DOI:
10.1039/C6FO00938G
(Paper)
Food Funct., 2017,
8, 315-322
Aloe activated P-glycoprotein and CYP 3A: a study on the serum kinetics of aloe and its interaction with cyclosporine in rats
Received
23rd June 2016
, Accepted 30th November 2016
First published on 23rd December 2016
Abstract
Aloe, the leaf juice of Aloe vera, is a popular functional food worldwide. The major constituents of aloe are polyphenolic anthranoids such as aloin, aloe-emodin and rhein. Cyclosporine (CSP), an immunosuppressant with a narrow therapeutic window, is a probe substrate of P-glycoprotein (P-gp), an efflux pump, and CYP 3A4. This study first investigated the serum kinetics of aloe, then evaluated the modulation effects of aloe on P-gp and CYP 3A through an aloe–CSP interaction study in rats. The serum kinetic study showed that aloe-emodin glucuronides (G) and rhein sulfates/glucuronides (S/G) were major molecules in the bloodstream. The aloe–CSP interaction study showed that the systemic exposure to CSP was significantly decreased by either a single dose or multiple doses of aloe. The results of in vitro studies indicated that aloe activated P-gp and aloe metabolites activated CYP 3A4. In conclusion, aloe ingestion activated the functions of P-gp and CYP 3A in rats.
Introduction
Aloe, the leaf juice of Aloe barbadensis MILL. (Liliaceae), is a popular functional food worldwide. Aloe is also a traditional Chinese medicine used for the treatment of constipation, digestive and bowel disorders, ulcers and skin problems.1,2 The major constituents of aloe are polyphenolic anthranoids such as aloin, aloe-emodin and rhein (chemical structures shown in Fig. 1). Pharmacological studies have reported a variety of beneficial effects of aloe and its constituents, such as purgative,3 anti-inflammatory,4 antioxidation5 and antitumor effects.6 However, pharmacokinetic information on aloe is still lacking. This study investigated the serum kinetics of aloe after single-dose and repeated administration in rats.
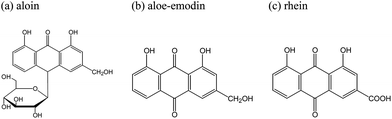 |
| Fig. 1 Chemical structures of anthranoids in aloe extract. | |
P-Glycoprotein (P-gp), an efflux transporter, and CYP 3A4 are recognized as playing pivotal roles in the bioavailability of various xenobiotics.7–9 Previous in vitro studies reported that aloe inhibited P-gp,10 CYP 3A4 and CYP 2D6.11 If these in vitro results could be extrapolated to in vivo results, aloe might increase the bioavailability of various medicines and toxins.8,12–14 However, until now no relevant in vivo evidence has been reported. Therefore, there is a hidden risk of aloe–drug interactions when aloe is taken as a functional food.
Cyclosporine (CSP), an immunosuppressant with a narrow therapeutic window, is a substrate of P-gp and CYP 3A4.15,16 This study measured the effect of aloe on the oral bioavailability of CSP, a probe substrate of P-gp and CYP 3A, in rats. Finally, the modulation effects of aloe on P-gp and CYP 3A4 were explored by using in vitro and ex vivo models.
Materials and methods
Chemicals and reagents
Aloin (purity 97%), aloe-emodin (purity 95%), rhein (purity 95%), β-glucuronidase (type B-1 from bovine liver, containing 1
240
000 units of β-glucuronidase) and sulfatase (type H-1 from Helix pomatia, containing 14
400 units of sulfatase and 574
000 units of β-glucuronidase) were purchased from Sigma-Aldrich Chemical Co. (Mo, USA). Cyclosporine (Neoral®) was kindly gifted by Novartis (Taiwan) Co. Ltd. 1,8-Dihydroxyanthraquinone (purity 98%) and berberine (98%) were obtained from Tokyo Pure Chemical Industries, Ltd (Tokyo, Japan). Wogonin (purity 98%) was supplied by Wako Pure Chemical Industries, Ltd (Osaka, Japan). Vivid® CYP450 screening kits were supplied by Invitrogen (NY, USA). The TDx kit was obtained from Abbott Laboratories (IL, USA).
Cell line and culture conditions
LS 180, the human colon adenocarcinoma cell line, was purchased from the Food Industry Research and Development Institute (Hsinchu, Taiwan). Cells were cultured at 37 °C with 5% CO2 and DMEM containing 10% FBS, 1% nonessential amino acid and 1% penicillin/streptomycin/glutamine.
Liquid chromatography
Two high performance liquid chromatography (HPLC) systems were used in this study. One system included an LC-2010C (Shimadzu, Japan) and a UV detector, which was used for the analysis of an aloe decoction. The other system used for the analysis of anthranoids in serum was composed of an LC-10AT (Shimadzu, Japan) and a fluorescence detector (RF-10AXL, Shimadzu, Japan). An autosampler (Series 200, Perkin Elmer, USA) and C18 column (4.6 × 250 mm, 5 μm) with a pre-filter (Isolation Technologies, USA) were used for both assay systems.
Preparation and characterization of aloe extract
Aloe was purchased from a herbal drugstore in Taichung, Taiwan. The plant material was identified by Dr Yu-Chi Hou. A voucher specimen (CMU-P-1905-12) was deposited in the Graduate Institute of Chinese Pharmaceutical Sciences, China Medical University. Water (8 L) was added to 400 g of aloe and heated on a gas stove. After boiling, gentle heating was continued until the volume was reduced to half volume and filtration was done while hot. The filtrate was gently boiled until the volume was below 800 mL, and sufficient water was added to make 800 mL. Each mL of extract represents 0.5 g of aloe.
Aloe extract (0.5 mL) was mixed with 9.5 mL of methanol and centrifuged to remove the precipitate. The appropriately diluted extract (100 μL) was mixed with an equal volume of wogonin solution (200 μg mL−1 in methanol) as the internal standard, and 20 μL was subjected to HPLC analysis. For calibrator preparation, various concentrations of standards were spiked with an equal volume of wogonin solution (200 μg mL−1 in methanol as internal standard). The mobile phase consisted of acetonitrile (A) and 0.1% phosphoric acid (B), and the elution gradient was programmed as follows: A/B: 20/80 (0–40 min), 38/62 (45–65 min), 20/80 (70–75 min). The flow rate was 1 mL min−1 and the detector was set at 230 nm.
Animals
Male Sprague-Dawley rats (340–440 g) were purchased from BioLASCO Taiwan Co., Ltd (Taipei, Taiwan). Rats were fasted for 12 h before drug administration. Water was supplied ad libitum. The animal study adhered to “The Guidebook for the Care and Use of Laboratory Animals (2002)” published by the Chinese Society for the Laboratory Animal Science, Taiwan, ROC. The experimental protocol was approved on 08/01/2015 (no. 104-339) by the Institutional Animal Care and Use Committee (IACUC) of China Medical University, Taiwan.
Drug administration and blood collection
In a pharmacokinetic study, twelve rats (n = 6 in each group) were orally administered with a single dose (2 g kg−1) and seven doses (2 g kg−1, thrice daily) of aloe extract via gastric gavage. Blood samples were withdrawn at 10, 30, 60, 120, 360, 720 and 1440 min after dosing.
In an aloe–CSP interaction study, rats were given CSP (Neoral®, 2.5 mg kg−1, n = 6) orally with and without a single dose (0.5 g kg−1, n = 5) or seven doses (0.25 g kg−1, twice daily, n = 5) of aloe extract in a parallel design. Blood samples were withdrawn at 20, 40, 60, 180, 300 and 540 min after CSP dosing and collected in a tube containing EDTA.
Quantitation of anthranoids and their metabolites in serum
The concentrations of aloin, aloe-emodin, rhein and their metabolites in serum were assayed by HPLC before and after hydrolysis with sulfatase and β-glucuronidase, respectively. For glucuronide (G) quantitation, serum (100 μL) was mixed with 50 μL of β-glucuronidase (1000 units per mL in pH 5 buffer) and 50 μL of L(+)-ascorbic acid (100 mg mL−1). After vortexing, the mixture was incubated at 37 °C for 30 min under anaerobic condition and protected from light. After hydrolysis, the serum was acidified with 0.1 N HCl (50 μL) and partitioned with 250 μL of ethyl acetate (containing 0.2 μg mL−1 of 1,8-dihydroxyanthraquinone as internal standard). After centrifugation, the ethyl acetate layer was dried by nitrogen gas and reconstituted with methanol (50 μL). Finally, 20 μL of sample was subjected to HPLC analysis.
For the quantitation of sulfate/glucuronides (S/G), serum (100 μL) was mixed with 50 μL of sulfatase (1000 units per mL containing 39
861 units per mL of β-glucuronidase in pH 5 buffer) and the mixture was incubated at 37 °C for 10 min. The latter procedure was identical to that described above for G. With regard to the assay of anthranoid free forms, the process was identical to that described above except for treatments with sulfatase or β-glucuronidase.
For calibrator preparation, serum (100 μL) was spiked with various concentrations of standards and mixed with 50 μL of pH 5 buffer. The latter procedure was identical to that described above for the serum sample. The mobile phase consisted of acetonitrile (A) and 0.1% phosphoric acid (B). The gradient elution was programmed as follows: A/B: 40/60 (0–25 min), 80/20 (30–35 min), 40/60 (40–45 min). The flow rate was 1 mL min−1 and the fluorescence detector was set at 440 nm for excitation and 520 nm for emission.
After hydrolysis, subtraction of the free form concentration from that liberated by β-glucuronidase could estimate the concentration of G. In addition, owing to considerable amounts of β-glucuronidase in the sulfatase (type H-1) used in this study, treatment with sulfatase led to the hydrolysis of both S and G. Accordingly, subtraction of the free form concentration from that released from sulfatase could estimate the concentration of S/G.
Validation of the analytical method of anthranoids in serum
The system suitability was evaluated through intra-run and inter-run assays of precision and accuracy. Recovery was estimated by comparing the peak area obtained from the extracted sample spiked with standards with that obtained from unextracted standards in the extracted sample matrix, which represents 100% recovery.
Quantitation of blood CSP concentration
Blood CSP concentration was analyzed by a specific monoclonal fluorescence polarization immunoassay (Abbott, IL, USA). All the processes, calibration curves and validation followed the manufacturer's protocol. The calibration range was 25.0–1500.0 ng mL−1 and LLOQ was 25.0 ng mL−1.
Effects of aloe and its anthranoids on P-gp-mediated transport
The transport study of rhodamine 123, a typical substrate of P-gp, was used to evaluate the effects of aloe, aloin, aloe-emodin and rhein on P-gp-mediated transport. The procedures essentially followed previous studies with some modifications.17–24 Briefly, after LS 180 cells were seeded onto 96-well plates overnight, the medium was removed. One hundred μL of rhodamine 123 (10.0 μM in HBSS) was added and incubated at 37 °C. After 1 h of incubation, the supernatants were removed and washed with cold PBS. Then, test agents including 6.3 and 25.0 μg mL−1 of aloe, 25.0 and 50.0 μM of aloin, 1.3 and 2.5 μM of aloe-emodin, 3.1 and 6.3 μM of rhein, 100.0 μM of verapamil (P-gp inhibitor)25 and 5.0 μM of berberine (P-gp activator)26 were added to the corresponding wells in triplicate and incubated at 37 °C. After 4 h of incubation, the cells were washed with cold PBS. Then 100 μL of 0.1% Triton X-100 was added to lyse the cells, and the fluorescence was assayed with excitation at 485 nm and emission at 528 nm. The relative intracellular accumulation of rhodamine 123 was calculated by comparison with that of the control after correction with the protein content.
Preparation and characterization of aloe serum metabolites (AM)
Because CYP 3A was located in the microsome of enterocytes and hepatocytes,27,28 in order to mimic the molecules interacting with CYP 3A after aloe intake, the AM of rats was prepared. Briefly, rats were repeatedly dosed with aloe extract for seven consecutive doses, and blood was collected at 1 h after the 7th dose. The serum obtained was vortexed with a 3-fold amount of methanol. After centrifugation, the supernatant was concentrated in a rotatory evaporator under vacuum to dryness, and then reconstituted with an appropriate amount of water to afford an AM solution equal to a 10 fold serum concentration. After proper dilution, the AM was quantitated prior to and after treatment with sulfatase/glucuronidase. The other procedures followed those described above in the quantitation of anthranoids and their metabolites in serum. On other hand, blank serum was collected from rats given water alone and processed similarly to prepare blank controls for comparison with AM.
Effects of AM, aloe-emodin and rhein on CYP 3A4 activity
Vivid® CYP450 screening kits were used to evaluate the influence of AM, aloe-emodin and rhein on the activity of CYP 3A4. All the procedures were performed according to standard protocols. Briefly, test agents including 1/4-, 1/2- and 1-fold serum concentrations of AM and blank serum specimens, 0.1 and 0.4 μM of aloe-emodin, 2.0 and 4.0 μM of rhein and ketoconazole (10.0 μM) as a positive control of the CYP 3A4 inhibitor were added to the corresponding wells in triplicate and incubated with glucose-6-phosphate, glucose-6-phosphate dehydrogenase and CYP450 recombinant BACULOSOMES® in 96-well black plates at room temperature for 20 min. In addition, a specific CYP 3A4 substrate (Vivid® BOMR) and NADP+ were added and incubated at room temperature for another 30 min. After the addition of a stop reagent (ketoconazole), the fluorescence was measured with excitation at 530 nm and emission at 590 nm.
Calculation of pharmacokinetic parameters and data analysis
The pharmacokinetic parameters were calculated using a noncompartment model with the aid of WinNonlin® (version 1.1, SCI software, Statistical Consulting, Inc., Apex, NC). Statistical analysis was performed using SPSS, version 17.0 (IL, USA). Unpaired student's t-test and ANOVA were used for statistical comparisons. Statistical significance level was set at p < 0.05.
Results
Characterization of aloe extract
Fig. 2 shows the HPLC chromatogram of aloin, aloe-emodin and rhein in aloe extract, which was satisfactorily resolved within 75 min by a gradient elution. The calibration curves of aloin (75.0–600.0 μg mL−1), aloe-emodin (0.6–10.0 μg mL−1) and rhein (1.9–30.0 μg mL−1) had good linearities. The coefficients of variation of intra-run and inter-run analysis were less than 8% and the relative errors were below 19%. The recoveries were 98–107%, 76–101% and 82–101% for aloin, aloe-emodin and rhein, respectively. The lower limits of quantitation (LLOQs) of aloin, aloe-emodin and rhein were 75.0, 0.6 and 1.9 μg mL−1, respectively. The limits of detection (LODs) of aloin, aloe-emodin and rhein were 0.3, 0.04 and 0.01 μg mL−1, respectively. The quantitation results indicated that the aloe extract contained 100.6, 1.0 and 0.3 mg mL−1 of aloin, aloe-emodin and rhein, respectively.
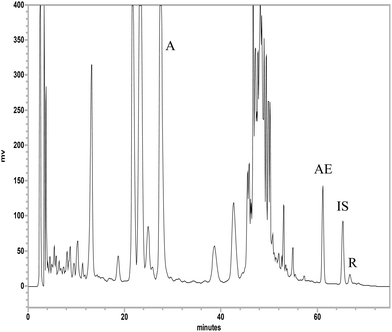 |
| Fig. 2 HPLC chromatogram of aloe extract. A: aloin, AE: aloe-emodin, R: rhein, IS: internal standard (wogonin). | |
Quantitation of aloe-emodin and rhein in serum
The calibration ranges of aloe-emodin (15.6–1000.0 ng mL−1) and rhein (62.5–2000.0 ng mL−1) in serum had good linearities. Validation of the method indicated that the coefficients of variation in intra-run and inter-run analysis were less than 10% and the relative errors were below 17%. The recoveries of aloe-emodin and rhein from serum were 80–113% and 69–111%, respectively. The LLOQs of aloe-emodin and rhein were 15.6 and 62.5 ng mL−1, and the LODs were 15.6 and 31.3 ng mL−1, respectively. All method validation meets the criteria of the Guidance on Bioanalytical Method Validation from FDA (September 2013).
Serum kinetics of anthranoids in rats after aloe ingestion
The quantitation of anthranoids in serum showed that aloe-emodin and rhein were present, whereas aloin was not detected. Fig. 3(a) and (b) depict the serum concentration–time profiles of aloe-emodin F and rhein F as well as their S/G and G after oral administration of the single dose and the 7th dose of aloe, respectively. The pharmacokinetic parameters are listed in Table 1. The results showed that either after the single dose or multiple doses, aloe-emodin mainly presented as aloe-emodin S/G in the blood, mainly G, and only a trace of aloe-emodin F was present. In contrast, rhein mainly existed as F after the single dose, whereas rhein S/G were the major forms after repeated dosing.
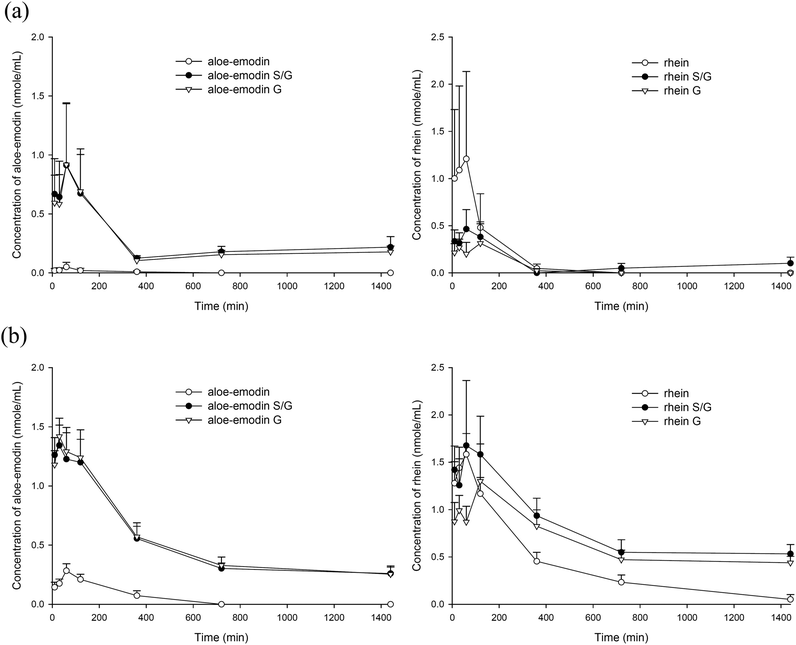 |
| Fig. 3 Mean (±S.E.) serum concentration–time profiles of the free form, sulfates/glucuronides (S/G) and glucuronides (G) of aloe-emodin and rhein in rats after oral administration of single dose (a) and 7th dose (b) of aloe (2 g kg−1). | |
Table 1 Pharmacokinetic parameters of the free forms (F), sulfate/glucuronides (S/G) and glucuronides (G) of aloe-emodin and rhein after oral administration of single dose and 7th dose of aloe (2 g kg−1) to rats
|
|
Single dose (n = 6) |
7th dose (n = 6) |
Parameters |
C
max
|
AUC0–1440 |
C
max
|
AUC0–1440 |
Data expressed as mean ± S.E. Cmax: maximum concentration (nmol mL−1). AUC0–1440: area under concentration–time curve to the last time (nmol min mL−1). *p < 0.05, **p < 0.01, compared with the single dose group. |
Aloe-emodin |
F |
0.05 ± 0.04 |
4.8 ± 3.3 |
0.3 ± 0.1** (500%) |
51.4 ± 16.8* (971%) |
S/G |
1.1 ± 0.5 |
374.1 ± 59.0 |
1.5 ± 0.2 |
711.3 ± 86.3** (91%) |
G |
1.0 ± 0.5 |
346.2 ± 75.6 |
1.6 ± 0.2 |
736.8 ± 98.6* (113%) |
|
Rhein |
F |
1.3 ± 0.9 |
160.8 ± 136.1 |
1.6 ± 0.2 |
501.4 ± 75.3 |
S/G |
0.6 ± 0.2 |
97.2 ± 17.5 |
2.4 ± 0.6* (300%) |
1134.2 ± 216.6** (1067%) |
G |
0.4 ± 0.2 |
48.6 ± 32.2 |
1.7 ± 0.2** (325%) |
931.4 ± 171.7** (1817%) |
Comparison between the two dosage regimens showed that the AUC0–1440 values of aloe-emodin S/G and aloe-emodin F after the 7th dose were significantly higher by 91% and 971%, respectively, than those after the single dose. In addition, the AUC0–1440 values of rhein S/G and rhein F after the 7th dose were higher by 1067% (p < 0.05) and 212% (p = 0.053), respectively, than those after the single dose.
Effect of aloe on the pharmacokinetics of CSP in rats
The blood concentration–time profiles of CSP with and without a single dose and 7th dose of aloe are shown in Fig. 4. The pharmacokinetic parameters of CSP after three treatments are listed in Table 2. The results showed that coadministration of the single dose and the 7th dose of aloe significantly lowered the Cmax of CSP by 86% and 81%, and decreased the AUC0–540 of CSP by 84% and 81%, respectively.
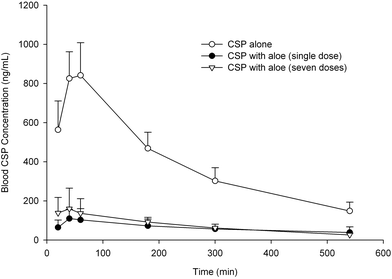 |
| Fig. 4 Mean (±S.E.) blood concentration–time profiles of CSP after oral administration of CSP alone (2.5 mg kg−1), and coadministration with single dose (0.5 g kg−1) and seven doses (0.25 g kg−1, twice daily) of aloe in rats. | |
Table 2 Pharmacokinetic parameters of CSP after oral administration of CSP alone (2.5 mg kg−1) and coadministration with aloe decoction in rats
Parameters |
CSP alone (n = 6) |
CSP + aloe (single dose) (n = 5) |
CSP + aloe (seven doses) (n = 5) |
Data expressed as mean ± S.E. Cmax: maximum concentration (ng mL−1). AUC0–540: area under concentration–time curve to the last time (μg min mL−1). MRT0–540: mean residence time (min). a,bSignificant difference at p < 0.05 between means are denoted by different letters. |
C
max
|
876.1 ± 55.0a |
118.8 ± 21.2b (−86%) |
168.6 ± 43.6b (−81%) |
AUC0–540 |
215.2 ± 15.8a |
34.2 ± 7.3b (−84%) |
40.7 ± 7.1b (−81%) |
MRT0–540 |
187.9 ± 4.7a |
219.6 ± 6.5b |
196.2 ± 10.4ab |
Effects of aloe and its anthranoids on the efflux transport mediated by P-gp
An MTT assay indicated that the concentrations of aloe extract, aloin, aloe-emodin and rhein used in this study showed no influence on the cell viability of LS 180 (data not shown). The intracellular accumulation of rhodamine 123, a typical P-gp substrate, in LS 180 cells determined after 4 h of incubation with tested agents is shown in Fig. 5. Verapamil (P-gp inhibitor) at 100.0 μM increased the intracellular accumulation of rhodamine 123 by 37%. Berberine (P-gp activator) at 5.0 μM decreased the intracellular accumulation of rhodamine 123 by 30%. Aloe (6.3 and 25.0 μg mL−1) significantly decreased the intracellular accumulation of rhodamine 123 by 24–25%. Aloin (25.0 and 50.0 μM) significantly reduced the intracellular accumulation of rhodamine 123 by 13–18%. Aloe-emodin (1.3 and 2.5 μM) significantly diminished the intracellular accumulation by 14–23%. Rhein (3.1 and 6.3 μM) significantly decreased the intracellular accumulation by 13–15%. These results indicated that aloe, aloin, aloe-emodin and rhein all activated the efflux transport mediated by P-gp.
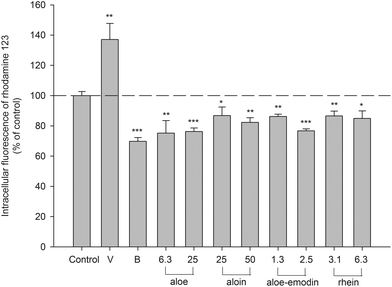 |
| Fig. 5 Effects of aloe (μg mL−1), aloin (μM), aloe-emodin (μM), rhein (μM), verapamil (V, 100.0 μM, P-gp inhibitor) and berberine (B, 5.0 μM, P-gp activator) on the accumulation of rhodamine 123 in LS 180 cells. *p < 0.05, **p < 0.01, ***p < 0.001. | |
Characterization of AM and effects of AM, aloe-emodin and rhein on CYP 3A4 activity
Based on the afore-mentioned pharmacokinetics of aloe, aloe-emodin G and rhein S/G were the major molecules in the systemic circulation. Therefore, in order to mimic the molecules interacting with CYP 3A4 in enterocytes and hepatocytes after aloe ingestion, AM was prepared and characterized. The results indicated that 1-fold AM contained 0.5 μM of aloe-emodin S/G, 1.0 μM of rhein S/G, 0.1 μM of aloe-emodin and 0.3 μM of rhein. The effects of AM, aloe-emodin and rhein on CYP 3A4 activity are shown in Fig. 6. AM at 1/2- and 1-fold serum concentrations significantly increased CYP 3A4 activity by 19% and 143%, respectively, when compared to those of the corresponding blank serum specimens. In contrast, 0.1 and 0.4 μM of aloe-emodin, and 2.0 and 4.0 μM of rhein all showed significant inhibition of CYP 3A4 activity by 10%, 21%, 17% and 34%, respectively. As a positive control of CYP 3A4 inhibitors, ketoconazole decreased the activity of CYP 3A4 by 16%.
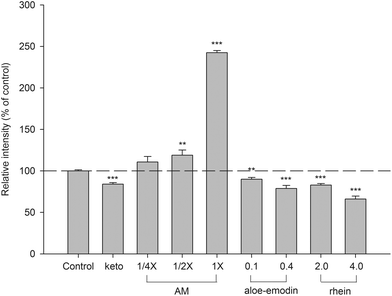 |
| Fig. 6 Effects of aloe serum metabolites (AM, 1/4-, 1/2- and 1-fold serum concentrations), aloe-emodin (μM), rhein (μM) and ketoconazole (keto, 10.0 μM, CYP 3A4 inhibitor) on CYP 3A4 activity. **p < 0.01, ***p < 0.001. | |
Discussion
Quantitation of the aloe extract showed that the major constituent was aloin, whereas aloe-emodin and rhein were minor extracts. However, aloin was not detected in serum, which might be accounted for by its poor lipophilicity to limit the passive diffusion across the cell membrane of enterocytes. A previous study has reported that aloin was metabolized to aloe-emodin 9-anthrone which was immediately oxidized to aloe-emodin.29 Subsequently, the benzylic alcohol group of aloe-emodin was feasibly oxidized to rhein.30,31 Then, both aloe-emodin and rhein were subjected to extensive conjugation metabolism to form S/G.
Owing to the unavailability of authentic standards, the conjugated metabolites of aloe-emodin and rhein in serum were quantitated indirectly through hydrolysis to their parent forms. Because the sulfatase (type H-1) used in this study contained a considerable amount of β-glucuronidase, treatment with sulfatase resulted in simultaneous hydrolysis of both S and G. Accordingly, the concentrations of aloe-emodin or rhein obtained after treatment with sulfatase represented a total of F plus S/G. The results showing that aloe-emodin was mainly present as G in the blood after either a single dose or multiple doses indicated that aloe-emodin was rapidly and extensively metabolized by glucuronidation. In contrast, rhein was mainly present as F after a single dose, which was consistent with a previous study.32 However, on repeated dosing with aloe, rhein S/G was the major form, which led us to speculate that glucuronidation or sulfation of rhein were probably induced after repeated dosing with aloe. The higher AUC0–1440 values of aloe-emodin S/G and rhein S/G after repeated dosing indicated that these conjugated metabolites accumulated considerably in the circulation after repeated ingestion of aloe.
Based on previous in vitro studies reporting that aloe inhibited P-gp and CYP 3A,10,11 this study in turn performed an in vivo functional assay of aloe in rats by using CSP as a probe substrate of P-gp and CYP 3A.15,16 The results showed that coadministration of a single dose and multiple doses of aloe both decreased the peak blood concentration and the systemic exposure to CSP, indicating that aloe decreased the oral bioavailability of CSP. Upon observing the blood profiles, we could infer that aloe markedly decreased the absorption of CSP, revealing that aloe ingestion did not exert significant inhibition of P-gp or CYP 3A in rats, which was not in agreement with the results of previous studies.10,11
In order to clarify the role of P-gp in the decreased absorption of CSP, the transport assay of rhodamine 123, a typical substrate of P-gp, was carried out. The results show that aloe extract decreased the intracellular accumulation of rhodamine 123 in LS 180, revealing that aloe activated the efflux transport mediated by P-gp. Likewise, aloin, aloe-emodin and rhein all decreased the intracellular accumulation of rhodamine 123, indicating these anthranoids all activated the efflux transport mediated by P-gp. Because P-gp is located on the apical membrane of enterocytes and is responsible for extruding substrates from enterocytes to the gut lumen,33 the activation of P-gp by aloe observed in the present study can in part explain the decreased absorption of CSP in rats. This result was contrary to a previous study reporting that aloe inhibited P-gp, in which an excised rat intestine model was used.10
On other hand, our serum kinetic study of aloe indicated that the conjugated metabolites of rhein and aloe-emodin were the major forms in the circulation, which led us to use AM to mimic the molecules interacting with CYP 3A localized in the microsome of enterocytes or hepatocytes. The result showed that AM activated CYP 3A4, which was contrary to a previous study reporting that CYP 3A4 was inhibited by aloe ethanol extract.11 Apparently, our study design of an ex vivo model demonstrating the activation of CYP 3A4 by aloe metabolites was more rational and could in part account for the decreased absorption of CSP. For the purpose of comparison, authentic compounds of aloe-emodin and rhein were evaluated in parallel although only traces of their free forms were detected in AM. Contrary to AM, aloe-emodin and rhein exhibited inhibiting effects on CYP 3A4 activity, which was in good agreement with a previous study,34 nevertheless, the inhibition of CYP 3A4 could not explain the decreased absorption of CSP. Therefore, these in vitro results indicated that aloe-emodin S/G and rhein S/G in AM potentially activated the activity of CYP 3A4, which had overwhelmed the inhibition effect by aloe-emodin and rhein. In recent years, we have discovered that polyphenol-rich foods including mulberry,21 soymilk and miso18 decreased the systemic exposure to CSP through activating CYP 3A4 by their metabolites. Together with the present study, these findings strongly suggest that understanding the serum kinetics of botanical foods was very important before bioactivity assays.
Taken together, ingestion of aloe activated both the P-gp-mediated efflux transport and CYP 3A-catalyzed metabolism of CSP, which interplayed to result in marked decrease of the oral bioavailability of CSP. Being an efflux transporter, P-gp has been recognized as playing crucial roles in the chemoprevention and detoxification of toxins and environmental carcinogens,14,35,36 such as polycyclic aromatic hydrocarbons which often present in grilled meat37,38 and tobacco smoke.13 On other hand, CYP 3A4 is a major enzyme involved in the metabolism of more than 50% of clinical drugs12 and other xenobiotics.39 Therefore, aloe ingestion might facilitate the extrusion and metabolism of a variety of drugs, toxins and environmental carcinogens. We thus recommend that aloe is a good functional food for chemoprevention and detoxification in healthy subjects. However, for patients taking substrate drugs of P-gp or CYP 3A4 such as paclitaxel, doxorubicin and tacrolimus, concomitant ingestion of aloe should be avoided to prevent probable pharmacokinetic interactions, which might result in decreased oral bioavailability of medicines.
Conclusions
Aloe ingestion markedly decreased the oral bioavailability of CSP, a substrate of P-gp and CYP 3A, in rats. The mechanism studies on this drug interaction revealed that aloe extract activated P-gp, and aloe metabolites activated CYP 3A. Therefore, aloe exhibited the functions of activating both P-gp and CYP 3A.
Abbreviations
AUC | The area under the curve of concentration |
C
max
| The peak plasma concentration |
MRT | The mean residence time |
P-gp | P-Glycoprotein |
CYP | Cytochrome P450 |
S | Sulfates |
G | Glucuronides |
S/G | Sulfates/glucuronides |
F | Free form |
AM | Aloe serum metabolites |
Conflict of interest
There are no conflicts of interest to declare.
Acknowledgements
This work was supported in part by the Ministry of Science and Technology, ROC (MOST 105-2314-B-039-018), China Medical University, ROC (CMU 104-S-31) and China Medical University Hospital, ROC (DMR-104-095, DMR-105-100, DMR-105-101).
Notes and references
- W. Y. Chen, B. E. Van Wyk, I. Vermaak and A. M. Viljoen, Phytochem. Lett., 2012, 5, 1–12 CrossRef CAS.
- M. H. Radha and N. P. Laxmipriya, J. Tradit. Complement. Med., 2015, 5, 21–26 CrossRef PubMed.
- T. Yagi and K. Yamauchi, J. Pharm. Pharmacol., 1999, 51, 93–95 CAS.
- R. F. Chen, Y. C. Shen, H. S. Huang, J. F. Liao, L. K. Ho, Y. C. Chou, W. Y. Wang and C. F. Chen, J. Pharm. Pharmacol., 2004, 56, 915–919 CrossRef CAS PubMed.
- L. O. Demirezer, A. Kuruuzum-Uz, I. Bergere, H. J. Schiewe and A. Zeeck, Phytochemistry, 2001, 58, 1213–1217 CrossRef CAS PubMed.
- J. M. Guo, B. X. Xiao, Q. Liu, S. Zhang, D. H. Liu and Z. H. Gong, Acta Pharmacol. Sin., 2007, 28, 1991–1995 CrossRef CAS PubMed.
- U. Christians, V. Schmitz and M. Haschke, Expert Opin. Drug Metab. Toxicol., 2005, 1, 641–654 CrossRef CAS PubMed.
- J. Sun, Z. G. He, G. Cheng, S. J. Wang, X. H. Hao and M. J. Zou, Med. Sci. Monit., 2004, 10, RA5–RA14 CAS.
- D. Pal and A. K. Mitra, Life Sci., 2006, 78, 2131–2145 CrossRef CAS PubMed.
- B. Carien, V. Alvaro and H. Josias, Pharmacogn. Mag., 2013, 9, S44–S48 CrossRef PubMed.
- A. Djuv and O. G. Nilsen, Phytother. Res., 2012, 26, 445–451 CAS.
- S. F. Zhou, Curr. Drug Metab., 2008, 9, 310–322 CrossRef CAS PubMed.
- W. C. Pan, R. M. Chen, Y. C. Shen, C. C. Chen and Y. F. Ueng, Toxicol. Lett., 2009, 185, 116–123 CrossRef CAS PubMed.
- B. Adamson, T. Pham, J. Kronenfeld, B. Reilly, M. Crowther, S. Fraijo, E. Cherrington, L. Futch and N. Cherrington, FASEB J., 2014, 28, LB109 Search PubMed.
- K. Yokogawa, T. Shimada, Y. Higashi, Y. Itoh, T. Masue, J. Ishizaki, M. Asahi and K. Miyamoto, Biochem. Pharmacol., 2002, 63, 777–783 CrossRef CAS PubMed.
- K. B. Goralski, P. D. Acott, A. D. Fraser, D. Worth and C. J. Sinal, Drug Metab. Dispos., 2006, 34, 288–295 CrossRef CAS PubMed.
- C. P. Yu, H. J. Lin, S. P. Lin, C. S. Shia, P. H. Chang, Y. C. Hou and Y. W. Hsieh, Xenobiotica, 2016, 46, 677–682 CrossRef CAS PubMed.
- C. P. Yu, Y. W. Hsieh, S. P. Lin, Y. C. Chi, P. Hariharan, P. D. Chao and Y. C. Hou, Food Chem., 2014, 149, 25–30 CrossRef CAS PubMed.
- Y. H. Peng, S. P. Lin, C. P. Yu, S. Y. Tsai, M. Y. Chen, Y. C. Hou and P. D. Chao, Planta Med., 2014, 80, 1291–1297 CrossRef CAS PubMed.
- Y. W. Hsieh, C. Y. Huang, S. Y. Yang, Y. H. Peng, C. P. Yu, P. D. Chao and Y. C. Hou, Sci. Rep., 2014, 4, 6587 CrossRef CAS PubMed.
- P. W. Hsu, C. S. Shia, S. P. Lin, P. D. Chao, S. H. Juang and Y. C. Hou, J. Agric. Food Chem., 2013, 61, 4464–4469 CrossRef CAS PubMed.
- S. Y. Yang, S. Y. Tsai, Y. C. Hou and P. D. L. Chao, Food Chem., 2012, 133, 683–688 CrossRef CAS.
- Y. C. Hou, S. P. Lin and P. D. Chao, Food Chem., 2012, 135, 2307–2312 CrossRef CAS PubMed.
- C. P. Yu, P. P. Wu, Y. C. Hou, S. P. Lin, S. Y. Tsai, C. T. Chen and P. D. Chao, J. Agric. Food Chem., 2011, 59, 4644–4648 CrossRef CAS PubMed.
- D. Lautier, Y. Canitrot and J. M. Salmon, Anticancer Res., 1994, 14, 2589–2595 CAS.
- I. A. Najar, B. S. Sachin, S. C. Sharma, N. K. Satti, K. A. Suri and R. K. Johri, Phytother. Res., 2010, 24, 454–458 CrossRef CAS PubMed.
- R. L. Slaughter and D. J. Edwards, Ann. Pharmacother., 1995, 29, 619–624 CAS.
- G. R. Wilkinson, N. Engl. J. Med., 2005, 352, 2211–2221 CrossRef CAS PubMed.
- Y. Ishii, H. Tanizawa and Y. Takino, Yakugaku Zasshi, 1988, 108, 904–910 CAS.
- M. Y. Park, H. J. Kwon and M. K. Sung, Biosci., Biotechnol., Biochem., 2009, 73, 828–832 CrossRef CAS PubMed.
- R. Song, H. Lin, Z. J. Zhang, Z. Li, L. Xu, H. J. Dong and Y. Tian, Rapid Commun. Mass Spectrom., 2009, 23, 537–547 CrossRef CAS PubMed.
- C. S. Shia, S. Y. Tsai, J. C. Lin, M. L. Li, M. H. Ko, P. D. Chao, Y. C. Huang and Y. C. Hou, J. Ethnopharmacol., 2011, 137, 1388–1394 CrossRef CAS PubMed.
- F. Staud, M. Ceckova, S. Micuda and P. Pavek, Methods Mol. Biol., 2010, 596, 199–222 CAS.
- J. C. Tang, H. Yang, X. Y. Song, X. H. Song, S. L. Yan, J. Q. Shao, T. L. Zhang and J. N. Zhang, Phytother. Res., 2009, 23, 159–164 CrossRef CAS PubMed.
- V. R. Tandon, B. Kapoor, G. Bano, S. Gupta, Z. Gillani and D. Kour, Indian J. Pharmacol., 2006, 38, 13–24 CrossRef CAS.
- T. Terada and D. Hira, J. Gastroenterol., 2015, 50, 508–519 CrossRef CAS PubMed.
- G. C. Yeh, J. Lopaczynska, C. M. Poore and J. M. Phang, Cancer Res., 1992, 52, 6692–6695 CAS.
- J. A. Albertus and R. O. Laine, J. Exp. Biol., 2001, 204, 217–227 CAS.
- L. Basheer and Z. Kerem, Oxid. Med. Cell. Longevity, 2015, 2015, 854015 Search PubMed.
|
This journal is © The Royal Society of Chemistry 2017 |