DOI:
10.1039/C5SM02043C
(Communication)
Soft Matter, 2016,
12, 26-30
Wetting films of aqueous solutions of Silwet L-77 on a hydrophobic surface
Received
15th August 2015
, Accepted 1st October 2015
First published on 2nd October 2015
Abstract
The formation of wetting films of aqueous solutions of Silwet L-77 on hydrophobic substrates takes place only at concentrations above the critical aggregation concentration (CAC). At concentrations above the critical wetting concentration (CWC) a new phenomenon was found: the formation of multilayered spots of thicker films in the wetting film of aqueous solutions of Silwet L-77 on hydrophobic surfaces. An expansion of the thicker spots within the film and the formation of “channels” between the spots and the edge of the film led to a continuous shrinkage of the wetting film and its disappearance in the end. We suggested that the multiple thicker films originate from the multilayer structuring of trisiloxane bilayers within the wetting film.
Trisiloxane polyoxyethylene surfactants are of great importance in the agricultural, textile and paint industries due to their ability to improve significantly the wetting of low energy surfaces.1,2 The phenomenon of spontaneous fast spreading of aqueous trisiloxane surfactant solutions is referred to as “superspreading”.1,2 The mechanism behind this phenomenon is a challenging problem and has been debated over two decades.2 Most of the research studies on this phenomenon have been concentrated on studying the spreading of trisiloxane solutions on hydrophobic surfaces,1–3 the adsorption of trisiloxanes on interfaces2,4,5 or the phase behavior of molecules in bulk solutions.6 At the same time, there are very limited published results on the behavior of wetting films of trisiloxane surfactant solutions7,8 that could give information on the role of the surface forces responsible for the superspreading mechanism. Churaev et al.7 studied a spontaneous climbing of trisiloxane surfactant solution on an inclined hydrophobic substrate against gravity action and found that the wetting film was up to 4 microns thick. It has been suggested that the formation of such a thick wetting film is due to the mutual repulsion of vesicles and the action of long-range structural forces.7 Recently, Sett et al.8 have shown that in the course of gravitational drainage of vertical free films of superspreader solutions the films stabilize at a thickness of about 35 nm and have a longer lifetime before rupture compared to that of non-superspreader trisiloxanes. The film stabilization of superspreader trisiloxanes was explained by the formation of long bilayers hanging from the free surfaces.8 The presence of vesicles and bilayer aggregates and their direct adsorption at the interfaces seem to be the dominating hypothesis1–5 proposed for the explanation of superspreading mechanisms.
An important feature of the superspreading phenomenon is the existence of the critical wetting concentration (CWC), above which superspreading starts to occur.3,9 The liquid/air surface tension of trisiloxane solutions reaches the minimum value at the critical aggregation concentration (CAC) (the concentration at which various aggregates in aqueous solutions appear and remain at equilibrium with monomers). However, superspreading or complete wetting is observed at the critical wetting concentration (CWC), which is several times higher than the CAC.3,9 Using AFM, Svitova et al.3 have found a long-time multilayered adsorption of trisiloxanes on a hydrophobic solid substrate (graphite) at/above CWC, whilst between CAC and CWC only a monolayer or hemispherical micelles has been observed. Bezuglyi et al.10 confirmed using FTIR that the adsorption of trisiloxanes on moderately hydrophobic surfaces sharply increases at C ≥ CWC in comparison with CAC. Moreover, using Brewster angle microscopy, Ritacco et al.5 detected surface aggregates on the liquid/air interfaces at C > CAC for the trisiloxanes which show superspreading on hydrophobic surfaces, while no aggregates were detected for the other trisiloxanes. It has been hypothesized5 that those surface aggregates may act as reservoirs of surfactant molecules to maintain the required low surface tension in the course of spreading.
Below, the influence of the concentration of trisiloxane surfactants on disjoining pressure isotherms and the dynamics of a wetting film of superspreader trisiloxane Silwet L-77 are investigated.
A commercial organosilicon surfactant Silwet L-77 (Momentive, Germany) was used without any further purification. Solutions of Silwet L-77 at concentrations ranging from C < CAC through C > CWC (where CAC is 0.007%3,9 and CWC is 0.025%3,9) were prepared using 10−4 mol l−1 KCl aqueous solutions. According to the manufacturer the surface tension of the aqueous Silwet L-77 solution is 20.5 mN m−1.
Glass slides were hydrophobized according to the following procedure. The slides were soaked in concentrated NH4OH solution for one hour and then thoroughly rinsed in pure water (18.2 MΩ·cm, Millipore). After that the cleaned glass slides were dried for one hour at 140 °C and then the slides were silanized by placing them in a 5 mM solution of trimethoxy(octadecyl)silane (90%, Sigma-Aldrich) in heptane (≥99%, Sigma-Aldrich) for 24 hours. The silanized glass slides were washed with heptane to remove unreacted materials, and then were heated for one hour at 70 °C. The slides were stored in methanol before use. The surface roughness of the silanized glass slides measured by AFM was found to be 1.9 ± 0.2 nm. The advancing contact angle of pure water on the slides at the humidity of 95% was, θw, 98 ± 1°.
An experimental setup for studying the film formation and the measurement of the disjoining pressure isotherms is shown in Fig. 1. The setup consists of an interference microscope and the cell with the hydrophobized glass slide on the top, connected by a plastic tube to a vessel. The wetting films were formed when a liquid meniscus in a cylindrical titanium capillary of inner radius of rc = 0.575 mm approached the hydrophobic surface of the slide. The distance between the capillary edge and the glass slide was around 0.2 mm. Upon changing the distance H (Fig. 1) between the level of solution in the vessel and the hydrophobic surface, the position of the liquid meniscus can be varied, which allows variation in the capillary pressure inside the wetting film. The capillary pressure in this system was calculated according to the following equation:11,12
|
Pc = 2γrc/(rc2 − rf2), | (1) |
where
rc is the radius of the capillary,
rf is the radius of the wetting film,
γ is the surface tension of the trisiloxane solution. Let us rewrite the this equation as
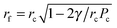
. That is, the film radius,
rf, is equal to zero at
Pc = 2
γ/
rc (at this pressure the meniscus touches the solid support) and then the radius of the film increases as
Pc increases in a non-linear way and tends to
rc as
Pc tends to infinity.
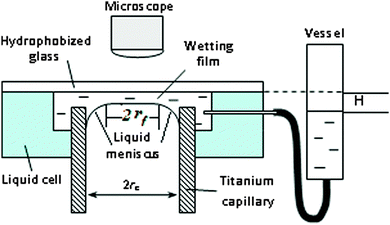 |
| Fig. 1 Diagram of experimental cell for the wetting film formation/measurements. | |
The equation for the disjoining pressure acting in the liquid film is Π(h) = Pc + Pg, or taking into account eqn (1):
|
Π(h) = 2γrc/(rc2 − rf2) + Pg, | (2) |
where
Pg =
ρgH is the hydrostatic pressure,
ρ is the density of the liquid, and
g is the gravity acceleration. According to
eqn (2) the disjoining pressure decreases when the film radius,
rf, decreases. According to
Fig. 4 the latter means that the smaller the radius of the film,
rf, the thicker the film becomes.
The film thickness and its dynamics were determined from an interference image formed by monochromatic light (λ = 715 nm) reflected from the wetting film. The film thickness was calculated from the intensities of reflected light using the following equation:12
|
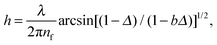 | (3) |
where
Δ = (
If −
Imin)/(
Imax −
Imin),
b = (
nf2 − 1)(
ns2 −
nf2)/
nf2(
ns + 1)
2,
If is an intensity of the reflected light from the film,
Imax and
Imin are the intensities of the first maximum and minimum of interference,
nf and
ns are the refractive indices of the liquid in the film (assuming that it is equal to that of the bulk solution,
nf = 1.33) and the solid substrates (the glass
ns = 1.51), respectively. At these values of
nf and
ns, the value of
b ∼ 0.0353 ≪ 1. According to
eqn (3)If(
h) reaches its maximum value
Imax at
h = 0.
Direct differentiation of dependency If(h) according to eqn (3) results in
|
 | (4) |
This means that the thicker the film is, the darker image will be, or the dark spots on the film show the presence of thicker spots.
It was allowed to equilibrate for not less than 5 minutes before the measurement of the film thickness.
Separately, the time evolutions of the advancing contact angle of droplets of aqueous Silwet L-77 solutions on the hydrophobized glass slides at different concentrations were measured by using the goniometry technique at T = 24 ± 1° and the humidity of 95% to prevent evaporation of droplets.
Wetting and spreading kinetics results
Before film formation and the measurement of the disjoining pressure, the kinetics of the spreading of trisiloxane solutions was investigated on the hydrophobized glass slides. The concentration range of Silwet L-77 was from 0.002 (below CAC) to 0.1 wt% (above CWC). In Fig. 2 the time evolution of the apparent advancing contact angles of Silwet L-77 solutions on the hydrophobized glass slides is shown. According to Fig. 2, the solutions of Silwet L-77 in the studied concentration range demonstrate either partial or complete wetting of the hydrophobized glass depending on the concentration. The superspreading behavior on these substrates was observed only at C ≥ CWC. Note that in this case the droplets did not spread out to a very thin layer (e.g. a monolayer) in the course of superspreading but to a layer of a finite thickness of about a few microns. At the lowest concentration (0.002 wt%, which is below CAC), a time lag of spreading of about 5 s was detected. This time lag is related to the slow adsorption kinetics of the surfactant molecules on both the liquid/air and the solid/liquid interfaces at C < CAC.13 The trends observed (Fig. 2) are in agreement with our previous findings on the spreading of trisiloxane solutions on hydrophobic polymer films.9
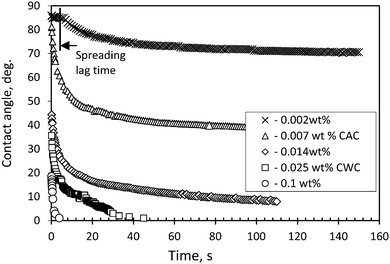 |
| Fig. 2 Time evolution of advancing contact angle of aqueous solutions of Silwet L-77 on silanized glass slides at different surfactant concentrations. | |
Disjoining pressure isotherms
It was found that at C = 0.002 wt% (below CAC) no wetting film was detected on the silanized glass either immediately after the cell was filled with the surfactant solution or in almost half an hour after the solution was kept in the cell. Note that 30 minutes is enough for the adsorption of molecules at the interface to form a wetting film according to the data on dynamic surface tension at the liquid/air interface13 and the data on spreading kinetics (Fig. 2). At C = 0.004 wt% (still below CAC) and C = 0.007 wt% (equal to CAC), an appearance of the wetting films with dimples was observed. However, the films ruptured immediately after the dimples began to disappear (Fig. 3). This process (the formation and rupture of films) occurred randomly, hence, there was no possibility to measure the film thickness and the pressures of the rupture. In the case of 0.004 wt% concentration, the film rupture resulted in an almost dry surface of silanized glass (see Fig. 3a). However, at C = 0.007 wt% (which is equal to CAC), small residual droplets remained on the surface, which meant the transition from a non-wetting to a partial wetting of the surface (Fig. 3b). At high concentrations in between CAC < C < CWC and at C ≥ CWC, different types of aggregates (lamellas, vesicles and others) exist in the solutions. In these cases thick films were formed in our experiments on the silanized glass slides. In Fig. 4 the disjoining pressure isotherms of the films at different (0.01, 0.015, 0.025 and 0.1 wt%) concentrations obtained for Silwet L-77 in our experiments and data from Churaev et al.7 obtained for the thick wetting film of trisiloxane D-8 at C = 0.16 wt% are presented. Note that our experimental conditions allowed the measurements of the thickness of the wetting film only in a relatively narrow range of variation of the capillary pressure: increasing the pressure over the limit resulted in the condition If < Imin, at which eqn (3) is not valid any more. According to Fig. 4 the thickness of the wetting film decreases significantly with an increase in the disjoining pressure, while an influence of the concentration within the studied range of concentrations is almost undistinguishable. Note that our data and the data from Churaev et al.7 show a common trend.
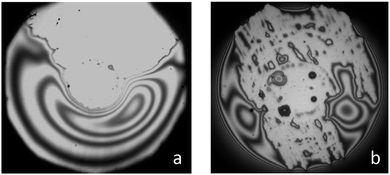 |
| Fig. 3 Images of the film ruptures in solutions of Silwet L-77 at concentrations: (a) 0.004 wt% and (b) 0.007 wt%. | |
It is important to note that the wetting films did not rupture when the hydrostatic pressure was significantly increased. Taking into account the presence of aggregates in the studied solutions, it is possible to assume that the wetting films were stabilized by the long-range structural forces between the aggregates.7Fig. 4 also shows that the more multilayers are formed the lower the disjoining pressure becomes.
Multilayered films: dark spots
At concentration C = 0.1%, which is above CWC, within the stable wetting film in few minutes (∼5 min) after the film was formed, the appearance of dark spots was observed, Fig. 5(a). The area of these dark spots expanded continuously and new spots appeared in the film in a multilayer manner: each new spot was darker than the previous one, Fig. 5(a). The increase in the quantity of the dark spots and their expansion was followed by the formation of “channels” from the dark spots to the border of the film Fig. 5(a). After the appearance of these “channels” the film began to shrink and disappeared in the end. This means that Pc according to eqn (1) began to decrease. The evolution of the diameter of the wetting film is shown in Fig. 5(b), which shows that the formation and growth of the dark spots did not change substantially the film diameter, until the appearance of the “channels”. It proves that the channels served as a way to solution flow from the surrounding meniscus to the wetting film.
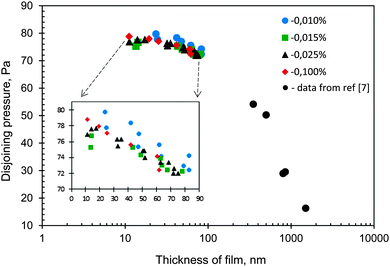 |
| Fig. 4 Disjoining pressure isotherms of films at different concentrations of Silwet L-77 and data adopted from Churaev et al.7 for trisiloxane with a similar number of ethylene oxide units at concentration 0.16%. | |
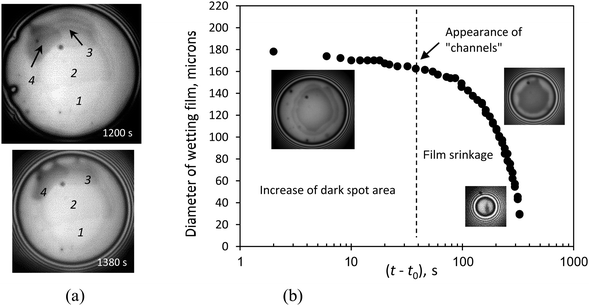 |
| Fig. 5 (a) Long-time multilayer films structuring within the wetting film at concentration 0.1 wt%. 1, 2, 3, and 4: the dark spots appeared in the film. Spots 3 and 4 are darker in comparison with spots 1 and 2. (b) Diameter of the wetting film as a function of time. Initial thickness of the film is 60 nm (t0 ≅ 600 s). Concentration of the solution is 0.1 wt%. | |
Note that the appearance of dark spots in the free liquid films (similar to that in foam films) has been intensively studied for a long time.11,14,15 The appearance of the dark spots is actually a demonstration of the stepwise thinning of the films.14,15 It means that the films exhibited a number of metastable equilibrium states until a final thinner equilibrium state was reached.
However, in our case the black spots meant not thinner but thicker parts. After a number of metastable equilibria were reached, the wetting film became very thick and eventually converted into a part of the surrounding meniscus. We assume that this behavior is related to some long-range structural transformation processes occurring within the film at C ≥ CWC: trisiloxane molecules in a stepwise manner form multilayer structures (for instance, bilayers) above the monolayer adsorbed at interfaces. Svitova et al.3 observed a long-time multilayer adsorption of trisiloxanes at C ≥ CWC on a graphite surface under spreading droplets. Later, a considerable increase in the adsorption at the hydrophobic solid/liquid interface at C ≥ CWC was found by Bezuglyi et al.10
An increase in the film thickness leads to the shrinkage of the film radius and its disappearance. The measurement of If for two subsequent dark spots within the wetting film and using eqn (2) allowed the estimation of the difference in thickness between two subsequent dark spots, which turns out to be within the range of 7–9 nm. According to Kunieda et al.6 for trisiloxane surfactants in the Lα phase (where the bilayers and/or the lamellar aggregates are present) the interlayer spacing ranged from 6 to 10 nm, which in a good agreement with our estimations of the interlayer spacing.
Conclusion
It was shown that wetting films do not form at concentrations below the critical wetting concentration (CAC) but form at higher concentrations. At concentrations at/above the CWC in equilibrium with the wetting films of aqueous solutions of Silwet L-77 on silanized glass, spontaneous formation of multilayered spots of thicker films occurred. The multilayer adsorption of trisiloxane bilayer aggregates within the wetting film at C ≥ CWC is a possible reason for the observed behavior. Indeed, the interlayer thickness estimated for each black film corresponds to the interlayer spacing for the bilayers formed by trisiloxane molecules. The multilayer adsorption of trisiloxane aggregates increases the thickness of the wetting film, which leads to the film shrinkage and the final disappearance.
Acknowledgements
This research was supported by the Royal Society UK–Russia collaboration grant, European Space Agency EVAPORATION MAP project, CoWet EU project and COST MP1106 EU project; Engineering and Physical Research Council, UK (EP/J010596/1). N. Ivanova was supported by the RFBR (Grant no. 14-01-92602 KO_a) and the Ministry of Education and Science of the Russian Federation, the program of The Organization of Scientific Research.
Notes and references
-
R. M. Hill, Silicone surfactants, Marcel Drekker, 1999 Search PubMed
.
- J. Venzmer, Curr. Opin. Colloid Interface Sci., 2011, 16, 335 CrossRef CAS
.
- T. Svitova, R. M. Hill and C. J. Radke, Colloids Surf., A, 2001, 183–185, 607 CrossRef CAS
.
- N. Kumar, A. Couzis and Ch. Maldarelli, J. Colloid Interface Sci., 2003, 267, 272 CrossRef CAS PubMed
.
- H. A. Ritacco, F. Ortega, R. G. Rubio, N. Ivanova and V. M. Starov, Colloids Surf., A, 2010, 365(1–3), 199 CrossRef CAS
.
- H. Kunieda, H. Taoka, T. Iwanaga and A. Harashima, Langmuir, 1998, 14, 5113 CrossRef CAS
.
- N. V. Churaev, N. E. Esipova, R. M. Hill, V. D. Sobolev, V. M. Starov and Z. M. Zorin, Langmuir, 2001, 17, 1338 CrossRef CAS
.
- S. Sett, R. P. Sahu, S. Sinha-Ray and A. L. Yarin, Langmuir, 2014, 30, 2619 CrossRef CAS PubMed
.
- N. Ivanova, V. Starov, R. Rubio, H. Ritacco, N. Hilal and D. Johnson, Colloids Surf., A, 2010, 354, 143 CrossRef CAS
.
- B. A. Bezuglyi, N. A. Ivanova and V. M. Starov, Prog. Colloid Polym. Sci., 2011, 138, 121 CAS
.
- A. Nikolov and D. Wasan, Adv. Colloid Interface Sci., 2014, 206, 207 CrossRef CAS PubMed
.
- A. Sheludko, Adv. Colloid Interface Sci., 1967, 1, 391 CrossRef CAS
.
- F. Han, Y. H. Chen, Y. Zhou and B. Xu, J. Dispersion Sci. Technol., 2012, 33, 396 CrossRef CAS
.
- P. A. Kralchevsky, A. D. Nikolov, D. T. Wasan and I. B. Ivanov, Langmuir, 1990, 6, 1180 CrossRef
.
- A. D. Nikolov and D. T. Wasan, Langmuir, 1992, 8, 2985 CrossRef CAS
.
|
This journal is © The Royal Society of Chemistry 2016 |
Click here to see how this site uses Cookies. View our privacy policy here.