DOI:
10.1039/C6MD00509H
(Research Article)
Med. Chem. Commun., 2017,
8, 135-147
Synthesis and biological evaluation of memantine nitrates as a potential treatment for neurodegenerative diseases†‡
Received
5th September 2016
, Accepted 18th October 2016
First published on 20th October 2016
Abstract
A series of memantine nitrate derivatives, as dual functional compounds with neuroprotective and vasodilatory activity for neurodegenerative diseases, was designed and synthesized. These compounds combined the memantine skeleton and a nitrate moiety, and thus inhibited the N-methyl-D-aspartic acid receptor and released NO in the central nervous system. The biological evaluation results revealed that the new memantine nitrates were effective in protecting neurons against glutamate-induced injury in vitro. Moreover, memantine nitrates dilated aortic rings against phenylephrine-induced contraction. The structure–activity relationships of neuroprotection and vasodilation were both analyzed. In further studies, compound MN-05 significantly protected cortical neurons by inhibiting Ca2+ influx, reducing free radical production and maintaining the mitochondrial membrane potential. Further research on MN-05 is warranted.
Introduction
N-Methyl-D-aspartic acid (NMDA) receptors, a subtype of glutamate receptor, play a crucial role in regulating a broad spectrum of processes in the central neuron system (CNS).1,2 Under pathological conditions, the presence of excessive glutamate overstimulates the NMDA receptors, resulting in extensive cell damage,2,3 and leads to a number of chronic neurodegenerative disorders, such as Alzheimer's disease, Parkinson's disease, vascular dementia (VaD) etc.4–7 Memantine (1-amino-3,5-dimethyladamantane, Fig. 1) is an open-channel, uncompetitive NMDA receptor blocker with low affinity, high voltage dependence and fast receptor kinetics.8–10 Memantine rapidly penetrates into the brain to bind to NMDA receptors and block the subsequent influx of calcium, affording neuronal protection.10–12 Memantine is well tolerated clinically and has been approved as a treatment for moderate to severe Alzheimer's disease around the world.13 Recently, memantine was reported to reduce the blood supply of the brain possibly through non-specific blocking of the nicotinic acetylcholine receptors in the CNS.14 This property of memantine may decrease its clinical efficacy since some CNS disorders, such as ischemic stroke and VaD, are characterized by reduced cerebral blood flow (CBF).
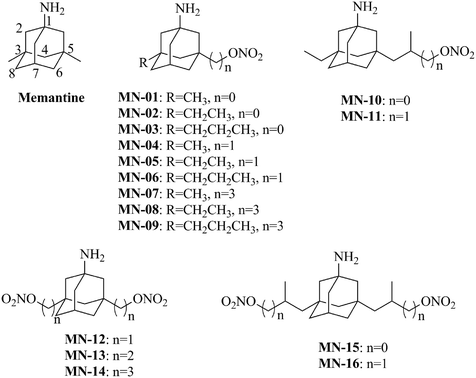 |
| Fig. 1 Structures of memantine and new memantine nitrates. | |
Nitric oxide (NO), a second messenger with high reactivity, plays an important role in the cardio-cerebrovascular system. It also participates in maintaining the normal function of the CNS and regulating neuronal death.15 Physiological concentrations of NO elicit anti-apoptotic/pro-survival effects against various neurotoxic challenges and brain insults through multiple mechanisms.16–18 It has been reported that NO donors, such as nitroglycerin, significantly reduce NMDA-induced neurotoxicity in cortical cultures and in vivo.18,19 Although nitroglycerin confers neuroprotective effects, it is not an ideal neuroprotectant because of its systematic release of NO in peripheral blood vessels, resulting in a high risk of hypotension. NO donors which release NO targeting brain tissue have great potential for the treatment of vascular neurodegenerative disease.
Based on these findings, Wang et al. have previously designed and synthesized a number of memantine nitrates to increase the neuroprotective effects of memantine and to avoid the peripheral hypotensive effects of traditional NO donors.20 Their stability in serum, electrophysiological properties and effects on blood vessels were characterized.20 It was found that memantine nitrates exerted a dual inhibitory effect on NMDA receptors, i.e., an open-channel blockage of the NMDA receptor by the memantine moiety and inhibition via S-nitrosylation by NO released from the nitrate group. Further study demonstrated that the memantine moiety was able to target NO to NMDA receptors in brain tissue, and thus reduced the risk of hypotension.21 Among the memantine nitrates, YQW-036, bearing two ethyl side groups, was extensively studied. YQW-036 displayed improved efficacy and safety in an animal model of cerebral ischemia.21 Although these memantine nitrates exert a dual inhibitory effect on NMDA receptors, they didn't display stronger activity than memantine for the inhibition of the NMDA current and have short half-lives.20 To find compounds with improved stability and better efficacy, in the current study, a series of new memantine nitrates were designed and synthesized (Fig. 1). The structure–activity relationships of the compounds were analyzed. Their neuroprotective and vasodilating effects were also investigated in vitro.
Results and discussion
Rational drug design and synthesis
The significant characteristics of previously synthesized memantine nitrates are that the nitrate group was introduced at the 7-position of the amantadine skeleton, and the hydrogens at the 3- and 5-positions were substituted with methyl, ethyl or propyl groups (Fig. 1). Previous studies of memantine distribution and its binding to the receptor have revealed that: (1) the free amino group is essential both for memantine to penetrate the blood–brain barrier22,23 and for it to bind to the receptors;24,25 (2) the hydrophobic group(s) on the 3- or (and) 5-position(s) of the memantine skeleton increase(s) its binding affinity for the NMDA receptors and decrease(s) its hydrophilicity.25 Based on these findings, the free amino group at the 1-position and the hydrogen at the 7-position of the memantine skeleton were preserved in the new memantine nitrates (Fig. 1). The modifications of the memantine skeleton were conducted on the 3- or (and) 5-position(s). The side group on the 3-position of memantine varied from a methyl to a propyl group. The nitrate moiety was introduced on the 5-position of the memantine skeleton. The length of the carbon chain linking the nitrate group and the memantine skeleton varied from 0–3. In addition, the new compounds may have improved plasma stability due to the replacement of a tertiary nitrate with a primary one. We also wondered whether increasing the number of nitrate groups would provide additional vasodilating and neuroprotective effects, and thus memantine derivatives MN-12–MN-16, armed with two nitrate groups, were designed and synthesized.
Compounds MN-01–MN-03 were prepared as illustrated in Scheme 1. The commercially available corresponding precursors were brominated with Br2 and then underwent the Ritter reaction to afford the respective acetamides 7–9. A hydroxyl group was introduced on compounds 7–9 to afford compounds 10–12. The amide groups of compounds 10–12 were hydrolyzed and the resulting amino groups were N-BOC protected to give compounds 13–15. Compounds 13–15 were nitrated in the presence of fuming nitric acid to afford compounds 16–18. The BOC groups of compounds 16–18 were removed by treatment with anhydrous hydrogen chloride in ethyl acetate to afford compounds MN-01–MN-03.
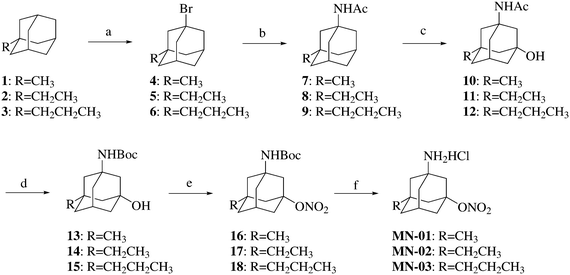 |
| Scheme 1 Synthesis of compounds MN-01–MN-03. Reagents and conditions: (a) (1) Br2, reflux, 4 h; (2) r.t., overnight; (b) CH3CN, conc. H2SO4, r.t., 12 h; (c) (1) conc. HNO3, conc. H2SO4, 0 °C, 2 h; r.t., 30 h; (2) NaOH, H2O; (d) (1) NaOH, diethylene glycol, 175 °C, 15 h; (2) (Boc)2O, THF, TEA, DMAP, r.t., 5 h; (e) fuming HNO3, Ac2O, CH2Cl2, 0 °C; (f) HCl, ethyl acetate. | |
The syntheses of compounds MN-04–MN-06 are shown in Scheme 2. Compounds 1–3 were first brominated followed by hydrolysis to afford adamantanols 19–21. Compounds 19–21 underwent the Koch–Haaf reaction to afford compounds 22–24. The resulting compounds 22–24 were converted into compounds 25–27via the Ritter reaction. Compounds 25–27 were treated with ethyl chloroformate and then reduced to afford compounds 28–30. Compounds MN-04–MN-06 were synthesized from compounds 28–30 using similar methods to those described for the synthesis of compound MN-01 from compound 10.
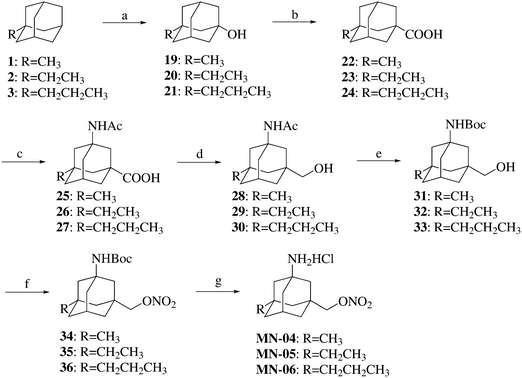 |
| Scheme 2 Synthesis of compounds MN-04–MN-06. Reagents and conditions: (a) (1) Br2, reflux, 4 h; (2) (COONa)2, H2O, 75 °C, 1.5 h; (b) HCOOH, conc. H2SO4, 0 °C, 4 h; (c) CH3CN, conc. HNO3, conc. H2SO4, 0 °C, 1 h; (d) (1) ClCOOC2H5, TEA, THF, r.t., 4 h; (2) NaBH4, H2O, 1 h; (e) (1) NaOH, diethylene glycol, 175 °C, 15 h; (2) (Boc)2O, THF, TEA, DMAP, r.t., 5 h; (f) fuming HNO3, Ac2O, CH2Cl2, 0 °C; (g) HCl, ethyl acetate. | |
Compounds MN-07–MN-09 and MN-11 were synthesized as illustrated in Scheme 3. Compounds 4–6 were reacted with substituted acrylates to give intermediates 37–40, which were then hydrolyzed to afford acids 41–44. The acids 41–44 were amidated and then reduced to give compounds 49–52. Compounds MN-07–MN-09 and MN-11 were prepared from compounds 49–52 using a similar method to that described for the synthesis of MN-01 from compound 10.
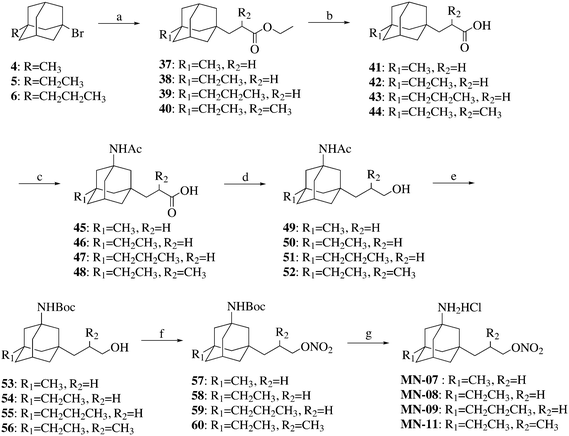 |
| Scheme 3 Synthesis of compounds MN-07–MN-09 and MN-11. Reagents and conditions: (a) ethyl acrylate for 37–39, ethyl methacrylate for 40, AIBN, n-Bu3SnH, toluene, reflux, 2 h; (b) KOH, MeOH, H2O, r.t., overnight; (c) CH3CN, conc. HNO3, conc. H2SO4, 0 °C, 1 h; (d) (1) ClCOOC2H5, TEA, THF, r.t., 4 h; (2) NaBH4, H2O, 1 h; (e) (1) NaOH, diethylene glycol, 175 °C, 15 h; (2) (Boc)2O, THF, TEA, DMAP, r.t., 5 h; (f) fuming HNO3, Ac2O, CH2Cl2, 0 °C; (g) HCl, ethyl acetate. | |
The synthesis of compound MN-10 is described in Scheme 4. Compound 5 was treated with isopropenyl acetate in the presence of AlBr3 to afford compound 61, which was then reduced to afford compound 62. The alcohol 62 was esterified and then amidated to produce compound 64. The target compound MN-10 was prepared from compound 64 using a similar method to that described for the synthesis of MN-01 from compound 10.
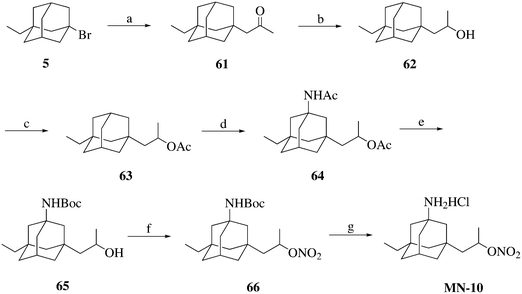 |
| Scheme 4 Synthesis of compound MN-10. Reagents and conditions: (a) isopropenyl acetate, AlBr3, CH2Cl2, 0 °C, 2 h; (b) NaBH4, EtOH, r.t., 4 h; (c) Ac2O, HClO4, r.t., 3 h; (d) CH3CN, conc. HNO3, conc. H2SO4, 0 °C, 1 h; (e) (1) NaOH, diethylene glycol, 175 °C, 15 h; (2) (Boc)2O, THF, TEA, DMAP, r.t., 5 h; (f) fuming HNO3, Ac2O, CH2Cl2, 0 °C; (g) HCl, ethyl acetate. | |
The synthesis of compounds MN-12–MN-13 is shown in Scheme 5. Adamantane (67) was dibrominated and then hydrolyzed to afford 1,3-adamantandiol (69). Compound 69 underwent the Koch–Haaf reaction to afford 1,3-adamantanedicarboxylic acid (70). Compound 70 and 1,3-adamantanediacetic acid (71) were reduced to adamantandiols 72 and 73, which were then esterified to afford compounds 74–75. An acetamido group was introduced on compounds 74–75via the Ritter reaction to produce compounds 76–77. Compounds MN-12–MN-13 were prepared from compounds 76–77 using a similar method to that described for the synthesis of compound MN-01 from compound 10.
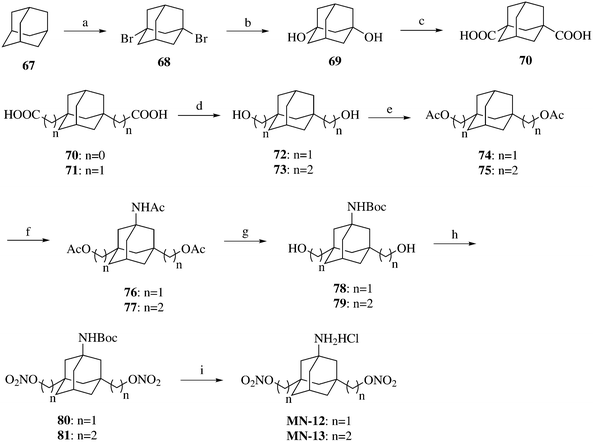 |
| Scheme 5 Synthesis of compounds MN-12–MN-13. Reagents and conditions: (a) Br2, Fe, 0 °C, 2 h; (b) CH3COCH3 : H2O = 1 : 1, reflux, 3 h; (c) HCOOH, conc. H2SO4, 0 °C, 2 h; r.t.,10 h; (d) (1) ClCOOC2H5, TEA, THF, 4 h; (2) NaBH4, H2O, 1 h; (e) Ac2O, HClO4, r.t., 3 h; (f) CH3CN, conc. HNO3, conc. H2SO4, 0 °C, 1 h; (g) (1) NaOH, diethylene glycol, 175 °C, 15 h; (2) (Boc)2O, THF, TEA, DMAP, r.t., 5 h; (h) fuming HNO3, Ac2O, CH2Cl2, 0 °C; (i) HCl, ethyl acetate. | |
Compound MN-15 was synthesized following the procedure illustrated in Scheme 6. 1,3-Dibromoadamantane (68) was treated with isopropenyl acetate in the presence of AlBr3 to afford compound 82. Compound 82 was reduced to afford compound 83, which was then esterified and amidated to produce compound 85. The target compound MN-15 was prepared from compound 85 using a similar method to that described for the synthesis of MN-01 from compound 10.
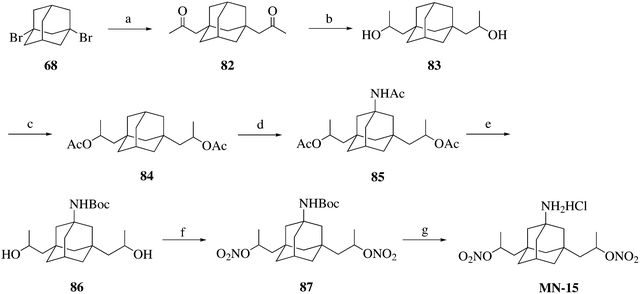 |
| Scheme 6 Synthesis of compound MN-15. Reagents and conditions: (a) isopropenyl acetate, AlBr3, CH2Cl2, 0 °C, 2 h; (b) NaBH4, EtOH, r.t., 4 h; (c) Ac2O, HClO4, r.t., 3 h; (d) CH3CN, conc. HNO3, conc. H2SO4, 0 °C, 1 h; (e) (1) NaOH, diethylene glycol, 175 °C, 15 h; (2) (Boc)2O, THF, TEA, DMAP, r.t., 5 h; (f) fuming HNO3, Ac2O, CH2Cl2, 0 °C; (g) HCl, ethyl acetate. | |
The synthesis of compounds MN-14 and MN-16 is described in Scheme 7. Dibromoadamantane (68) was reacted with the corresponding acrylates to produce compounds 88 and 89. Compounds 88–89 underwent the Ritter reaction to produce compounds 90–91, which were then reduced to compounds 92–93. MN-14 and MN-16 were prepared from compounds 92–93 using a similar method to that described for the synthesis of MN-01 from compound 10.
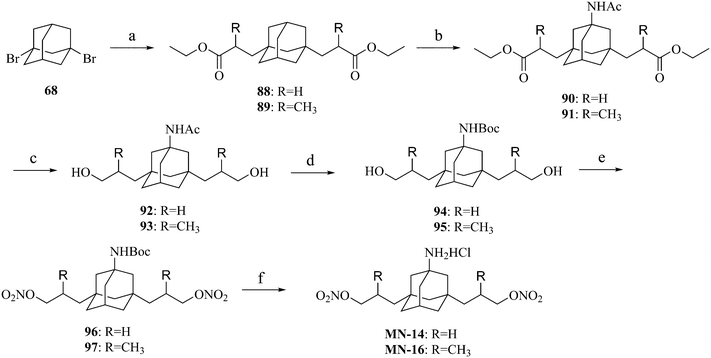 |
| Scheme 7 Synthesis of compounds MN-14 and MN-16. Reagents and conditions: (a) ethyl acrylate for 88, ethyl methacrylate for 89, AIBN, n-Bu3SnH, toluene, reflux, 2 h; (b) CH3CN, conc. HNO3, conc. H2SO4, 0 °C, 1 h; (c) NaBH4, AlCl3, THF, reflux, 3 h; (d) (1) NaOH, diethylene glycol, 175 °C, 15 h; (2) (Boc)2O, THF, TEA, DMAP, r.t., 5 h; (e) fuming HNO3, Ac2O, CH2Cl2, 0 °C; (f) HCl, ethyl acetate. | |
Biological evaluations
The target compounds were first evaluated for their protective effects against glutamate-induced injury in primary cerebellar granule neurons (CGNs) using the MTT assay. Compounds with promising protective effects were then chosen for further evaluation.
Protective effects against glutamate induced neurotoxicity
An excessive amount of glutamate overstimulates the NMDA receptors and results in a cascade of cell damage. Increasing evidence demonstrates that memantine is effective in protecting neurons against glutamate insult.26,27 In this project, we first investigated the protective effects of the new memantine nitrates on a primary culture of CGNs. CGNs were treated with the compounds at specified concentrations for 2 h and were then exposed to glutamate (200 μM) for 24 h in the presence of the compounds. Cell viability was measured by the MTT assay. As shown in Fig. 2, all the memantine nitrates showed moderate to strong protective effects against glutamate induced damage (F(69, 851) = 124.5; P < 0.001). Among these compounds, MN-02, MN-03, MN-05, MN-08, MN-12, MN-14 and MN-16 displayed stronger protective effects than the others, and they were active at a concentration of 1 μM, i.e. were as potent as the parent memantine. Moreover, compounds MN-02, MN-03, MN-05 and MN-12 had lower EC50 values (the concentration that gives half the response of the maximum protection) than the others (Table 1). The other compounds showed moderate protective effects with an initial effective concentration of up to 10 μM. In addition, compounds MN-06–MN-11 and MN-13–MN-16 displayed cytotoxicity at a concentration of 100 μM. By comparing the structures and the activity of these compounds, we found that for substitution at the 3-position an ethyl group was superior to a methyl or propyl group. The binding interactions of memantine and the NMDA receptor revealed that the methyl groups of memantine bind to the hydrophobic pocket formed by the residues V644, A645, and V656 in NMDA subunit GluN1.25 The mutation of A645 (Ala) to L645 (Leu) significantly reduced the affinity of memantine to the receptor,25 which means that steric clash plays an important role in binding; however, the ethyl group is smaller than the propyl group. Thus, the compounds substituted with an ethyl group may be more potent than those with a methyl or propyl group. In addition, we found that the EC50 values and cytotoxic effects were both increased upon lengthening the linker between the nitrate group and memantine moiety.
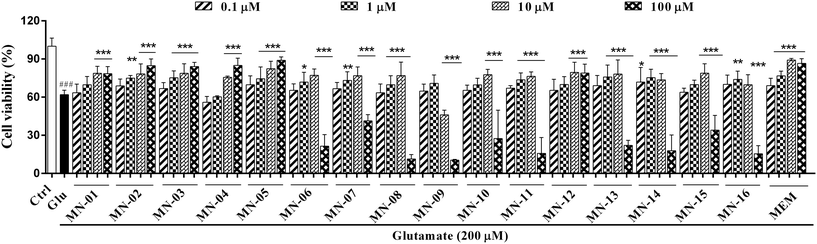 |
| Fig. 2 Protective effects against glutamate-induced excitotoxicity. Cerebellar granule neurons were pre-incubated with memantine nitrates or memantine for 2 h, and then exposed to 200 μM glutamate for another 24 h in the presence of the compound. Cell viability was measured by the MTT assay. ###P < 0.001 versus the control (Ctrl) group; *P < 0.05, **P < 0.01 and ***P < 0.001 versus the glutamate alone (Glu) group. | |
Table 1 The EC50 values of the new memantine nitrates in cultured cerebellar granule neurons
Compounds |
EC50 (μM) mean ± SD |
MN-01
|
24.60 ± 0.04 |
MN-02
|
15.36 ± 3.01 |
MN-03
|
17.08 ± 3.23 |
MN-04
|
25.20 ± 2.33 |
MN-05
|
10.68 ± 4.59 |
MN-06
|
38.06 ± 7.43 |
MN-07
|
39.82 ± 5.68 |
MN-08
|
35.24 ± 9.84 |
MN-09
|
57.55 ± 3.20 |
MN-10
|
58.43 ± 10.62 |
MN-11
|
55.23 ± 9.25 |
MN-12
|
18.91 ± 6.43 |
MN-13
|
25.88 ± 9.41 |
MN-14
|
39.82 ± 5.68 |
MN-15
|
43.01 ± 3.87 |
MN-16
|
53.22 ± 4.45 |
Memantine |
2.48 ± 0.43 |
Vasodilating effects on aortic rings
Organic nitrates are characterized by their ability to dilate blood vessels via the release of NO.28 In this work, we investigated the vasodilating activity of the new memantine nitrates on aortic rings pre-contracted with phenylephrine. Since compounds MN-02–MN-03, MN-05, MN-08, MN-12–MN-14 and MN-16 displayed stronger protective effects in cultured CGNs than the others, they were chosen for further evaluation in this vasodilation assay. To determine whether the vasodilating effect was endothelium dependent or not, investigations were conducted on aortic rings with or without the vascular endothelium (Fig. 3). The maximum effect (Emax) and EC50 of the compounds are listed in Table 2. As illustrated in Fig. 3A, compounds MN-02 and MN-03 showed weak activity for the dilation of blood vessels without endothelium, with an Emax 42.03 ± 3.70 and 61.68 ± 1.63 μM (Table 2), respectively. In contrast, compounds MN-05, MN-08, MN-12–MN-14 and MN-16 were much more potent for blood vessel dilation than the other compounds tested. The order of Emax values was MN-13 > MN-05 > MN-14 ≈ MN-16 > MN-12 ≈ MN-08, and that of EC50 values was MN-12 > MN-13 > MN-05 > MN-16 ≈ MN-14 > MN-08. All the compounds showed a similar pattern in dilating aortic rings with or without the vascular endothelium, indicating that the vasodilating effect of the memantine nitrate was endothelium independent. By comparing the structures and activity of MN-12–MN-14, and MN-16 with MN-02–MN-03, we found that the vasodilating efficacy is increased when the number of nitrate groups is increased. What's more, lengthening the side groups significantly increased the potency for vasodilation (EC50: MN-14 ≈ MN-16 < MN-13 < MN-12, MN-08 < MN-05).
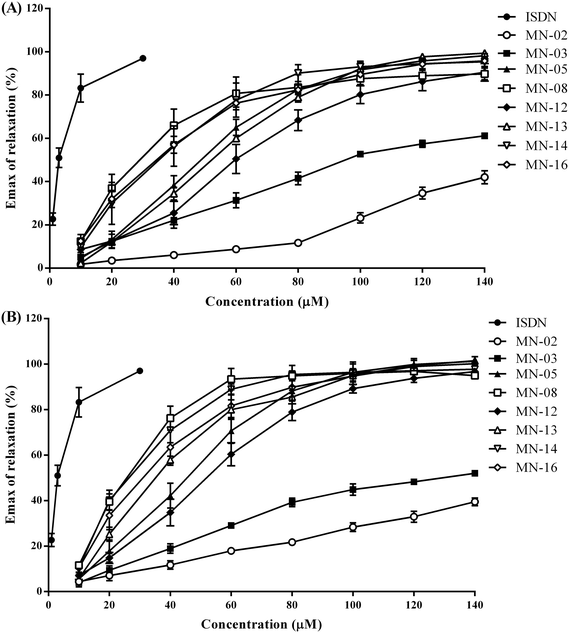 |
| Fig. 3 Vasodilation effects on aortic rings. Aortic rings with or without endothelium were pre-contracted with phenylephrine, and then incubated with memantine nitrates or the positive control drug isosorbide dinitrate, ISDN. The relaxation responses of the arterial rings are expressed as a percentage of the maximal contraction induced by phenylephrine. (A) Effect on aortic rings with endothelium (E+). (B) Effect on aortic rings without endothelium (E−). | |
Table 2 The EC50 and Emax values of memantine nitrates dilating aortic rings
Compounds |
E− |
E+ |
EC50 (μM) |
E
max (μM) |
EC50 (μM) |
E
max (μM) |
MN-02
|
146.33 ± 32.80 |
42.03 ± 3.70 |
— |
39.47 ± 2.41 |
MN-03
|
94.82 ± 10.11 |
61.68 ± 1.63 |
— |
51.99 ± 1.44 |
MN-05
|
50.57 ± 4.38 |
98.19 ± 1.01 |
46.35 ± 1.56 |
101.26 ± 2.46 |
MN-08
|
26.85 ± 7.23 |
89.76 ± 3.31 |
24.38 ± 0.97 |
94.96 ± 0.42 |
MN-12
|
62.81 ± 8.45 |
90.50 ± 4.94 |
54.97 ± 6.87 |
96.75 ± 2.15 |
MN-13
|
57.05 ± 1.23 |
99.37 ± 0.77 |
36.04 ± 2.02 |
101.47 ± 1.04 |
MN-14
|
34.40 ± 10.38 |
95.36 ± 2.62 |
24.60 ± 3.37 |
97.74 ± 2.16 |
MN-16
|
36.46 ± 6.84 |
95.79 ± 4.05 |
31.97 ± 9.55 |
100.21 ± 1.48 |
MN-05 protected cortical neurons against glutamate induced injury
Since compound MN-05 showed better properties for protecting CGNs against glutamate insult and dilating blood vessels, it was chosen for further investigation. Its neuroprotective effect was further investigated with primary cortical neurons by the MTT assay, and fluorescein diacetate (FDA) and propidium iodide (PI) double-staining. The parent memantine was used as a positive control. Since the plasma concentration of memantine in patients is 1–10 μM, the memantine concentration used in the present experiment was 5 μM. As shown in Fig. 4, cell viability was decreased significantly in the glutamate alone-treated group compared with that of the control group. In contrast, MN-05 treatment significantly protected cortical neurons against glutamate insult at concentrations ranging from 5–45 μM in a concentration-dependent manner (Fig. 4, F(5, 61) = 34.87; P < 0.001). FAD/PI double-staining can distinguish living (green) and dead (red) cells (Fig. 5A). The results shown in Fig. 5B demonstrated that the percentage of living cells (green) was decreased markedly in the glutamate alone-treated group compared with the control group. MN-05 treatment improved neuronal viability concentration-dependently. MN-05 is not as potent as the parent memantine at an equimolar concentration. This result was consistent with that seen in the glutamate induced CGN injury assay. Since the neuroprotective effect against glutamate-induced neuronal damage is attributed to NMDA receptor inhibition, we think that MN-05 is less potent than memantine for NMDA receptor inhibition. However, the nitrate group provides MN-05 with a new function, that is, the release of NO to dilate blood vessels in vivo. Thus the effects of MN-05 and memantine deserve to be further compared in an in vivo model.
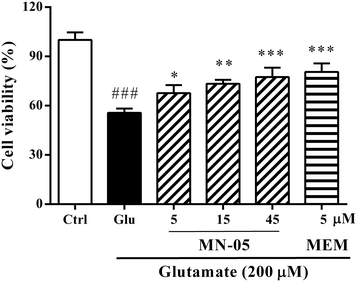 |
| Fig. 4 Protective effects against cortical neuron damage induced by glutamate. Rat primary cortical neurons were treated with the drug for 2 h, and then exposed to 200 μM glutamate for another 24 h without removal of the compound. Cell viability was tested using the MTT assay. ###P < 0.001 versus the control (Ctrl) group; *P < 0.05, **P < 0.01 and ***P < 0.001 versus the glutamate (Glu) group. | |
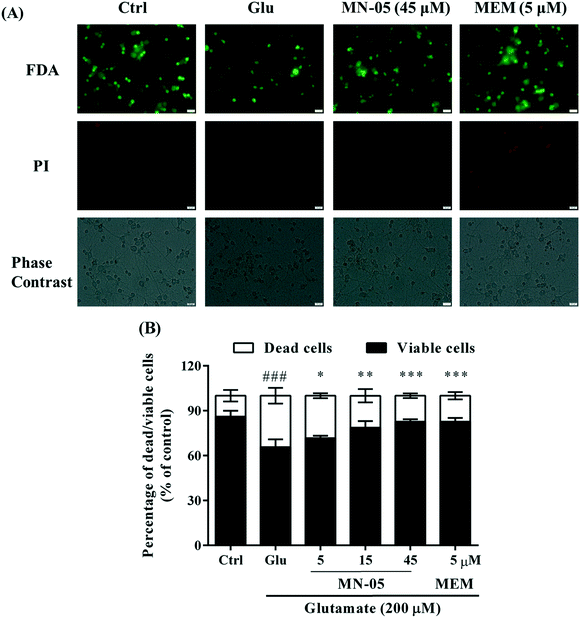 |
| Fig. 5
MN-05 enhanced cortical neuron viability in injury-inducing glutamate. Rat primary cortical neurons were treated with the drugs for 2 h, and then exposed to 200 μM glutamate for another 24 in the presence of the compound. Cell viability was tested using FDA/PI double staining. (A) Captured fluorescence images (green labeling, FDA (live cells) and red labeling, PI (dead cells)). (B) Statistical data for (FDA/PI). ###P < 0.001 versus the control (Ctrl) group; *P < 0.05, **P < 0.01 and ***P < 0.001 versus the glutamate (Glu) group. | |
MN-05 blocked Ca2+ influx induced by glutamate
Overstimulation of NMDA receptors results in an excessive amount of calcium influx and subsequently leads to the initiation of a cell damage cascade.29,30 Compelling evidence demonstrates that memantine binds to the NMDA receptor, blocks the Ca2+ influx, and thus affords neuroprotective effects. We next investigated the effect of memantine derivative MN-05 on intracellular Ca2+ levels (F(3, 136) = 19.98; P < 0.001). As shown in Fig. 6, glutamate triggered a significant increase in intracellular Ca2+. In contrast, MN-05 treatment markedly inhibited the elevation of intracellular Ca2+ at a concentration of 15 μM. Consistent with the neuroprotection effect in vitro, the intracellular Ca2+ blockage effect of MN-05 was not as potent as that of memantine at an equal concentration, indicating that MN-05 was weaker than memantine at inhibiting NMDA receptor activity. The weaker activity of MN-05 for the inhibition of NMDA receptors may be attributed to its lower affinity for NMDA receptors.
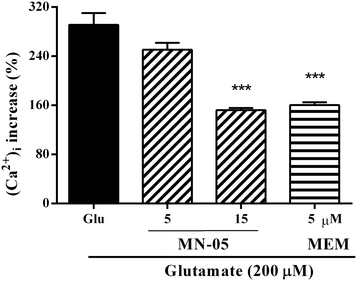 |
| Fig. 6
MN-05 inhibited calcium influx. Cortical neurons were loaded with Fluo-3/AM (5 μM) for 30 min at 37 °C, and then incubated with HBSS buffer for 30 min at room temperature. After washing out the Fluo-3/AM, the cortical neurons were treated with MN-05 or memantine for 5 min. The cells were imaged using a 20× objective lens. ***P < 0.001 versus the glutamate (Glu) group. | |
MN-05 inhibited the production of reactive oxygen/nitrogen species induced by glutamate
Overstimulated NMDA receptors mediate Ca2+ influx and trigger an overproduction of reactive species, including reactive oxygen species (ROS) and reactive nitrogen species (RNS). Experimental studies demonstrate that NMDA receptor blockade results in a decrease in free radical production.31 To further characterize the neuroprotective effects of MN-05 against neurotoxicity induced by glutamate, the inhibitory effects of MN-05 on ROS and RNS production were evaluated. As shown in Fig. 7, MN-05 can inhibit the production of ROS and RNS, including hydrogen peroxide (H2O2, Fig. 7A) (F(5, 146) = 46.67; P < 0.001), hydroxyl radicals (˙OH, Fig. 7B) (F(5, 86) = 18.43; P < 0.001), superoxide anions (O2˙−, Fig. 7C) (F(5, 106) = 32.63; P < 0.001), nitric oxide (NO, Fig. 7D) (F(5, 132) = 49.75; P < 0.001) and peroxynitrite (ONOO−, Fig. 7E) (F(5, 168) = 24.44; P < 0.001), in a concentration-dependent manner. Consistently, the parent memantine was more effective at inhibiting the production of ROS and RNS.
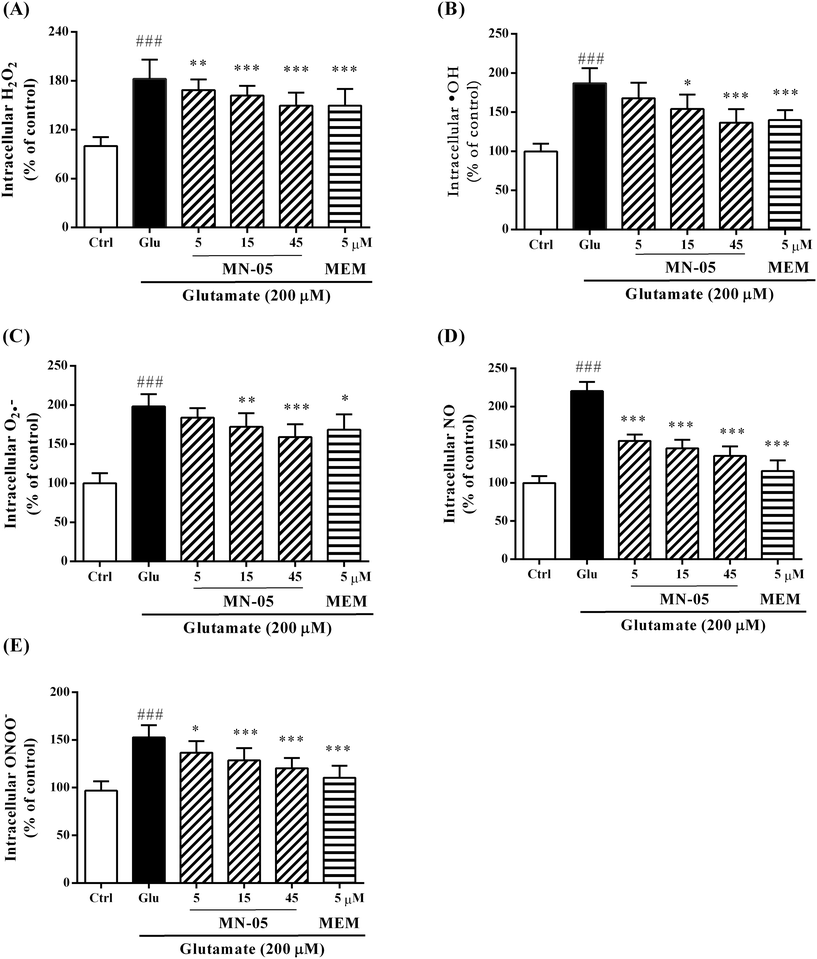 |
| Fig. 7
MN-05 inhibited the production of intracellular ROS and RNS. Cortical neurons were pretreated with MN-05 or memantine at the indicated concentrations for 2 h, followed by exposure to 200 μM glutamate for another 12 h in the presence of the compound. H2DCF-DA (5 μM), HPF (10 μM), DHE (5 μM), DAF-FM (5 μM) and DHR123 (10 μM) were added and incubated for another 30 min. MN-05 significantly inhibited the production of reactive species induced by glutamate in cortical neurons. (A) H2O2; (B) ˙OH; (C) O2˙−; (D) NO and (E) ONOO−. ###P < 0.001 versus the control (Ctrl) group, *P < 0.05, **P < 0.01 and ***P < 0.001 versus the glutamate (Glu) group. | |
MN-05 decreased the reduction of the mitochondrial membrane potential
Mitochondrial dysfunction results in an abnormal production of free radicals. The mitochondrial membrane potential (Δψm) plays an important role in maintaining mitochondrial physiological function and the reduction of Δψm is an early event in the cell apoptotic process.32 To evaluate the mitochondrial protection of MN-05, its effect on the glutamate-induced reduction of Δψm was investigated. Cortical neurons were incubated with MN-05 or memantine at the indicated concentrations for 2 h, followed by exposure to 200 μM glutamate for another 12 h in the presence of the compound. The reduction of Δψm was measured using fluorescence dye JC-1. As illustrated in Fig. 8, glutamate induced a significant reduction of Δψm compared with the control group. In contrast, MN-05 significantly inhibited the reduction of Δψm at concentrations ranging from 5–45 μM (F(5, 84) = 94.37; P < 0.001), suggesting that MN-05 was effective in preserving mitochondrial function. Consistently, memantine also showed stronger activity than MN-05 for the inhibition of the reduction of Δψm at a concentration of 5 μM.
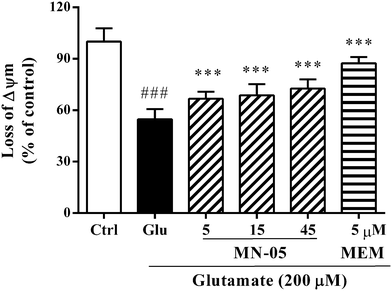 |
| Fig. 8
MN-05 inhibited the reduction of the mitochondrial membrane potential (Δψm). Cortical neurons were treated with MN-05 or memantine at the indicated concentrations for 2 h, followed by exposure to 200 μM glutamate for 12 h without removal of the compound. The cells were washed with JC-1 buffer and stained with 2 μM JC-1 for 20 min. We removed the supernatant and washed the cells with JC-1 buffer three times, and then added 100 μL of HBSS solution to each well. The fluorescence intensity was measured on a microplate reader with 488 nm excitation and 529 nm/590 nm dual emission. ###P < 0.001 versus the control (Ctrl) group, ***P < 0.001 versus the glutamate (Glu) group. | |
Compelling evidence suggests that the inhibition of NMDA receptors affords therapeutic benefits in the treatment of CNS disorders.4,33 Researchers have discovered a number of NMDA receptor antagonists with neuroprotective effects since the 1980s. However, few of them have succeeded in patients.3 For example MK-801 and phencyclidine, because of their excessively high affinity for NMDA receptors, affect the normal physiological functions of NMDA receptors.34 Memantine is an uncompetitive NMDA receptor blocker with low affinity and fast receptor kinetics.8–10 At low micromolar concentrations (1–10 μM), memantine is quite specific for the NMDA receptor compared to other ligand-gated and voltage-gated channels. The clinically achievable plasma concentration of memantine (1–10 μM) does not attenuate long-term potentiation in hippocampal slices and affect the NMDA component of excitatory postsynaptic currents.35 Therefore, memantine is clinically well tolerated.13 In cultured neurons, MN-05 significantly protected the neurons from glutamate insults at a low concentration, but the inhibitory effects of MN-05 on neuronal damage, intracellular calcium influx and ROS/RNS overproduction were not as potent as those of memantine, suggesting that MN-05 may be effective in neuroprotection with fewer side effects (such as cognitive disruption and psychotic-spectrum reactions) than memantine.
NO has great activity in the cardio-cerebrovascular system, where it regulates cerebral circulation and promotes angiogenesis.36,37 A variety of structurally different NO donors have been shown to reduce oxidative stress/inflammation, and increase blood supply.17 The traditional organic nitrates often cause hypotension as a side effect due to the systematic release of NO, which limits their use as neuroprotectants. However, memantine nitrates achieved the targeted release of NO in brain tissue since they have quick distribution to brain tissue (data not shown) and preferentially bind to NMDA receptors after administration. The targeted released NO reacts with a thiol residue of cysteine (S-nitrosylation) on NMDA receptors to achieve the inhibition of NMDA receptors. In another way, the targeted release of NO can selectively dilate brain vessels and increases CBF, which is important since some neurodegenerative disorders are characterized by decreased CBF due to vessel spasm. MN-05 significantly dilated aortic rings in vitro and increased the CBF in vivo (data not shown), however, there are no reports that memantine has this effect on contracted blood vessels. Taken together, MN-05 may be more effective and tolerable than memantine, providing greater therapeutic potential for the treatment of neurodegenerative diseases. The comprehensive comparison of MN-05 and memantine deserves an in-depth in vivo study.
Experimental
The detailed procedures for the synthesis of the memantine nitrates and the biological evaluation experiments can be found in the ESI.†
Conclusions
In summary, we have designed and synthesized a series of new memantine nitrates. Compounds MN-02, MN-03, MN-05, MN-08, MN-12–MN-14 and MN-16 displayed protective effects in cultured cells and exhibited vasodilating effects on aortic rings. The SAR investigation reveals that, in terms of cytoprotective effects, (1) substitution at the 3-position of the memantine skeleton with an ethyl group was superior to substitution with methyl and propyl groups; (2) lengthening the linker between the nitrate group and the memantine moiety decreased the potency; (3) increasing the number of nitrate groups didn't enhance the neuroprotective effects. In terms of the vasodilation effect, (1) the efficacy is increased by increasing the number of the nitrate groups; (2) lengthening the side chains significantly increased the potency. Among the new compounds, MN-05 showed better activity for the protection of cells from glutamate insult and in dilating blood vessels. MN-05 was effective at inhibiting the elevation of intracellular calcium, the production of intracellular reactive species and the reduction of the mitochondrial membrane potential. MN-05 may be a potential new treatment for neurodegenerative diseases including AD.
Acknowledgements
This work was partially supported by grants from the Science and Technology Planning Project of Guangdong Province (2016A050503030), the Natural Science Foundation of Guangdong Province (2014A030310174) and the National Natural Science Foundation of China (NSFC 81603106), as well as the ITSP-Guangdong-Hong Kong Technology Cooperation Funding Scheme (GHP/012/16GD).
Notes and references
- S. F. Traynelis, L. P. Wollmuth, C. J. McBain, F. S. Menniti, K. M. Vance, K. K. Ogden, K. B. Hansen, H. Yuan, S. J. Myers and R. Dingledine, Pharmacol. Rev., 2010, 62, 405–496 CrossRef CAS PubMed.
- A. Lau and M. Tymianski, Pflugers Archiv, 2010, 460, 525–542 CrossRef CAS PubMed.
-
J. C. Piña-Crespo, S. Sanz-Blasco and S. A. Lipton, Handbook of Neurotoxicity, 2014, pp. 1015–1038 Search PubMed.
- E. E. Benarroch, Neurology, 2011, 76, 1750–1757 CrossRef PubMed.
- F. H. Epstein, S. A. Lipton and P. A. Rosenberg, N. Engl. J. Med., 1994, 330, 613–622 CrossRef PubMed.
- D. Lemoine, R. T. Jiang, A. Taly, T. Chataigneau, A. Specht and T. Grutter, Chem. Rev., 2012, 112, 6285–6318 CrossRef CAS PubMed.
- S. A. Lipton, Nat. Rev. Drug Discovery, 2006, 5, 160–170 CrossRef CAS PubMed.
- J. W. Johnson and S. E. Kotermanski, Curr. Opin. Pharmacol., 2006, 6, 61–67 CrossRef CAS PubMed.
- D. Wilkinson, Int. J. Geriatr. Psychiatry, 2012, 27, 769–776 CrossRef PubMed.
- A. Witt, N. Macdonald and P. Kirkpatrick, Nat. Rev. Drug Discovery, 2004, 3, 109–110 CrossRef CAS PubMed.
- H. Chen, J. Pellegrini, S. Aggarwal, S. Z. Lei, S. Warach, F. E. Jensen and S. Lipton, J. Neurosci., 1992, 12, 4427–4436 CAS.
- P. E. Stieg, S. Sathi, S. Warach, D. A. Le and S. A. Lipton, Eur. J. Pharmacol., 1999, 375, 115–120 CrossRef CAS PubMed.
- K. McKeage, CNS Drugs, 2009, 23, 881–897 CrossRef CAS PubMed.
- R. H. Lee, T. Y. Tseng, C. Y. Wu, P. Y. Chen, M. F. Chen, J. S. Kuo and T. J. Lee, PLoS One, 2012, 7, e40326, DOI:10.1371/journal.pone.0040326.
- J. R. Steinert, T. Chernova and I. D. Forsythe, Neuroscientist, 2010, 16, 435–452 CrossRef CAS PubMed.
- M. Willmot, L. Gray, C. Gibson, S. Murphy and P. M. Bath, Nitric Oxide, 2005, 12, 141–149 CrossRef CAS PubMed.
- M. Khan, M. Jatana, C. Elango, A. S. Paintlia, A. K. Singh and I. Singh, Nitric Oxide, 2006, 15, 114–124 CrossRef CAS PubMed.
- Y. B. Choi, L. Tenneti, D. A. Le, J. Ortiz, G. Bai, H. V. Chen and S. A. Lipton, Nat. Neurosci., 2000, 3, 15–21 CrossRef CAS PubMed.
- D. Zurakowski, C. K. Vorwerk, M. Gorla, A. J. Kanellopoulos, N. Chaturvedi, C. L. Grosskreutz, S. A. Lipton and E. B. Dreyer, Vision Res., 1998, 38, 1489–1494 CrossRef CAS PubMed.
- Y. Wang, J. Eu, M. Washburn, T. Gong, H. Vincent Chen, S. W. Larrick James, S. A. Lipton, J. S. Stamler, G. T. Went and S. Porter, Curr. Alzheimer Res., 2006, 3, 201–204 CrossRef PubMed.
- H. Takahashi, P. Xia, J. Cui, M. Talantova, K. Bodhinathan, W. Li, E. A. Holland, G. Tong, J. Pina-Crespo, D. Zhang, N. Nakanishi, J. W. Larrick, S. R. McKercher, T. Nakamura, Y. Wang and S. A. Lipton, Sci. Rep., 2015, 5, 14781 CrossRef CAS PubMed.
- D. C. Mehta, J. L. Short and J. A. Nicolazzo, Mol. Pharmaceutics, 2013, 10, 4491–4498 CrossRef CAS PubMed.
- K. Higuchi, A. Kitamura, T. Okura and Y. Deguchi, Drug Metab. Pharmacokinet., 2015, 30, 182–187 CrossRef CAS PubMed.
- H. S. Chen and S. A. Lipton, J. Pharmacol. Exp. Ther., 2005, 314, 961–971 CrossRef CAS PubMed.
- W. Limapichat, W. Y. Yu, E. Branigan, H. A. Lester and D. A. Dougherty, ACS Chem. Neurosci., 2013, 4, 255–260 CrossRef CAS PubMed.
- C. Volbracht, J. van Beek, C. Zhu, K. Blomgren and M. Leist, Eur. J. Neurosci., 2006, 23, 2611–2622 CrossRef PubMed.
- H. Ota, S. Ogawa, Y. Ouchi and M. Akishita, Exp. Gerontol., 2015, 72, 109–116 CrossRef CAS PubMed.
- D. Bonaventura, C. N. Lunardi, G. J. Rodrigues, M. A. Neto and L. M. Bendhack, Nitric Oxide, 2008, 18, 287–295 CrossRef CAS PubMed.
- A. Mehta, M. Prabhakar, P. Kumar, R. Deshmukh and P. L. Sharma, Eur. J. Pharmacol., 2013, 698, 6–18 CrossRef CAS PubMed.
- M. Jia, S. A. Njapo, V. Rastogi and V. S. Hedna, CNS Drugs, 2015, 29, 153–162 CrossRef CAS PubMed.
- J. L. P. Velazquez, M. V. Frantseva and P. L. Carlen, J. Neurosci., 1997, 17, 9085–9094 CAS.
- A. Perl and K. Banki, Antioxid. Redox Signaling, 2000, 2, 551–573 CrossRef CAS PubMed.
- J. Gonzalez, J. C. Jurado-Coronel, M. F. Avila, A. Sabogal, F. Capani and G. E. Barreto, Int. J. Neurosci., 2015, 125, 315–327 CrossRef CAS PubMed.
- J. Kornhuber and M. Weller, Biol. Psychiatry., 1997, 41, 135–144 CrossRef CAS PubMed.
- H. S. Chen and S. A. Lipton, J. Neurochem., 2006, 97, 1611–1626 CrossRef CAS PubMed.
- F. M. Faraci, Am. J. Physiol., 1991, 261, 1038–1042 Search PubMed.
- K. Ranjbar, F. Nazem, A. Nazari, M. Gholami, A. R. Nezami, M. Ardakanizade, M. Sohrabi, H. Ahmadvand, M. Mottaghi and Y. Azizi, EXCLI J., 2015, 14, 1104–1115 Search PubMed.
Footnotes |
† The authors declare no competing interests. |
‡ Electronic supplementary information (ESI) available: The detailed procedures for the synthesis of the memantine nitrates and the biological evaluation experiments. See DOI: 10.1039/c6md00509h |
|
This journal is © The Royal Society of Chemistry 2017 |