DOI:
10.1039/C6MB00645K
(Paper)
Mol. BioSyst., 2017,
13, 122-131
Analysis of worldwide sequence mutations in Zika virus proteins E, NS1, NS3 and NS5 from a structural point of view†
Received
9th September 2016
, Accepted 19th October 2016
First published on 19th October 2016
Abstract
Zika virus (ZIKV) is an emergent arbovirus that has attracted attention in the last year as a possible causative agent of congenital malformation; it shows a remarkably increased microcephaly risk during otherwise healthy pregnancies. We present here an analysis of all ZIKV sequences available in Genbank up to April 2016, studying the mutations in the whole polyprotein and their possible structural implications for the proteins E, NS1, NS3 and NS5. This study suggests that microcephaly is not a consequence of any particular amino acid substitution but, conceivably, is a feature of ZIKV itself. Moreover, the structural analysis of ZIKV proteins, together with the mutational landscape of ZIKV and a structure-sequence comparison with other flaviviruses, allows the suggestion of regions that could be exploited as anti-ZIKV targets, including some allosteric sites found in the NS3 and NS5 proteins of DENV.
Introduction
Zika virus (ZIKV) was described in 1947 in yellow-fever sentinel monkeys from the Zika forest in Uganda; it was found to cause mild feverish symptoms in humans.1 For six decades, human ZIKV infection did not attract attention because no severe cases had been reported. However, in 2015, the first reports of the possible association between ZIKV infection during pregnancy and congenital abnormalities, especially microcephaly, were reported, mainly in the northeast region of Brazil. Moreover, association of ZIKV with Guillain-Barré syndrome, as well as evidence of viral infection and destruction of neural cells, has been suggested.2–5 Nevertheless, little is known about the pathogenesis of ZIKV in embryonic neuronal tissues or its congenital transmission.
ZIKV is a flavivirus that is transmitted through mosquito bites and is genetically related to other arboviruses, such as West Nile virus (WNV), Japanese Encephalitis virus (JEV), Yellow Fever virus (YFV) and Dengue virus (DENV). Like all flaviviruses, ZIKV has a single-stranded positive sense RNA genome with about 10 kb that encodes 10 proteins;6 these are first translated as a polyprotein that is then cleaved post-translationally into three structural proteins (C, PrM or M, and E) and seven nonstructural (NS) proteins (NS1, NS2A, NS2B, NS3, NS4A, NS4B and NS5). Protein C forms the viral capsid, while PrM/M and protein E, the main immunogenic glycoproteins of the flaviviruses, are located on the viral envelope. Among the NS proteins, NS1 is a multifunctional glycoprotein which forms dimers in the endoplasmic reticulum (ER) membrane through lipid associations in order to participate in viral replication and late infection. NS1 is also secreted in a hexameric form that is involved in immune evasion and pathogenesis.6,7 Little is known about the functions of proteins NS2A, NS4A, and NS4B; however, they are known to be transmembrane proteins that compose the viral replication complex on the ER membrane. NS3 is a serino-protease that cleaves the viral polyprotein and requires NS2B as a cofactor. It also participates in the viral replication complex, as it contains a helicase, a hydrolase and an RNA-triphosphatase domain. Finally, NS5, the largest NS, consists of a methyltransferase (MTase) and an RNA-dependent RNA polymerase (RdRp) domain. The first domain is believed to catalyse both the N7 and 29-O methylation steps, and it may also act as a guanylyltransferase (GTase) during the capping process. The RdRp domain synthesizes the viral RNA genome.6
Many efforts have been made to improve the understanding of how ZIKV infection may influence or even cause microcephaly. Evolutionary and phylogenetic studies pointed towards an Asiatic origin for ZIKV circulating in South America.8–10 Moreover, some studies have reported amino acid changes in American sequences from both common Zika virus infection and microcephaly cases.9,11–14 These amino acidic substitutions raise the question of whether these mutations could cause or somehow contribute to the clinical and pathological findings observed in microcephaly-related infections. Based on the analysis of all ZIKV sequences available in Genbank, and with the knowledge of the tridimensional structures of proteins E, NS1, NS3 and NS5, this study suggests that microcephaly is not a consequence of any particular amino acid substitution but is perhaps a feature of ZIKV itself. Moreover, this analysis, together with a structure-sequence comparison with other flaviviruses, enables the suggestion of regions that could be exploited as anti-ZIKV targets.
Methods
Selection of ZIKV sequences and alignment
54 ZIKV sequences, corresponding to all complete sequences available in the Genbank database up to April 2016, were used for the analysis; the human-derived sequence from French Polynesia was used as a reference (Genbank accession number: KJ776791). This sequence was selected because American-derived ZIKV sequences share a common ancestor with those circulating in French Polynesia in 2013.9 The alignment was conducted in ClustalW with default settings15 using the software MEGA 7.0.16 Three microcephaly-derived sequences were available (KU527068,17 KU49755518 and KU7292179).
Analysis of positive selection at amino acid sites
In this study, an adaptive molecular evolution test was performed on the ZIKV sequences with the Mixed Effects of Evolution method (MEME), available at the Datamonkey webserver;19 this test allows the identification of both episodic and pervasive positive selection at the level of an individual site. Prior to the evolution test, the sequences were scanned with the RDP v4.4.8 program to uncover evidence for recombination events.20 Briefly, this program identifies recombinants from a group of aligned sequences using a number of recombination detection and analysis algorithms. Only events with p-values ≤ 0.01 that were confirmed by four or more methods were considered.
Phylogenetic analysis
All 41 non-recombinant ZIKV sequences were used for the phylogenetic analysis, which was carried out using MEGA 7.0 software by the maximum likelihood (ML) algorithm, using the Tamura Ney model with Gamma distribution (TN93+G) as chosen by the ML model selection feature of MEGA 7.0.16 The statistical significance of clades was measured by 2000 bootstrap replicates.
Protein structures and structural models
Structural models of the ZIKV proteins were built or completed with the Modeller program version 9.13,21 using the sequence taken from the Swiss-Prot reviewed entry Q32ZE1. We selected this sequence because it is the only ZIKV entry in Swiss-Prot, which means that it is the only one that has been already reviewed and where the different protein sequences within the ZIKV polyprotein have been annotated. The amino acid numbers in the models correspond to that entry except for protein E, which presents a deletion in the Q32ZE1 sequence; therefore, the protein numbering corresponds to the published structure (PDB code 5IRE).22 The ZIKV E, NS1 and NS3 protein structures were taken from the Protein Data Bank (PDB codes 5IRE, 5IY3 and 5JMT, respectively).7,22,23 For the NS1 protein, amino acids 1 to 175 were absent in the ZIKV structure; therefore, this protein was modelled from the DENV structure (PDB code 4O6B).24 Also, the N-terminal domain of the ZIKV NS3 structure has not been solved; it was modelled from the DENV structure (PDB code 2WZQ).25 The structure of the ZIKV NS5 protein has not yet been determined; therefore, a structural model was created using the DENV structure (PDB code 5CCV) as a template.26 The models were afterwards refined with the ModRefiner webserver,27 and their quality was validated by Ramachandran plot using the Rampage server28 and the Verify_3D server.29 The results of the validation have been included in the ESI.†
Results
Phylogenetic analysis
The phylogenetic tree of the 41 non-recombinant ZIKV sequences generated by the maximum likelihood method (Fig. S1, ESI†) showed two main lineages: (i) an African lineage containing all 8 non-recombinant African sequences; and (ii) an Asian lineage comprising all 7 non-recombinant Asian-originated sequences and a subgroup of American sequences, completing the 41 sequences. All Brazilian sequences, including the three microcephaly-derived sequences, belong to and were well distributed throughout the American subgroup. Two of the three microcephaly-derived sequences were grouped together (KU527068 and KU729217).
Mutations of ZIKV
236 non-synonymous mutations were found among the 54 available ZIKV sequences. Of all mutations, 141 corresponded to human-derived ZIKV sequences despite clinical manifestations, and 30 were exclusively found in American sequences. 8 African and 5 Asian ZIKV sequences were not considered for evolution analysis due to the high frequency of recombination events. In addition, the African samples originated from mosquitoes, cell cultures and sentinel monkeys. Therefore, the structural analysis was performed only on human-derived samples of American and Asian origin in order to determine if there is any mutational pattern (i.e. similar mutations in similar protein regions) that could explain the appearance of microcephaly events in America. However, this pattern was not observed, and we found no common mutation that was exclusive to the microcephaly-derived sequences and simultaneously observed in all three of them. Only one position under positive selection was found, located on the NS5 protein; this will be discussed later.
Individual analysis of ZIKV proteins
All the mutational data for the ZIKV proteins can be found in the ESI.† A deeper study of all ZIKV proteins and their structures can be found elsewhere.30 Here, we focus only on mutations belonging to human-derived Zika sequences and on the four ZIKV proteins with known structures, which can be more easily targeted for rational drug design (E, NS1, NS3 and NS5).
E protein.
A total of 68 mutation sites were found in the searched sequences (Table 1 and Table S3, ESI†). No positive selection was found in the ZIKV E protein. The mutations were found to be equally distributed between the three domains, with a slight preference for domain III. Taking into consideration only samples from Zika patients, no relevant mutation (namely, involving residues with different physiochemical properties) was found near the glycosylation site (Asn154) (see Fig. S2, ESI†); this is surprising, given the variability of this region between flaviviruses, and contrasts with previous publications that described this region as variable between ZIKV strains.22 On the other hand, several mutations were found in regions that bind to the viral membrane, both in the transmembrane helices (F449I and T479A) and in the viral membrane-binding region of the trimer-fusion conformation (A333G, T335R and M349T) in domain III. This last region has already been observed to differ between ZIKV and DENV, and the authors suggested different viral recognition elements for both viruses.30 As observed here, differences also arose between ZIKV samples. Three mutations were found inside the monomer core (V255A, V213A, V33A), and another mutation was found in the dimer interface (D230A); however, all of these belong to non-conserved regions, and it does not appear that they could have any impact on protein structure. A last mutation, H323D, was found in a conserved histidine residue at the interface between domains I and III. The protonation of this histidine has been experimentally proven to be crucial for initiation of virus fusion.31 This mutation was found in the sample KU744693, a highly mutated sample that was sequenced in China but was obtained from a patient who was infected by ZIKV while in Venezuela.32
Table 1 Mutations in the E protein of ZIKV from human samples according to protein structural region, geographical origin, sequence accession number in Genbank and clinical data, where available
Amino acid location in the protein structure |
Mutationa |
Geographical origin (country) |
Sequence accession number in Genbank |
Clinical data |
PDB mutation numbers are in bold (according to UniProt entry Q32ZE1), and polyprotein mutation numbers are in brackets (according to Genbank accession number KJ776791).
|
Domain 1 (DI) |
V23(313)L |
America (Brazil) |
KU321639 |
ZIKV infection |
V33(323)A |
America (Chi/Ven) |
KU744693 |
ZIKV infection |
T40(330)A |
Asia (Micronesia) |
EU545988 |
ZIKV infection |
T47(337)S |
America (Brazil) |
KU729217 |
Microcephaly |
I152(442)L |
America (Chi/Ven) |
KU744693 |
ZIKV infection |
|
Domain 2 (DII) |
V56(346)I |
America (Guatemal) |
KU501217; KU501216 |
ZIKV infection |
S64(354)T |
America (Brazil) |
KU729217 |
Microcephaly |
M68(358)T |
America (Brazil) |
KU940227 |
Unknown |
M68(358)I |
America (Brazil) |
KU729217 |
Microcephaly |
V213(499)A |
America (Chi/Ven) |
KU744693 |
ZIKV infection |
A227(513)T |
Asia (Thailand) |
KU681081 |
ZIKV infection |
D230(516)A |
America (Chi/Ven) |
KU744693 |
ZIKV infection |
V255(541)A |
America (Brazil) |
KU729217 |
Microcephaly |
S260(546)T |
America (Brazil) |
KU497555 |
Microcephaly |
|
Domain 3 (DIII) |
T309(595)N |
America (Brazil) |
KU940227 |
Unknown |
L322(608)V |
America (Chi/Ven) |
KU744693 |
ZIKV infection |
H323(609)D |
America (Chi/Ven) |
KU744693 |
ZIKV infection |
V330(616)G |
America (Chi/Ven) |
KU744693 |
ZIKV infection |
A333(619)G |
America (Chi/Ven) |
KU744693 |
ZIKV infection |
T335(621)R |
America (Brazil) |
KU926310 |
ZIKV infection |
M349(635)T |
America (Brazil) |
KU729217 |
Microcephaly |
S368(654)G |
Asia (Thailand) |
KU681081 |
ZIKV infection |
|
Stem-anchor region |
K419(705)R |
Asia (Chi/ Sam) |
KU866423; KU820899 |
ZIKV infection |
A437(723)V |
Asia (Thailand) |
KU681081 |
ZIKV infection |
F449(735)I |
America (Chi/Ven) |
KU744693 |
ZIKV infection |
M473(759)V |
Asia (Philippines) |
KU681082 |
ZIKV infection |
Asia (Micronesia) |
EU545988 |
ZIKV infection |
Asia (Cambodia) |
JN860885 |
ZIKV infection |
|
Transmembrane region |
T479(765)A |
America (Suriname) |
KU312312 |
ZIKV infection |
M487(773)T |
Asia (Philippines) |
KU681082 |
ZIKV infection |
Asia (Micronesia) |
EU545988 |
ZIKV infection |
A504(790)G |
America (Chi/Ven) |
KU744693 |
ZIKV infection |
D505(791)G |
America (Chi/Ven) |
KU744693 |
ZIKV infection |
Six mutations were found exclusively in microcephaly samples: T47S, S64T, M68I, V255A and M349T in one sequence (KU729217), and S260T in a second sequence (KU497555). However, most of these involve substitutions between amino acids with very similar characteristics; therefore, no impact on protein structure and/or function should be expected. The only exception, M349T, is located in a loop that establishes contacts between monomers in the capsid (although this amino acid does not seem to be directly involved in those interactions) and binds to the viral membrane in the trimer-fusion conformation. It is interesting that two of those mutations (V255A and M68I) correspond to residues that interact with each other in the crystal structure; also, they correspond to the same sample (KU729217).9 Those residues are located on DIII and are involved in the stabilization of the beta sandwich. However, both amino acids in each pair exist in different DENV crystal structures, without major changes in the domain conformation. Therefore, these mutations are also expected to have no effect on ZIKV.
NS1 protein.
A total of 31 mutational sites were found in the searched sequences (Table 2 and Table S4, ESI†). No positive selection was found in the ZIKV NS1 protein. Again, the mutations are distributed throughout the protein structure; however, taking into consideration only human-derived Zika sequences, no mutation was found on the membrane binding surface (see Fig. S3, ESI†). Most mutations are located on the surface opposite to the membrane region, which remains solvent-accessible both in the dimer and the hexamer forms and which should therefore be responsible for interacting with protein partners, such as antibodies. The two ZIKV glycosylation sites (N130 and N207), conserved between flaviviruses,33 are also located on that surface. One mutation, R211W, in a conserved position, was found next to the N207 site. Several mutations are located at the dimer–dimer interface, some of which are directly involved in dimeric contacts. However, most of those substitutions were observed in different structures of the flaviviruses, with no modification of the dimer interface or structure. An exception is D1 residue, which is highly conserved between flaviviruses; this residue is located in the β-roll membrane-binding domain and experiences electrostatic interactions with K362, D359 and K541 of the other protomer, which is important for stabilization of the β-roll structure. This mutation was found in the same sample as that previously described for R211W (KU744693), together with the following substitutions: R324Q, S176W, T256A and C313S. This last residue forms a conserved disulphide bond with C316. The NS1 protein of this patient could be nonfunctional or even unfolded.
Table 2 Mutations in the NS1 protein of ZIKV from human samples according to protein structural region, geographical origin, sequence accession number in Genbank and clinical data, where available
Amino acid location in the protein structure |
Mutationa |
Geographical origin (country) |
Sequence accession number in Genbank |
Clinical data |
PDB mutation numbers are in bold, and UniProt polyprotein mutation numbers are in brackets (UniProt entry Q32ZE10).
|
Membrane-binding region |
E26(816)D |
Asia (Micronesia) |
EU545988 |
ZIKV infection |
|
Dimerization region |
D1(791)G |
America (Chi/Ven) |
KU744693 |
ZIKV infection |
V188(978)A |
Asia (Micronesia) |
EU545988 |
ZIKV infection |
Asia (Cambodia) |
JN860885 |
ZIKV infection |
Asia (Philippines) |
KU681082 |
ZIKV infection |
G190(980)E |
America (Brazil) |
KU729217 |
Microcephaly |
T233(1023)A |
America (Brazil) |
KU527068 |
Microcephaly |
|
Hexameration region |
R211(1001)W |
America (Chi/Ven) |
KU744693 |
ZIKV infection |
K213(1003)R |
Asia (Thailand) |
KU681081 |
ZIKV infection |
R324(1114)Q |
Asia (Chi/Sam) |
KU866423; KU820899 |
ZIKV infection |
Asia (Chi/Ven) |
KU744693 |
ZIKV infection |
R324(1114)W |
America (Mexico) |
KU922960; KU922923 |
ZIKV infection |
America (Colombia) |
KU820897 |
ZIKV infection |
America (Martiniq) |
KU647676 |
ZIKV infection |
|
Loop region (not resolved in crystal structures) |
Y122(912)H |
America (Brazil) |
KU321639 |
ZIKV infection |
America (Haiti) |
KU509998 |
ZIKV infection |
|
Solvent-accessible surface both in dimer and hexamer forms |
A45(835)V |
Asia (Thailand) |
KU681081 |
ZIKV infection |
G100(890)A |
America (Guatemal) |
KU501216; KU501217 |
ZIKV infection |
K146(936)E |
America (Brazil) |
KU527068 |
Microcephaly |
S176(966)W |
America (Chi/Ven) |
KU744693 |
ZIKV infection |
I236(1026)V |
Asia (Philippines) |
KU681082 |
ZIKV infection |
T256(1046)A |
America (Chi/Ven) |
KU744693 |
ZIKV infection |
C313(1103)S |
America (Chi/Ven) |
KU744693 |
ZIKV infection |
M349(1139)V |
America (Brazil) |
KU729217; KU527068 |
Microcephaly |
KU926310 |
ZIKV infection |
America (Dom Rep) |
KU853013; KU853012 |
ZIKV infection |
Two mutations were found exclusively in microcephaly sequences: G190E (KU7292217) and T233A (KU527068). Another mutation, M349V, was found in the same microcephaly sequence (KU7292217) and in another sequence derived from a male patient, which presents M349V alone. The G190E and T233A residues are located at the dimerization interface, while M349V is found on the solvent accessible surface. One mutation, E146K, was found in one microcephaly sample (KU527068). This amino acid is located near the N130 glycosylation site, which has been found to be important for binding to the complement components C1s and C4 in DENV.34 At any rate, it should be pointed out that in these microcephaly mutations, both amino acids in each pair are found on different flavivirus structures. In fact, position 146 is non-conserved between flaviviruses, and some DENV subtypes present a negative residue while others present a positive residue.
NS3 protein.
A total of 55 mutational sites were found in the studied sequences (Table 3 and Table S7, ESI†). No positive selection was found in the ZIKV NS3 protein; also, no mutations were found in the microcephaly sequences. Overall, the mutations were distributed widely on the protein structure; however, considering only the human ZIKV sequences, no mutation was found in Domain I of the NS3 helicase or in the inter-domain region (see Fig. S4, ESI†).
Table 3 Mutations in the NS3 protein of ZIKV from human samples according to protein structural region, geographical origin, sequence accession number in Genbank and clinical data, where available
Amino acid location in the protein structure |
Mutationa |
Geographical origin (country) |
Sequence accession number in Genbank |
Clinical data |
PDB mutation numbers are in bold, and UniProt polyprotein mutation numbers are in brackets (UniProt entry Q32ZE10).
|
NS3pro |
A106(1604)E |
America (Mexico) |
KU922960; KU922923 |
ZIKV infection |
L112(1610)P |
Asia (Micronesia) |
EU545988 |
ZIKV infection |
I115(1613)T |
Asia (Philippines) |
KU681082 |
ZIKV infection |
D120(1618)G |
America (Brazil) |
KU940224; KU940228 |
Unknown |
|
NS3hel |
M334(1832)T |
America (Brazil) |
KU729218 |
Fatal ZIKV infection |
S347(1845)T |
Asia (Philippines) |
KU681082 |
ZIKV infection |
D354(1852)E |
America (Chi/Ven) |
KU744693 |
ZIKV infection |
H355(1853)Y |
America (Chi/Ven) |
KU744693 |
ZIKV infection |
S365(1863)R |
America (Chi/Ven) |
KU744693 |
ZIKV infection |
Q396(1894)L |
Asia (Micronesia) |
EU545988 |
ZIKV infection |
H400(1898)N |
Asia (Philippines) |
KU681082 |
ZIKV infection |
Asia (Micronesia) |
EU545988 |
ZIKV infection |
D436(1934)G |
America (Chi/Ven) |
KU744693 |
ZIKV infection |
R525(2023)K |
Asia (Chi/Sam) |
KU866423 |
ZIKV infection |
K537(2035)R |
America (Brazil) |
KU926309 |
ZIKV infection |
R560(2058)K |
Asia (Micronesia) |
EU545988 |
ZIKV infection |
T567(2065)M |
Asia (Philippines) |
KU681082 |
ZIKV infection |
M572(2070)L |
America (Guatemal) |
KU501216; KU501217 |
ZIKV infection |
Y584(2082)H |
Asia (Philippines) |
KU681082 |
ZIKV infection |
Asia (Micronesia) |
EU545988 |
ZIKV infection |
Most mutations were found in residues that are not conserved between flaviviruses. For example, the mutation M334T, which is located next to the loop corresponding to the DENV epitope binding region (residues 460 to 469 of DENV) and was found in a fatal human case (KU729218), is a variable residue located within a conserved flavivirus motif. An exception is S365R, which is conserved within the family. As this residue is located on the nucleotide binding site (in comparison with DENV), its substitution with a positively charged amino acid could increase its binding affinity for nucleotides.35 The consequences of this substitution, if any, are unknown. However, this mutation was found in the highly mutated KU744693 sequence.
Finally, three mutations were found near the NS2B binding groove, namely A106E, L112P and I115T; each of these was found in different sequences from Asia and America. It is known that in the absence of the NS2B cofactor, NS3pro is unfolded and inactive.36 Moreover, experimental studies which characterized the YFV NS2B–NS3 complex observed that no second-site revertants could be obtained from mutations in the NS3 region and that most lethal alanine substitutions occurred in NS3; this indicates that the NS3 protease domain is not tolerant of structural perturbation.37
NS5 protein.
A total of 62 mutational sites were identified in the studied sequences (Table 4 and Table S10, ESI†). One position was found to be under positive selection: the amino acid Glu311, which is strongly conserved among flaviviruses. Interestingly, there are two mutations in that position, E311D and E311V; both are found in ZIKV from American patients, and the second one is found in a microcephaly case (KU497555). This residue is located in the finger region, on the index finger, at interacting distance from the tip of the middle finger; this finger, together with the ring finger, remains at the top-right rim of the NTP entry channel and interacts with the MTase domain.38 Mutations in that region could therefore modify access to the active site. Notably, most of the relevant mutations of ZIKV human samples (namely, those involving residues with different physiochemical properties) belong to residues located around the tip of the middle finger or at the interface between the MTase and RdRp domains (see Fig. S5a and b, ESI†). Between these two domains, mutations were found at residues R280 (K, N, D), S287N and H289K, which are located on the N-terminal extension of the MTase domain (which actually belongs to the RdRp domain); this region has been suggested to play auxiliary roles to the RdRp domain, such as stabilization.38 Mutations in these residues are found in several samples; however, it is interesting to mention that the R280D mutant belongs to a microcephaly case and that the H289 residue is usually either a histidine or an asparagine, not a positively charged amino acid. The two last substitutions were found, however, in the highly mutated KU744693 sequence, together with E311D, P313A and V814A. Also, in the region around the NTP entry channel, the mutations N454I and M455T are found on the same sequence and are located in a highly conserved region of the ring finger. Finally, the amino acid N258 is found on the MTase domain; similar to the DENV structure, it is located at the interface with the RdRp domain, within interacting distance of K359 of the RdRp. Therefore, its mutation to a negatively charged residue could increase the interaction between the two domains. It has been suggested that the different relative conformations of the MTase and the RdRp domains could regulate protein function39 and that the flexibility of the linker between the two regions is essential for virus replication.40
Table 4 Mutations in the NS5 protein of ZIKV from human samples according to protein structural region, geographical origin, sequence accession number in Genbank, and clinical data, where available
Amino acid location in the protein structure |
Mutationa |
Geographical origin (country) |
Sequence accession number in Genbank |
Clinical data |
PDB mutation numbers are in bold, and UniProt polyprotein mutation numbers are in brackets (UniProt entry Q32ZE10).
|
|
A91(2607)V |
America (P. Rico) |
KU501215 |
ZIKV infection |
M114(2630)V |
Asia (Chi/Sam) |
KU866423 |
ZIKV infection |
Asia (Philippines) |
KU681082 |
ZIKV infection |
Asia (Can/Thai) |
KF993678 |
ZIKV infection |
Asia (French Pol) |
KJ776791 |
ZIKV infection |
Asia (Cambodia) |
JN860885 |
ZIKV infection |
Asia (Micronesia) |
EU545988 |
ZIKV infection |
Asia (China) |
KU820899 |
ZIKV infection |
V124(2640)I |
Asia (Chi/Sam) |
KU866423 |
ZIKV infection |
V168(2684)A |
America (Brazil) |
KU926310 |
ZIKV infection |
K174(2690)R |
America (Guatemal) |
KU501216; KU501217 |
ZIKV infection |
I181(2697)V |
Asia (Thailand) |
KU681081 |
ZIKV infection |
T229(2745)I |
Asia (China) |
KU820898; KU740184 |
ZIKV infection |
|
Linker |
N259(2775)D |
America (Brazil) |
KU365777; KU365780 |
ZIKV infection |
V267(2783)A |
Asia (China) |
KU820898; KU740184 |
ZIKV infection |
|
RdRp domain (Fingers) |
N280(2796)D |
America (Brazil) |
KU729217 |
Microcephaly |
S287(2803)N |
America (Chi/Ven) |
KU744693 |
ZIKV infection |
H289(2805)Q |
America (Mexico) |
KU922960; KU922923 |
ZIKV infection |
H289(2805)K |
America (Chi/Ven) |
KU744693 |
ZIKV infection |
E311(2827)D |
America (Chi/Ven) |
KU744693 |
ZIKV infection |
E311(2827)V |
America (Brazil) |
KU497555 |
Microcephaly |
P313(2829)A |
America (Chi/Ven) |
KU744693 |
ZIKV infection |
N454(2970)I |
America (Chi/Ven) |
KU744693 |
ZIKV infection |
M455(2971)T |
America (Chi/Ven) |
KU744693 |
ZIKV infection |
|
RdRp domain (α-NLS) |
V322(2838)I |
America (Brazil) |
KU940228; KU940227 KU940224 |
Unknown |
America (Dom Rep) |
KU853012; KU853013 |
ZIKV infection |
|
RdRp domain (α,β-NLS) |
V374(2890)I |
Asia (Can/ Thai) |
KF993678 |
ZIKV infection |
|
RdRp domain (Palm) |
G510(3026)V |
America (Mexico) |
KU922960; KU922923 |
ZIKV infection |
R525(3041)C |
America (Guatemal) |
KU501216; KU501217 |
ZIKV infection |
N624(3140)S |
Asia (China) |
KU820899 |
ZIKV infection |
K670(3186)R |
Asia (Chi/Sam) |
KU866423; KU820899 |
ZIKV infection |
K670(3186)R |
Asia (Chi/Sam) |
KU866423; KU820899 |
ZIKV infection |
|
RdRp domain (Thumb) |
V814(3330)A |
America (Chi/Ven) |
KU744693 |
ZIKV infection |
T833(3349)P |
America (Martiniq) |
KU647676 |
ZIKV infection |
T833(3349)A |
America (Colombia) |
KU820897 |
ZIKV infection |
V872(3388)M |
Asia (Micronesia) |
EU545988 |
ZIKV infection |
Asia (Philippines) |
KU681082 |
ZIKV infection |
Asia (Cambodia) |
JN860885 |
ZIKV infection |
Asia (Chi/Sam) |
KU866423 |
ZIKV infection |
D878(3394)E |
America (Dom Rep) |
KU853012; KU853013 |
ZIKV infection |
M883(3399)V |
Asia (Chi/Sam) |
KU866423 |
ZIKV infection |
Asia (Cambodia) |
JN860885 |
ZIKV infection |
Apart from the aforementioned substitutions, the few other mutations found in human-derived ZIKV sequences do not suggest any relevant functional effects. Some mutations were found in the MTase domain. However, they involve non-conserved residues, and they are not located in any of the known binding sites. Also, the few mutations on the palm and the thumb domains belong to non-conserved residues and do not lie close to any known important sites.
Overall, the mutations found in the NS5 protein appear to modify the interactions between the MTase and the RdRp domains, regulating access to the active site and also regulating protein function.39
Discussion
In this study, a comprehensive analysis of all mutations found in the available sequences of ZIKV, along with a phylogenetic analysis, is presented. The phylogenetic analysis, performed by the Maximum Likelihood method, demonstrated two main groups: an African lineage and an Asian lineage, the latter of which encompasses all American sequences. These results are consistent with previous reports of phylogenetic proximity between American and Asian ZIKV strains.9
Regarding the mutational analysis, most mutations were found in non-conserved protein sites. This is expected, as the literature indicates that conserved regions are generally associated with key protein functions and, therefore, such mutations tend to be eliminated from the protein due to potentially deleterious effects on its functionality. On the other hand, the impact of mutations in non-conserved regions is lower, favouring the occurrence of positive selection.41,42 No specific mutation was present in all three microcephaly sequences; although some mutations were seen exclusively in microcephaly sequences, most of these correspond to non-conserved positions, being both residues of pairs existing in different flaviviruses, and therefore are not believed to have any effect on protein function. Interestingly, one mutation in the NS5 protein was found in a position that is under positive selection (E311). However, again, this mutation was found in only one of the microcephaly sequences. The first of these microcephaly sequences, KU527068, presents only two mutations in the NS1 protein (T233(1023)A e K146(936)E), which are located in different protein regions, and one mutation in the NS4B protein (T2502I). The KU497555 sequence presents one mutation in the E protein (S260(546)T), a second mutation in NS2A (L1255F), and a last mutation in NS5 (E311(2827)V). The KU729217 sequence, on the other hand, is highly mutated, presenting 5 mutations in the E protein ((T47(337)S, S64(354)T, M68(358)I, V255(541)A), M349(635)T), some of which are located close to each other in the protein structure: two mutations are found in NS1 ((G190(980)E, M349(1139)V)), one in NS2B (M1400I) and one in NS5 (N280(2796)D) (Fig. S6, ESI†). Overall, microcephaly development does not appear be related to a specific mutation, but may be an intrinsic property of ZIKV.
It has been already proven that ZIKV, like WNV, can cross the placental barrier.43–45 However, the simple ability to cross the placental barrier is not sufficient to produce microcephaly, as this has not been seen after intrauterine WNV infection; however, it is a potential first step. Unfortunately, the mechanisms of placenta crossing are still unknown. However, the design of E protein inhibitors, meant to prevent membrane fusion, could be an interesting approach. No study has yet been conducted for ZIKV; however, for DENV, a ligand-binding pocket was suggested after a protein structure was solved with a N-octyl-β-D-glucoside (β-OG) molecule bound between DI and DII.46 This pocket has been already studied as a target for the design of DENV inhibitors.47,48 However, comparing the structures of DENV and ZIKV, we can see that, in ZIKV, this pocket presents a much smaller volume; this may hinder the binding of small molecules (Fig. 1A). In fact, the sequence identity in this region is less than 50%, as has already been pointed out by others.30 In this study, no mutations in Zika sequences of human origin were found in protein regions exposed on the mature virus particle, including the glycosylation site (Asn154). These regions could therefore be interesting targets for ZIKV inhibitors.
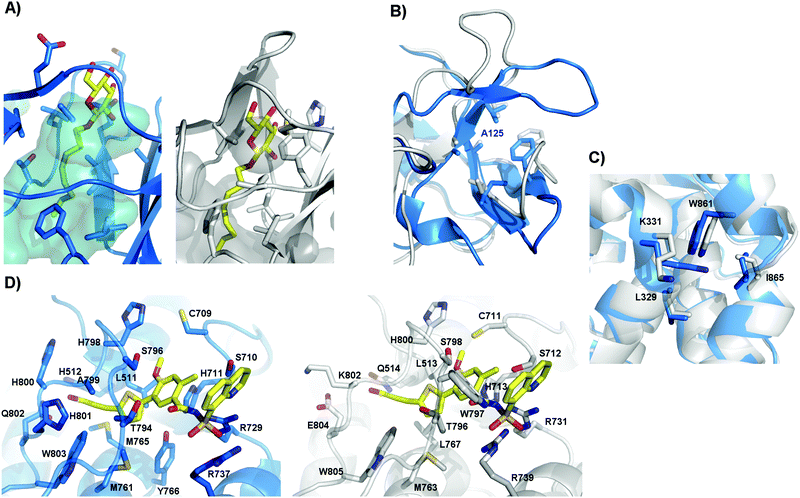 |
| Fig. 1 Inhibitor binding pockets found in DENV. Comparison between DENV (blue) and ZIKV (grey) structures. (A) The β-OG (yellow) binding pocket in DENV E protein (PDB ID 1OKE),46 and the same pocket in ZIKV E protein, where the β-OG pocket alone has been superimposed for visualization. The residues in the cavity are shown with sticks, and the volume of the cavity is shown as the negative surface. We can see that in ZIKV, this volume is greatly decreased. (B) Allosteric site of DENV NS3 found at the NS2B binding groove around A125.51 The residues of the site are shown as sticks. It can be seen that these residues are conserved between DENV and ZIKV. (C) Allosteric site found on DENV NS5 near the NS3 binding interface.52,53 The residues on this site are conserved between DENV and ZIKV. (D) Allosteric site found on DENV NS5 near the active site, with compound 68E from PDB ID 5JJR shown as yellow sticks.55 Compound 68E has been superposed on our ZIKV NS5 model for visualization. The residues on this site are mostly conserved between DENV and ZIKV. | |
The NS1 protein plays a role in the pathogenesis of flavivirus infection through interactions with innate immune proteins, such as toll-like receptor 3 (TLR3), and complement molecules, namely C1 and C4. Interestingly, ZIKV NS1-TLR3 interaction has been associated with human neural progenitor depletion in vitro.24,49 Therefore, the NS1 protein appears to be an attractive target for antiviral compounds; however, to our knowledge, just one in silico study has been published for flaviviruses,50 which is possibly explained by the complexity of the oligomeric glycosylated forms of NS1. The design of small molecules that can block the interaction of NS1 with its partners, or even NS1 hexamerization, would be an interesting option. However, the rational design of NS1 modulators is hampered by the lack of a proper ligand binding pocket, the unspecific interactions of the protomers in the hexamer form, the lack of information about the glycosylated forms of NS1 and, as we observed in this study, the mutational propensity of the exposed NS1 surface.
The NS3 and NS5 viral polymerases have been widely studied as targets for inhibitor design, due to the presence of binding pockets in both proteins. However, most nucleoside polymerase inhibitors are characterized by low selectivity and severe side effects. Nonetheless, allosteric binding pockets have been described for both proteins.51–56 For NS3, the most studied allosteric site is the NS2B binding groove.51,56 Yildiz et al. identified the region around DENV A125 as a possible allosteric site for inhibitor binding.51 In the present report, we noticed a high frequency of mutations around the NS2B groove. However, the amino acids around A125 are conserved both between ZIKV sequences and between ZIKV and DENV (Fig. 1B). Concerning NS5, at least two allosteric pockets have been described and have already been exploited for the design of DENV inhibitors. A group of compounds that interfere with the initiation of RNA synthesis and with the formation of the functional NS5–NS3 complex are believed to bind in an allosteric site conserved between DENV and ZIKV (Leu-329, Lys-331, Trp-861, and Ile-865) (Fig. 1C).52,53 A second allosteric site, termed the N-pocket, has been found close to the active site, and X-ray structures of two inhibitors bound at this site have been recently reported.55 The amino acids in the N-pocket are mostly conserved between DENV and ZIKV (Fig. 1D). Moreover, no relevant mutation was found in human-derived ZIKV sequences near these regions. Therefore, these three allosteric sites could be also studied for the design of inhibitors of ZIKV replication.
Conclusions
Analysis of the ZIKV sequences available at Genbank up to April 2016 suggests that microcephaly is not a consequence of any particular amino acid substitution, as no mutational pattern distinguished American sequences from Asian sequences; also, no mutation was observed in all microcephaly sequences. Only one position under positive selection, E311, was found in the NS5 protein. This residue is located in the finger region, close to the NTP entry. In fact, most mutations found on the NS5 protein appear to be involved in modifying the interactions between the MTase and the RdRp domains, which regulate the access to the active site and the overall protein function. A study of the mutations that focused on protein structure shows that the E-protein face exposed on the mature virus particle presents no mutation; therefore, this site could be exploited as a target for antiviral compounds. The contrary was seen for NS1, whose exposed face, which is potentially responsible for binding to protein partners such as TLR3 and the complements C1 and C4, is highly mutated. Some allosteric binding sites described in DENV NS3 and NS5 proteins correspond to conserved amino acids between ZIKV sequences and between ZIKV and DENV; therefore, these present attractive targets for anti-ZIKV inhibitor design. Overall, the present study aids the understanding of the ZIKV mutational landscape, which can be useful for the future design of anti-ZIKV agents.
Note added in proof
Upon submission, we found that another paper had just appeared in PubMed reporting similar results.30
References
- N. Wikan and D. R. Smith, Lancet Infect. Dis., 2016, 16, e119–e126 CrossRef PubMed.
- E. Oehler, L. Watrin, P. Larre, I. Leparc-Goffart, S. Lastère, F. Valour, L. Baudouin, H. Mallet, D. Musso and F. Ghawche, Eurosurveillance, 2014, 19, 20720 CrossRef PubMed.
- P. Brasil, P. C. Sequeira, A. D. Freitas, H. E. Zogbi, G. A. Calvet, R. V. de Souza, A. M. Siqueira, M. C. L. de Mendonca, R. M. R. Nogueira, A. M. B. de Filippis and T. Solomon, Lancet, 2016, 387, 1482 CrossRef.
- L. M. Araujo, M. L. B. Ferreira and O. J. Nascimento, Arq. Neuropsiquiatr, 2016, 74, 253–255 CrossRef PubMed.
- P. P. Garcez, E. C. Loiola, R. Madeiro da Costa, L. M. Higa, P. Trindade, R. Delvecchio, J. M. Nascimento, R. Brindeiro, A. Tanuri and S. K. Rehen, Science, 2016, 352, 816–818 CrossRef CAS PubMed.
- V. J. Klema, R. Padmanabhan and K. H. Choi, Viruses, 2015, 7, 4640–4656 CrossRef CAS PubMed.
- H. Song, J. Qi, J. Haywood, Y. Shi and G. F. Gao, Nat. Struct. Mol. Biol., 2016, 23, 456–458 CAS.
- D. Musso, Emerging Infect. Dis., 2015, 21, 1887 CrossRef PubMed.
- N. R. Faria, R. d. S. d. S. Azevedo, M. U. G. Kraemer, R. Souza, M. S. Cunha, S. C. Hill, J. Theze, M. B. Bonsall, T. A. Bowden, I. Rissanen, I. M. Rocco, J. S. Nogueira, A. Y. Maeda, F. G. d. S. Vasami, F. L. d. L. Macedo, A. Suzuki, S. G. Rodrigues, A. C. R. Cruz, B. T. Nunes, D. B. d. A. Medeiros, D. S. G. Rodrigues, A. L. Nunes Queiroz, E. V. P. d. Silva, D. F. Henriques, E. S. Travassos da Rosa, C. S. de Oliveira, L. C. Martins, H. B. Vasconcelos, L. M. N. Casseb, D. d. B. Simith, J. P. Messina, L. Abade, J. Lourenco, L. C. J. Alcantara, M. M. d. Lima, M. Giovanetti, S. I. Hay, R. S. de Oliveira, P. d. S. Lemos, L. F. d. Oliveira, C. P. S. de Lima, S. P. da Silva, J. M. d. Vasconcelos, L. Franco, J. F. Cardoso, J. L. d. S. G. Vianez-Junior, D. Mir, G. Bello, E. Delatorre, K. Khan, M. Creatore, G. E. Coelho, W. K. de Oliveira, R. Tesh, O. G. Pybus, M. R. T. Nunes and P. F. C. Vasconcelos, Science, 2016, 352, 345–349 CrossRef CAS PubMed.
- M. Giovanetti, T. Milano, L. C. Alcantara, L. Carcangiu, E. Cella, A. Lai, A. Lo Presti, S. Pascarella, G. Zehender, S. Angeletti and M. Ciccozzi, Asian Pac. J. Trop. Med., 2016, 9, 445–452 CrossRef PubMed.
- M. C. Bonaldo, I. P. Ribeiro, N. S. Lima, A. A. C. Dos Santos, L. S. R. Menezes, S. O. D. da Cruz, I. S. de Mello, N. D. Furtado, E. E. de Moura, L. Damasceno, K. A. B. da Silva, M. G. de Castro, A. L. Gerber, L. G. P. de Almeida, R. Lourenço-de-Oliveira, A. T. R. Vasconcelos and P. Brasil, PLoS Neglected Trop. Dis., 2016, 10, e0004816 Search PubMed.
- R. S. Lanciotti, O. L. Kosoy, J. J. Laven, J. O. Velez, A. J. Lambert, A. J. Johnson, S. M. Stanfield and M. R. Duffy, Emerging Infect. Dis., 2008, 14, 1232–1239 CrossRef CAS PubMed.
- O. Faye, C. C. M. Freire, A. Iamarino, O. Faye, J. V. C. de Oliveira, M. Diallo, P. M. A. Zanotto and A. A. Sall, PLoS Neglected Trop. Dis., 2014, 8, e2636 Search PubMed.
- A. D. Haddow, A. J. Schuh, C. Y. Yasuda, M. R. Kasper, V. Heang, R. Huy, H. Guzman, R. B. Tesh and S. C. Weaver, PLoS Neglected Trop. Dis., 2012, 6, e1477 CAS.
- M. A. Larkin, G. Blackshields, N. P. Brown, R. Chenna, P. A. McGettigan, H. McWilliam, F. Valentin, I. M. Wallace, A. Wilm, R. Lopez, J. D. Thompson, T. J. Gibson and D. G. Higgins, Bioinformatics, 2007, 23, 2947–2948 CrossRef CAS PubMed.
- S. Kumar, M. Nei, J. Dudley and K. Tamura, Briefings Bioinf., 2008, 9, 299–306 CrossRef CAS PubMed.
- J. Mlakar, M. Korva, N. Tul, M. Popović, M. Poljšak-Prijatelj, J. Mraz, M. Kolenc, K. Resman Rus, T. Vesnaver Vipotnik, V. Fabjan Vodušek, A. Vizjak, J. Pižem, M. Petrovec and T. Avšič Županc, N. Engl. J. Med., 2016, 374, 951–958 CrossRef CAS PubMed.
- G. Calvet, R. S. Aguiar, A. S. O. Melo, S. A. Sampaio, I. de Filippis, A. Fabri, E. S. M. Araujo, P. C. de Sequeira, M. C. L. de Mendonça, L. de Oliveira, D. A. Tschoeke, C. G. Schrago, F. L. Thompson, P. Brasil, F. B. dos Santos, R. M. R. Nogueira, A. Tanuri and A. M. B. de Filippis, Lancet Infect. Dis., 2016, 16, 653–660 CrossRef PubMed.
- W. Delport, A. F. Y. Poon, S. D. W. Frost and S. L. Kosakovsky Pond, Bioinformatics, 2010, 26, 2455–2457 CrossRef CAS PubMed.
- D. Martin and E. Rybicki, Bioinformatics, 2000, 16, 562–563 CrossRef CAS PubMed.
-
N. Eswar, B. Webb, M. A. Marti-Renom, M. S. Madhusudhan, D. Eramian, M.-Y. Shen, U. Pieper and A. Sali, Curr. Protoc. Protein Sci. Editor. Board John E Coligan Al, 2007, ch. 2, Unit 2.9 Search PubMed.
- D. Sirohi, Z. Chen, L. Sun, T. Klose, T. C. Pierson, M. G. Rossmann and R. J. Kuhn, Science, 2016, 352, 467–470 CrossRef CAS PubMed.
- H. Tian, X. Ji, X. Yang, W. Xie, K. Yang, C. Chen, C. Wu, H. Chi, Z. Mu, Z. Wang and H. Yang, Protein Cell, 2016, 7, 450–454 CrossRef PubMed.
- D. L. Akey, W. C. Brown, S. Dutta, J. Konwerski, J. Jose, T. J. Jurkiw, J. DelProposto, C. M. Ogata, G. Skiniotis, R. J. Kuhn and J. L. Smith, Science, 2014, 343, 881–885 CrossRef CAS PubMed.
- D. Luo, N. Wei, D. N. Doan, P. N. Paradkar, Y. Chong, A. D. Davidson, M. Kotaka, J. Lescar and S. G. Vasudevan, J. Biol. Chem., 2010, 285, 18817–18827 CAS.
-
V. J. Klema, M. Ye, A. Hindupur, T. Teramoto, K. Gottipati, R. Padmanabhan, et al., Crystal Structure of Full-Length NS5 from Dengue Virus Type 3, Available from: http://www.rcsb.org/pdb/explore/explore.do?structureId=5CCV, DOI:10.2210/pdb5ccv/pdb, (Unpublished).
- D. Xu and Y. Zhang, Biophys. J., 2011, 101, 2525–2534 CrossRef CAS PubMed.
- RAMPAGE, http://mordred.bioc.cam.ac.uk/~rapper/rampage.php.
- J. U. Bowie, R. Lüthy and D. Eisenberg, Science, 1991, 253, 164–170 CAS.
- B. D. Cox, R. A. Stanton and R. F. Schinazi, Antiviral Chem. Chemother., 2015, 24, 118–126 CrossRef PubMed (http://www.ncbi.nlm.nih.gov/pubmed/27296393).
- R. Fritz, K. Stiasny and F. X. Heinz, J. Cell Biol., 2008, 183, 353–361 CrossRef CAS PubMed.
- L. Liu, W. Wu, X. Zhao, Y. Xiong, S. Zhang, X. Liu, J. Qu, J. Li, K. Nei, M. Liang, Y. Shu, G. Hu, X. Ma and D. Li, Genome Announc, 2016, 4, e00291 Search PubMed.
- D. A. Muller and P. R. Young, Antiviral Res., 2013, 98, 192–208 CrossRef CAS PubMed.
- P. Somnuke, R. E. Hauhart, J. P. Atkinson, M. S. Diamond and P. Avirutnan, Virology, 2011, 413, 253–264 CrossRef CAS PubMed.
- T. Xu, A. Sampath, A. Chao, D. Wen, M. Nanao, P. Chene, S. G. Vasudevan and J. Lescar, J. Virol., 2005, 79, 10278–10288 CrossRef CAS PubMed.
- R. Assenberg, E. Mastrangelo, T. S. Walter, A. Verma, M. Milani, R. J. Owens, D. I. Stuart, J. M. Grimes and E. J. Mancini, J. Virol., 2009, 83, 12895–12906 CrossRef CAS PubMed.
- T. J. Chambers, D. A. Droll, Y. Tang, Y. Liang, V. K. Ganesh, K. H. M. Murthy and M. Nickells, J. Gen. Virol., 2005, 86, 1403–1413 CrossRef CAS PubMed.
- G. Lu and P. Gong, PLoS Pathog., 2013, 9, e1003549 CAS.
- Y. Zhao, T. S. Soh, J. Zheng, K. W. K. Chan, W. W. Phoo, C. C. Lee, M. Y. F. Tay, K. Swaminathan, T. C. Cornvik, S. P. Lim, P.-Y. Shi, J. Lescar, S. G. Vasudevan and D. Luo, PLoS Pathog., 2015, 11, e1004682 Search PubMed.
- Y. Zhao, T. S. Soh, K. W. K. Chan, S. S. Y. Fung, K. Swaminathan, S. P. Lim, P.-Y. Shi, T. Huber, J. Lescar, D. Luo and S. G. Vasudevan, J. Virol., 2015, 89, 10717–10721 CrossRef CAS PubMed.
- M. Ciccozzi, M. Babakir-Mina, A. Lo Presti, F. Farchi, G. Zehender, E. Ebranati, C. F. Perno and M. Ciotti, Arch. Virol., 2010, 155, 1433–1438 CrossRef CAS PubMed.
- K. M. Cardoso, N. C. Diaz, M. A. A. M. Guimarães, M. G. Zalis, S. Delbue, P. Ferrante and R. B. Varella, J. Med. Virol., 2015, 87, 1418–1426 CrossRef CAS PubMed.
- J. G. Julander, Q. A. Winger, L. F. Rickords, P.-Y. Shi, M. Tilgner, I. Binduga-Gajewska, R. W. Sidwell and J. D. Morrey, Virology, 2006, 347, 175–182 CrossRef CAS PubMed.
- D. R. O'Leary, S. Kuhn, K. L. Kniss, A. F. Hinckley, S. A. Rasmussen, W. J. Pape, L. K. Kightlinger, B. D. Beecham, T. K. Miller, D. F. Neitzel, S. R. Michaels, G. L. Campbell, R. S. Lanciotti and E. B. Hayes, Pediatrics, 2006, 117, e537–545 CrossRef PubMed.
- L. de Noronha, C. Zanluca, M. L. V. Azevedo, K. G. Luz and C. N. D. D. Santos, Mem. Inst. Oswaldo Cruz, 2016, 111, 287–293 CrossRef PubMed.
- Y. Modis, S. Ogata, D. Clements and S. C. Harrison, Proc. Natl. Acad. Sci. U. S. A., 2003, 100, 6986–6991 CrossRef CAS PubMed.
- A. G. Schmidt, K. Lee, P. L. Yang and S. C. Harrison, PLoS Pathog., 2012, 8, e1002627 CAS.
- S. S. Jadav, S. Kaptein, A. Timiri, T. De Burghgraeve, V. N. Badavath, R. Ganesan, B. N. Sinha, J. Neyts, P. Leyssen and V. Jayaprakash, Bioorg. Med. Chem. Lett., 2015, 25, 1747–1752 CrossRef CAS PubMed.
- J. Dang, S. K. Tiwari, G. Lichinchi, Y. Qin, V. S. Patil, A. M. Eroshkin and T. M. Rana, Cell Stem Cell, 2016, 19, 258–265 CrossRef CAS PubMed.
- M. T. U. Qamar, A. Mumtaz, R. Naseem, A. Ali, T. Fatima, T. Jabbar, Z. Ahmad and U. A. Ashfaq, Bioinformation, 2014, 10, 460–465 CrossRef PubMed.
- M. Yildiz, S. Ghosh, J. A. Bell, W. Sherman and J. A. Hardy, ACS Chem. Biol., 2013, 8, 2744–2752 CrossRef CAS PubMed.
- G. Zou, Y.-L. Chen, H. Dong, C. C. Lim, L. J. Yap, Y. H. Yau, S. G. Shochat, J. Lescar and P.-Y. Shi, J. Biol. Chem., 2011, 286, 14362–14372 CrossRef CAS PubMed.
- P. Vincetti, F. Caporuscio, S. Kaptein, A. Gioiello, V. Mancino, Y. Suzuki, N. Yamamoto, E. Crespan, A. Lossani, G. Maga, G. Rastelli, D. Castagnolo, J. Neyts, P. Leyssen, G. Costantino and M. Radi, J. Med. Chem., 2015, 58, 4964–4975 CrossRef CAS PubMed.
- R. G. Gentles, M. Ding, J. A. Bender, C. P. Bergstrom, K. Grant-Young, P. Hewawasam, T. Hudyma, S. Martin, A. Nickel, A. Regueiro-Ren, Y. Tu, Z. Yang, K.-S. Yeung, X. Zheng, S. Chao, J.-H. Sun, B. R. Beno, D. M. Camac, C.-H. Chang, M. Gao, P. E. Morin, S. Sheriff, J. Tredup, J. Wan, M. R. Witmer, D. Xie, U. Hanumegowda, J. Knipe, K. Mosure, K. S. Santone, D. D. Parker, X. Zhuo, J. Lemm, M. Liu, L. Pelosi, K. Rigat, S. Voss, Y. Wang, Y.-K. Wang, R. J. Colonno, M. Gao, S. B. Roberts, Q. Gao, A. Ng, N. A. Meanwell and J. F. Kadow, J. Med. Chem., 2014, 57, 1855–1879 CrossRef CAS PubMed.
- S. P. Lim, C. G. Noble, C. C. Seh, T. S. Soh, A. El Sahili, G. K. Y. Chan, J. Lescar, R. Arora, T. Benson, S. Nilar, U. Manjunatha, K. F. Wan, H. Dong, X. Xie, P.-Y. Shi and F. Yokokawa, PLOS Pathog., 2016, 12, e1005737 Search PubMed.
- H. Wu, S. Bock, M. Snitko, T. Berger, T. Weidner, S. Holloway, M. Kanitz, W. E. Diederich, H. Steuber, C. Walter, D. Hofmann, B. Weißbrich, R. Spannaus, E. G. Acosta, R. Bartenschlager, B. Engels, T. Schirmeister and J. Bodem, Antimicrob. Agents Chemother., 2015, 59, 1100–1109 CrossRef PubMed.
Footnote |
† Electronic supplementary information (ESI) available. See DOI: 10.1039/c6mb00645k |
|
This journal is © The Royal Society of Chemistry 2017 |
Click here to see how this site uses Cookies. View our privacy policy here.