Acid-protected Eu(III) coordination nanoparticles covered with polystyrene†
Received
12th August 2015
, Accepted 17th November 2015
First published on 19th November 2015
Abstract
Acid-protected Eu(III) coordination nanoparticles composed of Eu(III) complexes, joint ligands, terminate ligands and a protection layer are reported. The prepared acid-protected Eu(III) coordination nanoparticles [Eu(hfa)3(dpbp)x(SDPO)y]n-PS and Eu(III) coordination nanoparticles [Eu(hfa)3(dpbp)x(SDPO)y]n were characterized using ESI-mass spectrometry (ESI-MS), X-ray diffraction (XRD), scanning electron microscopy (SEM), dynamic light scattering (DLS) measurements, and thermogravimetric analysis (TGA). [Eu(hfa)3(dpbp)x(SDPO)y]n-PS shows an effective acid-resistance property based on the polystyrene layer on its nanoparticle surface. The emission intensity is retained under 3000 s in an acidic condition (pH = 0.92). In this study, acid-protected Eu(III) coordination nanoparticles are demonstrated.
Introduction
Lanthanide complexes with narrow emission bands and long emission lifetimes have been regarded as attractive luminescent materials for use in electroluminescent optical materials,1 organic light-emitting diodes (OLEDs),2 and luminescent bio-sensing applications.3 At present, various types of luminescent lanthanide complexes have been reported.4 Lanthanide coordination polymers composed of lanthanide ions and organic joint ligands have also been focused on for new luminescent materials,5 recently. Their tight packing structures promote effective thermo-stability and have a small non-radiative rate constant for strong luminescence. Our research group has reported thermostable lanthanide coordination polymers composed of lanthanide ions and organo-phosphine oxides (decomposition temperature > 300 °C). The lanthanide coordination polymers exhibit a high emission quantum yield (quantum yield = 83%).6 Thermostable lanthanide coordination polymers are expected to be promising candidates for practical opto-electric devices and temperature-sensitive dyes. In particular, temperature-sensitive dyes under 300 °C are used for temperature distribution measurements of material surfaces, such as in wind tunnel experiments with aerospace planes.7
Lanthanide coordination polymers with tight-stacking structures, however, lead to the formation of insoluble compounds, micro-sized particles, in water and organic solvents. The insoluble micro-sized particles cause multiple light scattering in the UV-Vis region. Nanoparticles of lanthanide coordination polymers without multiple light scattering may increase the transmission of optical and luminescent materials. We recently successfully reported that luminescent nanoparticles of lanthanide coordination polymers can be prepared by the addition of monodentate phosphine oxide (triphenyl phosphine oxide: TPPO) as a polymerization terminator.8
In order to improve these nanoparticles for practical material applications, surface protection on the lanthanide coordination nanoparticles is required for the acidic conditions of industrial manufacturing processes. Generally, industrial plastic materials are prepared with an acid generator such as benzoyl peroxide under water. The presence of a H+ source in the polymer material results in decomposition of the lanthanide coordination nanoparticles under moisture. The acid-protection on the lanthanide coordination nanoparticles is expected to be achieved by an encapsulation technique using glass materials. Hongshang has demonstrated luminescent Eu3+ chelate nanoparticles covered with octyltrimethoxysilane for encapsulation.9 Fukuda has reported lanthanide complexes encapsulated with sol–gel glass.10 Silica glass or materials are, however, decomposed under acidic conditions. Encapsulation using an organic polymer attached to the lanthanide coordination nanoparticles may be the most favourable for luminescent materials under moisture.
Here we have attempted to introduce terminator ligands with vinyl groups on the surface of the lanthanide coordination nanoparticles. The vinyl groups of terminator ligands, diphenyl(p-vinylphenyl)phosphine oxide (SDPO),11 can promote polymerization for surface protection under acidic conditions (Fig. 1a). The size-controlled nanoparticles are obtained by the reaction of Eu(hfa)3(H2O)2 (hfa: hexafluoroacetylacetonate) with joint ligands (dpbp: 4,4′-bis(diphenylphosphoryl)biphenyl) and terminator ligands (SDPO) ([Eu(hfa)3(dpbp)x(SDPO)y]n). The acid-protected Eu(III) coordination nanoparticles are prepared by the polymerization of [Eu(hfa)3(dpbp)x(SDPO)y]n with styrene monomers under 80 °C ([Eu(hfa)3(dpbp)x(SDPO)y]n-PS (PS: polystyrene, Fig. 1b)).
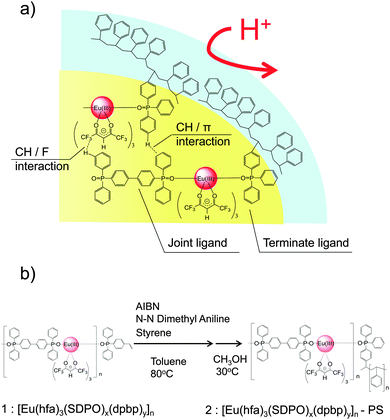 |
| Fig. 1 Preparation scheme of acid protected lanthanide coordination nanoparticles covered with polystyrene. | |
[Eu(hfa)3(dpbp)x(SDPO)y]n and [Eu(hfa)3(dpbp)x(SDPO)y]n-PS were characterized using ESI-MS, XRD, SEM, DLS measurements, and TGA. The decomposition temperatures of [Eu(hfa)3(dpbp)x(SDPO)y]n and [Eu(hfa)3(dpbp)x(SDPO)y]n-PS from TGA were determined to be 236 °C and 250 °C, respectively. The emission properties of [Eu(hfa)3(dpbp)x(SDPO)y]n and [Eu(hfa)3(dpbp)x(SDPO)y]n-PS were estimated using emission spectra, excitation spectra, diffuse reflection spectra and emission lifetimes. We have successfully observed the effective acid-resistant ability of [Eu(hfa)3(dpbp)x(SDPO)y]n-PS for practical use under moisture conditions. In this study, acid-protected Eu(III) coordination nanoparticles are demonstrated for the first time.
Experimental
Materials
Europium acetate monohydrate (99.9%), styrene monomer (>99%), and diphenyl(p-vinylphenyl)phosphine (>97%) were purchased from Wako Pure Chemical Industries Ltd. 1,1,1,5,5,5-Hexafluoro-2,4-pentanedione, 4,4′-dibromobiphenyl (>98%) and potassium peroxymonosulfate [>45%(T) as KHSO5] were obtained from Tokyo Kasei Organic Chemicals. All other chemicals and solvents were reagent grade and were used without further purification.
Apparatus
Infrared spectra were recorded with a JASCO FTIR-350 spectrometer. 1H NMR (400 MHz) spectra were recorded with a JEOL ECS400 spectrometer. Chemical shifts are reported in ppm and are referenced to an internal tetramethylsilane standard for 1H NMR spectroscopy. Elemental analyses were performed with a Yanaco CNH MT-6 analyzer. Thermogravimetric analysis (TGA) was performed on a SEIKO EXSTAR 6000 (TG-DTA 6300). Size-distributions were measured with a BECKMAN COULTER Delsa Nano HC. Scanning electron microscopy (SEM) was performed with a JEOL JSM-6510LA (acceleration voltage, 15 kV). Mass spectrometry was performed with a JEOL JMS-T100LP. X-ray photoelectron spectroscopy (XPS) was performed with a JPS-9200 using unmonochromatized Al Kα X-ray.
Preparation of tris(hexafluoroacetylacetonato)europium dihydrate [Eu(hfa)3(H2O)2]
Europium acetate monohydrate (5.0 g, 13 mmol) was dissolved in distilled water (20 mL) in a 100 mL flask. A solution of 1,1,1,5,5,5-hexafluoro-2,4-pentanedione (7.0 g, 34 mmol) was added dropwise to the solution. The reaction mixture produced a white-yellow precipitate after stirring for 3 h at room temperature. The powder was collected by filtration and recrystallized from methanol/water to afford colorless needle crystals of the title compound, yield 9.6 g (95%). IR (KBr): 1650 (s, C
O), 1145–1258 (s, C–F) cm−1. Anal. calcd for C15H7EuF18O8: C, 22.48%; H, 0.88%; found: C, 22.12%; H, 1.01%.
Preparation of 4,4′-bis(diphenylphosphoryl)biphenyl [dpbp]
4,4′-Bis(diphenylphosphoryl)-biphenyl was synthesized according to the published procedure.6 A solution of n-BuLi (9.3 mL, 1.6 M hexane, 15 mmol), was added dropwise to a solution of 4,4′-dibromobiphenyl (1.9 g, 6.0 mmol) in dry THF (30 mL) at −80 °C. The addition was completed in ca. 15 min, during which time a yellow precipitate was formed. The mixture was allowed to stir for 3 h at −10 °C, after which PPh2Cl (2.7 mL, 15 mmol) was added dropwise at −80 °C. The mixture was gradually brought to room temperature, and stirred for 14 h. The product was extracted with ethyl acetate, and the extracts were washed with brine three times and dried over anhydrous MgSO4. The solvent was evaporated, and the resulting residue was washed with acetone and ethanol several times. The obtained white solid and dichloromethane (ca. 40 mL) were placed in a flask. The solution was cooled to 0 °C and then 30% H2O2 aqueous solution (5 mL) was added to it. The reaction mixture was stirred for 2 h. The product was extracted with dichloromethane, and the extracts were washed with brine three times and dried over anhydrous MgSO4. The solvent was evaporated to afford a white powder. Recrystallization from dichloromethane gave white crystals of the titled compound.
Yield: 1.1 g (33%). IR (KBr): 1120 (st, P
O) cm−1.1H NMR (400 MHz, CDCl3, 25 °C) δ 7.67–7.80 (m, 16H; P-C6H5, C6H4), 7.45–7.60 (m, 12H; P-C6H5, C6H4) ppm. MS (ESI) found: m/z = 555.2, calcd for C36H29O2P2: [M + H]+, 555.2. Anal. calcd for C36H28O2P2: C, 77.97%; H, 5.09%. Found: C, 77.49%; H, 5.20%.
Preparation of diphenyl(p-vinylphenyl)phosphine oxide [SDPO]
Diphenyl(p-vinylphenyl)phosphine oxide (SDPO) was synthesized according to the published procedure.12 Diphenyl(p-vinylphenyl)phosphine (5 g, 17.4 mmol) was dissolved in dichloromethane (100 mL) in a 300 mL flask. A saturated aqueous solution of oxone (21.4 g, 34.8 mmol) and methanol (20 mL) was added to the mixture. The mixture was stirred for 3 h. The product was extracted with dichloromethane, and the extracts were washed with brine three times and dried over anhydrous MgSO4. The solvent was evaporated, and the resulting residue was washed with cyclohexane several times. 1H NMR (400 MHz, CDCl3, 25 °C) δ 7.46–7.70 (m, 14H; P-C6H5, C6H4), 6.71 (dd, 1H; CH), 5.84 (d, 1H; CH2), 5.37 (d, 1H; CH2) ppm. MS (ESI) found: m/z = 327.1, calcd for C20H17OPNa: [SDPO + Na]+, 327.1. Anal. calcd for C20H17OP: C, 78.93%; H, 5.63%. Found: C, 77.30%; H, 5.64%.
Preparation of nanoparticles composed of lanthanide coordination polymers [Eu(hfa)3(SDPO)x(dpbp)y]n
The amount of bidentate dpbp ligands in [Eu(hfa)3(SDPO)x(dpbp)1−x]n (x: molecular ratio of dpbp) was controlled under 0.70 (70%). In the molecular ratio of [Eu(hfa)3(SDPO)0.70(dpbp)0.30]n, bidentate dpbp ligands (204 mg, 0.37 mmol) and monodentate SDPO ligands (262 mg, 0.86 mmol) were dissolved in methanol (25 mL) in a 50 mL flask. A methanol solution (10 mL) of Eu(hfa)3(H2O)2 (1 g, 1.23 mmol) was added at room temperature. The reaction mixture was stirred for 6 h. The product was centrifuged at 4000 rpm for 20 minutes and the white powder of nanoparticles composed of Eu(hfa)3(H2O)2, dpbp and SDPO was prepared. Nanoparticles composed of lanthanide coordination polymers were washed twice with methanol, and then dried. MS (ESI) found: m/z = 1425.2, calcd for C66H47EuF12O7P3: [Eu(hfa)2(dpbp)(SDPO)]+, 1425.2, found: m/z = 1633.2, calcd for C71H49EuF18O9P3: [Eu(hfa)3(dpbp)(SDPO) + H]+, 1633.1.
Preparations of Eu(III) coordination nanoparticles covered with polystyrene
The Eu(III) coordination nanoparticles of [Eu(hfa)3(SDPO)x(dpbp)y]n (100 mg) and AIBN (azobis(isobutyronitrile))(2.2 mg, 13.4 μmol) were mixed in toluene (10 mL) in a 50 mL flask. The mixture was allowed to stir for 1 h at 80 °C, after which a styrene monomer (1 mL, 8.7 mmol) was injected by syringe. The reaction mixture was stirred for 2 h at 80 °C and then was cooled to room temperature. The reaction mixture was added to methanol (50 mL). The solvent was evaporated, and the resulting residue was washed with hexane several times. MS (ESI) found: m/z = 1199.2, calcd for C44H33EuF18O7P: [Eu(hfa)3(SDPO)(PS)1 + H]+, 1199.1, found: m/z = 1407.1, calcd for C60H49EuF18O7P: [Eu(hfa)3(SDPO)(PS)3 + H]+, 1407.2, found: m/z = 2240.0, calcd for C124H113EuF18O7P: [Eu(hfa)3(SDPO)(PS)11 + H]+, 2239.7, found: m/z = 2447.9, calcd for C140H129EuF18O7P: [Eu(hfa)3(SDPO)(PS)13 + H]+, 2447.8, found: m/z = 2677.9, calcd for C156H144EuF18O7PNa: [Eu(hfa)3(SDPO)(PS)15 + Na]+, 2677.9, found: m/z = 2864.2, calcd for C172H161EuF18O7P: [Eu(hfa)3(SDPO)(PS)17 + H]+, 2864.1, found: m/z = 3510.1, calcd for C220H208EuF18O7PNa: [Eu(hfa)3(SDPO)(PS)23 + Na]+, 3510.4 (PS: unit of polystyrene).
Optical measurements
The emission, excitation and diffuse reflection spectra of [Eu(hfa)3(dpbp)x(SDPO)y]n and [Eu(hfa)3(dpbp)x(SDPO)y]n-PS were measured with a JASCO V-670 spectrophotometer and JASCO F-6300-H spectrometer, which corrected for the response of the detector system for the spectral correction system of optical measurement. The emission lifetimes of the luminescent nanoparticles (the powder) were measured using the third harmonic (355 nm) of a Q-switched Nd:YAG laser [Spectra Physics, INDI-50, full width at half maximum (fwhm) = 5 ns and λ = 1064 nm] and a photomultiplier (Hamamatsu photonics, R5108, response time 1.1 ns). The Nd:YAG laser response was monitored with a digital oscilloscope (Sony Tektronix, TDS3052, 500 MHz) synchronized to the single pulse excitation. The emission lifetimes were determined from the slope of the logarithmic plots of the decay profiles. The emission quantum yields of [Eu(hfa)3(dpbp)x(SDPO)y]n and [Eu(hfa)3(dpbp)x(SDPO)y]n-PS (powders) excited at 370 nm were estimated using a JASCO F-6300-H spectrometer. The photophysical properties of [Eu(hfa)3(dpbp)x(SDPO)y]n and [Eu(hfa)3(dpbp)x(SDPO)y]n-PS in the solid-state were investigated from the estimation of the 4f–4f emission quantum yields (ΦLn), and the radiative (kr) and non radiative (knr) rate constants from the radiative (τrad) and observed lifetimes (τobs). The radiative and observed lifetimes are expressed by:
ΦLn, kr and knr of [Eu(hfa)3(dpbp)x(SDPO)y]n and [Eu(hfa)3(dpbp)x(SDPO)y]n-PS are given by:
where AMD,0 is the spontaneous emission probability for the 5D0–7F1 transition in vacuo (14.65 s−1), n is the refractive index of the medium (an average index of refraction equal to 1.5 was employed), and (Itot/IMD) is the ratio of the total area of the corrected Eu(III) emission spectrum, to the area of the 5D0–7F1 band.
Estimation of acid-resistant properties
Acid-resistant properties of [Eu(hfa)3(dpbp)x(SDPO)y]n and [Eu(hfa)3(dpbp)x(SDPO)y]n-PS were estimated by the change of the emission intensity under acidic conditions. An amount (5 mg) of [Eu(hfa)3(dpbp)x(SDPO)y]n and [Eu(hfa)3(dpbp)x(SDPO)y]n-PS was dispersed in ethanol (2 mL), and then 12% nitric acid (100 μL) was added. The decomposition processes are observed using the emission spectra of [Eu(hfa)3(dpbp)x(SDPO)y]n and [Eu(hfa)3(dpbp)x(SDPO)y]n-PS excited at 370 nm (JASCO F-6300-H spectrometer). The decomposition indexes are calculated using the emission intensity at 613 nm (for 0–4000 s).
Results and discussion
Structural characterizations
The structures of the prepared [Eu(hfa)3(dpbp)x(SDPO)y]n and [Eu(hfa)3(dpbp)x(SDPO)y]n-PS were characterized using powder XRD measurements. These XRD spectra are shown in Fig. 2a. Peaks for [Eu(hfa)3(dpbp)x(SDPO)y]n were observed at 7.59°, 8.85°, 9.46°, 20.13°, 20.65° and 21.45°. Peaks for [Eu(hfa)3(dpbp)x(SDPO)y]n-PS were also observed at 7.59°, 8.85°, 9.46°, 20.13°, 20.65° and 21.45°, which are the same as those for [Eu(hfa)3(dpbp)x(SDPO)y]n. The 2θ diffraction peaks of [Eu(hfa)3(dpbp)x(SDPO)y]n and [Eu(hfa)3(dpbp)x(SDPO)y]n-PS were similar to the corresponding peaks for the previously reported [Eu(hfa)3(dpbp)]n.6 These results indicate that the geometrical structures of the nanoparticles are the same as those of [Eu(hfa)3(dpbp)]n (see ESI,† Fig. S1).
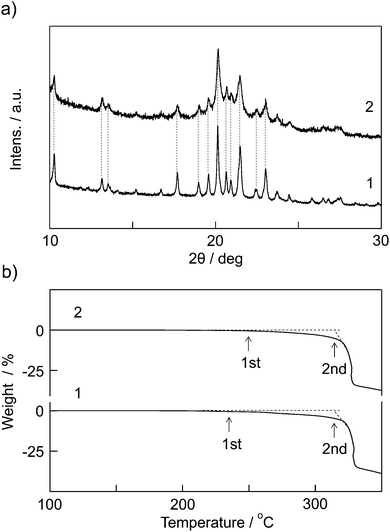 |
| Fig. 2 (a) XRD patterns of 1: [Eu(hfa)3(dpbp)x(SDPO)y]n and 2: [Eu(hfa)3(dpbp)x(SDPO)y]n-PS. (b) TGA curves of 1: [Eu(hfa)3(dpbp)x(SDPO)y]n and 2: [Eu(hfa)3(dpbp)x(SDPO)y]n-PS in an argon atmosphere at a heating rate of 2 °C min−1. | |
The polystyrene on the nanoparticle surface was observed using ESI-MS. This result indicates the formation of [Eu(hfa)3(dpbp)x(SDPO)y]n covered with amorphous polystyrene. Unfortunately, we did not observed an effective IR-signal of the polystyrene thin layer on the nanoparticles (see ESI,† Fig. S2).
Quantitative analysis of carbon in [Eu(hfa)3(dpbp)x(SDPO)y]n and [Eu(hfa)3(dpbp)x(SDPO)y]n-PS was carried out using X-ray photoelectron spectroscopy (XPS). The XPS of C1s, Eu3d5/2, and F1s are shown in the ESI,† Fig. S3. The atomic ratio of carbon in [Eu(hfa)3(dpbp)x(SDPO)y]n and [Eu(hfa)3(dpbp)x(SDPO)y]n-PS using the C1s and F1s spectra were found to be 3.0 and 11.1, respectively. The atomic ratio in [Eu(hfa)3(dpbp)x(SDPO)y]n-PS is 3.7 times larger than that in [Eu(hfa)3(dpbp)x(SDPO)y]n. We consider that [Eu(hfa)3(dpbp)x(SDPO)y]n-PS is covered with a large amount of polystyrene in the layer.
The thermal stability of [Eu(hfa)3(dpbp)x(SDPO)y]n and [Eu(hfa)3(dpbp)x(SDPO)y]n-PS was evaluated using TGA (Fig. 2b). The Eu(III)-nanoparticle [Eu(hfa)3(dpbp)x(SDPO)y]n and [Eu(hfa)3(dpbp)x(SDPO)y]n-PS show two decomposition temperatures. The first decomposition temperatures of [Eu(hfa)3(dpbp)x(SDPO)y]n and [Eu(hfa)3(dpbp)x(SDPO)y]n-PS were found to be 236 °C and 250 °C, respectively. The first decomposition temperatures are due to the elimination of terminator ligands (SDPO) and polystyrene. The second decomposition temperatures for Eu(III)-nanoparticle [Eu(hfa)3(dpbp)x(SDPO)y]n and [Eu(hfa)3(dpbp)x(SDPO)y]n-PS were found to be 316 °C and 314 °C, respectively. The second decomposition temperatures agree well with that of the standard coordination polymer [Eu(hfa)3(dpbp)]n (see ESI,† Fig. S4).
The SEM images of [Eu(hfa)3(dpbp)x(SDPO)y]n and [Eu(hfa)3(dpbp)x(SDPO)y]n-PS are shown in Fig. 3a. We found a sphere shape for the nanoparticles. The particle size distribution of [Eu(hfa)3(dpbp)x(SDPO)y]n and [Eu(hfa)3(dpbp)x(SDPO)y]n-PS was characterized using DLS measurements. These size distributions are shown in Fig. 3b (for the scattering intensities of the DLS measurement: see ESI,† Fig. S5). The maximum distributions of [Eu(hfa)3(dpbp)x(SDPO)y]n and [Eu(hfa)3(dpbp)x(SDPO)y]n-PS are estimated to be 156.7 nm and 272.9 nm, respectively. We observed an increase of the particle size of [Eu(hfa)3(dpbp)x(SDPO)y]n-PS for the formation of the amorphous polystyrene thin layers on the [Eu(hfa)3(dpbp)x(SDPO)y]n surface.
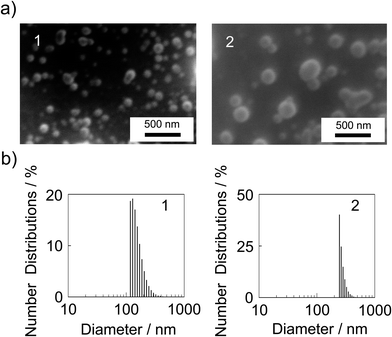 |
| Fig. 3 (a) SEM images of 1: [Eu(hfa)3(dpbp)x(SDPO)y]n and 2: [Eu(hfa)3(dpbp)x(SDPO)y]n-PS. (b) Number distributions of 1: [Eu(hfa)3(dpbp)x(SDPO)y]n and 2: [Eu(hfa)3(dpbp)x(SDPO)y]n-PS using DLS measurements. | |
Emission properties
The emission spectra of [Eu(hfa)3(dpbp)x(SDPO)y]n and [Eu(hfa)3(dpbp)x(SDPO)y]n-PS in the solid-state are shown in Fig. 4a. Emission bands of [Eu(hfa)3(dpbp)x(SDPO)y]n and [Eu(hfa)3(dpbp)x(SDPO)y]n-PS excited at 370 nm were observed at 578, 592, 613, 650 and 698 nm, and are attributed to the 4f–4f transitions of 5D0–7FJ with J = 0, 1, 2, 3 and 4, respectively. The emission spectra were normalized with respect to the magnetic dipole transition intensities at 592 nm (Eu(III): 5D0–7F1), which are known to be insensitive to the surrounding environment of the lanthanide ions. The decrease in the emission intensity of [Eu(hfa)3(SDPO)x(dpbp)y-PS]n is caused by the polystyrene layer on the surface of the Eu(III) coordination nanoparticles.
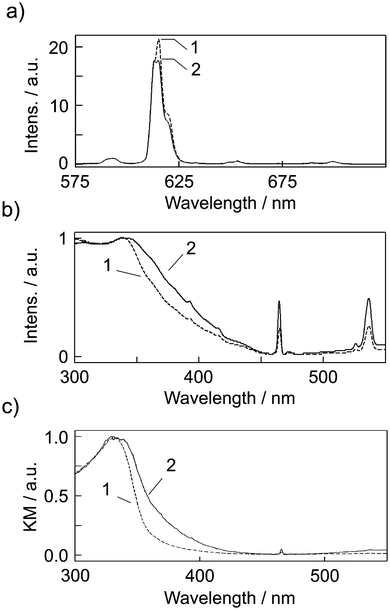 |
| Fig. 4 (a) Emission spectra of 1: [Eu(hfa)3(dpbp)x(SDPO)y]n and 2: [Eu(hfa)3(dpbp)x(SDPO)y]n-PS in the solid state at room temperature. Excited at 370 nm. (b) Excitation spectra of 1: [Eu(hfa)3(dpbp)x(SDPO)y]n and 2: [Eu(hfa)3(dpbp)x(SDPO)y]n-PS in the solid state at room temperature. Monitored at 613 nm. (c) Diffuse reflection spectra of 1: [Eu(hfa)3(dpbp)x(SDPO)y]n and 2: [Eu(hfa)3(dpbp)x(SDPO)y]n-PS in the solid state at room temperature. | |
Fig. 4b and c show excitation and diffuse reflection spectra for [Eu(hfa)3(dpbp)x(SDPO)y]n and [Eu(hfa)3(dpbp)x(SDPO)y]n-PS in the solid state at room temperature. The unit of the diffuse reflection spectra (Fig. 4c) is the Kubelka–Munk (KM), which depends on the surface of the materials. The excitation and KM intensity of [Eu(hfa)3(SDPO)x(dpbp)y-PS]n increases in comparison with [Eu(hfa)3(dpbp)x(SDPO)y]n. This phenomenon is caused by the polymerization of polystyrene on the surface of the Eu(III) coordination nanoparticles. The increased absorbance bands of [Eu(hfa)3(SDPO)x(dpbp)y-PS]n from 340 nm to 450 nm are derived from the hfa ligands, because the absorbance bands of the polystyrene are less than 300 nm. We consider that the increased absorbance bands of [Eu(hfa)3(SDPO)x(dpbp)y-PS]n are caused by the interaction between the hfa ligands and polystyrene. The specific absorbance bands are similar to an ILCT (inter-ligand charge transfer) band of hfa ligands in the solid-state.13
Fig. 5 shows time-resolved emission decay profiles for [Eu(hfa)3(dpbp)x(SDPO)y]n and [Eu(hfa)3(dpbp)x(SDPO)y]n-PS in the solid-state. The emission lifetimes were determined from the slopes of the logarithmic plots of the decay profiles. The emission decay of [Eu(hfa)3(dpbp)x(SDPO)y]n (Fig. 5a) and [Eu(hfa)3(dpbp)x(SDPO)y]n-PS (Fig. 5b) have one component (0.66 ± 0.01 ms and 0.64 ± 0.01 ms).
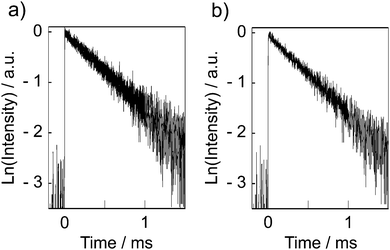 |
| Fig. 5 Emission decays of (a): [Eu(hfa)3(dpbp)x(SDPO)y]n and (b): [Eu(hfa)3(dpbp)x(SDPO)y]n-PS in the solid state at room temperature excited at 355 nm (third harmonic of a Q-switched Nd:YAG laser: fwhm = 5 ns and λ = 1064 nm). | |
The emission lifetimes are similar to that of the previous [Eu(hfa)3(dpbp)0.20(TPPO)0.80]n (τ = 0.66 ± 0.01 ms).8 These emission lifetimes are smaller than that of the bulk Eu(III) coordination powder [Eu(hfa)3(dpbp)]n (τ = 0.91 ± 0.01 ms). The smaller emission lifetimes of [Eu(hfa)3(dpbp)x(SDPO)y]n and [Eu(hfa)3(dpbp)x(SDPO)y]n-PS may be due to the presence of vibrational relaxation on the nanoparticle surface covered with terminate ligands (SDPO).
The intrinsic 4f–4f emission quantum yields of [Eu(hfa)3(dpbp)x(SDPO)y]n and [Eu(hfa)3(dpbp)x(SDPO)y]n-PS in the solid-state were also determined based on the emission lifetime with a single-exponential decay and 4f–4f transition probability (Table 1).6 The 4f–4f emission quantum yield (ΦLn) of [Eu(hfa)3(dpbp)x(SDPO)y]n and [Eu(hfa)3(dpbp)x(SDPO)y]n-PS are calculated to be 70% and 63%, respectively.
Table 1 Photophysical properties of [Eu(hfa)3(dpbp)x(SDPO)y]n and [Eu(hfa)3(dpbp)x(SDPO)y]n-PS in the solid-state
Eu(III) nanoparticles |
τ
obs
/ms |
τ
rad
/ms |
Φ
Ln
/% |
k
r
/s−1 |
k
nr
/s−1 |
Observed lifetimes (τobs) of the Eu(III) coordination nanoparticles were measured by excitation at 355 nm (Nd:YAG 3ω).
Radiative lifetimes τrad = 1/AMD,0n3(Itot/IMD). AMD,0 is the spontaneous emission probability for the 5D0–7F1 transition in vacuo (14.65 s−1), n is the refractive index of the medium (an average index of refraction equal to 1.5 was employed), and (Itot/IMD) is the ratio of the total area of the corrected Eu(III) emission spectrum to the area of the 5D0–7F1 band.
4f–4f emission quantum yields ΦLn = τobs/τrad.
Radiative rate constants kr = 1/τrad.
Non-radiative rate constants knr = 1/τobs − kr.
|
[Eu(hfa)3(dpbp)x(SDPO)y]n |
0.66 |
0.94 |
0.70 |
1.1 × 103 |
4.5 × 102 |
[Eu(hfa)3(dpbp)x(SDPO)y]n-PS |
0.64 |
1.02 |
0.63 |
9.8 × 102 |
5.8 × 102 |
Fig. 6 shows the time dependences of the emission intensities of [Eu(hfa)3(dpbp)x(SDPO)y]n and [Eu(hfa)3(dpbp)x(SDPO)y]n-PS under an acidic condition (pH = 0.92). We observed an effective decrease of the emission intensity of [Eu(hfa)3(dpbp)x(SDPO)y]n (circles). The emission intensity was found to be 60% at around 3000 s. In contrast, the emission intensity of [Eu(hfa)3(dpbp)x(SDPO)y]n-PS was decreased at 500 s (88%), and then the emission intensity was retained under 3000 s (80%, squares). The acid resistant property of [Eu(hfa)3(dpbp)x(SDPO)y]n-PS is successfully improved using a polystyrene layer composed of SDPO and styrene monomers on the nanoparticles surface.
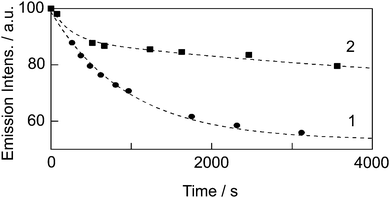 |
| Fig. 6 Time dependences of the emission intensities of 1: [Eu(hfa)3(dpbp)x(SDPO)y]n (circles) and 2: [Eu(hfa)3(dpbp)x(SDPO)y]n-PS (squares) under an acidic condition (pH = 0.92). | |
Conclusions
We successfully prepared acid-protected Eu(III) coordination nanoparticles covered with polystyrene. The [Eu(hfa)3(dpbp)x(SDPO)y]n-PS is effectively protected by a polystyrene layer on the nanoparticle surface. The emission intensity is retained under 3000 s in an acidic condition (pH = 0.92). Acid-protected Eu(III) coordination nanoparticles may be useful in applications such as temperature-sensitive dyes and luminescent display under high temperature and humidity conditions. Recently, we successfully prepared thin films composed of Eu(III) coordination polymers for EL devices.14 Polystyrene-coated Eu(III) luminescent nanoparticles are expected to open up new material fields of photo-functional organo and metal complexes.
Acknowledgements
This work was partly supported by Grants-in-Aid for Scientific Research on Innovative Areas of “New Polymeric Materials Based on Element-Blocks (No. 2401)” (24102012) of the Ministry of Education, Culture, Sports, Science and Technology (MEXT) of Japan.
References
-
(a)
G. Blasse and B. C. Grabmaier, Luminescent Materials, Springer-Verlag, New York, 1994 Search PubMed;
(b)
A. Vogler and H. Kunkely, Luminescent Metal Complexes: Diversity of Excited States, in Transition Metal and Rare Earth Compounds, ed. H. Yersin, Topics in Current Chemistry, Springer-Verlag, New York, 2001, vol. 213 Search PubMed.
-
(a) R. Gao, D. G. Ho, B. Hernandez, M. Selke, D. Murphy, P. I. Djurovich and M. E. Thompson, J. Am. Chem. Soc., 2002, 124, 14828 CrossRef CAS PubMed;
(b) J. Li, P. I. Djurovich, B. D. Alleyne, M. Yousufuddin, N. N. Ho, J. C. Thomas, J. C. Peters, R. Bau and M. E. Thompson, Inorg. Chem., 2005, 44, 1713 CrossRef CAS PubMed;
(c) X.-M. Yu, G.-J. Zhou, C.-S. Lam, W.-Y. Wong, X.-L. Zhu, J.-X. Sun, M. Wong and H.-S. Kwok, J. Organomet. Chem., 2008, 693, 1528 CrossRef.
-
(a) N. Weibel, L. J. Charbonniere, M. Guardigli, A. Roda and R. Ziessel, J. Am. Chem. Soc., 2004, 126, 4888 CrossRef CAS PubMed;
(b) J.-C. G. Bünzli and C. Piguet, Chem. Soc. Rev., 2005, 34, 1048 RSC;
(c) S. Faulkner and B. P. Burton-Pye, Chem. Commun., 2005, 259 RSC;
(d) J. Yu, D. Parker, R. Pal, R. A. Poole and M. J. Cann, J. Am. Chem. Soc., 2006, 128, 2294 CrossRef CAS PubMed;
(e) B. McMahon, P. Mauer, C. P. McCoy, T. C. Lee and T. Gunnlaugsson, J. Am. Chem. Soc., 2009, 131, 17542 CrossRef CAS PubMed.
-
(a) A. Ablet, S.-M. Li, W. Cao, X.-J. Zheng, W.-T. Wong and L.-P. Jin, Chem. – Asian J., 2013, 8, 95 CrossRef CAS PubMed;
(b) J. Xu, L. Jia, N. Jin, Y. Ma, X. Liu, W. Wu, W. Liu, Y. Tang and F. Zhou, Chem. – Eur. J., 2013, 19, 4556 CrossRef CAS PubMed;
(c) N. Wartenberg, O. Raccurt, E. Bourgeat-Lami, D. Imbert and M. Mazzanti, Chem. – Eur. J., 2013, 19, 3477 CrossRef CAS PubMed;
(d) C. Camp, J. Pécaut and M. Mazzanti, J. Am. Chem. Soc., 2013, 135, 12101 CrossRef CAS PubMed;
(e) C. Zhao, Q.-F. Sun, W. M. Hart-Cooper, A. G. DiPasquale, F. D. Toste, R. G. Bergman and K. N. Raymond, J. Am. Chem. Soc., 2013, 135, 18802 CrossRef CAS PubMed;
(f) S. Biju, N. Gopakumar, J.-C. G. Bünzli, R. Scopelliti, H. K. Kim and M. L. P. Reddy, Inorg. Chem., 2013, 52, 8750 CrossRef CAS PubMed;
(g) D. Sykes, S. C. Parker, I. V. Sazanovich, A. Stephenson, J. A. Weinstein and M. D. Ward, Inorg. Chem., 2013, 52, 10500 CrossRef CAS PubMed;
(h) M. L. P. Reddy and S. Sivakumar, Dalton Trans., 2013, 42, 2663 RSC;
(i) A. C. Mendonça, A. F. Martins, A. Melchior, S. M. Marques, S. Chaves, S. Villette, S. Petoud, P. L. Zanonato, M. Tolazzi, C. S. Bonnet, É. Tóth, P. D. Bernardo, C. F. G. C. Geraldesb and M. A. Santos, Dalton Trans., 2013, 42, 6046 RSC;
(j) J. W. Walton, A. Bourdolle, S. J. Butler, M. Soulie, M. Delbianco, B. K. McMahon, R. Pal, H. Puschmann, J. M. Zwier, L. Lamarque, O. Maury, C. Andraudb and D. Parker, Chem. Commun., 2013, 49, 1600 RSC;
(k) K. L. Gempf, S. J. Butler, A. M. Funk and D. Parker, Chem. Commun., 2013, 49, 9104 RSC;
(l) E. R. Trivedi, S. V. Eliseeva, J. Jankolovits, M. M. Olmstead, S. Petoud and V. L. Pecoraro, J. Am. Chem. Soc., 2014, 136, 1526 CrossRef CAS PubMed;
(m) A. J. Metherell, W. Cullen, A. Stephenson, C. A. Hunter and M. D. Ward, Dalton Trans., 2014, 43, 71 RSC;
(n) E. Debroye, S. V. Eliseeva, S. Laurent, L. V. Elst, R. N. Mullerd and T. N. Parac-Vogt, Dalton Trans., 2014, 43, 3589 RSC;
(o) N. J. Rogers, S. Claire, R. M. Harris, S. Farabi, G. Zikeli, I. B. Styles, N. J. Hodges and Z. Pikramenou, Chem. Commun., 2014, 50, 617 RSC.
-
(a) J. Rocha, L. D. Carlos, F. A. A. Paza and D. Ananias, Chem. Soc. Rev., 2011, 40, 926 RSC;
(b) C. Daiguebonne, N. Kerbellec, O. Guillou, J.-C. Bunzli, F. Gumy, L. Catala, T. Mallah, N. Audebrand, Y. Gérault, K. Bernot and G. Calvez, Inorg. Chem., 2008, 47, 3700 CrossRef CAS PubMed;
(c) Y. Hasegawa and T. Nakanishi, RSC Adv., 2015, 5, 338 RSC.
- K. Miyata, T. Ohba, A. kobayashi, M. Kato, T. Nakanishi, K. Fushimi and Y. Hasegawa, ChemPlusChem, 2012, 77, 277 CrossRef CAS.
- K. Miyata, Y. Konno, T. Nakanishi, A. kobayashi, M. Kato, T. Nakanishi, K. Fushimi and Y. Hasegawa, Angew. Chem., Int. Ed., 2013, 52, 6413 CrossRef CAS PubMed.
- H. Onodera, A. Nakajima, T. Nakanishi, K. Fushimi and Y. Hasegawa, e-J. Surf. Sci. Nanotechnol., 2015, 13, 219 CrossRef CAS.
- H. Peng, C. Wu, Y. Jiang, S. Huang and J. McNeill, Langmuir, 2007, 23, 1591 CrossRef CAS PubMed.
- T. Fukuda, S. Kato, E. Kin, K. Okaniwa, H. Morikawa, Z. Honda and N. Kamata, Opt. Mater., 2009, 32, 22 CrossRef CAS.
- A. Kusumaatmaja, T. Ando, K. Terada, S. Hirohara, T. Nakashima, T. Kawai, T. Terashima and M. Tanihara, J. Polym. Sci., Part A: Polym. Chem., 2013, 51(12), 2527 CrossRef CAS.
- J. Sanmartin, M. R. Bermejo, A. Sousa, M. Fondo, E. Gomez-Forneas and C. McAuliffe, Acta Chem. Scand., 1997, 51, 59 CrossRef CAS.
-
(a) S. V. Eliseeva, O. V. Kotova, F. Gumy, S. N. Semenov, V. G. Kessler, L. S. Lepnev, J.-C. G. Bünzli and N. P. Kuzmina, J. Phys. Chem. A, 2008, 112, 3614 CrossRef CAS PubMed;
(b) Y. Hasegawa, R. Hieda, T. Nakagawa and T. Kawai, Helv. Chim. Acta, 2009, 92, 2238 CrossRef CAS;
(c) Y. Hasegawa, R. Hieda, K. Miyata, T. Nakagawa and T. Kawai, Eur. J. Inorg. Chem., 2011, 4978 CrossRef CAS.
- Y. Hasegawa, T. Sugawara, T. Nakanishi, Y. Kitagawa, M. Takada, A. Niwa, H. Naito and K. Fushimi, ChemPlusChem, 2015 DOI:10.1021/cplu.201500382.
Footnote |
† Electronic supplementary information (ESI) available: XRD pattern and TGA curve of [Eu(hfa)3(dpbp)]n. Infrared spectra and scattering intensities of Eu(III) coordination nanoparticles. See DOI: 10.1039/c5tc02497h |
|
This journal is © The Royal Society of Chemistry 2016 |