DOI:
10.1039/C5SC03262H
(Edge Article)
Chem. Sci., 2016,
7, 188-190
Concise synthesis of (+)-fastigiatine†‡
Received
31st August 2015
, Accepted 25th September 2015
First published on 6th October 2015
Abstract
(+)-Fastigiatine was assembled in six steps from (R)-5-methylcyclohex-2-en-1-one. Intermolecular Diels–Alder reaction introduced most of the carbon atoms for the target. The two Boc-protected nitrogen atom building blocks were introduced by a Suzuki coupling and a cuprate addition. A biomimetic transannular Mannich reaction generated the two quaternary centers at a late stage. Each step builds core bonds, and combined with a minimalist protecting group strategy, this approach led to a very concise synthesis.
Introduction
The family of Lycopodium alkaloids has long been of interest to synthetic chemists.1 Intramolecular Mannich reactions have often been featured in efficient syntheses of Lycopodium alkaloids, as illustrated in seminal reports by Stork2 and Heathcock.3 Recently, more complex Lycopodium alkaloids such as himeradine A,4,5 fastigiatine (1)6 and lyconadin A7 have attracted the attention of synthetic chemists (Fig. 1).8 Members of the family were shown to increase the expression of mRNA for neurotropic growth factor biosynthesis in 1321N1 human astrocytoma cells,9 and to show moderate anticancer activity.4,7 Lyconadin A has been synthesized multiple times, with notable improvements in step count and efficiency10 bringing later approaches closer to an ideal synthesis.11 Fastigiatine shares the core ring structure of himeradine A, and was recently synthesized by the Shair group.12 We report a new synthesis of (+)-fastigiatine that applies a simplified transannular Mannich cyclization to assemble the core structure.
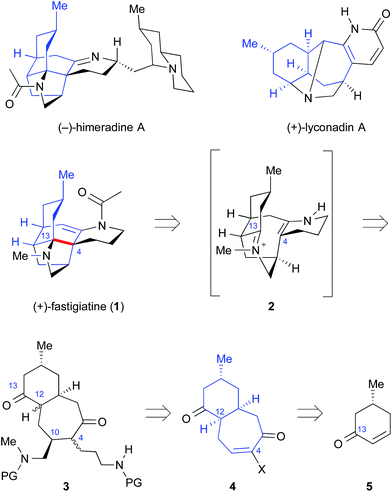 |
| Fig. 1 Lycopodium alkaloids and retrosynthetic analysis of fastigiatine using a transannular Mannich addition. The common benzo[7]annulene core (i.e.4) is illustrated in blue. | |
(+)-Fastigiatine contains five rings, two quaternary centers, and six stereogenic centers. Despite this structural complexity, a plausible biomimetic transannular Mannich reaction,13 proceeding through intermediate 2 in Fig. 1, leads to a dramatic simplification of the structure. We were intrigued by comparison of the cis benzo[7]annulene 4 with (+)-fastigiatine: it contains twelve of the carbon atoms and three of the stereogenic centers in the correct absolute configurations. The benzo[7]annulene structure 4 could be prepared by Diels–Alder reaction and ring expansion from cyclohexenone 5. This approach was developed in the retrosynthetic analysis illustrated in Fig. 1. One intriguing feature of this strategy is that the key transannular Mannich addition would proceed from intermediate 3 with no added functional groups: just two ketones and two amines. The key benzo[7]annulene intermediate 4 also maps onto the core structures of himeradine A and lyconadin A.
Results and discussion
The synthesis of (+)-fastigiatine is presented in Scheme 1. The sequence began with the preparation of a benzo[7]annulene similar to 4. Diels–Alder reaction of (R)-5-methylcyclohex-2-en-1-one (5)14 and diene 6 gave decalin 7 as a 14
:
1 mixture favoring the cis isomer.15 Dibromocarbene ring expansion16 produced bromo enone 8 in a ∼3
:
1 ratio favoring the cis isomer. (+)-Fastigiatine required the addition of a 3-carbon chain with a protected nitrogen atom, and precursor 9 was selected. Suzuki coupling17 between the borane derived from 9 and the bromo enone 8 led to a thermodynamic mixture of cis and trans isomers of 10 in excellent yield.
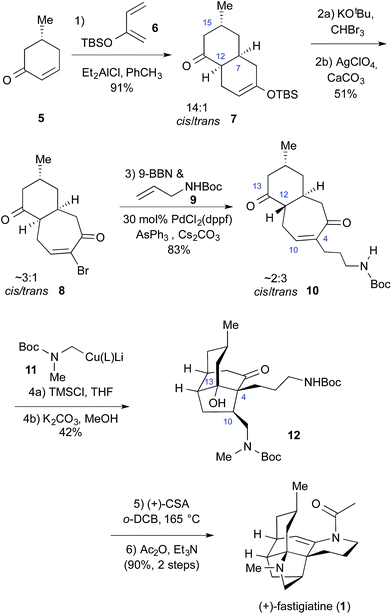 |
| Scheme 1 Synthesis of (+)-fastigiatine. | |
The first three intermediates in the synthesis are mixtures at the critical C12 center, reflecting the configurational lability of this position. Prior work in our lab demonstrated that epimerization could be avoided by protecting the C13 ketone, but that tactic added both steps and complexity to the synthesis. A key insight to simplify the strategy was the realization that a facile transannular aldol reaction12 between C4 and C13 could be exploited to correct the C12 configuration at a late stage in the synthesis. Abandoning protection of the C13 ketone enabled the direct approach to the synthesis of (+)-fastigiatine presented in Scheme 1.
A final tactical concern was that the synthesis required a “methylene amine” synthon that could be added to the 4-position of an enone. There are many possibilities including reagents as simple as diethylaluminium cyanide,18 but most of them would require extensive manipulation after the addition. A new cuprate reagent was developed from the crystalline phenylthio carbamate 13, Scheme 2. Reductive lithiation of 13 produced the alkyllithium reagent 14.19 Addition of 1-hexynylcopper(I) and trimethylphosphite at low temperature led to the desired reagent 11. Cuprate 11 added to cyclohexenone in excellent yield to deliver the N-Boc protected methylamine 15. Cuprate 11 delivers the exact fragment necessary for the (+)-fastigiatine synthesis, and by doing so, avoids late-stage manipulations.
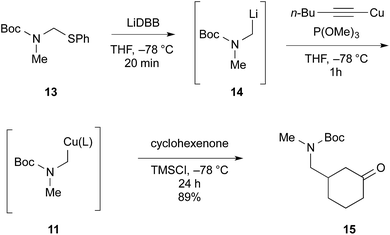 |
| Scheme 2 Development of cuprate reagent 11 for alkaloid synthesis. | |
Completion of the synthesis is shown in Scheme 1. Conjugate addition with cuprate 11 led to a very complex mixture of products, with epimers possible at C4, C10, and C12. The mixture was simplified by working up the reaction with K2CO3 and methanol, which generated tricyclic product 12 by epimerization and transannular aldol reaction. Compound 12 was isolated as a ca. 1
:
1 mixture with its C10 epimer in excellent yield, and the two isomers were separated by chromatography. The modest cuprate selectivity was not unexpected, as previous cuprate reactions with the protected C13 ketones also gave low selectivity.
Ketone 12 has the correct configuration at C10 for subsequent cyclization. Treatment of 12 with CSA in 1,2-dichlorobenzene (o-DCB) at elevated temperature8,20 removed the two Boc protecting groups and set up a retro-aldol equilibrium that permitted the formation of intermediate 2 en route to a transannular Mannich reaction. Use of 0.30 M CSA ensured complete conversion in the reaction. Acylation of the crude reaction mixture produced (+)-fastigiatine in excellent yield. Although high temperatures were required to access the key Mannich intermediate, this synthesis demonstrates that a minimally functionalized transannular Mannich intermediate is viable in a biomimetic cyclization.
Conclusions
The synthesis of (+)-fastigiatine was accomplished in six steps from enone 5 (and seven steps from a commercially available ketone) in 14.6% overall yield.14 Each step is productive in building molecular complexity.11 Suzuki coupling and cuprate addition with the novel cuprate reagent 11 were particularly effective for introducing Boc-protected amine synthons into bromo enone 8. The decision to embrace stereochemical lability at C12 simplified the sequence, while the transannular aldol reaction reestablished the correct configuration. The biomimetic transannular Mannich addition was very effective. The synthetic strategy developed for (+)-fastigiatine should be applicable to other Lycopodium alkaloids.
Acknowledgements
Support was provided by the UROP and SURF programs (C. M. O.) and the University of California, Irvine. We thank Richard Hill for helpful discussions.
Notes and references
-
(a) X. Ma and D. R. Gang, Nat. Prod. Rep., 2004, 21, 752–772 RSC;
(b) M. Kitajima and H. Takayama, Top. Curr. Chem., 2012, 309, 1–32 CrossRef CAS PubMed;
(c)
W. A. Ayer and L. S. Trifonov, in The Alkaloids, ed. G. A. Cordell and A. Brossi, Academic Press, New York, 1994, vol. 45, pp. 233−274 Search PubMed;
(d)
J. Kobayashi and H. Morita, in The Alkaloids, ed. G. A. Cordell, Academic Press, New York, 2005, vol. 61, pp. 1–57 Search PubMed.
- G. Stork, R. A. Kretchmer and R. A. Schlessinger, J. Am. Chem. Soc., 1968, 90, 647–1648 Search PubMed.
- C. H. Heathcock, E. F. Kleinman and E. S. Binkley, J. Am. Chem. Soc., 1982, 104, 1054–1068 CrossRef CAS.
- H. Morita, Y. Hirasawa and J. Kobayashi, J. Org. Chem., 2003, 68, 4563–4566 CrossRef CAS PubMed.
-
(a) N. D. Collett and R. G. Carter, Org. Lett., 2011, 13, 4144–4147 CrossRef CAS PubMed;
(b)
N. D. Collet, Ph.D. thesis, Oregon State University, OR, 2013.
-
(a) R. V. Gerard, D. B. MacLean, R. Fagianni and C. J. Lock, Can. J. Chem., 1986, 64, 943–949 CrossRef CAS;
(b) R. V. Gerard and D. B. MacLean, Phytochemistry, 1986, 25, 1143–1150 CrossRef CAS.
- J. Kobayashi, Y. Hirasawa, N. Yoshida and H. Morita, J. Org. Chem., 2001, 66, 5901–5904 CrossRef CAS PubMed.
- Conceptually similar approaches to lycodine: M. Azuma, T. Yoshikawa, N. Kogure, M. Kitajima and H. Takayama, J. Am. Chem. Soc., 2014, 136, 11618–11621 CrossRef CAS PubMed.
- K. I. Ishiuchi, T. Kubota, H. Ishiyama, S. Hayashi, T. Shibata, K. Mori, Y. Obara, N. Nakahata and J. Kobayashi, Bioorg. Med. Chem., 2011, 19, 749–753 CrossRef CAS PubMed.
-
(a) D. C. Beshore and A. B. Smith, J. Am. Chem. Soc., 2007, 129, 4148–4149 CrossRef CAS PubMed;
(b) A. Bisai, S. P. West and R. Sarpong, J. Am. Chem. Soc., 2008, 130, 7222–7223 CrossRef CAS PubMed;
(c) T. Nishimura, A. K. Unni, S. Yokoshima and T. Fukuyama, J. Am. Chem. Soc., 2011, 133, 418–419 CrossRef CAS PubMed;
(d) T. Nishimura, A. K. Unni, S. Yokoshima and T. Fukuyama, J. Am. Chem. Soc., 2013, 135, 3243–3247 CrossRef CAS PubMed;
(e) Y. Yang, C. W. Haskins, W. Zhang, P. L. Low and M. Dai, Angew. Chem., Int. Ed., 2014, 53, 3922–3925 CrossRef CAS PubMed.
-
(a) P. A. Wender and B. L. Miller, Nature, 2009, 460, 197–201 CrossRef CAS PubMed;
(b) T. Gaich and P. S. Baran, J. Org. Chem., 2010, 75, 4657–4673 CrossRef CAS PubMed;
(c) S. H. Bertz, J. Am. Chem. Soc., 1982, 104, 5801–5803 CrossRef CAS.
-
(a) B. B. Liau and M. D. Shair, J. Am. Chem. Soc., 2010, 132, 9594–9595 CrossRef CAS PubMed;
(b) A. S. Lee, B. B. Liau and M. D. Shair, J. Am. Chem. Soc., 2014, 136, 13442–13452 CrossRef CAS PubMed.
- McLean's proposed biosynthesis involved an intramolecular Mannich reaction (ref. 6), but Shair has made a strong argument for a transannular Mannich reaction in the biosynthesis (ref. 12).
- Ketone 5 was prepared in one step from commercially available (R)-3-methylcyclohexan-1-one or in four steps from pulegone. Ketone 5 was also an
intermediate in Shair's syntheses of (+)-fastigiatine and (–)-himeradine A.
(a) W. Oppolzer and M. Petrzilka, Helv. Chim. Acta, 1978, 61, 2755–2762 CrossRef CAS;
(b) D. Caine, K. Procter and R. A. Cassell, J. Org. Chem., 1984, 49, 2647–2648 CrossRef CAS;
(c) K. C. Nicolaou, Y. L. Zhong and P. S. Baran, J. Am. Chem. Soc., 2000, 122, 7596–7597 CrossRef CAS;
(d) K. Yamamoto, M. F. Hentemann, J. G. Allen and S. J. Danishefsky, Chem.–Eur. J., 2003, 9, 3242–3252 CrossRef CAS PubMed.
- X. Cheng and S. P. Waters, Org. Lett., 2010, 12, 205–207 CrossRef CAS PubMed.
-
(a) P. Amice, L. Blanco and J. M. Conia, Synthesis, 1976, 196–197 CrossRef CAS;
(b) P. Hudson, G. Pairaudeau, P. J. Parsons, A. W. Jahans and M. G. Drew, Tetrahedron Lett., 1993, 34, 7295–7298 CrossRef CAS;
(c) Q. Xiao, W.-W. Ren, Z.-X. Chen, T.-W. Sun, Y. Li, Q.-D. Ye, J.-X. Gong, F.-K. Meng, L. You, Y.-F. Liu, M.-Z. Zhao, L.-M. Xu, Z.-H. Shan, Y. Shi, Y.-F. Tang, J.-H. Chen and Z. Yang, Angew. Chem., Int. Ed., 2011, 50, 7373–7377 CrossRef CAS PubMed.
-
(a) N. Miyaura, T. Ishiyama, H. Sasaki, M. Ishikawa, M. Satoh and M. Suzuki, J. Am. Chem. Soc., 1989, 111, 314–321 CrossRef CAS;
(b) B. Bradshaw, C. Luque-Corredera, G. Saborit, C. Cativiela, R. Dorel, C. Bo and J. Bonjoch, Chem.–Eur. J., 2013, 19, 13881–13892 CrossRef CAS PubMed;
(c) S. R. Chemler, D. Trauner and S. J. Danishefsky, Angew. Chem., Int. Ed., 2001, 40, 4544–4568 CrossRef CAS.
- W. Nagata and M. Yoshioka, Org. React., 2005, 25, 255–476 Search PubMed.
- Alkyllithium 14 was previously prepared by reductive lithiation of the chloromethyl carbamate analogous to 13. We found the chloromethyl carbamate to be prone to decomposition. J. Ortiz, A. Guijarro and M. Yus, Tetrahedron, 1999, 55, 4831–4842 CrossRef CAS.
- Related cyclization reaction: B. Hong, H. Li, J. Wu, J. Zhang and X. Lei, Angew. Chem., Int. Ed., 2015, 54, 1011–1015 CrossRef CAS PubMed.
Footnotes |
† This manuscript is dedicated to Professor Gilbert Stork. |
‡ Electronic supplementary information (ESI) available: Experimental procedures and characterization data for all compounds are included. See DOI: 10.1039/c5sc03262h |
|
This journal is © The Royal Society of Chemistry 2016 |
Click here to see how this site uses Cookies. View our privacy policy here.