 6a–6c. One outlier substrate was 4h, where decyanation conditions using LN produced only double decyanation product 6d in modest yield. At this point, other reductive conditions were examined and we found that SmI2
16e was an excellent reagent for mono-decyanation of these substrates producing 6e in 74% isolated yield.
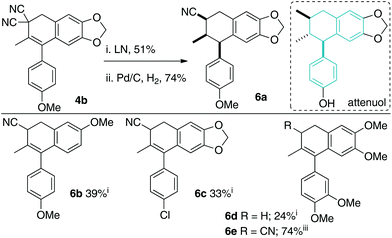 |
| Scheme 6 Reductive decyanation produces nitrile-containing analogues of aryl tetralin natural products. (i) 3 equiv. LN, THF, −78 °C, (ii) Pd/C H2 (1 atm), EtOAc, (iii) 2 equiv. SmI2, 4 equiv. MeOH, THF : DMPU 1 : 15, 0 °C. | |
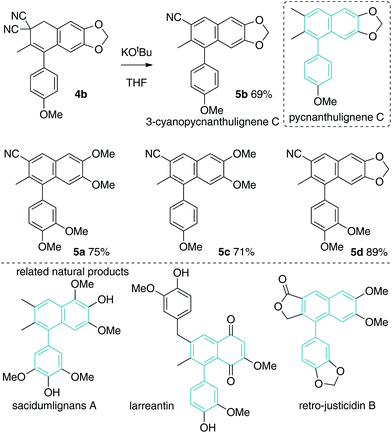 |
| Scheme 7 Retro-hydrocyanation produces nitrile-containing analogues of aryl naphthalene natural products. | |
The molecules prepared in Scheme 5 are unnatural nitrile-containing analogues of attenuol (Scheme 5),23 magnoshinin (Fig. 1),24 and other related 2,3-dimethyl aryl tetralins.25 Such natural products have a variety of biological activities.23–26 Replacement of a methyl group with a nitrile is unprecedented both naturally and synthetically but could have interesting effects on structure–activity relationships.15
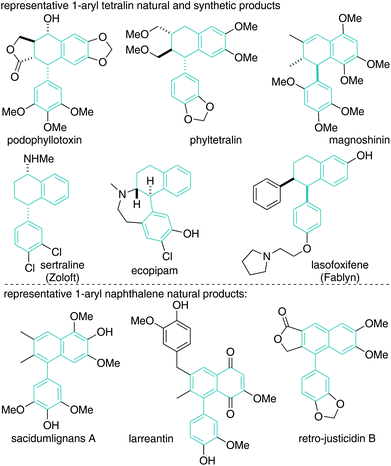 |
| Fig. 1 Natural products and synthetic molecules of interest to this study. | |
The second decyanation reaction examined was base-promoted dehydrocyanation leading to 3-cyano aryl naphthalenes 5a–5d (Scheme 7).27,28 Cyanide is not a common leaving group, but due to the aromaticity driving force, the reaction was found to be facile under simple conditions (1.1 equiv. KOtBu, THF, 0 °C). As above, the synthetic strategy results in medicinally relevant cyano-containing analogues of 1-aryl naphthalene natural products. The parent 1-aryl-napthalene core is found in many bioactive natural products.27,28
The gem-dinitrile moiety could also react with alcohols under Pinner reaction29 conditions to yield cyanoacetic esters 7a and 7b following hydrolysis (Scheme 8). Notably, synthesis of the allyl cyanoacetate 7b allowed us to utilize decarboxylative allylation (DcA) to install an allyl group onto scaffold 7c.30 We also examined oxidative protocols for the naphthalene scaffolds and found that radical benzylic bromination to 7d was high yielding. The halogen could then serve as a functional handle for the incorporation of nucleophiles (e.g. acetate, 7e) or allow further oxidation to an aldehyde (the Sommelet protocol was utilized, 7f).31
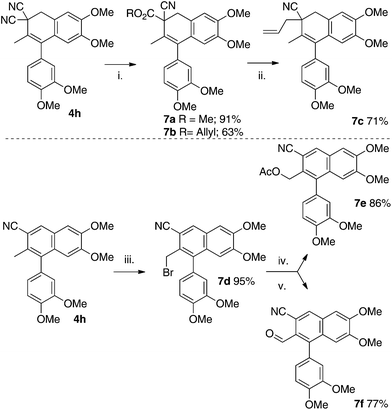 |
| Scheme 8 Other scaffold manipulations. (i) MeOH or AllylOH, 10 equiv. K2CO3, 0 °C, 5 min, (ii) Pd(PPh3)4 (1 mol%), Tol, 60 °C, 3 h, (iii) NBS (10 equiv.), AIBN (0.1 equiv.), CCl4, 90 °C, 1 h, (iv) K2CO3 (4 equiv.) AcOH (4 equiv.), DMF, rt, (v) hexamethylenetetramine (1.5 equiv.), CHCl3, 63 °C, 40 min. AcOH. | |
Conclusions
We have devised a platform for carbocycle synthesis that utilizes Knoevenagel adducts as conjunctive reagents. Knoevenagel adducts can react with bis-electrophilic o-bromobenzyl iodides allowing for formal [3 + 3]-cyclization by deconjugative benzylation then 6-endo-trig Heck cyclization. The developed cyclization uses abundant carbon-sources, is operationally simple, and yields useful carbocyclic frameworks. We describe the synthesis of previously unknown 3-cyano aryl tetralin and naphthalenes, which are being analysed for biological activity. Future synthetic efforts are aimed at designing related cyclization reactions for Knoevenagel adducts based on the general reaction protocol outlined in Scheme 1.
Acknowledgements
We thank the College of Liberal Arts and Sciences and the Department of Chemistry at the University of Florida for start-up funds.
Notes and references
-
(a) G. Jones, Org. React., 1967, 15, 204–599 CAS;
(b) E. Knoevenagel, Berichte der Dtsch. Chem. Gesellschaft, 1898, 31, 2596–2619 Search PubMed;
(c) A. C. Cope, J. Am. Chem. Soc., 1937, 59, 2327–2330 Search PubMed.
- Knoevenagel adducts can undergo deconjugative alkylation:
(a) W.-B. Liu, N. Okamoto, E. J. Alexy, A. Y. Hong, K. Tran and B. M. Stoltz, J. Am. Chem. Soc., 2016, 138, 5234 Search PubMed;
(b) S. R. Waetzig, D. K. Rayabharapu, J. D. Weaver and J. A. Tunge, Angew. Chem., Int. Ed., 2006, 45, 4977–4980 CrossRef CAS PubMed;
(c) M. Bell, K. Frisch and K. A. Jørgensen, J. Org. Chem., 2006, 71, 5407–5410 Search PubMed;
(d) Y. Sato, Y. Oonishi and M. Mori, J. Org. Chem., 2003, 68, 9858–9860 Search PubMed;
(e) H. Nakamura, H. Iwama, M. Ito and Y. Yamamoto, J. Am. Chem. Soc., 1999, 121, 10850–10851 CrossRef CAS;
(f) H. Karlsen, P. H. Songe, L. K. Sunsby, L. C. Hagen, P. Kolsaker and C. Romming, J. Chem. Soc., Perkin Trans., 2001, 497–507 Search PubMed;
(g) R. B. Grossman and M. A. Varner, J. Org. Chem., 1997, 62, 5235–5237 Search PubMed;
(h) A. C. Cope and K. E. Hoyle, J. Am. Chem. Soc., 1941, 63, 733–736 Search PubMed.
- Knoevenagel adducts can undergo γ-alkylation, commonly with Michael acceptors:
(a) T. B. Poulsen, C. Alemparte and K. A. Jørgensen, J. Am. Chem. Soc., 2005, 127, 11614–11615 Search PubMed;
(b) D. Xue, Y.-C. Chen, Q.-W. Wang, L.-F. Cun, J. Zhu and J.-G. Deng, Org. Lett., 2005, 7, 5293–5296 Search PubMed;
(c) T.-Y. Liu, H.-L. Cui, J. Long, B.-J. Li, Y. Wu, L.-S. Ding and Y.-C. Chen, J. Am. Chem. Soc., 2007, 129, 1878–1879 CrossRef CAS PubMed;
(d) T. B. Poulsen, M. Bell and K. A. Jørgensen, Org. Biomol. Chem., 2006, 4, 63–70 Search PubMed;
(e) B. Niess and K. A. Joergensen, Chem. Commun., 2007, 1620–1622 Search PubMed;
(f) J.-W. Xie, L. Yue, D. Xue, X.-L. Ma, Y.-C. Chen, Y. Wu, J. Zhu and J.-G. Deng, Chem. Commun., 2006, 1563–1565 Search PubMed;
(g) J.-W. Xie, W. Chen, R. Li, M. Zeng, W. Du, L. Yue, Y.-C. Chen, Y. Wu, J. Zhu and J.-G. Deng, Angew. Chem., Int. Ed., 2007, 46, 389–392 CrossRef CAS PubMed;
(h) J. Aleman, C. B. Jacobsen, K. Frisch, J. Overgaard and K. A. Jorgensen, Chem. Commun., 2008, 632–634 Search PubMed;
(i) J. Lu, F. Liu and T.-P. Loh, Adv. Synth. Catal., 2008, 350, 1781–1784 CrossRef CAS;
(j) X.-L. Zhu, W.-J. He, L.-L. Yu, C.-W. Cai, Z.-L. Zuo, D.-B. Qin, Q.-Z. Liu and L.-H. Jing, Adv. Synth. Catal., 2012, 354, 2965–2970 Search PubMed.
- We are exploring Knoevenagel adducts as reagents for multifunctionalization: P. Vertesaljai, P. V. Navaratne and A. J. Grenning, Angew. Chem., Int. Ed., 2016, 55, 317–320 Search PubMed.
- For examples of 6-endo-Heck cyclization
see:
(a) X. Dong, Y. Han, F. Yan, Q. Liu, P. Wang, K. Chen, Y. Li, Z. Zhao, Y. Dong and H. Liu, Org. Lett., 2016, 18, 3774–3777 Search PubMed;
(b) J. M. Aurrecoechea, C. A. Coy and O. J. Patino, J. Org. Chem., 2008, 73, 5194–5197 Search PubMed;
(c) J. W. Dankwardt and L. A. Flippin, J. Org. Chem., 1995, 60, 2312–2313 Search PubMed;
(d) H. Ishibashi, K. Ito, T. Hirano, M. Tabuchi and M. Ikeda, Tetrahedron, 1993, 49, 4173–4182 Search PubMed.
- For a review of intramolecular Heck cyclization see:
(a) J. T. Link, Org. React., 2002, 60, 157–561 Search PubMed;
(b) A. B. Dounay and L. E. Overman, Chem. Rev., 2003, 103, 2945–2963 Search PubMed.
- For other examples of tetralin synthesis by 6-endo-Heck cyclization see:
(a) D. Stadler and T. Bach, Angew. Chem., Int. Ed., 2008, 47, 7557–7559 Search PubMed;
(b) J. J. Kennedy-Smith, L. A. Young and F. D. Toste, Org. Lett., 2004, 6, 1325–1327 Search PubMed.
- Bioactive tetralin lignans:
(a) M. Saleem, H. J. Kim, M. S. Ali and Y. S. Lee, Nat. Prod. Rep., 2005, 22, 696–716 Search PubMed;
(b) S. Apers, A. Vlietinck and L. Pieters, Phytochem. Rev., 2004, 2, 201–207 CrossRef;
(c) C. Canel, R. M. Moraes, F. E. Dayan and D. Ferreira, Phytochemistry, 2000, 54, 115–120 Search PubMed;
(d) R. S. Ward, Nat. Prod. Rep., 1999, 16, 75–96 RSC;
(e) W. D. MacRae and G. H. N. Towers, Phytochemisrty, 1984, 23, 1207–1220 CrossRef CAS.
- Sertraline: D. Murdoch and D. McTavish, Drugs, 1992, 44, 604–624 Search PubMed.
- Ecopipam: E. F. McCance-Katz, T. A. Kosten and T. R. Kosten, Psychopharmacology, 2001, 155, 327–329 Search PubMed; M. Haney, A. S. Ward, R. W. Foltin and M. W. Fischman, Psychopharmacology, 2001, 155, 330–337 Search PubMed.
- Lasofoxifene: S. Mocellin, P. Pilati, M. Briarava and D. Nitti, J. Natl. Cancer Inst., 2016, 108 Search PubMed , djv318; L. Gennari, D. Merlotti, G. Martini and R. Nuti, Expert Opin. Invest. Drugs, 2006, 15, 1091–1103 CrossRef CAS PubMed.
- Naphthalene lignans:
(a) D. L. Minor, S. D. Wyrick, P. S. Charifson, V. J. Watts, D. E. Nichols and R. B. Mailman, J. Med. Chem., 1994, 37, 4317–4328 Search PubMed;
(b) P. Abrams and K.-E. Andersson, BJU Int., 2007, 100, 987–1006 CrossRef CAS PubMed.
- For a review of reductive decyanation see: J.-M. Mattalia, C. Marchi-Delapierre, H. Hazimeh and M. Chanon, ARKIVOC, 2006, 90–118 Search PubMed.
- For select examples of reductive decyanation see:
(a) J. T. Reeves, C. A. Malapit, F. G. Buono, K. P. Sidhu, M. A. Marsini, C. A. Sader, K. R. Fandrick, C. A. Busacca and C. H. Senanayake, J. Am. Chem. Soc., 2015, 137, 9481–9488 Search PubMed;
(b) E. Doni and J. A. Murphy, Org. Chem. Front., 2014, 1, 1072–1076 Search PubMed;
(c) J.-P. Tsao, T.-Y. Tsai, I.-C. Chen, H.-J. Liu, J.-L. Zhu and S.-W. Tsao, Synthesis, 2010, 4242–4250 Search PubMed;
(d) S. D. Rychnovsky and L. R. Takaoka, Angew. Chem., Int. Ed., 2003, 42, 818–820 Search PubMed;
(e) H.-Y. Kang, W. Sang Hong, Y. Seo Cho and H. Yeong Koh, Tetrahedron Lett., 1995, 36, 7661–7664 Search PubMed;
(f) D. Guijarro and M. Yus, Tetrahedron, 1994, 50, 3447–3452 Search PubMed;
(g) D. P. Curran and C. M. Seong, Synlett, 1991, 107–108 Search PubMed.
- For a review of arene synthesis by dehydrocyanation see: N. Otto and T. Opatz, Chem. – Eur. J., 2014, 20, 13064–13077 Search PubMed.
- For select examples of dehydrocyanation see:
(a) M. M. Nebe, M. Kucukdisli and T. Opatz, J. Org. Chem., 2016, 81, 4112–4121 Search PubMed;
(b) A.-K. Bachon and T. Opatz, J. Org. Chem., 2016, 81, 1858–1869 Search PubMed;
(c) N. A. Mir, S. Choudhary, P. Ramaraju, D. Singh and I. Kumar, RSC Adv., 2016, 6, 39741–39749 Search PubMed;
(d) G. Lahm, J.-G. Deichmann, A. L. Rauen and T. Opatz, J. Org. Chem., 2015, 80, 2010–2016 Search PubMed;
(e) D. M. Gale and S. C. Cherkofsky, J. Org. Chem., 1973, 38, 475–478 Search PubMed;
(f) C. R. Hauser and W. R. Brasen, J. Am. Chem. Soc., 1956, 78, 82–83 Search PubMed.
-
(a) L. H. Jones, N. W. Summerhill, N. A. Swain and J. E. Mills, MedChemComm, 2010, 1, 309–318 Search PubMed;
(b) F. F. Fleming, L. Yao, P. C. Ravikumar, L. Funk and B. C. Shook, J. Med. Chem., 2010, 53, 7902–7917 CrossRef CAS PubMed.
- For reviews of aryl tetralin synthesis see:
(a) J. D. Sellars and P. G. Steel, Eur. J. Org. Chem., 2007, 3815–3828 Search PubMed;
(b) J.-S. Sun, H. Liu, X.-H. Guo and J.-X. Liao, Org. Biomol. Chem., 2016, 14, 1188–1200 RSC.
- For select examples of aryl tetralin synthesis by intramolecular Friedel–Crafts alkylation see:
(a) S. Hajra, B. Maji and D. Mal, Adv. Synth. Catal., 2009, 351, 859–864 Search PubMed;
(b) B. L. Yvon, P. K. Datta, T. N. Le and J. L. Charlton, Synthesis, 2001, 1556–1560 Search PubMed;
(c) J. E. Cochran and A. Padwa, J. Org. Chem., 1995, 60, 3938–3939 Search PubMed;
(d) A. Pelter, R. S. Ward and R. R. Rao, Tetrahedron, 1985, 41, 2933–2938 Search PubMed;
(e) A. S. Kende, L. S. Liebeskind, J. E. Mills, P. S. Rutledge and D. P. Curran, J. Am. Chem. Soc., 1977, 99, 7082–7083 CrossRef CAS PubMed.
- For select examples of aryl tetralin synthesis by a Diels–Alder-centered strategy see:
(a) M. Jinno, Y. Kitano, M. Tada and K. Chiba, Org. Lett., 1999, 1, 435–437 Search PubMed;
(b) T. Kuroda, M. Takahashi, K. Kondo and T. Iwasaki, J. Org. Chem., 1996, 61, 9560–9563 CrossRef CAS;
(c) D. M. Coltart and J. L. Charlton, Can. J. Chem., 1996, 74, 88–94 Search PubMed;
(d) S. P. Maddaford and J. L. Charlton, J. Org. Chem., 1993, 58, 4132–4138 Search PubMed;
(e) J. L. Charlton, G. L. Plourde, K. Koh and A. S. Secco, Can. J. Chem., 1990, 68, 2022–2027 Search PubMed;
(f) M. B. Glinski and T. Durst, Can. J. Chem., 1983, 61, 573–575 Search PubMed;
(g) D. Rajapaksa and R. Rodrigo, J. Am. Chem. Soc., 1981, 103, 6208–6209 Search PubMed.
-
(a) C. P. Ting and T. J. Maimone, Angew. Chem., Int. Ed., 2014, 53, 3115–3119 Search PubMed;
(b) M. Davoust, J. A. Kitching, M. J. Fleming and M. Lautens, Chem. – Eur. J., 2010, 16, 50–54 Search PubMed;
(c) C. V. Kavitha, K. Mantelingu, G. Sarala, S. Naveen, S. M. Anandalwar, J. S. Prasad and K. S. Rangappa, J. Chem. Res., 2006, 730–732 Search PubMed;
(d) A. J. Reynolds, A. J. Scott, C. I. Turner and M. S. Sherburn, J. Am. Chem. Soc., 2003, 125, 12108–12109 Search PubMed;
(e) R. C. Andrews, S. J. Teague and A. I. Meyers, J. Am. Chem. Soc., 1988, 110, 7854–7858 Search PubMed.
-
(a) M.-Y. Chang and Y.-C. Cheng, Org. Lett., 2016, 18, 1682–1685 Search PubMed;
(b) E. S. Gutman, V. Arredondo and D. L. Van Vranken, Org. Lett., 2014, 5464–5467 Search PubMed;
(c) S. M. Miles, S. P. Marsden, R. J. Leatherbarrow and W. J. Coates, Chem. Commun., 2004, 2292–2293 Search PubMed;
(d) V. Nair, R. Rajan and N. P. Rath, Org. Lett., 2002, 4, 1575–1577 Search PubMed;
(e) A. S. Kende, M. L. King and D. P. Curran, J. Org. Chem., 1981, 46, 2826–2828 CrossRef CAS.
-
(a) T. Jeffery, Tetrahedron Lett., 1985, 26, 2667–2670 Search PubMed;
(b) T. Jeffery, J. Chem. Soc., Chem. Commun., 1984, 1287–1289 Search PubMed.
-
(a) M. T. Reetz and J. G. de Vries, Chem. Commun., 2004, 1559–1563 Search PubMed;
(b) M. T. Reetz, E. Westermann, R. Lohmer and G. Lohmer, Tetrahedron Lett., 1998, 39, 8449–8452 Search PubMed.
-
(a) M. Loriot, E. Brown and J. P. Robin, Tetrahedron, 1983, 39, 2795–2798 Search PubMed;
(b) B. S. Joshi, N. Viswanathan, V. Balakrishnan, D. H. Gawad and K. R. Ravindranath, Tetrahedron, 1979, 35, 1665–1671 Search PubMed.
-
(a) K. Takahashi, H. Kobayashi, S. Kobayashi, I. Kimura, K. Terasawa and M. Kimura, Phytother. Res., 1996, 10, 42–48 Search PubMed;
(b) M. Kimura, S. Kobayashi, B. Luo and I. Kimura, Agents Actions Suppl., 1991, 32, 197–201 Search PubMed;
(c) M. Kimura, J. Suzuki, T. Yamada, M. Yoshizaki, T. Kikuchi, S. Kadota and S. Matsuda, Planta Med., 1985, 291–293 CrossRef CAS PubMed.
-
(a) Y. Li, W. Cheng, C. Zhu, C. Yao, L. Xiong, Y. Tian, S. Wang, S. Lin, J. Hu, Y. Yang, Y. Guo, Y. Yang, Y. Li, Y. Yuan, N. Chen and J. Shi, J. Nat. Prod., 2011, 74, 1444–1452 Search PubMed;
(b) W. Cheng, C. Zhu, W. Xu, X. Fan, Y. Yang, Y. Li, X. Chen, W. Wang and J. Shi, J. Nat. Prod., 2009, 72, 2145–2152 Search PubMed;
(c) M. K. Lee, H. Yang, C. J. Ma and Y. C. Kim, Biol. Pharm. Bull., 2007, 30, 814–817 Search PubMed;
(d) H. Li, L. Wang, Z. Yang and S. Kitanaka, J. Nat. Prod., 2007, 70, 1999–2002 Search PubMed;
(e) C. J. Ma, S. R. Kim, J. Kim and Y. C. Kim, Br. J. Pharmacol., 2005, 146, 752–759 Search PubMed;
(f) C. J. Ma, S. H. Sung and Y. C. Kim, Planta Med., 2004, 70, 79–80 CrossRef CAS PubMed;
(g) J. S. Lee, J. Kim, Y. U. Yu and Y. C. Kim, Arch. Pharmacal Res., 2004, 27, 1043–1047 Search PubMed;
(h) D. H. S. Silva, F. C. Pereira, M. V. B. Zanoni and M. Yoshida, Phytochemistry, 2001, 57, 437–442 CrossRef CAS PubMed.
-
(a) Y. Ren, D. D. Lantvit, Y. Deng, R. Kanagasabai, J. C. Gallucci, T. N. Ninh, H.-B. Chai, D. D. Soejarto, J. R. Fuchs, J. C. Yalowich, J. Yu, S. M. Swanson and A. D. Kinghorn, J. Nat. Prod., 2014, 77, 1494–1504 CrossRef CAS PubMed;
(b) F. Bailly, R.-A. Toillon, O. Tomavo, N. Jouy, H. Hondermarck and P. Cotelle, Bioorg. Med. Chem. Lett., 2013, 23, 574–578 Search PubMed;
(c) D. Janmanchi, Y. P. Tseng, K.-C. Wang, R. L. Huang, C. H. Lin and S. F. Yeh, Bioorg. Med. Chem., 2010, 18, 1213–1226 CrossRef CAS PubMed;
(d) E. C. N. Nono, P. Mkounga, V. Kuete, K. Marat, P. G. Hultin and A. E. Nkengfack, J. Nat. Prod., 2010, 73, 213–216 Search PubMed;
(e) J. Dai, Y. Liu, Y.-D. Zhou and D. G. Nagle, J. Nat. Prod., 2007, 70, 1824–1826 Search PubMed;
(f) L.-S. Gan, S.-P. Yang, C.-Q. Fan and J.-M. Yue, J. Nat. Prod., 2005, 68, 221–225 Search PubMed;
(g) H. Yeo, Y. Li, L. Fu, J.-L. Zhu, E. A. Gullen, G. E. Dutschman, Y. Lee, R. Chung, E.-S. Huang, D. J. Austin and Y.-C. Cheng, J. Med. Chem., 2005, 48, 534–546 Search PubMed;
(h) J. S. Albert, C. Ohnmacht, P. R. Bernstein, W. L. Rumsey, D. Aharony, Y. Alelyunas, D. J. Russell, W. Potts, S. A. Sherwood, L. Shen, R. F. Dedinas, W. E. Palmer and K. Russell, J. Med. Chem., 2004, 47, 519–529 Search PubMed;
(i) K. S. Sagar, C.-C. Chang, W.-K. Wang, J.-Y. Lin and S.-S. Lee, Bioorg. Med. Chem., 2004, 12, 4045–4054 Search PubMed;
(j) E. Navarro, S. J. Alonso, J. Trujillo, E. Jorge and C. Pérez, J. Nat. Prod., 2001, 64, 134–135 Search PubMed;
(k) T. Ukita, Y. Nakamura, A. Kubo, Y. Yamamoto, M. Takahashi, J. Kotera and T. Ikeo, J. Med. Chem., 1999, 42, 1293–1305 Search PubMed;
(l) M. Therien, B. J. Fitzsimmons, J. Scheigetz, D. Macdonald, L. Y. Choo, J. Guay, J. P. Falgueyret and D. Riendeau, Bioorg. Med. Chem. Lett., 1993, 3, 2063–2066 CrossRef CAS.
- R. Roger and D. G. Neilson, Chem. Rev., 1961, 61, 179–211 CrossRef CAS.
-
(a) A. Recio and J. A. Tunge, Org. Lett., 2009, 11, 5630–5633 Search PubMed;
(b) J. D. Weaver, A. Recio, A. J. Grenning and J. A. Tunge, Chem. Rev., 2011, 111, 1846–1913 Search PubMed.
- E. E. Campaigne, R. C. Bourgeois and W. C. McCarthy, Org. Synth., 1953, 33, 93–94 Search PubMed.
Footnote |
† Electronic supplementary information (ESI) available. See DOI: 10.1039/c6ob02250b |
|
This journal is © The Royal Society of Chemistry 2017 |
Click here to see how this site uses Cookies. View our privacy policy here.