Mesoporous lignite-coke as an effective adsorbent for coal gasification wastewater treatment
Received
26th September 2016
, Accepted 16th November 2016
First published on 17th November 2016
Abstract
This paper introduces a novel adsorbent lignite coke (LC), which is suitable for macromolecular contaminant removal in coal gasification wastewater. Adsorption results show that LC had higher decolorization ability and removed more refractory compounds than activated carbons (ACs), and can improve wastewater biodegradability. Pore size distribution and electron microscopy results show that LC had more mesopores than ACs, which supplied enough area for high molecular weight pollutant removal. Equilibrium adsorption isotherms show that LC had high affinity with pollutants in coal gasification wastewater.
Water impact
Refractory compounds are key factors that impede industrial wastewater treatment and reuse. Here we developed a low cost mesoporous lignite-coke adsorbent. The results showed that the lignite-coke can adsorb more high molecular weight, refractory compounds compared to traditional expensive activated carbons. This work offers a new promising method to improve refractory wastewater treatment.
|
1. Introduction
Shortages of petroleum and natural gas in China have stimulated the development of liquefaction and gasification processes which convert coal into liquid and gaseous products. Coal gasification entailing the reaction of coal with steam and air or oxygen to yield a synthetic gaseous fuel is one of the best methods for acquiring clean coal energy to meet the rising demands in China. However, a coal gasification plant consumes a large amount of water during its manufacturing processes. Coal gasification wastewater (CGWW) is discharged mainly from the gas washing and condensing operations of the coal gasifier. Considering its complex composition and the toxic pollutants contained in it, coal gasification wastewater is regarded as a serious problem in the world. Although raw coal gasification wastewater is pretreated through solvent extraction and steam stripping to remove phenols and ammonia, the effluent especially from the fixed bed gasifier still contains high concentrations of refractory and inhibitory compounds.1
Biological technologies, such as conventional activated sludge,2,3 anoxic–oxic,4 and anaerobic–anoxic–oxic,5–9 are usually used to treat coal gasification wastewater, which provide cost effective and efficient treatments for most of the organic pollutants. However, it is inefficient for refractory organics removal and unstable when the influent wastewater quality fluctuates. Reducing the effluent chemical oxygen demand (COD) to less than 200 mg L−1 remains difficult, which severely restricts the wastewater reuse.10 The key point is that the wastewater contains high amounts of organic toxic components which may be harmful to biological systems, making high performance pretreatment processes more important.
In recent years, numerous attempts have been made to improve the biodegradability of coal gasification wastewater before biological processes. Many methods, such as ozonation,11 acid demulsion,12 catalyzed iron internal electrolysis,13 and manganese oxide oxidation,14 have been investigated and were able to improve the biochemical oxygen demand (BOD) of coal gasification wastewater. However, these methods are either economically unfavorable or technically complicated, which makes them difficult to be used in practice. The adsorption technique is widely used for wastewater, but the actual application of this process is restricted by the high cost of adsorbents, e.g., activated carbon (AC). Besides, ACs with higher micropore volumes exhibit higher adsorption affinities to low molecular weight (MW) organic matter, but are expected to be less efficient in adsorption of large size pollutants in wastewater.15
Adsorption technology using commercial activated carbons is proven to have high cost and poor performance in the treatment of coal gasification wastewater. Lignite with a porous structure and high reactivity holds promise as a precursor for synthesizing low cost adsorbents. At present, lignite costs less than $20 per ton in China, whereas the precursors bituminous coal and anthracite cost more than $100 per ton.16 Previous research, using lignite as a precursor mainly in drinking water or synthetic solutions, limited study focuses on the adsorption of real industrial wastewater, especially with high concentrations of refractory pollutants.17–20
In this paper, a new adsorbent lignite-coke (LC) was developed, and the adsorption properties of LC were investigated and compared with those of commercial ACs in the treatment of coal gasification wastewater. The adsorption mechanism and adsorption thermodynamics of LC were studied. Meanwhile, the industrial manufacturing cost was analyzed to shed light on this potentially promising method for the removal of refractory wastewater from coal gasification.
2. Experimental
2.1. Coal gasification wastewater
The coal gasification wastewater was obtained from the Yima coal gasification wastewater treatment plant. Before discharging to the wastewater treatment plant, physico-chemical pretreatments were employed to recover phenols and ammonia by the Lurgi gasification process. The qualities of the wastewater used in this study were as follows: CODCr: 2860 mg L−1, BOD5: 941 mg L−1, volatile phenol: 331 mg L−1, NH3–N: 232 mg L−1, oil: 95 mg L−1, and color: 3000 times. Predominant pollutants in coal gasification wastewater include phenolic compounds, heterocyclic compounds, polycyclic aromatic hydrocarbons, long chain alkenes, amine compounds, ammonia, sulfide, thiocyanate, and cyanide.
2.2. Adsorbents
Lignite from Inner Mongolia, China was used as a precursor in this study. A two-stage activation procedure (pyrolysis under nitrogen flow, followed by activation by steam) was established for the production of LC. A bench scale rotary kiln system (HB-IN2060, Zhilan) equipped with the appropriate gas was used for the production of samples. The furnace can be operated under various conditions of temperature and reacting gases, to prepare samples with varying characteristics aiming at the determination of the optimum activation conditions and preparation of samples with the best adsorption properties.
Two other commercially available activated carbons (AC1 and AC2) with high surface areas were selected for comparison with LC. Table 1 summarizes the major properties of these three adsorbents. In order to minimize the effect of the size distribution, all adsorbents were screened with a sieve of 8 × 30 mesh.
Table 1 Physical properties of the adsorbents
Adsorbent |
Manufacturer |
Raw material |
BET surface area (m2 g−1) |
LC |
Guodian futong |
Lignite |
448 |
AC1 |
Datong longshan |
Bituminous coal |
835 |
AC2 |
Shenhua ningmei |
Anthracite |
951 |
2.3. Adsorption experiments
Adsorption experiments were conducted using the method static adsorption. Different kinds of adsorbents were added to 150 mL of wastewater in a 250 mL iodine flask in a stable temperature horizontal shaking shaker (SKY-100C, Sukun). After reaching the adsorption equilibrium, the water quality (CODCr, BOD5, toxicity, etc.) was determined.
2.4. Analysis methods
The characteristics of the wastewater such as CODCr, BOD5, NH3–N, oil, toxicity, and volatile phenols were analyzed according to Water and Wastewater Monitoring and Analysis Methods (4th edition).21 For the molecular size distribution of organic matters, batch filtration experiments using a stirred pressure cell (Model 8400, Amicon Corp., USA) were carried out.22 The BET surface area and pore size distribution of all the adsorbents were measured using a nitrogen adsorption and desorption test at 77 K (Autosorb-6, Quanta Chrome). The nitrogen adsorption data were analyzed using the Barrett–Joyner–Halenda (BJH) model, based on the Kelvin equation and corrected for multilayer adsorption. The surface morphology images of the adsorbents were obtained using a Hitachi S-5500 scanning electron microscope (SEM).
3. Results and discussion
3.1. Adsorption selectivity
Fig. 1 and 2 present the performance of the different adsorbents during the static adsorption of coal gasification wastewater. The adsorbent dosage is 4.5 g per 150 mL. Fig. 1 shows the change of COD, BOD/COD ratio and toxicity by LC and AC adsorption, respectively. It is shown in Fig. 2 that the decolorization of LC was better than those of the other two ACs. This result is in accordance with the removal performance for pollutants larger than 500 Da. It is because pollutants larger than 500 Da mainly contributed to the color of the coal gasification wastewater.23 It is also shown in Fig. 1 that after adsorption by LC, the BOD/COD ratio (biodegradability) of wastewater increased to 0.55, indicating that more refractory organics in wastewater were removed. However, the BOD/COD ratio of wastewater decreased to below 0.10 after adsorption by the two ACs, showing that AC would first remove BOD during the adsorption of coal gasification wastewater. It can also be seen from Fig. 1 that the toxicity was reduced significantly after the adsorption by LC, while the other two ACs had a limited effect on the decrease of toxicity. Overall, it shows that LC adsorption can remove more large molecular organic pollutants which are toxic and refractory constituents in coal gasification wastewater, and LC is more suitable to be used in coal gasification wastewater treatment than ACs, especially as pretreatment of biological processes, for its selective adsorption.
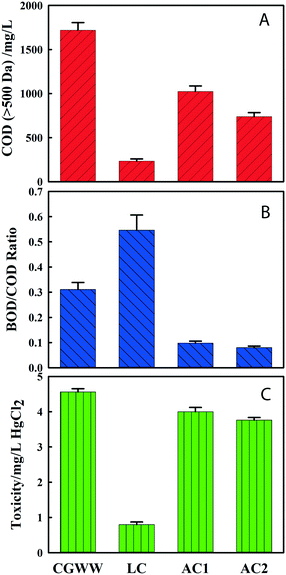 |
| Fig. 1 Variations before and after adsorption of coal gasification wastewater by the three adsorbents (A: COD (>500 Da); B: biodegradability; C: toxicity). | |
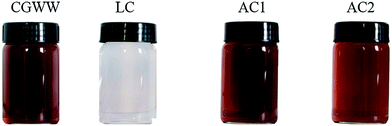 |
| Fig. 2 Color variations before and after adsorption of coal gasification wastewater by the three adsorbents. | |
3.2. Pore distribution analysis
The nitrogen adsorption–desorption isotherms of the three adsorbents are shown in Fig. 3. It can be seen in Fig. 3 that all the isotherms were typical type IV, according to International Union of Pure and Applied Chemistry (IUPAC), which implies that all the three adsorbents were amorphous carbon with a random pore distribution and interconnected pore system. From the shape and hysteresis of the isotherms, it is clear that LC showed a more extensive mesoporous structure than AC1 and AC2. The hysteresis loops of LC at higher P/P0 are associated with capillary condensation in mesoporous structures. These results are similar to a previous activated carbon study using lignite as the raw material.24,25
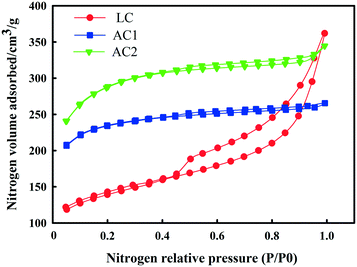 |
| Fig. 3 Nitrogen adsorption–desorption isotherms of the three adsorbents. | |
The pore size distributions of the three adsorbents were obtained by applying the BJH method and are presented in Fig. 4. According to the IUPAC definition, the pore size range of the mesopores is 2–50 nm. It is shown in Fig. 4 that LC had significantly higher mesoporosity than ACs, and had the tallest peak at the pore radius between 2 and 10 nm. The random pore size distributions and interconnected pore systems of LC can be observed from SEM images at magnification of 200k and 500k (Fig. 5).
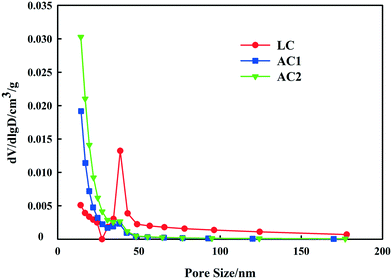 |
| Fig. 4 Pore size distribution of the three adsorbents. | |
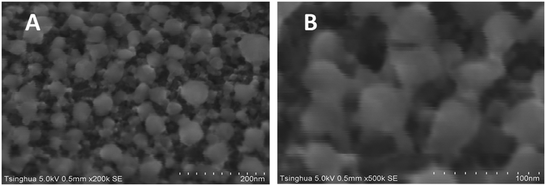 |
| Fig. 5 SEM image of LC (A: 200k; B: 500k). | |
Considering the steric effect, adsorbents are mostly well adsorbed on the surface of the pores with diameters 1.7–6 times their respective molecular diameter (MD, nm).25,26 The MD of many organic compounds may be estimated from their MW (Da) by eqn (1).
|  | (1) |
For the main pollutant in coal gasification wastewater (MW > 500 Da), the minimum pore sizes for effective adsorption are very close to 2 nm, and mesopores (2–50 nm) might be mainly responsible for the removal of large MW pollutants in coal gasification wastewater. The pore size distribution results indicated that the high mesoporosity of LC supplied enough area for adsorption of large MW organic pollutants, which were refractory for biological degradation. Micropores mainly contributed to the large surface area of ACs, and shortage in mesopores resulted in the poor performances of ACs for the removal of color and toxic organics.
3.3. Equilibrium isotherms
Equilibrium adsorption equations are necessary for the design of an adsorption system and the subsequent optimization. Langmuir and Freundlich isotherms were mostly used and analyzed in order to establish an appropriate correlation for the equilibrium isotherm curve in this study.
The Langmuir model describes reversible adsorption processes of a gas on solid surfaces, and it can also be applied to adsorption from solution of substances on insoluble adsorbents. The theoretical Langmuir isotherm is described in eqn (2)
|  | (2) |
where
q is the equilibrium adsorption capacity (mg g
−1),
Ce is the equilibrium liquid phase concentration (mg L
−1),
qm is the maximum adsorption capacity (mg g
−1), and
Ka is the adsorption equilibrium constant (L mg
−1).
The Freundlich isotherm is an empirical equation employed to describe heterogeneous systems, and is the earliest known relationship describing the adsorption isotherm. An ordinary adsorption isotherm is expressed by eqn (3)
where
KF and 1/
n are empirical constants (
Fig. 6 and
Table 2).
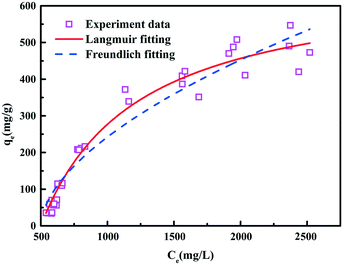 |
| Fig. 6 Equilibrium adsorption isotherms of coal gasification wastewater by LC (experimental data points are given by symbols, and line and dashes were predicted by the Langmuir and Freundlich isotherm models, respectively). | |
Table 2 Isotherm parameters for the treatment of coal gasification wastewater by LC
|
Langmuir |
q
m
|
K
a
|
(mg g−1) |
(L mg−1) |
LC |
676 |
0.001 |
|
|
Freundlich |
1/n |
K
F
|
|
(mg g−1)(L mg−1)1/n |
LC |
0.57 |
7.04 |
The value of 1/n obtained from the Freundlich equation indicates the affinity between the adsorbate and adsorbent, and the heterogeneity of the adsorbent sites. 1/n > 2 means adsorbents are difficult to be adsorbed, while 1/n < 0.5 usually shows high affinity between the adsorbate and adsorbent. The Freundlich isotherms indicated that COD in coal gasification wastewater was easy to be adsorbed by LC. The maximum amount adsorbed by LC, which was obtained from the Langmuir isotherm, was 676 mg g−1.
3.4. Cost analysis for industrial production of lignite coke
For industrial production of LC with a capacity of 10
000 tons per year, an operational cost analysis was conducted here. Referring to Table 3, it is shown that the estimated direct cost involved in the production of LC is 195 US$ per ton. If the indirect cost including the capital expense and the return of investment was considered, the total cost should be around 450 US$ per ton. By contrast, according to our research of the international trading data, the price of commercial AC for water and wastewater treatment varies between US$ 1000–1500 per ton depending on the grade of AC.
Table 3 Operational cost analysis for industrial production of LC
Component |
Cost ($ per ton) |
Raw material |
68 |
Transportation |
3 |
Power |
20 |
Steam |
60 |
Labor |
16 |
Package |
10 |
Environmental protection |
13 |
Others |
6 |
Total |
195 |
It should be pointed out that the prime components that need to be considered in the production of LC are the raw material and steam consumption. For the raw material, lignite from Ximeng coal mine in Inner Mongolia's Xilingol League north of China, which is one of the largest lignite deposits in China, was selected in this study and for further industrial production. In this region, more than 50% of the total coal reserve is suitable for opencast coal mining. Steam gasification is an endothermic process. At the industrial scale, the external heating process for maintaining the activation temperatures will be very costly for production. So direct fire-heated systems with both steam and air fed into the furnace were designed. The power consumption contributed only 10% of the operational cost as a highly efficient vertical furnace configuration was used.
4. Conclusion
Adsorptions of coal gasification wastewater by LC and ACs were conducted in batch adsorption systems. The results show that LC could remove toxicity and more refractory constituents than ACs, which makes LC more suitable to be used in the adsorption of coal gasification wastewater.
The pore size distributions of LC and ACs were also investigated, and the results indicated that the high mesoporosity of LC supplied enough area for adsorption of large MW organic pollutants, which were refractory for biological degradation. However, micropores mainly contributed to the large surface area of ACs, and shortage in mesopores resulted in the poor performances of ACs for the removal of color and toxic organics.
The results of the equilibrium adsorption isotherms of coal gasification wastewater by LC show that COD in coal gasification wastewater was easy to be adsorbed by LC. The maximum amount adsorbed by LC was 676 mg g−1.
Acknowledgements
This work was funded by the MOST of China, 863 project (2009AA050904, 2012AA063506). The authors also acknowledge the support of Prof. Xia Huang, Dr. Fang Zhang and the State Key Laboratory of Environmental Simulation and Pollution Control in Tsinghua University.
Notes and references
- W. Wang, H. J. Han, M. Yuan, H. Q. Li, F. Fang and K. Wang, Bioresour. Technol., 2011, 102, 5454–5460 CrossRef CAS PubMed.
- R. G. Luthy and J. T. Tallon, Water Res., 1980, 14, 1269–1282 CrossRef CAS.
- W. Q. Zhang, P. H. Rao, H. Zhang and J. L. Xu, Chin. J. Chem. Eng., 2009, 17, 167–170 CrossRef CAS.
- J. X. Liu, B. Z. Wang, W. G. Li, C. J. Jin and X. D. Cao, Water Sci. Technol., 1996, 34, 17–24 CAS.
- Y. S. Jeong and J. S. Chung, Process Biochem., 2006, 41, 1141–1147 CrossRef CAS.
- Y. Jeong, B. G. Park and J. S. Chung, Water Sci. Technol., 2005, 52, 325–334 CAS.
- Z. X. Wang, X. C. Xu, Z. Gong and F. L. Yang, J. Hazard. Mater., 2012, 235–236, 78–84 CrossRef CAS PubMed.
- G. F. Nakhla and M. T. Suidan, J. Hazard. Mater., 1995, 42, 71–86 CrossRef CAS.
- W. Wang, W. C. Ma, H. J. Han, H. Q. Li and M. Yuan, Bioresour. Technol., 2011, 102, 2441–2447 CrossRef CAS PubMed.
- J. L. Wang, X. C. Quan, L. B. Wu, Y. Qian and W. Hegemann, Process Biochem., 2002, 38, 777–781 CrossRef CAS.
- C. Han, J. X. Ye and D. Z. Sun, Res. J. Environ. Sci., 2012, 23, 970–974 Search PubMed.
- W. Q. Zhang, J. Ma, S. D. Yang, T. Zhang and Y. F. Li, Chin. J. Chem. Eng., 2006, 14, 398–401 CrossRef CAS.
- K. Xie, L. Wang, X. Wang, Y. X. Luan, C. Jia and A. Q. Huang, Huanjing Wuran Yu Fangzhi, 2010, 32, 28–31 CAS.
- C. Z. Fan, A. H. Lu, Y. Li and C. Q. Wang, Chem. Eng. J., 2010, 160, 20–26 CrossRef CAS.
- S. J. Zhang, T. Shao, H. S. Kose and T. Karanfil, Environ. Sci. Technol., 2010, 44, 6377–6383 CrossRef CAS PubMed.
- J. J. Li, G. F. Liu, J. T. Zhou, A. J. Wang, J. Wang and R. F. Jin, RSC Adv., 2016, 6, 66930–66937 RSC.
- M. Aivalioti, D. Pothoulaki, P. Papoulias and E. Gidarakos, J. Hazard. Mater., 2012, 207–208, 136–146 CrossRef CAS PubMed.
- A. M. Redding, F. S. Cannon, S. A. Snyder and B. J. Vanderford, Water Res., 2009, 43, 3849–3861 CrossRef CAS PubMed.
- P. Vassileva, P. Tzvetkova and R. Nickolov, Fuel, 2009, 88, 387–390 CrossRef CAS.
- J. Mizera, G. Mizerová, V. Machovič and L. Borecká, Water Res., 2007, 41, 620–626 CrossRef CAS PubMed.
-
W. A. W. M. B, Water and Wastewater Monitoring and Analysis Methods, China Environmental Science Press, Beijing, 4th edn, 2002 Search PubMed.
- W. T. Zhao, Y. X. Shen, K. Xiao and X. Huang, Bioresour. Technol., 2010, 101, 3876–3883 CrossRef CAS PubMed.
- S. R. Liu, W. H. Zhang, L. Wang and X. M. Shan, Jiejingmei Jishu, 2004, 10, 43–45 Search PubMed.
-
N. E. T. Laboratory, Activated carbon from lignite for water treatment, U. S. Department of Energy, 2000 Search PubMed.
-
Z. S. Wang and W. J. Liu, Micropolluted potable water treatment, China Architecture Press, Beijing, 1999 Search PubMed.
- W. Zhang, Q. G. Chang, W. D. Liu, B. J. Li, W. X. Jiang, L. J. Fu and W. C. Ying, Environ. Prog., 2007, 26, 289–298 CrossRef CAS.
|
This journal is © The Royal Society of Chemistry 2017 |
Click here to see how this site uses Cookies. View our privacy policy here.