DOI:
10.1039/C6SC00972G
(Edge Article)
Chem. Sci., 2016,
7, 5983-5988
A regenerative metal–organic framework for reversible uptake of Cd(II): from effective adsorption to in situ detection†
Received
2nd March 2016
, Accepted 24th May 2016
First published on 25th May 2016
Abstract
An extraordinary metal–organic framework FJI-H9 with high adsorption and selectivity for the reversible uptake of Cd(II) has been developed. Further research indicates that such high absorption results from an unusual synergy from active sites and the confined cavity. In addition, fast detection of Cd(II) at low concentrations down to 10 ppm and in situ reconstruction of the used framework into a fresh one have also been successfully achieved.
Introduction
Heavy metal pollution which can cause brain damage and disease in humans and other species has become a serious threat to environment. For example, cadmium is known to directly damage the nervous, reproductive, renal and skeletal systems, and can also cause some cancers.1 Therefore, how to remove cadmium effectively from industrial processes is highly urgent. Many sorbent materials, such as carbon materials,2 biomass,3 nanoparticles4 and chelating polymers5 have been extensively investigated for the adsorptive removal of cadmium; however those sorbents face several challenges, such as low uptake and moderate affinity for Cd(II).
Metal–organic frameworks (MOFs) are a new type porous material composed of organic bridging ligands and metal ions or metal ion clusters, possessing high porosities and ready tenability.6 Many applications based on MOFs including gas storage,7 separation8 and catalysis9 have been developed. Recently, MOFs have been used as solid-phase sorbents to remove heavy metals by the introduction of suitable functional groups.10 So far, many excellent sorbents for Hg(II) based on MOFs or COFs have been developed based on strong Hg–S interactions.10a,e,i However, the exploration of MOFs for Cd(II) removal is highly challenging, and only two examples with moderate capacity have been reported.10m,j Herein, a novel metal–organic framework FJI-H9 for the reversible uptake of Cd(II) has been developed, and the adsorption of Cd(II) achieved is up to 286 mg g−1. Furthermore, fast detection of Cd(II) at low concentrations down to 10 ppm and the in situ reconstruction of the used framework into a fresh one have also been successfully carried out (Scheme 1).
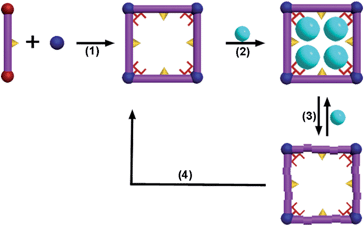 |
| Scheme 1 A regenerative MOF for reversible uptake of Cd(II): (1) self-assembly 3D framework from organic ligand and hard metal ion; (2) effective absorption with high capacity; (3) absorption and desorption; (4) reconstruction of the used sample into a fresh sample. | |
Results and discussion
Syntheses and structures of FJI-H9
Using organic donors composed of carboxylic and thiophenol with hard metal ions has proved to be a powerful strategy in the construction of porous frameworks for the adsorption of Hg(II).10e So we tried to react 2,5-thiophenedicarboxylate (H2thb), composed of two carboxylic groups and one thiophenyl, with hard metal ions to obtain a MOF framework for the adsorption of Cd(II). Herein, a novel MOF FJI-H9, formulated as [Me2NH2+][Ca2(thb2−)2(CH3COO−)(DMA)]·DMA has been synthesized by reacting CaCl2 with 2,5-thiophenedicarboxylate. Single crystal X-ray analysis reveals that FJI-H9 crystallizes in the orthorhombic space group Pca21, its asymmetric unit contains two calcium ions, two thb2− ligands, one coordinated CH3COO− and a DMA molecule, as well as one dissociative DMA molecule and one [Me2NH2]+ ion. The Ca(1) atom is coordinated by seven oxygen atoms, in which four oxygen atoms from four thb2− ligands, two oxygen atoms from one CH3COO− molecule and one oxygen atom from the DMA molecule (Ca–O, 2.292–2.647 Å). Meanwhile the Ca(2) atom is coordinated by eight oxygen atoms, with six oxygen atoms from four thb2− molecules and two oxygen atoms from two CH3COO− molecules (Ca–O, 2.292–2.647 Å). As shown in Fig. 1b, a series of 1D channels (11.349 × 11.235 Å) based on Ca(II) and the ligand array along the a axis in the framework of FJI-H9, in which sulfur-functions highlighted as yellow balls remain free-standing, both the coordinated DMA molecules and free DMA molecules stack in its cavity orderly .
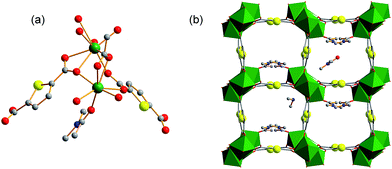 |
| Fig. 1 (a) The coordination environment of FJI-H9, (C = grey, N = blue, O = red, S = yellow, Ca = green, H was omitted for clarity); (b) the 3D framework of FJI-H9 (sulfur atoms are highlighted as yellow balls, Ca atoms are highlighted as green polyhedra). | |
Heavy metal ion adsorption test of FJI-H9
Both the free and coordinated DMA molecules are occupied in the channels of FJI-H9, which may highly improve the adsorption of Cd(II). Thus, fresh FJI-H9 without activation has been used to adsorb heavy metal ions directly. The as-made fresh crystals of FJI-H9 (28 mg) were directly soaked in 25 mL of a 0.1 mol L−1 acetonitrile solution of Cd(NO3)2. The solid was then isolated further and washed with acetonitrile to remove the residual Cd(NO3)2 on the exterior of the FJI-H9 powder. The solid sample thus obtained (denoted as FJI-H9-Cd) was subjected to regular CHN, inductively coupled plasma (ICP) elemental and thermogravimetry-mass spectrometry analyses. ICP analysis determined the Cd/Ca ratio to be 2.5
:
1 (Table 1), TG-MS analysis demonstrates that there are about 15.5% volatile solvents which contain H2O and DMA molecules. All of these lead to a composition of (Cd2+)0.5Ca2(tda2−)2(CH3COO−)(DMA)1.2·[Cd(NO3)2]1.28·(H2O)7.1, (calculated: [C (22.87%), H (3.10%), N (5.66%)]; found: [C (22.95%), H (3.38%), N (5.35%)]). This demonstrates that 1 mol FJI-H9 can adsorb 1.78 mol Cd(II), and the adsorption of Cd(II) is up to 286 mg g−1. However, the saturated adsorption based on the adsorption isotherm is more practical in the application of decontaminating heavy metal ions; so adsorption isotherm for Cd(II) removal from acetonitrile was carried out, and the maximum Cd(II) adsorption capacity of FJI-H9 was found to be about 225 mg g−1 (ESI, Fig. S5†). Further research demonstrated that only 0.13 mol Hg are absorbed by per mol FJI-H9. In contrast, other metal ions such as Mg(II), Co(II), Ni(II), Mn(II), Zn(II), Fe(II) and Pb(II) cannot be absorbed by FJI-H9. The activation sites for the adsorption of Hg(II) may be these thiophenyl groups, so Hg is only moderately absorbed. It is highly interesting that Cd(II) can be highly adsorbed while Hg(II) is only moderately adsorbed. In order to further investigate the Cd(II) absorption character of FJI-H9, many other necessary tests such as extended X-ray absorption fine structure (EXAFS) and infrared radiation (IR) have been carried out (Fig. 2). EXAFS analysis shows that there is no Cd–S interaction in the FJI-H9-Cd powder, indicating that the Cd atoms do not bond with S atoms from the thb2− ligands (Fig. 2a). Further quantitative EXAFS curve-fitting demonstrates that there are three different kinds of Cd–O interactions around Cd atoms in the FJI-H9-Cd powder, including four Cd–O1 (R = 2.26 Å), two Cd–O2 (R = 2.39 Å) and two Cd–O3 (R = 2.54 Å) (Table 2). IR analysis implies that the strong N–H (Vs = 3360 cm−1) spectra from [Me2NH2]+ ion disappears while another strong hydrogen interaction (Vs = 3310 cm−1, 3471 cm−1) is formed. So the high adsorption of Cd(II) may result from the following: (1) electronegative framework is beneficial to adsorption of Cd(II), where isolated [Me2NH2]+ can be replaced by Cd2+, which can be confirmed by the EA analysis and IR analysis (as shown in Fig. 2, the characteristic spectrum of [Me2NH2]+ ion has disappeared); (2) this-sized square cavity of FJI-H9 may be very suitable for the closing pack of Cd(II), and a suitable cavity may play the most important role for the adsorption of metal ions here. Both the EXAFS analysis [four Cd–O1 (R = 2.26 Å)] and EA analysis (the ration of Cd/H2O = 1
:
4) imply the existence of Cd(H2O)42+. Another two different Cd–O interactions [Cd–O2 (R = 2.39 Å), Cd–O3 (R = 2.54 Å)] may indicate that NO3− anions are stacked around Cd(H2O)42+ closely, which is also confirmed by the new-formed strong hydrogen interaction (Vs = 3310 cm−1, 3471 cm−1). (3) The activated sites for adsorbing Cd(II) may be these coordinated DMA molecules rather than the thiophenyl, in which the Cd(H2O)42+ ions are immobilized by DMA molecules through strong hydrogen bond. In summary, the high adsorption of Cd(II) of FJI-H9 results from an unusual synergy from the active sites and confined cavity in Scheme 2(a).
Table 1 The weight ratios of M/Ca in FJI-H9
|
Cd2+ |
Hg2+ |
Ni2+ |
Mn2+ |
Zn2+ |
Fe2+ |
Pb2+ |
Co2+ |
Mg2+ |
M/Ca |
2.50 |
0.34 |
0.08 |
0.07 |
0.06 |
0.07 |
0.009 |
0.07 |
0.03 |
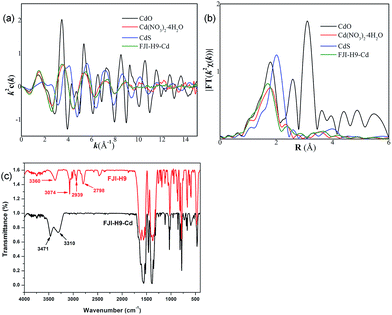 |
| Fig. 2 (a) Cd K-edge extended XAFS oscillation function k2χ(k); (b) the corresponding Fourier transforms FT(k3w(k)); (c) the IR spectrum of FJI-H9 and FJI-H9-Cd (red = FJI-H9, black = FJI-H9-Cd), Vs = 3360 cm−1 belongs to NH, Vs = 3074 cm−1, 2939 cm−1, 2798 cm−1 belong to CH3 from [Me2NH2]+ and DMA molecules. | |
Table 2 Structural parameters extracted from quantitative EXAFS curve-fitting
Sample |
Path |
N
|
R (Å) |
σ
2 (10−3 Å2) |
ΔE0 (eV) |
CdO |
Cd–O |
6.0 |
2.34 |
3.8 |
3.0 |
CdS |
Cd–S |
4.0 |
2.52 |
3.3 |
4.8 |
Cd–O1 |
4.0 |
2.31 |
5.6 |
5.0 |
Cd(NO3)2·4H2O |
Cd–O2 |
2.0 |
2.45 |
6.2 |
5.0 |
Cd–O3 |
2.0 |
2.59 |
7.5 |
5.0 |
Cd–O1 |
4.0 |
2.26 |
4.8 |
3.8 |
FJI-H9-Cd
|
Cd–O2 |
2.0 |
2.39 |
5.3 |
3.8 |
Cd–O3 |
2.0 |
2.54 |
6.5 |
3.8 |
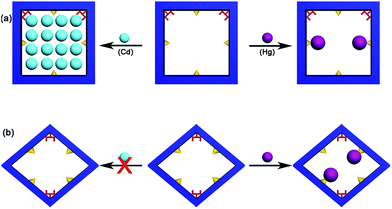 |
| Scheme 2 Possible adsorption mechanisms: (a) with help by DMA molecules highlighted as red fork and confined cavity, the Cd(II) ions close-pack in the square channel of FJI-H9; only part of the thiophenyl groups highlighted as yellow triangles from FJI-H9 adsorb Hg(II). (b) Nearly no Cd(II) is absorbed by FJI-H10, only part of the thiophenyl groups highlighted as yellow triangles from FJI-H10 adsorb Hg(II). | |
The selective adsorption of FJI-H9 under background metal ions
Selectivity tests were also performed on FJI-H9 in a mixed solution containing Cd(II), Hg(II), Ca(II), Mg(II), Co(II), Ni(II), Mn(II), Zn(II), Fe(II) and Pb(II) ions. As shown in Fig. 3, FJI-H9 can remain effective in the presence of high concentrations of these background metal ions, Cd(II) ions can be effectively adsorbed while Hg(II) can be moderately adsorbed. In contrast, other background metal ions such as Ca(II), Mg(II), Co(II), Ni(II), Mn(II), Zn(II), Fe(II) and Pb(II) do not quite bind to FJI-H9.
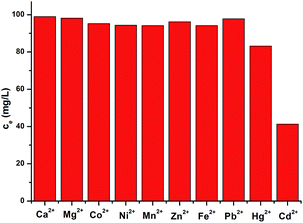 |
| Fig. 3 The selective adsorption of Cd(II) by FJI-H9. | |
In order to prove the importance of the suitable cavity for adsorption of Cd(II), another framework FJI-H10 formulated as [Ca(thb2−)(DMA)(H2O)] was also prepared from CaCl2 and 2,5-thiophenedicarboxylate. Single crystal X-ray analysis indicates that FJI-H10 crystallizes in the monoclinic space group P21/n, and the asymmetric unit contains one calcium atom, one thb2− ligand, one coordinated DMA and H2O molecule. The Ca(II) atom is coordinated by six oxygen atoms and exhibits a distorted octahedron coordination geometry, with four oxygen atoms from four different thb2− ligands, one oxygen atom from a H2O molecule and one oxygen atom from a DMA molecule (Ca–O, 2.289–2.434 Å). Compared with FJI-H9, FJI-H10 also has coordinated DMA molecules and free thiophenyl, herein the cavity is rhombic (11.744 × 11.648 Å) rather than square (Fig. 4). Further research demonstrates that about 0.11 mol Hg(II) are absorbed by per mol FJI-H10, and nearly no Cd(II) can be absorbed by FJI-H10 (Table S4,†Scheme 2b). Moderate adsorption of Hg(II) from FJI-H10 also may result from thiophenyl groups. It is highly interesting that nearly no Cd(II) can be captured by FJI-H10, which may further prove that a high adsorption of Cd(II) by FJI-H9 indeed results from an unusual synergy from active sites and a confined cavity (Table 3).
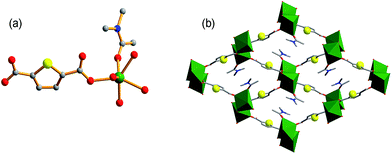 |
| Fig. 4 (a) Coordination environment of FJI-H10, (C = grey, N = blue, O = red, S = yellow, Ca = green, H was omitted for clarity); (b) the 3D framework of FJI-H10 (sulfur atoms highlighted as yellow balls, Ca atoms highlighted as green polyhedra). | |
Table 3 Comparison of cadmium adsorption performances by various adsorbents
Sorbent |
Adsorption capacity (mg g−1) |
Directly obtain from the solid ICP test.
Obtain from the adsorption isotherm.
|
FJI-H9 (MOF) |
286a/225b |
Mg-MTMS5e |
210b |
Manganese dioxide formed in situ4d |
176b |
PCN-100 (ref. 10m) (MOF) |
147a |
ZrO2/B2O3 (ref. 4c) |
109.9b |
Cu3(BTC)2-SO3H10j (MOF) |
88.7b |
MPGI5d |
87.7b |
Sulfonic-functionalized poly(dimethylsiloxane) networks5a |
78.7b |
Magnetic yeast treated with EDTA dianhydride4b |
48.7b |
Salicylic acid type chelate5b |
45.0b |
PPBM3 |
43.5b |
Si-DTC5c |
40.5b |
Fe3O4@APS@AA-co-CA4a |
29.6b |
Desorption and resorption of Cd(NO3)2 on FJI-H9
FJI-H9 is an effective adsorbent for Cd(II), which represents a new record for adsorption of Cd(II). Can this effective adsorbent be reused? In order to remove the Cd(II) adsorbed in FJI-H9-Cd powder, ethylenediamine (EDA), which has more strong interactions with Cd(II), has been selected (Scheme 3). Further researches indicate that about 99.9% of Cd(NO3)2 can be removed from FJI-H9-Cd powder with the help of EDA (ESI†). The used FJI-H9 framework was re-used to adsorb Cd(NO3)2 once again, and the ICP reveals the weight ratio of Cd/Ca reduce to 2.03
:
1, indicating that the efficiency of FJI-H9 was little reduced after multiple usages. Powder XRD analyses demonstrate that the main framework of FJI-H9 remains stable after loading and reloading (Fig. 5).
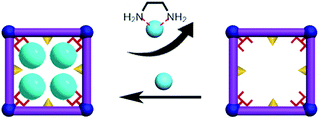 |
| Scheme 3 Desorption and resorption of Cd(NO3)2 on FJI-H9. | |
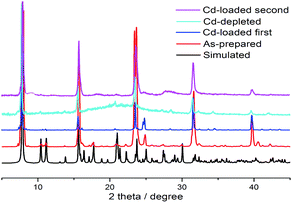 |
| Fig. 5 Powder X-ray diffraction analyses. Black: a simulation sample of FJI-H9; red: a synthesized sample of FJI-H9; blue: a first Cd(NO3)2-loaded sample of FJI-H9; light blue: a Cd(NO3)2-depleted sample of FJI-H9-Cd; purple: a second Cd(NO3)2-loaded sample of FJI-H9. | |
Reconstruction of the used FJI-H9
Although FJI-H9 is reusable, its adsorption capacity is reduced after multiple usages. Can the used FJI-H9 be reconstructed into a fresh one? If so, it will greatly reduce the costs and improve efficiency. Based on the reversibility of the coordination bond, reconstruction of the used framework into a fresh framework is possible. After many attempts, we finally find that the used FJI-H9 powder can be in situ reconstructed into a fresh FJI-H9 crystal as follows: the 10 mg used FJI-H9 powder and 50 uL HNO3 (about 16 mol L−1) were added to 5 mL mother liquor which had been used to prepare fresh FJI-H9. Heating such a solution at 85 °C for six days leads to 9 mg of fresh FJI-H9 crystals. The cost of remove Cd(II) is highly reduced through the effective regeneration of FJI-H9, which may provide a new strategy to develop more economical adsorbents for heavy metal ions. As far as we know, reconstruction of used adsorbents into fresh ones has not been reported until now (Scheme 4).
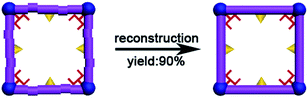 |
| Scheme 4
In situ reconstruction of the used framework into fresh framework. | |
Fluorescence indicator
The literature shows that MOFs as heavy metal adsorbents also can effectively detect heavy metal ions, but such a process usually needs long times or high concentrations of heavy metal ions.10 However, the industrial cadmium pollution level is only 10 ppm in general, so a more effective indicator for heavy metal ions should be developed. We have found that FJI-H9 is a good adsorbent for Cd(II), and it also can be reused and in situ regenerated. Can such a material in situ detect Cd(II) more effectively and quickly? For this purpose, FJI-H9 was added to a Cd(NO3)2 solution of different concentration. As shown in Fig. 6, the intensity slightly reduced when the concentration gradually increased from 10 ppm to 200 ppm. Interestingly, even soaking FJI-H9 in the 10 ppm solution of Cd(II) for 10 minutes can also highly quench its emission (λem = 460 nm), in which 92% of the total fluorescence intensity of FJI-H9 can be quenched. The quenched fluorescence in Fig. 6 likely originates from the interaction between the coordinated DMA molecules and Cd(II) ions. Such quick and effective fluorescence quenching may result from the fact that so many coordinated DMA molecules and free DMA molecules are stacked in the 1D channels of the FJI-H9 framework, which make intermolecular energy transfer between the FJI-H9 framework and Cd(II) ions more easily. In contrast, for other metal ions such as Hg(II), Mg(II), Co(II), Ni(II), Mn(II), Zn(II), Fe(II) and Pb(II), no such obvious fluorescence quenching can be observed under the same conditions. To the best of our knowledge, using MOFs to detect Cd(II) ions in such an effective way has not been reported previously.
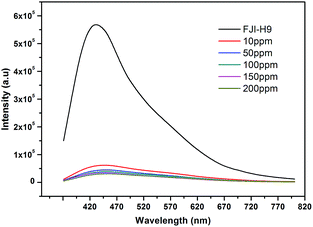 |
| Fig. 6 Rapid detection of cadmium with low concentration. | |
Conclusions
An exceptional MOF FJI-H9 for reversible uptake of Cd(II) with high absorption and selectivity has been obtained successfully, and in situ reconstruction of the used FJI-H9 framework into a fresh one also has been achieved. Furthermore, the quick detection of Cd(II) at low concentrations down to 10 ppm also has been realized for the first time. All of these characters of FJI-H9, which contains high adsorption, regeneration, and quick detection, will provide a new strategy to prepare more effective adsorbents to remove and detect heavy metal ions.
Acknowledgements
This work was supported by the 973 Program (2014CB932101, 2013CB933200), the National Natural Science Foundation of China (21471148, 21131006, 21390392) and “Strategic Priority Research Program” of the Chinese Academy of Sciences (XDB20000000, XDA09030102). We also thank Professor Shiqiang Wei and Professor Shuhong Yu, from National Synchrotron Radiation Laboratory, University of Science and Technology of China, for the EXAFS analysis.
Notes and references
-
(a) N. Lubick and D. Malakoff, Science, 2013, 341, 1443 CrossRef CAS PubMed;
(b) M. McNutt, Science, 2013, 341, 1430 CrossRef CAS PubMed.
-
(a) A. Duran, M. Tuzen and M. Soylak, J. Hazard. Mater., 2009, 169, 466 CrossRef CAS PubMed;
(b) M. Tuzen, K. O. Saygi and M. Soylak, J. Hazard. Mater., 2008, 152, 632 CrossRef CAS PubMed.
- A. Dubey, A. Mishra and S. Singhal, Int. J. Environ. Sci. Technol., 2014, 11, 1043 CrossRef CAS.
-
(a) F. Ge, M.-M. Li, H. Ye and B.-X. Zhao, J. Hazard. Mater., 2012, 211, 366 CrossRef PubMed;
(b) M. Xu, Y. Zhang, Z. Zhang, Y. Shen, M. Zhao and G. Pan, Chem. Eng. J., 2011, 168, 737 CrossRef CAS;
(c) Ö. Yalçınkaya, O. M. Kalfa and A. R. Türker, J. Hazard. Mater., 2011, 195, 332 CrossRef PubMed;
(d) Q. Qin, Q. Wang, D. Fu and J. Ma, Chem. Eng. J., 2011, 172, 68 CrossRef CAS.
-
(a) F. A. Silva and F. L. Pissetti, J. Colloid Interface Sci., 2014, 416, 95 CrossRef CAS PubMed;
(b) F. An, B. Gao, X. Dai, M. Wang and X. Wang, J. Hazard. Mater., 2011, 192, 956 CrossRef CAS PubMed;
(c) L. Bai, H. Hu, W. Fu, J. Wan, X. Cheng, L. Zhuge, L. Xiong and Q. Chen, J. Hazard. Mater., 2011, 195, 261 CrossRef CAS PubMed;
(d) C.-Y. Chen, C.-L. Chiang and P.-C. Huang, Sep. Purif. Technol., 2006, 50, 15 CrossRef CAS;
(e) I. L. Lagadic, M. K. Mitchell and B. D. Payne, Environ. Sci. Technol., 2001, 35, 984 CrossRef CAS PubMed.
-
(a) O. K. Farha and J. T. Hupp, Acc. Chem. Res., 2010, 43, 1166 CrossRef CAS PubMed;
(b) N. W. Ockwig, O. Delgado-Friedrichs, M. O'Keeffe and O. M. Yaghi, Acc. Chem. Res., 2005, 38, 176 CrossRef CAS PubMed;
(c) D. Zhao, D. J. Timmons, D. Yuan and H.-C. Zhou, Acc. Chem. Res., 2011, 44, 123 CrossRef CAS PubMed;
(d) J. J. I. V. Perry, J. A. Perman and M. J. Zaworotko, Chem. Soc. Rev., 2009, 38, 1400 RSC;
(e) S. Kitagawa and R. Matsuda, Coord. Chem. Rev., 2007, 251, 2490 CrossRef CAS;
(f) L. Chen, Q. Chen, M. Wu, F. Jiang and M. Hong, Acc. Chem. Res., 2015, 48, 201 CrossRef CAS PubMed;
(g) G. Ferey, Chem. Soc. Rev., 2008, 37, 191 RSC;
(h) D. J. Tranchemontagne, J. L. Mendoza-Cortes, M. O'Keeffe and O. M. Yaghi, Chem. Soc. Rev., 2009, 38, 1257 RSC;
(i) Y. Cui, Y. Yue, G. Qian and B. Chen, Chem. Rev., 2012, 112, 1126 CrossRef CAS PubMed;
(j) S. L. James, Chem. Soc. Rev., 2003, 32, 276 RSC;
(k) N. C. Burtch, H. Jasuja and K. S. Walton, Chem. Rev., 2014, 114, 10575 CrossRef CAS PubMed;
(l) M. Li, D. Li, M. O'Keeffe and O. M. Yaghi, Chem. Rev., 2014, 114, 1343 CrossRef CAS PubMed;
(m) M. Eddaoudi, D. F. Sava, J. F. Eubank, K. Adil and V. Guillerm, Chem. Soc. Rev., 2015, 44, 228 RSC;
(n) P. Silva, S. M. F. Vilela, J. P. C. Tome and F. A. Almeida Paz, Chem. Soc. Rev., 2015, 44, 6774 RSC;
(o) B. Li, M. Chrzanowski, Y. Zhang and S. Ma, Coord. Chem. Rev., 2016, 307, 106 CrossRef CAS;
(p) C. Pettinari, A. Tabacaru and S. Galli, Coord. Chem. Rev., 2016, 307, 1 CrossRef CAS.
-
(a) Y. Yan, S. Yang, A. J. Blake and M. Schroeder, Acc. Chem. Res., 2014, 47, 296 CrossRef CAS PubMed;
(b) W. Lu, Z. Wei, Z.-Y. Gu, T.-F. Liu, J. Park, J. Park, J. Tian, M. Zhang, Q. Zhang, T. Gentle III, M. Bosch and H.-C. Zhou, Chem. Soc. Rev., 2014, 43, 5561 RSC;
(c) T. A. Makal, J.-R. Li, W. Lu and H.-C. Zhou, Chem. Soc. Rev., 2012, 41, 7761 RSC;
(d) M. P. Suh, H. J. Park, T. K. Prasad and D.-W. Lim, Chem. Rev., 2012, 112, 782 CrossRef CAS PubMed;
(e) Q. Yang, D. Liu, C. Zhong and J.-R. Li, Chem. Rev., 2013, 113, 8261 CrossRef CAS PubMed;
(f) B. Seoane, J. Coronas, I. Gascon, M. Etxeberria Benavides, O. Karvan, J. Caro, F. Kapteijn and J. Gascon, Chem. Soc. Rev., 2015, 44, 2421 RSC.
-
(a) B. Chen, S. Xiang and G. Qian, Acc. Chem. Res., 2010, 43, 1115 CrossRef CAS PubMed;
(b) B. Van de Voorde, B. Bueken, J. Denayer and D. De Vos, Chem. Soc. Rev., 2014, 43, 5766 RSC.
-
(a) S. M. Cohen, Chem. Rev., 2012, 112, 970 CrossRef CAS PubMed;
(b) J. Lee, O. K. Farha, J. Roberts, K. A. Scheidt, S. T. Nguyen and J. T. Hupp, Chem. Soc. Rev., 2009, 38, 1450 RSC;
(c) L. Ma, C. Abney and W. Lin, Chem. Soc. Rev., 2009, 38, 1248 RSC;
(d) M. Yoon, R. Srirambalaji and K. Kim, Chem. Rev., 2012, 112, 1196 CrossRef CAS PubMed;
(e) A. Corma, H. Garcia, F. X. L. I. Llabres and I. Xamena, Chem. Rev., 2010, 110, 4606 CrossRef CAS PubMed;
(f) A. H. Chughtai, N. Ahmad, H. A. Younus, A. Laypkov and F. Verpoort, Chem. Soc. Rev., 2015, 44, 6804 RSC;
(g) A. Dhakshinamoorthy, A. M. Asiri and H. Garcia, Chem. Soc. Rev., 2015, 44, 1922 RSC.
-
(a) C. W. Abney, J. C. Gilhula, K. Lu and W. Lin, Adv. Mater., 2014, 26, 7993 CrossRef CAS PubMed;
(b) M. Carboni, C. W. Abney, S. Liu and W. Lin, Chem. Sci., 2013, 4, 2396 RSC;
(c) J. Cui, Y.-L. Wong, M. Zeller, A. D. Hunter and Z. Xu, Angew. Chem., Int. Ed., 2014, 53, 14438 CrossRef CAS PubMed;
(d) J. He, M. Zha, J. Cui, M. Zeller, A. D. Hunter, S.-M. Yiu, S.-T. Lee and Z. Xu, J. Am. Chem. Soc., 2013, 135, 7807 CrossRef CAS PubMed;
(e) K.-K. Yee, N. Reimer, J. Liu, S.-Y. Cheng, S.-M. Yiu, J. Weber, N. Stock and Z. Xu, J. Am. Chem. Soc., 2013, 135, 7795 CrossRef CAS PubMed;
(f) J. He, K.-K. Yee, Z. Xu, M. Zeller, A. D. Hunter, S. S.-Y. Chui and C.-M. Che, Chem. Mater., 2011, 23, 2940 CrossRef CAS;
(g) X. P. Zhou, Z. Xu, M. Zeller and A. D. Hunter, Chem. Commun., 2009, 5439 RSC;
(h) M. Zha, J. Liu, Y.-L. Wong and Z. Xu, J. Mater. Chem. A, 2015, 3, 3928 RSC;
(i) B. Li, Y. Zhang, D. Ma, Z. Shi and S. Ma, Nat. Commun., 2014, 5, 5537 CrossRef CAS PubMed;
(j) Y. Wang, G. Ye, H. Chen, X. Hu, Z. Niu and S. Ma, J. Mater. Chem. A, 2015, 3, 15292 RSC;
(k) Y. Wang, Z. Liu, Y. Li, Z. Bai, W. Liu, Y. Wang, X. Xu, C. Xiao, D. Sheng, J. Diwu, J. Su, Z. Chai, T. E. Albrecht-Schmitt and S. Wang, J. Am. Chem. Soc., 2015, 137, 6144 CrossRef CAS PubMed;
(l) X. Meng, R.-L. Zhong, X.-Z. Song, S.-Y. Song, Z.-M. Hao, M. Zhu, S.-N. Zhaoa and H.-J. Zhang, Chem. Commun., 2014, 50, 6406 RSC;
(m) Q.-R. Fang, D.-Q. Yuan, J. Sculley, J.-R. Li, Z.-B. Han and H.-C. Zhou, Inorg. Chem., 2010, 49, 11637 CrossRef CAS PubMed;
(n) T. Liu, J.-X. Che, Y.-Z. Hu, X.-W. Dong, X.-Y. Liu and C.-M. Che, Chem.–Eur. J., 2014, 20, 14090 CrossRef CAS PubMed;
(o) F. Luo, J. L. Chen, L. L. Dang, W. N. Zhou, H. L. Lin, J. Q. Li, S. J. Liu and M. B. Luo, J. Mater. Chem. A, 2015, 3, 9616 RSC.
Footnote |
† Electronic supplementary information (ESI) available: Additional details of experimental procedure, PXRD, additional figures for FJI-H9 and FJI-H10. CCDC 1047502 and 1047503. For ESI and crystallographic data in CIF or other electronic format see DOI: 10.1039/c6sc00972g |
|
This journal is © The Royal Society of Chemistry 2016 |
Click here to see how this site uses Cookies. View our privacy policy here.