DOI:
10.1039/C6FO01230B
(Paper)
Food Funct., 2017,
8, 177-188
Phenolics extracted from tartary (Fagopyrum tartaricum L. Gaerth) buckwheat bran exhibit antioxidant activity, and an antiproliferative effect on human breast cancer MDA-MB-231 cells through the p38/MAP kinase pathway†
Received
18th August 2016
, Accepted 28th November 2016
First published on 28th November 2016
Abstract
Phenolics extracted from tartary buckwheat (Fagopyrum tartaricum L. Gaerth) bran were analyzed quantitatively and qualitatively. The bioactivity of the phenolic extracts was evaluated, such as the antioxidant activity, and the inhibition capacity on the growth of cancer cells. The molecular mechanism for the inhibitive effect on cancer cells was explored. Results indicated that tartary buckwheat bran phenolics mainly exist in a free form, and free phenolics were twice as abundant as bound phenolics. Free caffeic acid (119.75 μg per 100 mg DW) and bound rutin (51.66 μg per 100 mg DW) represented the main free and bound phenolic compounds, respectively. The free phenolic extract contributed to the major (>90%) antioxidant activities including the oxygen radical antioxidant capacity (ORAC) and cellular antioxidant activity (CAA). The free phenolic extract exhibited anticancer activity for human breast cancer MDA-MB-231 cells in a dose-dependent manner. This significant inhibition effect was achieved through the p38/MAP kinase pathway by inducing cell apoptosis (up-regulating p-p38 and p-ASK1 expressions and down-regulating TRAF2 and p-p53 expressions), and negatively regulating the progression of the cell cycle from the G1 to S phase (increased expression of p21 and suppressed expressions of PCNA, cyclin D1 and CDK4). All these results indicated that tartary buckwheat bran could be a rich resource of natural antioxidants and inhibitors for the growth of MDA-MB-231 cells.
1. Introduction
Tartary buckwheat (Fagopyrum tartaricum L. Gaerth) has received increasing attention due to its rich nutritional content and high potential against chronic diseases such as diabetes, cancer, acute myelogenous leukemia and inflammation.1–4 Although tartary buckwheat bran, a milling by-product, is often discarded, it shows higher phenolic content than buckwheat flour, wheat flour or wheat bran.5,6 The content or composition of buckwheat phenolics varied depending on the buckwheat species, growing area, climate and growing conditions.7
Studies showed that the phenolics extracted from various fractions (i.e., hull, bran, flour) or products (i.e., noodles etc.) of tartary buckwheat grain showed antioxidant activities measured by various chemical assays such as DPPH, ORAC, FRAP, and ABTS.8–11 Compared to chemical methods, the cellular antioxidant activity (CAA) experiment more accurately reflects the antioxidant activity in vivo, as it accounts for the uptake, metabolism and distribution of antioxidants under physiological conditions.12 However, little information on the CAA of phenolics extracted from tartary buckwheat bran has been reported.
Cancer is a worldwide health problem, it is estimated that about 30% of all cancers have been attributed to diet, which suggests that changes in dietary composition such as increasing consumption of foods rich in bioactive phytochemicals, are practical strategies to decrease the incidence of cancers.13 Studies revealed that plant phytochemicals might account for the inhibitory effects in the promotion and progression of carcinogenesis.3,14 There are complementary mechanisms for cancer prevention by phytochemicals, such as scavenging free radicals, deactivation and removal of carcinogens, inhibition of cell proliferation, regulation of cell cycles and gene expression through signal transduction pathways, induction of apoptosis, inhibition of nuclear factor κB activation and so on.14–16
The anticancer activity of tartary buckwheat phytochemicals (i.e., protein, phenolics and phenylpropanoid glycosides) has been evaluated in diverse cancerous cell lines including breast cancer cell lines Bcap37, human hepatoma cells HepG2, and human cancer cell lines A549, HL-60, HCT116, and ZR-75.2,17,18 The anticancer capacity of tartary buckwheat phenolics on HepG2 cells was triggered by inducting the G2/M arrest, apoptosis and generation of cell reactive oxygen species.2 However, to the best of our knowledge, the anticancer activity of phenolics extracted from tartary buckwheat bran against human breast cancer MDA-MB-231cells and its mechanisms were still unclear.
We evaluated the anticancer activity of phenolics extracted from tartary buckwheat bran against MDA-MB-231 cells, and discussed whether the inhibited cell proliferation occurred through the p38 MAPK pathway. The phenolic composition and cellular antioxidant activity were also analyzed.
2. Materials and methods
2.1 Main chemicals
The Folin–Ciocalteu reagent, gallic acid, 6-hydroxy-2,5,7,8-tetramethylchroman-2-carboxylic acid (Trolox), fluorescein disodium salt, 2′,7′-dichlorofluorescin diacetate (DCFH-DA), methylene blue, 4-(2-hydroxyethyl)-1-piperazineethanesulfonic acid (HEPES) and insulin were obtained from Sigma-Aldrich, Inc. (St Louis, MO, USA). 2,2′-Azobis (2-amidinopropane) dihydrochloride (ABAP) was purchased from Wako Chemicals USA, Inc. (Richmond, VA). Minimum Essential Medium alpha (α-MEM), Williams’ Medium E (WME), fetal bovine serum (FBS), Hanks’ balanced salt solution (HBSS), and antibiotic-antimycotic (100×) were supplied by Gibco Life Technologies (Grand Island, NY, USA). Acetonitrile and methanol (HPLC grade) were purchased from Merck (Darmstadt, Germany). Phenolic standards were purchased from Chengdu Biopurify Phytochemicals (Chengdu, China). Other chemicals and reagents used were obtained from recognized distributors and were of analytical grade.
2.2 Antibodies
The primary antibody against proliferating cell nuclear antigen (PCNA) was purchased from Calbiochem (Billerica, MA, USA). Others, including cyclin-dependent kinases-4 (CDK4), cyclin D1, p21, phospho-p53 (p-p53), phospho-p38 (p-p38), phospho-ASK1 (p-ASK1) and TRAF-2 were purchased from Santa Cruz Biotechnolgy, Inc. (Santa Cruz, CA, USA). β-Actin, HRP-conjugated secondary antibodies, anti-mouse IgG, anti-rabbit IgG were obtained from Sigma-Aldrich Inc. (St Louis, MO, USA).
2.3 Cell culture
Human hepatoma HepG2 cells and human breast cancer MDA-MB-231 cells were obtained from the American Type Culture Collection (Rockville, MD, USA). HepG2 cells were kept in WME medium containing 5% heat-inactivated fetal bovine serum (FBS), 10 mmol L−1 HEPES, 2 mmol L−1L-glutamine, 5 μg mL−1 insulin, 0.05 μg mL−1 hydrocortisone, 50 units per mL penicillin, 50 μg mL−1 streptomycin at 37 °C and 5% CO2. MDA-MB-231 cells were maintained in α-MEM medium containing 10% FBS, 10 mmol L−1 HEPES, 50 unitsper mL pencilin, 50 mL streptomycin at 37 °C and 5% CO2. Cells between passages 12 and 35 were used.
2.4 Preparation of tartary (Fagopyrum tartaricum L. Gaerth, Youyang 1#) buckwheat bran
Whole grains of tartary (Fagopyrum tartaricum L. Gaerth, Youyang 1#) buckwheat were harvested from Youyang county, Chongqing city at its maturation stage (Nov. 2011). The tartary buckwheat grains were crushed in a blender (JYL-A110, Jiuyang, CN) for 4 min. The resultant granules were fractioned into three parts (hull on the first sieve, bran on the second sieve and flour under the second sieve) by successively passing through a 40-mesh sieve and a 60-mesh sieve. The bran was collected, sealed in a plastic bag, and stored at −80 °C for further analysis within three months.
2.5 Extraction of free and bound phenolics
The free and bound phenolics were extracted using the methods reported by Adom and Liu19 with a slight modification. Briefly, the tartary buckwheat bran (1.0 g) was mixed with 50 mL of 80% chilled acetone for 10 min. Then, the mixture was centrifuged at 3500g for 10 min and the supernatant was collected. The residue was repeatedly extracted twice with 50 mL of 80% chilled acetone under similar conditions. All supernatants were combined and evaporated at 45 °C under reduced pressure. The concentrated slurry was freeze-dried and stored at −80 °C until analysis within three months. For the extraction of the bound phenolics, 20 mL of 2 mol L−1 NaOH was added to the residues from the free phenolics extraction for 1.5 h, with continuous shaking and N2 purging at room temperature. The mixture was neutralized with concentrated HCl (pH 2.0–3.0) in the cooling ice bath, followed by adding 20 mL of hexane to remove any lipids. The bound phenolics were extracted by 100 mL of ethyl acetate five times. The ethyl acetate fractions were collected and evaporated at 45 °C under reduced pressure and reconstituted in 10 mL of deionized water. The extracts were stored at −80 °C until analysis within three months.
2.6 Determination and characterization of free and bound phenolics
Phenolic contents were quantified using the Folin–Ciocalteau colorimetric method described previously.20 In brief, 0.2 mL the extract diluent was added to 0.2 mL Folin–Ciocalteu reagents. Then 7% of sodium carbonate was added, the mixture left at room temperature for 1.5 h in the dark. The absorbance was measured at 760 nm (722 visible spectrophotometer, Jinghua Instruments Inc., Shanghai, CN). Results are expressed as milligrams of gallic acid equivalents per gram dry weight of sample (mg GAE per g DW).
2.7 Phenolic compounds determination by HPLC analysis
HPLC analysis was performed by Shimadzu series liquid chromatography with SPD-M20A diode-array detector (LC-20A, Shimadzu, Inc., JP). The extract solutions were filtered through a 0.2 μm syringe filter (Gelman Laboratory, Ann Arbor, MI, USA) before being separated by Thermo BDS C18 column (250 × 4.6 mm, 5 μm). The mobile phases were 100% acetonitrile (Elute A) and 0.4% (v/v) acetic acid (Elute B). The flow rate was kept at 1.0 mL min−1 and column temperature was at 30 °C. The injection volume was 40 μL. The gradient elution procedures were performed as follows: 0–5 min, 3% A; 5–10 min, 3%–7% A; 10–25 min, 7%–10% A; 25–30 min, 10%–12% A; 30–45 min, 12%–15% A; 45–50 min, 15% A; 50–70 min, 15%–30% A; 70–75 min, 30%–3% A; 75–80 min, 3% A. Phenolic compounds were identified with DAD spectra (280 nm wavelength) and a retention time (Rt) similar to those of the phenolic standards. The concentrations of all the detected phenolic compounds in the extracts were quantified using standard curves (R2 > 0.9949) and expressed as microgram per 100 milligram dry weight of tartary buckwheat bran (μg per 100 mg DW).
2.8 Oxygen radical antioxidant capacity (ORAC) assay
ORAC of buckwheat bran was measured using the method described by Prior et al.21 Briefly, 20 μL of blank, Trolox standard, or buckwheat bran extract in 75 mmol L−1 potassium phosphate buffer (PBS, pH 7.4), was added into a 96-well microplate at the specified position. 200 μL of 0.96 μmol L−1 fluorescein was added into each well and incubated at 37 °C for 10 min, followed by the addition of 20 μL of freshly prepared 119 mmol L−1 ABAP. The microplate was immediately inserted into a fluoroskan ascent plate reader (Synegry H1, BioTek Instruments, Inc., Winooski, VT, USA) at 37 °C. The decay of fluorescence at 538 nm with excitation at 485 nm was measured every 4.5 min for 2.5 h. To quantify the antioxidant capacity, a calculation of areas under the fluorescence versus time curve for the samples minus the area under the curve for the blank was performed. The ORAC value was expressed as micromoles of Trolox equivalents (TE) per gram dry weight of sample (μmol TE per g DW).
2.9 Cellular antioxidant activity (CAA) assay
The CAA of the extracts was measured using the method developed by Wolfe and Liu.12 HepG2 cells were seeded at a density of 6 × 104 per well on a 96-well black and clear-bottom micro plate. After incubating for 24 h, the cells were washed by fresh PBS and treated with 100 μL of medium containing the extracts and 25 μmol L−1 DCFH-DA for one hour. Wells were washed with or without PBS, followed by adding 600 μmol L−1 ABAP in 100 μL of HBSS. The micro plate was then inserted into a fluoroskan ascent plate reader (Spectra Max M5e, Molecular Devices Inc., Sunnyvale, CA, USA) at 37 °C. Emission at 538 nm was measured after excitation at 485 nm every 5 min for one hour. The CAA values were expressed as micromoles of quercetin equivalents per 100 g dry weight of tartary buckwheat bran (μmol QE per 100 g DW).
2.10 Cytotoxicity and antiproliferative activity
The cytotoxicity of the phenolic extracts on human breast cancer MDA-MB-231 cells and human liver cancer HepG2 cells were measured using the methylene blue method described by Wolfe and Liu.12 Cells were seeded at 4 × 104 per well on a 96-well plate and incubated for 24 h at 37 °C and 5% CO2. Then cells were washed by PBS, treated with graduated concentrations of the phenolic extracts and incubated for 24 h. Cells were washed using PBS and stained by 50 μL per well of methylene blue solution (98% HBSS, 0.67% glutaraldehyde, and 0.6% methylene blue) for one hour at 37 °C. Finally, the methylene blue stain was eluted by buffer (50% ethanol, 49% PBS, and 1% acetic acid) for 30 min at room temperature. Absorbance was measured at 570 nm in MRX II Dynex plate reader (Dynex Technologies, Inc., Chantilly, VA, USA). A 10% or greater decrease in absorbance was considered toxic, when compared with the control. Data was collected in triplicate for statistical analysis.
The antiproliferative effect of the phenolic extracts was measured using the methylene blue method described by Felice et al.22 Human breast cancer MDA-MB-231 cells were plated into a 96-well plate at a density of 2.5 × 104 cells per well and incubated for 6 h. Then fresh growth mediums containing phenolic extracts were added and incubated for 72 h. Then, the cells were rinsed with cold PBS and stained with 50 μL per well of methylene blue solution (98% HBSS, 0.67% glutaraldehyde, and 0.6% methylene blue) for one hour. Stained cells were eluted by 50% ethanol, 49% PBS, and 1% acetic acid for 30 min. The absorbance was measured at 570 nm in a MRX II Dynex plate reader (Dynex Technologies, Inc., Chantilly, VA, USA). Cell proliferation was expressed as the percentage of the sample absorbance versus the control absorbance. The median effective doses (EC50) were calculated and expressed as milligrams of the extract powder per milliliter of the extract. All data were collected in triplicate for statistical analysis.
2.11 Immunoblotting analysis
The protein analysis and western blotting were followed the protocol reported by Liu et al.23 Briefly, human breast cancer MDA-MB-231 cells were plated in 6-well plates and incubated for 6 h at 37 °C in 5% CO2. Then, the medium was replaced by fresh α-MEM containing the free phenolics extract at the designed concentrations. After 48 h or 60 h treatment (varies depending on the target proteins), the cells were rinsed twice by cold PBS, collected and lysed by RIPA buffer (50 mmol L−1 Tris Base, 1% Igepal, 150 mmol L−1 sodium chloride, 1 mmol L−1 EDTA, pH = 7.4) with protease inhibitors (1 mg mL−1 aprotinine, 1 mg mL−1 leupeptin, 1 mg mL−1 pepstain, 200 mmol L−1 sodium orthovanadate). The cell suspensions were shaken periodically for 30 min, centrifuged at 12
000g for 15 min at 4 °C, then the supernatant was collected as cytoplasmic protein stored at −20 °C. Protein concentrations of the lysates were determined using a Sigma Diagnostics Micro Protein Determination Kit and a FilterMax F5 Multi-Mode microplate reader (Molecular Devices). The proteins were subjected to 10% or 12% (w/v) sodium dodecyl sulfate-polyacrylamide gel electrophoresis (SDS-PAGE) where 100 V were applied for 1.5 h to 2 h. After electrophoresis, proteins were transferred to polyvinylidene fluoride (PVDF) membrane in a 20% methanol transfer buffer solution at 100 V for 50 min. Then, the membrane was blocked by 5% non-fat milk dissolved in Tris-buffered saline with Tween-20 (TBST) at the room temperature for 2 h. The primary antibody were applied to the protein membrane at a dilution of 1
:
1000 overnight at 4 °C, followed by the corresponding secondary antibody in 5% non-fat milk incubation for 2 h at room temperature. Western blot results were revealed by Lumi GLO chemiluminescent substrate (Cell Signaling, Beverly, MA, USA) followed by Phototope-HRP detection assay. Protein expression was visualized on Kodak Biomax MR Film (Kodak, Rochester, NY, USA) by developing and fixing process. The bands were analyzed by ImageJ2x software (Wayne Rasband, National Institutes of Health, Bethesda, MD, USA).
2.12 Statistical analysis
Data were analyzed using Sigmaplot software version 12.5 (Aspire Software International, Ashburn, VA), ANOVA and Tukey's test, and the Pearson's correlation coefficient (r) were performed using SPSS Statistics 17.0 version (IBM, USA). Deference with p < 0.05 was considered to be statistically significant. Data are presented as the mean ± SD for at least three independently performed experiments.
3. Results and discussion
3.1 Total phenolic content (TPC)
As shown in Table 1, the amount of free, bound, and total phenolics extracted from the tartary buckwheat bran were 24.6, 1.8 and 26.4 mg GAE per g DW, respectively. It indicated that phenolics in the tartary buckwheat bran mainly existed in a free form. Similar results were also found in tartary buckwheat hull, coarse bran, fine bran and light flour or tartary buckwheat whole grain growing in different locations.24,25 Free phenolics account for 85%–89% of TPC in black rice bran.26 However, the amount of bound phenolics was much higher than free phenolics in rice bran, corn bran and wheat bran.27–29 On the other hand, the TPC of the tartary buckwheat bran was even higher than the TPC of fruits such as apple, pear and grapes, as well as vegetables.30
Table 1 Contents and antioxidant activities of phenolics extracted from tartary (Fagopyrum tartaricum L. Gaerth, Youyang 1#) buckwheat bran (mean ± SD, n = 3)
|
Phenolicsa (mg GAE per g DW) |
ORACa (μmol TE per g DW) |
CAAb (μmol of QE per 100 g) |
Antiproliferative activity (EC50) of MDA-MB-231b (mg mL−1) |
Cytotoxicity of (CC50) HpeG2 b (mg mL−1) |
Cytotoxicity of (CC50) MDA-MB-231b (mg mL−1) |
PBS wash |
Non-PBS wash |
Values are converted to the amount of the bran.
Values are expressed as the amount of the freeze-dried powder of the phenolic extract.
Values in parentheses indicate percentage to the total.
“nc” means not be calculated.
|
Free |
24.6 ± 0.3 (93.2%)c |
68.2 ± 5.2 (90.5%) |
34.5 ± 1.8 |
39.8 ± 2.4 |
2.9 |
21.9 |
7.9 |
Bound |
1.8 ± 0.2 (6.8%) |
7.2 ± 0.7 (9.5%) |
ncd |
nc |
nc |
nc |
nc |
Total |
26.4 ± 0.4 |
75.3 ± 5.8 |
|
|
|
|
|
This indicates that the tartary buckwheat bran could be a rich source of phenolics, particularly for free phenolics.
3.2 Qualitative analysis of phenolic compounds by HPLC method
Phenolic acids and flavonoids in the extracts of tartary buckwheat bran were qualified by the HPLC method. The identification of these phenolic compounds was performed by comparing their retention time (Rt.) and UV spectra (λnm) with those of the standards, and chromatograms of the free and bound extract are shown in Fig. 1B and C, respectively.
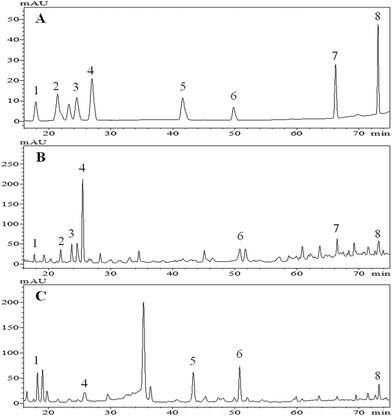 |
| Fig. 1 Base peak chromatograms (BPC) of phenolic standards (A), free phenolic compounds (B) and bound phenolic compounds (C) of tartary (Fagopyrum tartaricum L. Gaerth, Youyang 1#) buckwheat bran. Peaks (peak 1 to peak 8) sequentially represent p-hydroxybenzoic acid, catechin, vanillic acid, caffeic acid, ferulic acid, rutin, resveratrol and quercetin, respectively. These peak numbers coincide with the compound numbers in Table 2. | |
A total of eight phenolic compounds were identified in the tartary buckwheat bran (Fig. 1). Vanillic acid (peak 3), catechin (peak 2) and resveratrol (peak 7) were only detected in the free extract. Ferulic acid (peak 5) was only found in the bound. p-Hydroxybenzoic acid (peak 1), caffeic acid (peak 4), rutin (peak 6) and quercetin (peak 8) were found in both the free and bound extract.
The above eight phenolic compounds were detected in tartary buckwheat by others.24,31–33 However, Guo et al., reported that vanillic acid and ferulic acid were detected in both the free and bound phenoic extract of tartary buckwheat bran (Chuanqiao #1).24 This inconsistency might correspond to factors such as cultivars, growing phases and planting environment, and levels of enzymes involved in the phenolic compound metabolism of the plant, that influenced the phenolic composition and/or existing forms (free, soluble-conjugated, and bound forms) in the plants.25,34,35 In addition, the absence of vanillic acid, catechin and resveratrol in the bound phenolic extract probably resulted from the extraction conditions. It was found that the acidified procedure after alkaline extraction may result in the precipitation of the proteins, and phenolic compounds coupled with protein, therefore, could be discarded with the precipitate.36
Above all, the buckwheat free extract contained richer phenolic compounds than the bound, and a similar result was reported by Inglett et al.36 Apart from phenolic compounds detected here, there are probably much more or new phenolic compounds in the tartary buckwheat bran ready to be explored. As suggested by Zhang et al., techniques such as reverse phase HPLC coupled to electrospray ionization-time of flight-mass spectrometry (ESI-TOF-MS) and other high effective technologies could contribute to the accurate and comprehensive identification of buckwheat phenolic compounds.37
3.3 Quantification analysis of phenolic compounds by HPLC method
The quantitative data of the detected phenolic compounds were obtained using external calibration curves obtained for each phenolic standard, and results are shown in Table 2.
Table 2 Qualitative and quantification analysis of phenolic compounds detected in tartary (Fagopyrum tartaricum L. Gaerth, Youyang 1#) buckwheat bran extracts by HPLC method
No. |
Rt. (min) |
HPLC-DAD (λnm max) |
Phenolic compounds |
Regression equations, R2 |
Content (μg per 100 mg DW) |
Free |
Bound |
Values in parentheses indicate percentage contribution to the total (free + bound).
nc = not calculated.
|
1 |
17.8 |
198 254 315 |
p-Hydroxybenzoic acid |
y = 161 886x − 29 447, R2 = 0.9998 |
8.05 ± 0.30 (2.3)a |
13.51 ± 0.08 (3.8) |
3 |
24.4 |
239 322 |
Vanillic acid |
y = 117 274x + 58 743, R2 = 0.9997 |
19.44 ± 0.12 (5.5) |
ncb |
4 |
26.2 |
221 280 321 |
Caffeic acid |
y = 66 215x − 92 574, R2 = 0.9963 |
119.75 ± 1.63 (33.8) |
16.83 ± 0.56 (4.7) |
5 |
42.3 |
240 322 |
Ferulic acid |
y = 135 104x − 26 427, R2 = 0.9991 |
nc |
26.88 ± 0.43 (7.6) |
|
|
|
Total phenolic acids
|
|
147.24 (41.6)
|
57.22 (16.1)
|
|
2 |
21.8 |
234 278 |
Catechin |
y = 145 677x − 11 855, R2 = 0.9999 |
10.38 ± 0.14 (2.9) |
nc |
6 |
50.2 |
255 282 352 |
Rutin |
y = 67 925x − 38 416, R2 = 0.9949 |
36.62 ± 1.25 (10.3) |
51.66 ± 2.17 (14.6) |
7 |
66.2 |
236 306 |
Resveratrol |
y = 157 070x − 37 540, R2 = 0.9968 |
28.40 ± 0.27 (8.0) |
nc |
8 |
73.1 |
254 370 |
Quercetin |
y = 221 031x + 614 469, R2 = 0.9981 |
13.64 ± 0.19 (3.8) |
9.40 ± 0.63 (2.7) |
|
|
|
Total flavonoids
|
|
89.04 (25.0)
|
61.06 (17.3)
|
|
|
|
Total polyphenolos
|
|
236.28 (66.6)
|
118.28 (33.4)
|
The concentration of total phenolic compounds obtained by the HPLC method (354.56 μg per 100 mg DW) was drastically lower than that estimated by the Folin–Ciocalteau method (26.4 mg GAE per g DW) (p < 0.05) (Tables 1 and 2). The Folin–Ciocalteau method might overestimate the phenolic contents due to the interference of non-phenolic compounds (i.e., sugars, aromatic amines, sulphur dioxide, ascorbic acid, organic acids, and iron) contained in the crude extract.38
With respect to the contents of free/bound phenolic acids and flavonoids, free phenolic acids (147.24 μg per 100 mg DW) were approximately twice as abundant as the free flavonoids (89.04 μg per 100 mg DW). There were slightly, but not significantly, more bound flavonoids (61.06 μg per 100 mg DW) than bound phenolic acids (57.22 μg per 100 mg DW) and (p < 0.05) (Table 2). There was approximately twice the amount of free phenolics (236.28 μg per 100 mg DW) compared to the bound (118.28 μg per 100 mg DW). This indicated that free phenolic acids were the main phenolics in the tartary buckwheat bran extract.
As for the concentration of detected individual phenolic compounds, caffeic acid (136.58 μg per 100 mg DW) presented as the main phenolic acid, and rutin (88.28 μg per 100 mg DW) was the major flavonoid, which accounted for about 38.5% and 24.9% of total concentration of detected phenolics, respectively.
Above all, our results indicated the detected phenolic compounds in the tartary buckwheat bran extract mainly existed in a free form with higher concentrations than that of the bound. This result was consistent with the literature.25,39 As suggested by researchers, free phenolic compounds might be easily digested in the upper gastrointestinal tract, and bound phenolic compounds might reach the colon to exert their health benefits.19 Therefore, it could infer that phenolic compounds in the tartary buckwheat bran might be more readily available in the upper gastrointestinal tract.
3.4 Antioxidant activity
3.4.1 Oxygen radical absorbance capacity (ORAC).
The oxygen radical absorbance capacity of both free and bound phenolic extracts was quantified and shown in Table 1. The free phenolics extract contributed to 90.5% of the total oxygen radical absorbance capacity with an ORAC value of 68.2 μmol TE per g DW, which was approximately ten times higher than that of the bound extract (7.2 μmol TE per g DW). Previous researchers reported that bound phenolic of oat flour and whole wheat displayed the main antioxidant activities, and the major antioxidant phenolics in rice bran were either free or bound phenolics depending on the rice cultures.40–42 The higher concentration of free phenolics in the free extract might contribute to its stronger oxygen radical scavenging activity, since there was close correlation between the concentration of phenolics and their antioxidant activity, as suggested by other researchers.40,41
3.4.2 Cellular antioxidant activity (CAA).
The CAA assay was performed fundamentally based on the mechanism that antioxidants could quench peroxyl radicals and inhibit the generation of fluorescent DCF, where the level of fluorescence is negatively proportional to the level of antioxidant capacity.15 The cellular antioxidant activities were measured using two protocols: the PBS-wash and non-PBS wash procedures, the former showed the intracellular antioxidant capacities, and the latter mainly revealed the antioxidant effects on the cell membranes (extracellular antioxidant capacities).12,15
The time and dose–response kinetics of fluorescent probe oxidation in HepG2 cells exposed to various concentrations of quercetin (the standard) and the free phenolics extract were shown in Fig. 2. The quantified cellular antioxidant activity (CAA value) was presented in Table 1.
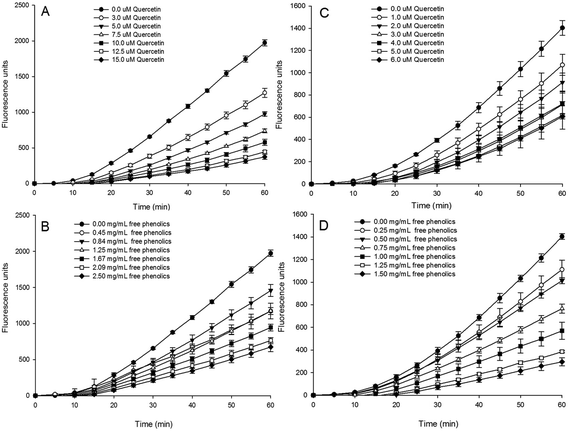 |
| Fig. 2 The inhibitory effects of quercetin (A, C) and free phenolics (B, D) extracted from tartary (Fagopyrum tartaricum L. Gaerth, Youyang 1#) buckwheat bran on the peroxyl radical-induced oxidation of DCFH to DCF in HepG2 cells. A and B used the protocol involving a PBS wash, C and D used the protocol involving no PBS wash. | |
Visually, the increase in fluorescence was inhibited by quercetin (Fig. 2A and C) and the free extract (Fig. 2B and D) in a dose-dependent manner in both the PBS wash (Fig. 2A and B) and non-PBS wash (Fig. 2C and D) protocols. This result indicates that the free phenolics extract of tartary buckwheat bran could quench oxygen radicals involved in the cell or on the cell surface.
A significantly (p < 0.05) higher CAA value of the free phenolics extract was found in the non-PBS wash protocol (39.8 μmol QE per 100 g DW) than that of the PBS wash (34.5 μmol QE per 100 g DW). It indicated that the cellular antioxidant capacity of the free phenolics extract mainly took place on the cell membrane. The difference in CAA values between the PBS wash protocol and no PBS wash protocol might be related to the different cellular bioavailability of the phytochemical compounds12,43,44 Epicatechin and procyanidine B2, due to their low cell uptake rate or loosely associated with the cell membrane, displayed weak cellular antioxidant capacity and a significant difference (p < 0.05) between the two protocols of CAA assay.12 Wen et al., found that the presence of proanthocyanidins probably improved the cell antioxidant activity of quercetin due to the improvement of the solubility and stability of quercetin by proanthocyanidins addition.45
With respect to the bound phenolic extract, it did not show extra/intracellular antioxidant properties in either the PBS wash or the non-PBS wash procedures in non-cytotoxic concentrations (not shown). A similar result was found in the bound phenolic extracts of other grains, such as corn, rice, wheat, oats, barley, quinoa, amaranth, and common buckwheat.44 The much lower level of TPC and the lower number of phenolic compounds in the bound phenolics extract might be responsible for its weaker cellular antioxidant activity, when compared to the free phenolics extract (Table 2, Fig. 1). Upon further analysis, researchers concluded that the cellular antioxidant activity of the phytochemicals depends on their efficiency of cellular uptake and/or membrane binding combined with their radical scavenging activity, and their physical properties such as hydrophobicity, polarity, structural principles, etc. Hydrophobic flavonoids and more polar compounds may become deeply embedded in membranes or interact with membrane surfaces (high bioavailability), where they are able to protect membranes from external and internal oxidative stresses and therefore exhibit high cellular antioxidant activity. It was found that flavonoids (i.e., quercetin, kaempferol and epicatechin etc.) with a hydroxyl group at the 3 position of the C-ring and a keto group and the 4 position of the C-ring had a high antioxidant effectiveness in the CAA assay. In particular, quercetin was the most efficacious antioxidant in the CAA assay.46 Caffeic acid, due to the presence of two hydroxyl groups located next to each other on the aromatic ring, also showed effective antioxidant activity in the cell culture.44,46 Therefore, quercetin and caffeic acid probably contribute most of the antioxidant activity in the cell culture, and the higher concentration of quercetin and caffeic acid in the free phenolic extract might account for its higher CAA values compared with the bound extract (Tables 1 and 2). In addition, caffeic acid adsorbs loosely to the cell membrane and is taken up less readily, which might explain why the cellular antioxidant capacity of the free phenolics extract mainly took place on the cell membrane (non-PBS wash).12 Moreover, the interaction among phenolic compounds or other phytochemical compounds present in the crude extract might also account for its cellular antioxidant activity.47
These above results indicate that the free phenolics extract was superior in both content and composition, as well as antioxidant capacities (Tables 1 and 2) compared to the bound phenolics. Therefore, it could be inferred that the free phenolics of the tartary buckwheat bran extract were probably the major contributor to its bioactivity. Hence, only the free phenolics extract was evaluated for its in vitro antiproliferative activity against cancer cell lines.
3.5 Antiproliferative activity against human breast cancer MDA-MB-231 cells
As shown in Fig. 3A, there was an obvious decrease in the number of MDA-MB-231 cells when exposed to the free phenolics extract compared to the control cells, and the reduction in cell density and number of living cells followed a dose-effect manner.
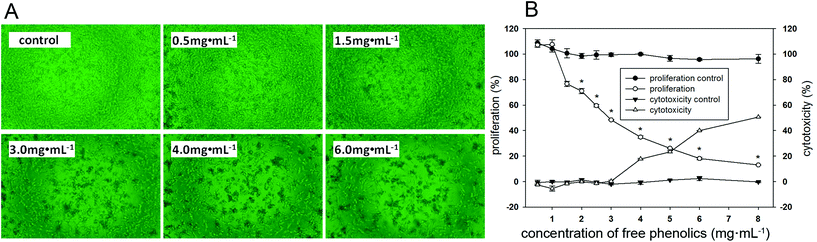 |
| Fig. 3
In vitro antiproliferative activity against human breast cancer MDA-MB-231 cells. (A) Photomicrographs of the antiproliferative activity against MDA-MB-231 cells treated with different concentrations of free phenolics extracted from tartary (Fagopyrum tartaricum L. Gaerth, Youyang 1#) buckwheat bran. (B) Effect of the free phenolics on cell proliferation and cytotoxicity in MDA-MB-231 cells. An asterisk (*) indicates a significant difference from the control (p < 0.05). | |
MDA-MB-231 cells presented 24%–52% inhibition rates when exposing 1.5–3.0 mg mL−1 of the free phenolics extract, this inhibitory effect was attributed to its antiproliferative effects (Fig. 3B). However, the inhibition effect on the proliferation of MDA-MB-231 cells by the free phenolics extract at or higher than 4 mg mL−1 might be mainly due to the cell cytotoxicity, since the cell viability reduced by approximately 17% compared to the control in the cell cytotoxicity assay.
It was noted that the concentration of free phenolics extract at the median effective dose (EC50 = 2.9 mg mL−1) had no cytotoxic effect. The proliferative inhibition effect of buckwheat phenolic extracts has also been reported on human hepatoma cancer cells, colon cancer cells, lung cancer cells, leukemia cancer cells and HL-60 cells.2,48,49
Studies have revealed that the proliferative inhibition effect of phenolic compounds on cancer cells is through multifactorial pathways, including the induction of apoptosis, regulation of the cell cycle, alteration of the activity of certain intracellular enzymes and the modulation of cellular signaling pathways relating to DNA damage repair.50–52 It has been found that catechin hydrate possesses anticancer effects in breast cancer cells through TP53/caspase-mediated apoptosis.53 Rutin showed a remarkable induction of hepatic cancer cell apoptosis.2 Quercetin could induce apoptotic hepatic cancer cell death.54 Ferulic acid displayed anticancer activity by reducing cell viability.55 Caffeic acid demonstrated anti-proliferative activities by means of inducing cell growth arrest and apoptosis.56 Meanwhile, vanillic acid could suppress neuro-2A cell apoptosis.57
Therefore, it could be inferred that phenolic compounds in the tartary buckwheat bran extract were closely related to its proliferation inhibition activity against MDA-MB-231cells. The synergistic, additive and antagonistic effects among phenolic compounds and/or non-phenolics in the crude extract might also contribute to its anti-proliferative activities in cancer cells.58–60 The proliferative inhibition effect of the free phenolics extracted from the tartary buckwheat bran on MDA-MB-231 cells was associated with its regulation effect on the cell cycle and/or apoptosis, which was further analyzed in the following text.
3.6 Effects of the free phenolics extract on the expression of proteins involved in the proliferation and cell cycle of MDA-MB-231 human breast cancer cells
The inhibition properties of phytochemicals on cancer cells could be revealed by retarding the cell cycle progression, inhibiting phase I enzymes, the induction of phase II enzymes, scavenging DNA reactive agents and so on.61,62
The proliferating cell nuclear antigen (PCNA) is involved in several metabolic pathways, including DNA repair, translesion DNA synthesis, chromatin remodeling and cell cycle regulation, and the excessive expression PCNA is closely related to the proliferation of cancer cells.63 Cyclin D1 and cyclin-dependent kinases-4 (CDK4) are essential regulators that control the G1to S transition of the cell cycle.64 The inhibited expression of cyclin D1 and CDK4 could cause cells to arrest in the G1 phase of the cell cycle. The protein p21, known as a cyclin-dependent kinases inhibitor, could inhibit the transcription of the cyclin D1 gene.65 Investigation on the expression of cell proliferation-related PCNA, cell cycle-related cyclin D1, CDK4 and protein p21 might fundamentally explain the mechanism of antiproliferation from the view of the cell cycle.
The expressions of PCNA, cyclin D1, CDK4 and p21 were measured by western blotting analysis to confirm the antiproliferative activity of the free phenolics extract against human breast cancer MDA-MB-231 cells. As shown in Fig. 4, the protein expressions of both PCNA (Fig. 4A), cyclin D1 (Fig. 4B) and CDK4 (Fig. 4C) were down-regulated in a dose dependent manner within non-cytotoxic concentrations of the free extract. In particular, comparing the control (cells incubated in WME medium), and the group receiving 3 mg mL−1 of free phenolics exxtract, the protein expressions of PCNA, cyclin D1 and CDK4 exposed to the extract were inhibited to 63.3%, 68.4% and 71.0%, respectively (p < 0.05), which might thereby induce the antiproliferation and G1 cell cycle arrest. Significant improvement in the expression of p21 (Fig. 4D) was observed in each concentration (1.5–3.0 mg mL−1), particularly when incubated in 3 mg mL−1 of free phenolics extract, where the expression of p21 increased by about three times.
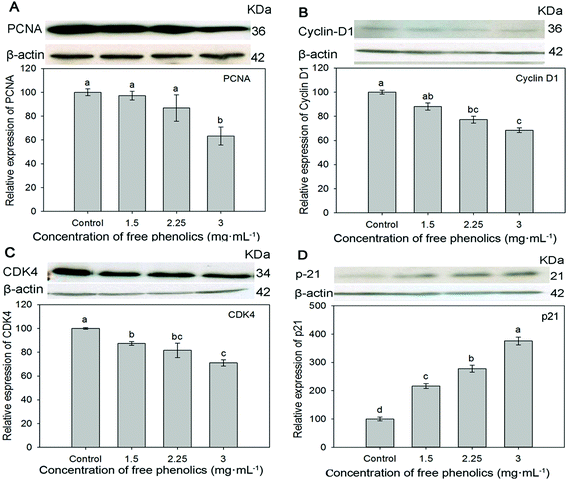 |
| Fig. 4 Effects of free phenolics extracted from tartary (Fagopyrum tartaricum L. Gaerth, Youyang 1#) buckwheat bran on the expression of PCNA (A), Cyclin D1 (B), CDK4 (C) and p21 (D) in human breast cancer MDA-MB-231 cells. Bars with no letters in common are significantly different (p < 0.05). | |
Similarly, Li et al. (2014) found that quercetin extracted from tartary buckwheat inducted G2/M arrest accompanied by an increase in the expression level of p21, decreasing the level of cyclin D1 in HepG2 cells.2 Studies also indicated that quercetin might mediate growth inhibition by perturbation in the G0/G1 phase of the cell cycle.66 The antiproliferation activity of rutin was through inducing the G2/M phase cell cycle arrest.67 Caffeic acid inhibited cell growth by means of prolonging the G0/G1phase and shortening G2/M phase.68 Resveratrol can produce arrests at both G0/G1 and G2/M depending on the context of cell type and dose.69
Therefore, it could be inferred that the free phenolics (caffeic acid, rutin, quercetin and resveratrol especially) extracted from the tartary buckwheat bran might mainly contribute to up-regulate the expression of p21, and therefore lead to down-regulating the downstream proteins expressions of cyclin D1, PCNA and CDK4, which might negatively regulate the progression of cell cycle resulting in an antiproliferative effect in MDA-MB-231 human breast cancer cells.
3.7 Free phenolics extract affects proliferation and apoptosis through the p38/MAP kinase pathway
Apoptosis or programmed cell death is a crucial process in keeping the balance between cell death and cell renewal.70 The p38 MAP kinase (MAPK) pathway plays an important role in the regulation of cell growth, differentiation, apoptosis and invasion.71,72 Studies indicated that the activation of apoptosis signal-regulating kinase 1 (ASK1) contributed to the expression of p38 MAPK in cell signaling pathways and therefore might induct cell apoptosis.73–75 p53, a well-known tumor suppressor protein, plays a key role in multiple central cellular processes, including transcription, DNA repair, genetic stability, senescence, cell cycle control and apoptosis.76–78 p53 protein with its unique C- and N-terminal structures is rigidly modulated by important biological processes such as phosphorylation, acetylation and ubiquitination, through which it effectively regulates cell growth or cell death. The phosphorylated p53 protein (p-p53) may be related to the loss or change of p53 binding activity to its downstream targets, and thus induce malignant cellular transformation.78 Phosphorylated p38 (p-p38) protein functions as a crucial mediator in cellular responses to extracellular stimuli and a high p-p38 expression was associated with poor survival in cancer cells.79 Tumor necrosis factor (TNF) and receptor-associated factor 2 (TRAF2) associate with and mediate the signal transduction from members of the TNF receptor superfamily.80 To confirm whether the free phenolics extract inhibited cell proliferation by inducing apoptosis through the p38 MAPK pathway, we investigated the expression level of ASK1, TRAF2, p-p38 and p-p53, which are related to antiproliferation and apoptosis in cancer cells.
As shown in Fig. 5, compared to the control, the free phenolics extract significantly (p < 0.05) down-regulated the expressions of p-p53 (Fig. 5A) and TRAF2 (Fig. 5D) in a dose-dependent manner. The expressions of p-p38 (Fig. 5B) and p-ASK1 (Fig. 5C) increased. Particularly, treatment of human breast cancer MDA-MB-231 cells with 3 mg mL−1 of free phenolics extract increased the expression of p-p38 and p-ASK1 by 134.0% and 250.8%, respectively (Fig. 5B and C).
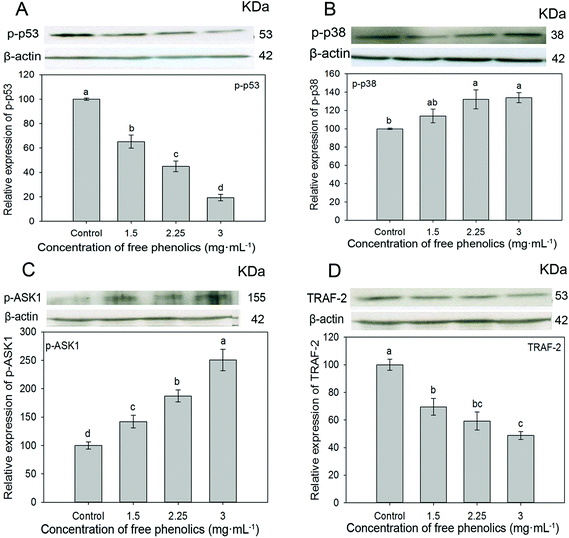 |
| Fig. 5 Effects of free phenolics extracted from tartary (Fagopyrum tartaricum L. Gaerth, Youyang 1#) buckwheat bran on the expression of p-p53 (A), p-p38 (B), p-ASK1 (C), and TRAF 2 (D) in human breast cancer MDA-MB-231 cells. Bars with no letters in common are significantly different (p < 0.05). | |
Phenolic compounds are considered to play an important role as anticarcinogenic agents due to their antioxidant properties, since free radicals, produced by cancer cells, could activate a number of genes and signal transduction pathways that mediate cancer cell proliferation, survival or apoptosis, and the amount of free radicals reduced by phenolic compounds thus decreasing cancer progression. In addition, direct interactions between phenolic compounds and multiple key elements in the signaling transduction pathways have been reported.81 Studies indicated that phenolic extracts of medicinal plants and individual phenolic compounds (rosmarinic acid, quercetin and luteolin) could act as modulators of the MAPK cancer signaling pathways.82 Quercetin could induce a significant time-dependent inactivation of the NF-κB pathway and a time-dependent activation of the AP-1/JNK pathway, as well as trigger apoptosis in HepG2 cells via the themitochondrial, Akt, and ERK pathway.82,83 Rosa et al., and Liao et al., indicated that phenolic acids (caffeic acid and its derives especially) and flavonoids (quercetin, catechins, etc.) were found to potentiate the antitumor effects by inducing apoptosis in MAP kinases pathways.3,52 A cautionary note is warranted, it may not be just one individual compound in the free phenolic extract that is producing the results we observed. Mixtures of polyphenols have been shown to have synergistic/enhanced effects on cellular processes compared with those when individual compounds are assessed.3
Accordingly, it is concluded that the free phenolics extract suppressed TRAF2 expression, activated expression of ASK1, then up-regulated the expression of p-p38 and down-regulated the expression of p-p53, which indicated that the free phenolics extract targeted the p38 MAPK pathway to inhibit cell proliferation and induced apoptosis in human breast cancer MDA-MB-231 cells. It has also been reported that JNK, another MAPK, may also be triggered by ASK1 activation and involved in the induction of cell apoptosis.75 Further research to determine the interactions of key proteins in cell signaling pathways are needed, since signaling networks are highly interconnected with cross-talks among several pathways.
4. Conclusion
The detected phenolic compounds in the tartary buckwheat bran included phenolic acids and flavonoids, which mainly existed in a free form with about two-fold higher content than the bound phenolics. Free phenolics extracted from the tartary buckwheat bran contributed the major antioxidant activity (ORAC and CAA), and also inhibited the proliferation of human breast cancer MDA-MB-231 cells in a dose-depended manner.
Western blotting analysis of cells treated with various the free extract indicated that the significant inhibition on the proliferation of MDA-MB-231 cells was through the p38 MAPK signaling pathway by regulating the cell cycle and/or inducting cell apoptosis. In the cell cycle pathway, the increased expression of p21 triggered the down-regulated expressions of cyclin D1, PCNA and CDK4 leading to cell cycle arrest from G1 to S phase and resulting in inhibition effect on cell proliferation. In the apoptosis pathway, the down-regulated TRAF2 and up-regulated p-ASK1 trigger the downstream kinase p38 pathway and thereby up-regulated expression of p-p38 and down-regulated expression of p-p53, which induced the apoptosis in human breast cancer MDA-MB-231 cells.
Our results suggested that the tartary buckwheat bran could be a rich natural source of phenolics, particularly for free phenolics with potent antioxidant activity and antiproliferative capacity. The significant inhibitory effect of the free phenolics on MDA-MB-231 cells proliferation was introduced by inducting cell apoptosis and/or cell cycle arresting through the p38 MAPK signaling pathway.
Conflict of interest
On behalf of all the authors, the corresponding author states that there is no conflict of interest.
Acknowledgements
This work was supported by Special National Key Research and Development Plan (2016YFD0400203-2; 2016YFD0400204-15) and the National Natural Science Foundation of China (31471576).
Notes and references
- Y. Yao, F. Shan and J. Bian, J. Agric. Food Chem., 2008, 56, 10027–10031 CrossRef CAS PubMed.
- Y. Li, S. Duan, H. Jia, C. Bai, L. Zhang and Z. Wang, Acta Biochim. Biophys. Sin., 2014, 46, 460–470 CrossRef CAS PubMed.
- R. H. Liu, J. Nutr., 2004, 134, 3479–3485 Search PubMed.
- R. Karki, C. H. Park and D. W. Kim, J. Integr. Med., 2013, 11, 246–252 CrossRef PubMed.
- M. Holasova, V. Fiedlerova, H. Smrcinova, M. Orsak, J. Lachman and S. Vavreinova, Food Res. Int., 2002, 35, 207–211 CrossRef CAS.
- I. Sedeja, M. Sakača, A. Mandića, A. Mišana, V. Tumbasb and M. Hadnađeva, J. Cereal Sci., 2011, 54, 347–353 CrossRef.
- M. Sakac, I. Sedej, A. Mandic and A. Misan, Hem. Ind., 2014, 62–62 Search PubMed.
- Y. Ma, X. Guo, H. Liu, B. Xu and M. Wang, Food Sci. Biotechnol., 2013, 22, 153–159 CrossRef CAS.
- H. Tsai, H. Deng, S. Tsai and Y. Hsu, Chem. Cent. J., 2012, 6, 1–5 CrossRef PubMed.
- L. Wang, X. Yang, P. Qin, F. Shan and G. Ren, Ind. Crops Prod., 2013, 49, 312–317 CrossRef CAS.
- A. Wang, J. Zhang and Z. Li, Food Res. Int., 2012, 49, 65–71 CrossRef CAS.
- K. L. Wolfe and R. H. Liu, J. Agric. Food Chem., 2007, 55, 8896–8907 CrossRef CAS PubMed.
- R. Peto, J. Natl. Cancer Inst., 1981, 66, 1191–1308 Search PubMed.
- R. H. Liu, Adv. Nutr., 2013, 4, 384S–392S CrossRef CAS PubMed.
- R. H. Liu and J. Finley, J. Agric. Food Chem., 2005, 53, 4311–4314 CrossRef CAS PubMed.
- R. H. Liu and J. Sun, J. Agric. Food Chem., 2003, 51, 1718–1723 CrossRef CAS PubMed.
- X. Guo, K. Zhu, H. Zhang and H. Yao, Int. J. Mol. Sci., 2010, 11, 5201–5211 CrossRef CAS PubMed.
- C. Zheng, C. Hu, X. Ma, C. Peng, H. Zhang and L. Qin, Food Chem., 2012, 132, 433–438 CrossRef CAS PubMed.
- K. K. Adom and R. H. Liu, J. Agric. Food Chem., 2002, 50, 6182–6187 CrossRef CAS PubMed.
- V. L. Singleton, R. Orthofer and R. M. Lamuela-Raventós, Methods Enzymol., 1998, 299, 152–178 Search PubMed.
- R. L. Prior, H. Hoang, L. Gu, X. Wu, M. Bacchiocca, L. Howard, M. Hampsch-Woodill, D. Huang, B. Ou and R. Jacob, J. Agric. Food Chem., 2003, 51, 3273–3279 CrossRef CAS PubMed.
- D. L. Felice, J. Sun and R. H. Liu, J. Funct. Foods, 2009, 1, 109–118 CrossRef.
- R. H. Liu, J. Jacob and B. Tennant, BioTechniques, 1997, 22, 594–595 CAS.
- X. D. Guo, C. S. Wu, Y. J. Ma, J. Parry, Y. Y. Xu, H. Liu and M. Wang, Food Res. Int., 2012, 49, 53–59 CrossRef CAS.
- X. D. Guo, Y. J. Ma, J. Parry, J. M. Gao, L. L. Yu and M. Wang, Molecules, 2011, 16, 9850–9867 CrossRef CAS PubMed.
- H. Ti, R. Zhang, M. Zhang, Z. Wei, J. Chi, Y. Deng and Y. Zhang, Food Chem., 2015, 178, 186–194 CrossRef CAS PubMed.
- D. Sumczynski, E. Kotásková, H. Družbíková and J. Mlček, Food Chem., 2016, 211, 339–346 CrossRef CAS PubMed.
- T. Wang, Y. Zhu, X. Sun, J. Raddatz, Z. Zhou and G. Chen, Food Chem., 2014, 152, 37–45 CrossRef CAS PubMed.
- S. Narwal, V. Thakur, S. Sheoran, S. Dahiya, S. Jaswal and R. K. Gupta, J. Plant Biochem. Biotechnol., 2014, 23, 11–17 CrossRef CAS.
- C. Kaur and H. C. Kapoor, Int. J. Food Sci. Technol., 2002, 37, 153–161 CrossRef CAS.
- N. Lenka, J. Zima, J. Barek and J. Dagmar, Food Chem., 2011, 126, 374–378 CrossRef.
- Y. B. Kim, A. A. Thwe, Y. Kim, S. K. Yeo, C. Lee and S. U. Park, Nat. Prod. Commun., 2013, 8, 1571–1574 CAS.
- Q. Ren, C. Wu, Y. Ren and J. Zhang, Food Chem., 2013, 136, 1377–1389 CrossRef CAS PubMed.
- R. Thierry and J. J. Macheix, J. Agric. Food Chem., 1996, 44, 1727–1730 CrossRef.
- J. M. Seo, D. B. Lee, M. V. Arasu, Q. Wu, T. Suzuki, Y. H. Yoon, S. W. Lee, S. U. Park and S. J. Kim, J. Agric. Chem. Environ., 2013, 2, 109–116 Search PubMed.
- G. E. Inglett, D. Chen, M. Berhow and S. Lee, Food Chem., 2011, 125, 923–929 CrossRef CAS.
- Z. Zhang, M. Zhou, Y. Tang, F. Li, Y. Tang, J. Shao, W. Xue and Y. Wu, Food Res. Int., 2012, 49, 389–395 CrossRef CAS.
- M. R. Rover and R. C. Brown, J. Anal. Appl. Pyrolysis, 2013, 104, 366–371 CrossRef CAS.
- P. V. Hung and N. Morita, Food Chem., 2008, 109, 325–331 CrossRef CAS PubMed.
- A. Kilci and D. Gocmen, Food Chem., 2014, 151, 547–553 CrossRef CAS PubMed.
- N. Okarter, C. S. Liu, M. E. Sorrells and R. H. Liu, Food Chem., 2010, 119, 249–257 CrossRef CAS.
- B. Min, A. M. Mcclung and M. H. Chen, J. Food Sci., 1900, 76, 117–126 CrossRef PubMed.
- K. L. Wolfe, X. Kang, X. He, M. Dong, Q. Zhang and R. H. Liu, J. Agric. Food Chem., 2008, 56, 8418–8426 CrossRef CAS PubMed.
- N. Okarter, Life Sci. Med. Res., 2012, 37, 1–11 Search PubMed.
- L. Wen, X. Guo, R. H. Liu, L. You, A. M. Abbasi and X. Fu, Food Chem., 2015, 186, 54–62 CrossRef CAS PubMed.
- C. F. Zhao, D. J. Lei, G. H. Song, H. Zhang, H. Xu and L. J. Yu, Food Chem., 2015, 169, 484–491 CrossRef CAS PubMed.
- F. M. F. Roleira, E. J. Tavares-Da-Silva, C. L. Varela, S. C. Costa, T. Silva, J. Garridob and F. Borgesc, Food Chem., 2015, 183, 235–258 CrossRef CAS PubMed.
- W. Ren, Z. Qiao, H. Wang, L. Zhu, L. Zhang, Y. Lu, Y. Cui, Z. Zhang and Z. Wang, Methods Find. Exp. Clin. Pharmacol., 2001, 23, 427–432 CrossRef CAS PubMed.
- A. A. Al-Hazzani and A. A. Alshatwi, Food Chem. Toxicol., 2011, 49, 3281–3286 CrossRef CAS PubMed.
- N. Horie, N. Hirabayashi, Y. Takahashi, Y. Miyauchi, H. Taguchi and K. Takeishi, Biol. Pharm. Bull., 2005, 28, 574–579 CAS.
- S. B. Lotito and B. Frei, Free Radicals Biol. Med., 2006, 41, 1727–1746 CrossRef CAS PubMed.
- L. S. Rosa, N. J. A. Silva, N. C. P. Soares, M. C. Monteiro and A. J. Teodoro, J. Nutr. Food Sci., 2016, 6, 468–474 Search PubMed.
- A. A. Alshatwi, J. Exp. Clin. Cancer Res., 2010, 29, 167–176 CrossRef CAS PubMed.
- R. H. Lee, J. H. Cho, Y. J. Jeon, W. Bang, J. J. Cho, N. J. Choi, K. S. Seo, J. H. Shim and J. I. Chae, Drug Dev. Res., 2015, 76, 9–16 CAS.
- B. Janicke, G. Önning and S. M. Oredsson, J. Agric. Food Chem., 2005, 53, 6658–6665 CrossRef CAS PubMed.
- D. Xiang, D. Wang, Y. He, J. Xie, Z. Zhong, Z. Li and J. Xie, Anti-Cancer Drugs, 2006, 17, 753–762 CrossRef CAS PubMed.
- S. M. Huang, C. L. Hsu, H. C. Chuang, P. H. Shih, C. H. Wu and G. C. Yen, Neurotoxicology, 2008, 29, 1016–1022 CrossRef CAS PubMed.
- J. Yang and R. H. Liu, J. Agric. Food Chem., 2009, 57, 8581–8586 CrossRef CAS PubMed.
- T. Eitsuka, N. Tatewaki, H. Nishida, T. Kurata, K. Nakagawa and T. Miyazawa, Biochem. Biophys. Res. Commun., 2014, 453, 606–611 CrossRef CAS PubMed.
- J. Sun and R. H. Liu, J. Agric. Food Chem., 2008, 56, 11661–11667 CrossRef CAS PubMed.
- L. Wang, D. Liu, T. Ahmed, F. L. Chung, C. Conaway and J. W. Chiao, Int. J. Oncol., 2004, 24, 187–192 Search PubMed.
- G. Maga and U. Hübscher, J. Cell Sci., 2003, 116, 3051–3060 CrossRef CAS PubMed.
- J. Kato, H. Matsushime, S. W. Hiebert, M. E. Ewen and C. J. Sherr, Genes Dev., 1993, 7, 331–342 CrossRef CAS PubMed.
- A. Ilundáin, Oncogene, 1998, 16, 1513–1523 Search PubMed.
- C. B. Thompson, Science, 1995, 267, 1456–1462 CrossRef CAS PubMed.
- M. Yoshida, M. Yamamoto and T. Nikaido, Cancer Res., 1992, 52, 6676–6681 CAS.
- H. Chen, Q. Miao, M. Geng, J. Liu, Y. Hu, L. Tian, J. Pan and Y. Yang, Sci. World J., 2013, 2013, 1–8 Search PubMed.
- L. D. Murad, N. C. Soares, C. Brand, M. C. Monteiro and A. J. Teodoro, Nutr. Cancer, 2015, 67, 532–542 CrossRef CAS PubMed.
- G. Notas, A. P. Nifli, M. Kampa, J. Vercauteren, E. Kouroumalis and E. Castanas, Biochim. Biophys. Acta, Gen. Subj., 2006, 1760, 1657–1666 CrossRef CAS PubMed.
- H. Y. Yong, M. S. Koh and A. Moon, Expert Opin. Invest. Drugs, 2009, 18, 1893–1905 CrossRef CAS PubMed.
- T. Zarubin and J. Han, Cell Res., 2005, 15, 11–18 CrossRef CAS PubMed.
- A. Matsuzawa, K. Saegusa, T. Noguchi, C. Sadamitsu, H. Nishitoh, S. Nagai, S. Koyasu, K. Matsumoto, K. Takeda and H. Ichijo, Nat. Immunol., 2005, 6, 587–592 CrossRef CAS PubMed.
- J. Pan, Q. Chang, X. Wang, Y. Son, Z. Zhang, G. Chen, J. Luo, Y. Bi, F. Chen and X. Shi, Chem. Res. Toxicol., 2010, 23, 568–577 CrossRef CAS PubMed.
- T. Zeng, L. Peng, H. Chao, H. Xi, B. Fu, Y. Wang, Z. Zhu and G. Wang, Biochem. Biophys. Res. Commun., 2015, 460, 530–536 CrossRef CAS PubMed.
- M. G. van Oijen and P. J. Slootwegvan, Clin. Cancer Res., 2000, 6, 2138–2145 CAS.
- C. J. Sherr and F. Mccormick, Cancer Cell, 2002, 2, 103–112 CrossRef CAS PubMed.
- J. H. Jeong, H. Nakajima, J. Magae, C. Furukawa, K. Taki, K. Otsuka, M. Tomita, I. S. Lee, C. H. Kim, H. W. Chang, K. S. Min, K. K. Park and Y. C. Chang, Int. J. Cancer, 2009, 124, 2797–2803 CrossRef CAS PubMed.
- L. Bai and W. G. Zhu, J. Cancer Mol., 2006, 2, 141–153 CAS.
- S. N. Wang, K. T. Lee, C. J. Tsai, Y. J. Chen and Y. T. Yeh, Eur. J. Clin. Invest., 2012, 42, 1295–1301 CrossRef CAS PubMed.
- C. H. Régnier, C. Tomasetto and M.-C. Rio, Atlas Genet. Cytogenet. Oncol. Haematol., 2000, 4, 461–465 Search PubMed.
- V. S. Neergheen, T. Bahorun, E. W. Taylor, L. S. Jen and O. I. Aruoma, Toxicology, 2010, 278, 229–241 CrossRef CAS PubMed.
- P. R. X. Cristina and P. W. Cristina, PharmaNutrition, 2016, 4, 112–122 CrossRef.
- C. Y. Liao, C. C. Lee, C. C. Tsai, C. W. Hsueh, C. C. Wang, I. H. Chen, M. K. Tsai, M. Y. Liu, A. T. Hsieh, K. J. Su, H. M. Wu, S. C. Huang, Y. C. Wang, C. Y. Wang, S. F. Huang, Y. C. Yeh, R. J. Ben, S. T. Chien, C. W. Hsu and W. H. Kuo, BioMed Res. Int., 2015, 2015, 1–26 Search PubMed.
Footnotes |
† Electronic supplementary information (ESI) available. See DOI: 10.1039/c6fo01230b |
‡ These authors contributed equally. |
|
This journal is © The Royal Society of Chemistry 2017 |