DOI:
10.1039/C6FO01452F
(Paper)
Food Funct., 2017,
8, 381-386
The effect of arginine on the Wnt/β-catenin signaling pathway during porcine intramuscular preadipocyte differentiation
Received
3rd October 2016
, Accepted 12th December 2016
First published on 16th December 2016
Abstract
Dietary L-arginine supplementation decreases backfat thickness and increases intramuscular fat content in growing-finishing pigs, but the underlying mechanisms are unknown. In this study, the effect of arginine on differentiation of porcine intramuscular preadipocytes was investigated in vitro. We showed that the mRNA and protein expressions of the peroxisome proliferator-activated receptor γ (PPARγ) and CCAAT/enhancer binding protein α were upregulated by arginine supplementation. Furthermore, the intracellular triglyceride level was increased by arginine supplementation. We also showed that activation of the Wnt/β-catenin signal pathway by using lithium chloride (LiCl) significantly attenuated arginine-induced upregulation of PPARγ and increased the phospho-β-catenin level. These findings suggested that arginine promotes porcine intramuscular preadipocyte differentiation, which might be via repressing the Wnt/β-catenin signaling pathway.
Introduction
Adipogenesis is the development of fat cells from preadipocytes to mature adipocytes, including preadipocyte proliferation, differentiation, and intracellular lipid accumulation.1 The process is regulated by adipogenic transcriptional factors, such as the peroxisome proliferator-activated receptor γ (PPARγ) and CCAAT/enhancer binding proteins α (C/EBPα). In addition, growing evidence has been accumulated supporting a role for Wnt/β-catenin signaling in the regulation of adipogenesis.2–5 There is evidence that the Wnt/β-catenin signaling specific activator lithium chloride (LiCl) could activate the Wnt signaling pathway, promote stabilization and nuclear translocation of β-catenin, subsequently inhibit adipogenesis by blocking the expression of PPARγ and C/EBPα.6,7
Arginine is one of the 20 most common natural amino acids, which is classified as a semi-essential or a conditionally essential amino acid. Arginine plays multiple nutritional and physiological functions in animals.8–10 A growing body of in vivo and in vitro evidence indicates that arginine supplementation reduces body fat deposition but increases fat deposition in skeletal muscle.11–18 Arginine supplementation has also been reported to increase the intramuscular fat (IMF) content of longissimus dorsi and improve meat quality.14,19 Although the roles of arginine in adipogenesis have been widely recognized, the underlying molecular mechanisms and the signaling pathway of arginine remain largely unknown.
In the present study, we firstly investigated the role of arginine in differentiation of porcine intramuscular preadipocytes. We also examined whether arginine affects differentiation of porcine intramuscular preadipocytes by modulating adipogenic transcription factors and the Wnt/β-catenin signaling pathway.
Materials and methods
Ethics statement
All experiments were performed in compliance with the recommendations in the Guide for the Care and Use of Laboratory Animals of Sichuan Agricultural University. All experiments were approved by the Animal Care Advisory Committee of Sichuan Agricultural University.
Materials
Newborn (about 3-day-old) healthy Duroc, Landrace and Yorkshire crossbred (DLY) piglets (obtained from the pig farm of the Sichuan Zhengyuan swine industry Co., Ltd, Chengdu, China) were used to isolate porcine intramuscular preadipocytes. Dulbecco's modified Eagle's medium (DMEM)/F12 medium and fetal bovine serum (FBS) were obtained from Gibco (PaisLey, Scotland, UK). Primers were synthesized by Tsingke (Chengdu, China). LiCl and arginine were obtained from Sigma (St Louis, MO, USA). Anti-PPARγ and anti-β-actin antibodies were purchased from Santa Cruz Biotechnology (Santa Cruz, CA, USA). Anti-C/EBPα, anti-β-catenin and anti-phospho-β-catenin antibodies were purchased from Cell Signaling Technology (Beverly, MA, USA).
Cell culture
Porcine intramuscular preadipocytes were cultured essentially as described previously.20 Briefly, the cells were maintained in DMEM/F12 medium supplemented with 15% FBS, 100 U mL−1 penicillin and 100 μg mL−1 streptomycin. For adipogenic differentiation, the cells that had been confluent for two days (day 0) were treated with DMEM/F12 supplemented with 10% fetal bovine serum, 1 mM dexamethasone and 0.5 mM 3-isobutyl-1-methylxanthine (IBMX) and 10 μg mL−1 insulin for three days. Subsequently, the cells were treated with DMEM/F12 containing 10% FBS and 10 μg mL−1 insulin for another 2 days. Subsequently, the medium was replaced with DMEM/F12 containing 10% FBS every 2 days. To determine whether arginine affects adipogenic differentiation mediated via the Wnt/β-catenin signaling pathway, porcine intramuscular preadipocytes were treated with arginine in the presence or absence of LiCl. LiCl was added to the medium at a concentration of 20 mM.
Real-time quantitative PCR
At day 8 of differentiation, total RNA was isolated from the cultured porcine intramuscular preadipocytes using the RNAiso Plus reagent (TaKaRa, Dalian, China) according to the manufacturer's instructions and quantified spectrophotometrically at 260 nm. One μg of total RNA was reverse-transcribed to cDNA using the PrimeScript® RT reagent Kit with a gDNA Eraser (TaKaRa) according to the manufacturer's instructions.
Real-time quantitative PCR was performed using SYBR select Master Mix (Applied Biosystems, Foster, CA, USA) on the 7900HT real-time PCR system (Applied Biosystems). The primer sequences used in this study are listed in Table 1. The PCR cycling conditions used were 45 cycles at 95 °C for 15 s and 60 °C for 30 s. Relative gene expression was evaluated using the comparative cycle threshold (Ct) method21 and normalized to the housekeeping gene β-actin expression.
Table 1 List of genes, primer sequences, GenBank accession numbers, and product sizes
Gene name |
Primer |
Sequence |
GenBank accession no. |
Product size (bp) |
PPARγ
|
Forward |
5′-AATTAGATGACAGCGACCTGGCGA-3′ |
NM_214379
|
102 |
Reverse |
5′-TGTCTTGAATGTCCTCGATGGGCT-3′ |
CEBPα
|
Forward |
5′-CGTGGAGACTCAACAGAAGG-3′ |
AF103944
|
95 |
Reverse |
5′-GCAGCGTGTCCAGTTCGCGG-3′ |
LPL
|
Forward |
5′-ACCGTTGCAACAACTTGGGCTATG-3′ |
NM_214286
|
98 |
Reverse |
5′-ACTTTGTAGGGCATCTGAGCACGA-3′ |
Reverse |
5′-CCAATGACTGAGCCGACTG-3′ |
β-Actin
|
Forward |
5′-CCACGAAACTACCTTCAACTCC-3′ |
DQ845171
|
132 |
Reverse |
5′-GTGATCTCCTTCTGCATCCTGT-3′ |
Western blot analysis
The cells were treated with arginine and induced to differentiate as described above. The cultured cells were lysed in RIPA buffer (Pierce, Rockford, IL, USA) supplemented with a protease inhibitor cocktail (Sigma) at day 8 of differentiation. The supernatant was collected and the protein concentrations were determined using the BCA protein assay kit (Pierce) following the manufacturer's instructions.
Equal amounts of total proteins were separated by 12% sodium dodecylsulfate polyacrylamide gel electrophoresis (SDS-PAGE) and then transferred to nitrocellulose membranes. The membranes were immunoblotted with anti-PPARγ (1
:
200), anti-C/EBPα (1
:
1000), anti-β-catenin (1
:
200), or anti-β-actin (1
:
3000) and developed with horseradish peroxidase-conjugated secondary antibodies. Protein bands were visualized with a Clarity™ Western ECL Substrate (Bio-Rad, Hercules, CA, USA) using a ChemiDoc XRS Imager System (Bio-Rad).
Triglyceride assay
At day 8 of differentiation, lipid accumulation was detected by the triglyceride assay. Triglyceride quantification was performed with a triacylglycerol detection kit (Nanjing Jiancheng Bioengineering Institute, Nanjing, China) according to the manufacturer's protocols.
Statistical analysis
All data were expressed as means ± SE (standard error). The results were analyzed by using one-way ANOVA followed by the Tukey test using statistical software SPSS (windows version 11.5). Statistically significance was set at p < 0.05.
Results
Effect of arginine on PPARγ and C/EBPα expressions in porcine intramuscular preadipocytes
To test whether arginine affects the expressions of PPARγ and C/EBPα in porcine intramuscular preadipocytes, the cells treated with different concentrations of arginine were cultured in adipogenic medium for 8 d. As shown in Fig. 1, the maximal increase of PPARγ and C/EBPα expressions was observed after treatment with 200 μg mL−1 of arginine. This dose was chosen and used for subsequent studies.
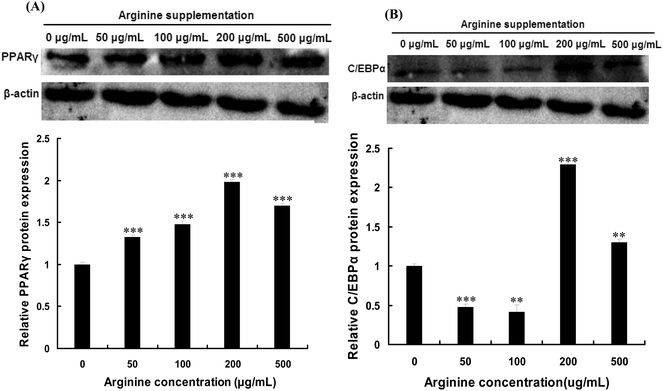 |
| Fig. 1 Effect of arginine on PPARγ and C/EBPα expressions in porcine intramuscular preadipocytes. Porcine intramuscular preadipocytes were treated with different concentrations of arginine and cultured in adipogenic medium for 8 d. The amounts of PPARγ (A) and C/EBPα (B) were analyzed by Western blotting. Results are presented as mean ± SE (n = 3). **p < 0.01, ***p < 0.001 as compared with the control group. | |
Promotion of porcine intramuscular preadipocyte differentiation by arginine supplementation
Real-time quantitative PCR analysis showed that the mRNA levels of PPARγ, C/EBPα and lipoprotein lipase (LPL) in porcine intramuscular preadipocytes significantly increased by arginine supplementation (Fig. 2A). In addition, arginine treatment exhibited promotion of lipogenesis, as indicated by more lipid droplets (data not shown) and an increase of triglyceride content (Fig. 2B). These results indicated that arginine promotes porcine intramuscular preadipocyte differentiation.
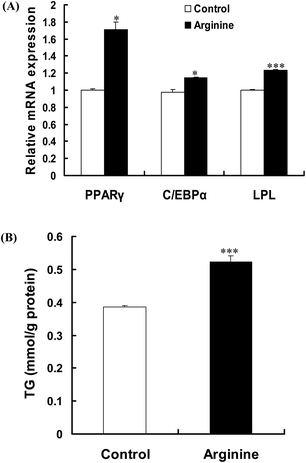 |
| Fig. 2 Effects of arginine on adipogenesis and lipid accumulation. Porcine intramuscular preadipocytes were treated with 200 μg mL−1 of arginine and cultured in adipogenic medium for 8 d. (A) mRNA expression levels of PPARγ, C/EBPα, and LPL were detected by real-time quantitative PCR. The results are represented as mean ± SE (n = 3). *p < 0.05, ***p < 0.001. (B) TG content was determined and the results are presented as mean ± SE (n = 3). ***p < 0.001 as compared with the control group. | |
Arginine promotes adipogenic differentiation by repressing Wnt/β-catenin signaling
To investigate whether arginine promotes adipogenic differentiation by modulating the Wnt/β-catenin signaling pathway, porcine intramuscular preadipocytes were treated with arginine in the presence or absence of LiCl. As shown in Fig. 3A, arginine decreased the protein expression of total β-catenin. This decrease in the total β-catenin protein was inhibited in the cells treated with the Wnt/β-catenin signaling specific activator LiCl (Fig. 3A). As shown in Fig. 3B, arginine increased the phosphorylation level of the β-catenin protein. This increase in the phospho-β-catenin level was partially inhibited in the cells treated with LiCl (Fig. 3B). A similar result was also observed in the PPARγ protein levels (Fig. 3C). These findings suggested that arginine promotes adipogenic differentiation by repressing the Wnt/β-catenin signaling pathway.
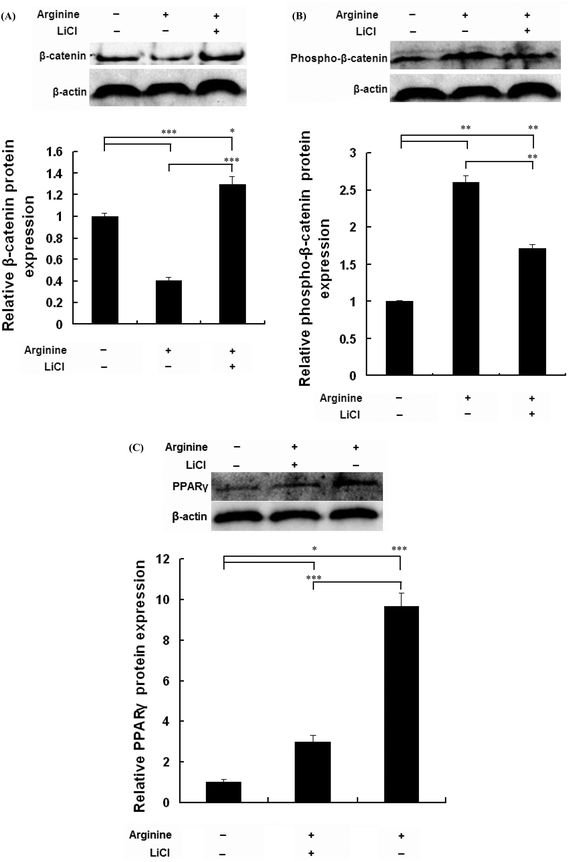 |
| Fig. 3 Arginine promotes porcine intramuscular preadipocyte differentiation through the Wnt/β-catenin signaling pathway. Porcine intramuscular preadipocytes were treated with 200 μg mL−1 of arginine and/or 20 mM of LiCl, and cultured in adipogenic medium for 8 d. The amount of β-catenin (A), phospho-β-catenin (B), and PPARγ (C) were analyzed by Western blotting. The results are presented as mean ± SE (n = 3). *p < 0.05, **p < 0.01, and ***p < 0.001 as compared with the control group. | |
Discussion
Over the course of the last few decades, the growth performance of domestic animals has been improved by genetic selection, although this advance has been at the expense of meat quality traits, namely IMF percentage.22 IMF is one of the important factors influencing meat quality, and it is positively correlated with the tenderness, juiciness and flavor of meat.23,24 The IMF content depends on the adipocyte number and lipid accumulation.25 There is some evidence that the number and size of porcine intramuscular preadipocytes are the main determinants of the muscle lipid content.26,27
During the terminal adipocyte differentiation, PPARγ and C/EBPα act as the master regulators of adipogenesis and lipid storage.28 Previous studies have provided convincing evidence that dietary or media supplementation with arginine increased expressions of adipocyte-specific genes, such as PPARγ, C/EBPα and LPL, as well as the content of IMF in skeletal muscle.14,16,18,19,29,30 In this study, the mRNA and protein expressions of PPARγ and C/EBPα were significantly increased by arginine supplementation, indicating that arginine could promote the differentiation of porcine intramuscular preadipocytes.
Previous studies have demonstrated that LiCl could activate the Wnt/β-catenin signal pathway, increase the accumulation of β-catenin, and subsequently lead to the inhibition of adipocyte differentiation through suppression of the adipogenic factors and PPARγ and CEBPα expressions.3,6,31 It has also been reported that Wnt/β-catenin signaling alters adipogenic differentiation primarily by suppressing the expressions of PPARγ and CEBPα.32,33 In the present study, we showed that the Wnt/β-catenin signal pathway was involved in arginine-regulated differentiation promotion of porcine intramuscular preadipocytes. We found that LiCl, a Wnt/β-catenin signaling specific activator, has a strong impact on the role of arginine in porcine intramuscular preadipocyte differentiation, suggesting that Wnt/β-catenin signaling plays a role in arginine-regulated differentiation promotion.
Conclusion
In conclusion, the results of this study suggest that arginine promotes adipogenic differentiation of porcine intramuscular preadipocytes and the Wnt/β-catenin signaling pathway may be involved in arginine-regulated differentiation promotion.
Acknowledgements
This work was supported by the National Natural Science Foundation of China (No. 31472108) and the Specific Research Supporting Program for Discipline Construction in Sichuan Agricultural University.
References
- E. D. Rosen and B. M. Spiegelman, Annu. Rev. Cell Dev. Biol., 2000, 16, 145–171 CrossRef CAS PubMed.
- J. L. Teo and M. Kahn, Adv. Drug Delivery Rev., 2010, 62, 1149–1155 CrossRef CAS PubMed.
- L. Qin, Y. Chen, Y. Niu, W. Chen, Q. Wang, S. Xiao, A. Li, Y. Xie, Y. Li, X. Zhao, Z. He and D. Mo, BMC Genomics, 2010, 11, 320 CrossRef PubMed.
- M. K. Kim, H. R. Choi, A. Park, S. M. Shin, K. H. Bae, S. C. Lee, I. C. Kim and W. K. Kim, Biophys. Res. Commun., 2013, 434, 455–459 CrossRef PubMed.
- M. B. Kim, Y. Song, C. Kim and J. K. Hwang, Biochem. Biophys. Res. Commun., 2014, 445, 433–438 CrossRef CAS PubMed.
- S. E. Ross, N. Hemati, K. A. Longo, C. N. Bennett, P. C. Lucas, R. L. Erickson and O. A. MacDougald, Science, 2000, 289, 950–953 CrossRef CAS PubMed.
- H. Lee, S. Bae and Y. Yoon, J. Nutr. Biochem., 2013, 24, 1232–1240 CrossRef CAS PubMed.
- G. Wu, F. W. Bazer, T. A. Davis, L. A. Jaeger, G. A. Johnson, S. W. Kim, D. A. Knabe, C. J. Meininger, T. E. Spencer and Y. Yin, Livest. Sci., 2007, 112, 8–22 CrossRef.
- W. W. Wang, S. Y. Qiao and D. F. Li, Amino Acids, 2009, 37, 105–110 CrossRef CAS PubMed.
- G. Wu, Amino Acids, 2009, 37, 1–17 CrossRef CAS PubMed.
- W. J. Fu, T. E. Haynes, R. Kohli, J. Hu, W. Shi, T. E. Spencer, R. J. Carroll, C. J. Meininger and G. Wu, J. Nutr., 2005, 135, 714–721 CAS.
- Q. H. He, X. F. Kong, G. Y. Wu, P. P. Ren, H. R. Tang, F. H. Hao, R. L. Huang, T. J. Li, B. Tan, P. Li, Z. R. Tang, Y. L. Yin and Y. N. Wu, Amino Acids, 2009, 37, 199–208 CrossRef CAS PubMed.
- W. J. Jobgen, C. J. Meininger, S. C. Jobgen, P. Li, M. J. Lee, S. B. Smith, T. E. Spencer, S. K. Fried and G. Y. Wu, J. Nutr., 2009, 139, 230–237 CrossRef CAS PubMed.
- B. Tan, Y. L. Yin, Z. Q. Liu, X. G. Li, H. J. Xu, X. F. Kong, R. L. Huang, W. J. Tang, I. Shinzato, S. B. Smith and G. Y. Wu, Amino Acids, 2009, 37, 169–175 CrossRef CAS PubMed.
- B. Tan, Y. L. Yin, Z. Q. Liu, W. J. Tang, H. J. Xu, X. F. Kong, X. G. Li, K. Yao, W. T. Gu, S. B. Smith and G. Y. Wu, J. Nutr. Biochem., 2011, 22, 441–445 CrossRef CAS PubMed.
- H. L. Lei, B. Yu, X. R. Yang, Z. H. Liu, Z. Q. Huang, X. B. Mao, G. Tian, J. He, G. Q. Han, H. Chen, Q. Mao and D. W. Chen, Sci. China: Life Sci., 2011, 54, 908–916 CrossRef CAS PubMed.
- A. M. Fouad, H. K. EI-Senousey, X. J. Yang and J. H. Yao, Animal, 2013, 7, 1239–1245 CrossRef CAS PubMed.
- J. E. Huh, J. Y. Choi, Y. O. Shin, D. S. Park, J. W. Kang, D. Nam, D. Y. Choi and J. D. Lee, Int. J. Mol. Sci., 2014, 15, 13010–13029 CrossRef CAS PubMed.
- X. Y. Ma, Y. C. Lin, Z. Y. Jiang, C. T. Zheng, G. L. Zhou, D. Q. Yu, T. Cao, J. Wang and F. Chen, Amino Acids, 2010, 38, 95–102 CrossRef CAS PubMed.
- X. L. Chen, Z. Q. Huang, H. Wang, G. Jia, G. M. Liu, H. Zhao and H. Nie, Biologia, 2013, 68, 1010–1014 CAS.
- K. J. Livak and T. D. Schmittgen, Methods, 2001, 25, 402–408 CrossRef CAS PubMed.
- C. R. Schwab, T. J. Baas, K. J. Stalder and J. W. Mabry, J. Anim. Sci., 2007, 85, 1540–1546 CrossRef CAS PubMed.
- H. W. Yong, A. B. Keren, R. Antonio, S. Gregory, T. Masaaki, M. Sean, M. William, M. Hideyuki, O. Kenji and A. L. Sigrid, Mamm. Genome, 2005, 16, 201–210 CrossRef PubMed.
- C. R. Schwab, B. E. Mote, Z. Q. Du, R. Amoako, T. J. Baas and M. F. Rothschild, J. Anim. Breed. Genet., 2009, 126, 228–236 CrossRef CAS PubMed.
- S. M. Niemela, S. Miettinen, Y. Konttinän, N. A. Ashammakhi and T. Ylikomi, J. Craniofac. Surg., 2007, 18, 325–335 CrossRef PubMed.
- F. Gondret and B. Lebret, J. Anim. Sci., 2002, 80, 3184–3193 CrossRef CAS PubMed.
- D. Gardan, F. Gondret and I. Louveau, Am. J. Physiol.: Endocrinol. Metab., 2006, 291, E372–E380 CrossRef CAS PubMed.
- I. Takada, A. P. Kouzmenko and S. Kato, Expert Opin. Ther. Targets, 2009, 13, 593–603 CrossRef CAS PubMed.
- K. Y. Chung, C. B. Choi, H. Kawachi, H. Yano and S. B. Smith, Adipocytes, 2006, 2, 93–100 CAS.
- X. Ma, C. Zheng, Y. Hu, L. Wang, X. Yang and Z. Jiang, PLoS One, 2015, 10, e0117294 Search PubMed.
- C. M. Hedgepeth, L. J. Conrad, J. Zhang, H. C. Huang, V. M. Lee and P. S. Klein, Dev. Biol., 1997, 185, 82–91 CrossRef CAS PubMed.
- S. Kang, C. N. Bennett, I. Gerin, L. A. Rapp, K. D. Hankenson and O. A. MacDougald, J. Biol. Chem., 2007, 282, 14515–14524 CrossRef CAS PubMed.
- M. Kawai, S. Mushiake, K. Bessho, M. Murakami, N. Namba, C. Kokubu, T. Michigami and K. Ozono, Biochem. Biophys. Res. Commun., 2007, 363, 276–282 CrossRef CAS PubMed.
|
This journal is © The Royal Society of Chemistry 2017 |
Click here to see how this site uses Cookies. View our privacy policy here.