DOI:
10.1039/C6RA18987C
(Paper)
RSC Adv., 2016,
6, 104338-104344
A novel class of nickel(II) complexes containing selenium-based bidentate ligands applied in ethylene oligomerization†
Received
26th July 2016
, Accepted 26th October 2016
First published on 27th October 2016
Abstract
A series of nickel(II) complexes [NiBr2(N^Se)2] (Ni1–Ni5) based on bidentate N^Se ligands were prepared by reacting arylselenyl–pyrazolyl ligands (L1–L5) with NiBr2(DME) (DME = 1,2-dimethoxyethane). All Ni complexes were characterized by IR spectroscopy, elemental analysis, high-resolution mass spectrometry (HRMS), and by X-ray crystallography for Ni4. X-ray crystallographic analyses of Ni4 revealed an octahedral environment for the nickel atom and the presence of selenium–nickel bonds. Upon activation with methylaluminoxane (MAO), all Ni complexes showed moderate to good activities in ethylene oligomerization [TOF = 6.2–23.0 × 103 (mol ethylene) per (mol Ni) per h], producing mostly α-C4 (87.2–94.1 wt%) as the main product. The ligand environment, i.e. the substituents on the pyrazolyl and arylselenyl units, as well as the reaction parameters, influence the catalytic activity and selectivity towards the production of 1-butene. Under optimized reaction conditions (toluene, 400 equiv. of MAO, 40 °C and 20 min), the Ni2/MAO catalytic system yielded TOF values up to 109.2 × 103 (mol ethylene) per (mol Ni) per h with good selectivities for α-C4 (85.4 wt%).
Introduction
The oligomerization of ethylene is one of the most important industrial processes, moving billions of dollars worldwide.1 The linear α-olefins (LAOs) obtained by the oligomerization processes are valuable chemical commodities for a variety of industrial and household applications. The most important ones are the α-C4–C8 range, which are used as comonomers for the production of linear low-density polyethylene (LLDPE); α-C6–C10, used as plasticizers; α-C8–C10, used as trimers in lubricants and synthetic lube-oils; and α-C12–C20, utilized as surfactants in detergents.2 In this context, a considerable amount of research effort is focused on improving and understanding this industrially relevant catalytic process.
One of the most important examples is the SHOP (Shell Higher Olefins Process), where the use of a bidentate P^O homogeneous nickel catalyst selectively oligomerizes ethylene to produce higher-molecular-weight olefins (C4–C20+).2a Among the classes of nickel catalysts bearing bidentate ligands used for the production of LAOs, those containing P^P,3 P^N,4 P^O,5 N^N,6 and N^O7 are further described in the literature.
Our group has also been interested in exploring the potential applications of nickel complexes in the field of oligomerization catalysis.8 For instance, we have demonstrated that the catalytic performance of nickel complexes towards the production of 1-butene is strongly dependent on the donor groups (N, O, P, and S) in the ligand structure. In the case of nickel complexes based on tridentate pyrazolyl ligands, the replacement of the central nitrogen- or oxygen-donor by a sulphur atom leads to higher-activity systems.8c,d,9 In view of these previous results, the use of a softer donor atom seems best stabilize the catalytically active specie, leading in a higher activities. In this context, ligands containing selenium-donor groups appear as candidates to generate high-activity oligomerization systems. In the past few years, several examples of selenium-based ligands have been synthesized and used in organometallic catalysis, e.g. in Heck,10 Suzuki10a,d and Sonogashira11 coupling reactions. On the other hand, no examples of metal complexes based on selenium ligands have been used in ethylene oligomerization reactions.
In this context, we decided to synthesize and characterize a new class of nickel(II) complexes containing arylselenyl–pyrazolyl bidentate ligands [NiBr2(N^Se)2] and evaluate their catalytic performance for ethylene oligomerization in the presence of methylaluminoxane (MAO) as cocatalyst. We also discuss the effect of the substituents, backbone chain and reaction conditions on their activity and selectivity towards the production of 1-butene.
Results and discussion
Synthesis of nickel complexes containing selenium-based ligands
The bidentate arylselenyl–pyrazolyl ligands (L1–L5) were prepared by reacting chloroalkyl pyrazoles with the corresponding nucleophilic species of selenium in a THF/EtOH mixture as presented in Scheme 1. These ligands were obtained in moderate to high yields (57–82%) after purification by column chromatography, and were characterized by elemental analysis, IR, 1H and 13C NMR spectroscopy.
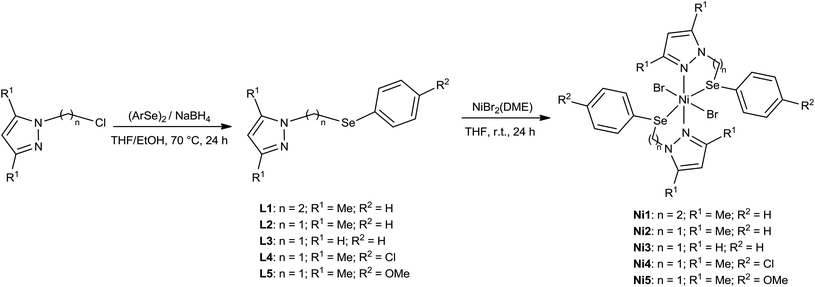 |
| Scheme 1 Synthesis of arylselenyl–pyrazolyl ligands (L1–L5) and [NiBr2(N^Se)2] complexes (Ni1–Ni5). | |
Treatment of NiBr2(DME) with 2.0 equiv. of L1–L5 yielded the corresponding [NiBr2(N^Se)2] complexes (Ni1–Ni5) (Scheme 1). The nickel complexes were isolated as light green in moderate to good yields (48–81%). These compounds, which are air- and moisture-sensitive, show moderate solubility at room temperature in coordinating solvents such as THF and acetonitrile, and are slightly soluble in alkane and aromatic solvents. The characterization of Ni1–Ni5 was established on the basis of elemental analysis, IR spectroscopy, and high-resolution mass spectrometry (HRMS). The HRMS afforded spectra in which the main peak corresponded to the [NiBr(N^Se)2]+ ions. Informative NMR data could not be obtained due to the paramagnetic nature of these nickel complexes.
Crystal structure of Ni4·PhMe
Single crystals of Ni4·PhMe suitable for X-ray diffraction studies were grown at room temperature from a concentrated toluene solution. The molecular structure of the complex is shown in Fig. 1. The main crystallographic data and structure refinement parameters are reported in Table 1. Selected bond lengths and angles are listed in Table 2. The single-crystal X-ray diffraction analysis reveals a six-coordinated nickel centre, with a slightly distorted octahedral geometry (Fig. 1). Moreover, one solvate toluene molecule crystallizes per nickel complex. The central nickel atom is chelated by two arylselenyl–pyrazolyl ligands, and its coordination sphere is completed by other two bromide ligands. All equivalent donor atoms are in a trans position. The Ni–N bond distances [2.0830(18) Å and 2.1038(18) Å] are close to the values previously reported for Ni(II) complexes containing pyrazolyl ligands.12 The average Ni–Se bond distances [2.5627(4) Å and 2.6092(4) Å] are comparable to those found in other selenoether–nickel complexes.13 The Ni–Br bond distances [2.5711(4) Å and 2.5063(4) Å] are shorter than the sum of the covalent radii and are in accordance with other nickel–bromide complexes.12 The chelate bite angles of the arylselenyl–pyrazolyl ligand [N(1)–Ni–Se(1) 84.37(5)° and N(3)–Ni–Se(2) 79.51(5)°], as well as the other bond lengths and angles, confirm the distorted octahedral geometry for the central nickel atom.
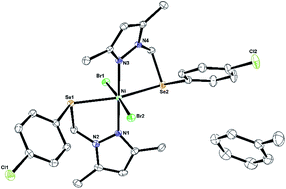 |
| Fig. 1 Molecular structure of Ni4·PhMe with the key atoms labelled (thermal ellipsoids drawn at 50% probability level). For clarity the hydrogen atoms have been omitted. | |
Table 1 Crystallographic data and structure refinement for Ni4·PhMe
As defined by the SHELXL program.17 |
Molecular formula |
C24H26Br2Cl2N4NiSe2·C7H8 |
Formula weight (g mol−1) |
909.97 |
T (K) |
100(2) |
Crystal system |
Triclinic |
Space group |
P![[1 with combining macron]](https://www.rsc.org/images/entities/char_0031_0304.gif) |
a (Å) |
7.9476(5) |
b (Å) |
13.7447(10) |
c (Å) |
16.6566(13) |
α (°) |
67.955(3) |
β (°) |
89.956(3) |
γ (°) |
83.980(3) |
V (Å3) |
1675.7(2) |
Z |
2 |
ρcalcd (g cm−3) |
1.803 |
μ (mm−1) |
5.323 |
F(000) |
896 |
Crystal size (mm) |
0.214 × 0.125 × 0.042 |
θ range (°) |
2.521 to 30.567 |
Limiting indices (h, k, l) |
−11 ≤ h ≤ 11 |
−19 ≤ k ≤ 19 |
−23 ≤ l ≤ 23 |
Reflections collected |
42 143 |
Reflections unique (Rint) |
10 249 (0.0340) |
Completeness to θmax (%) |
99.9 |
Data/restraints/param. |
10 249/0/379 |
Absorption correction |
Gaussian |
Min. and max. transmission |
0.5442 and 0.7461 |
R1 [I > 2σ(I)]a |
0.0272 |
wR2 [I > 2σ(I)]a |
0.0629 |
R1 (all data)a |
0.0387 |
wR2 (all data)a |
0.0669 |
S on F2a |
1.023 |
Largest diff. peak and hole (e Å−3) |
0.835 and −0.935 |
Table 2 Selected bond lengths (Å) and angles (°) for Ni4·PhMe
Bond lengths |
Bond angles |
Ni–Se(1) |
2.5627(4) |
N(1)–Ni–N(3) |
179.23(7) |
Ni–Se(2) |
2.6092(4) |
N(1)–Ni–Se(1) |
84.37(5) |
Ni–Br(1) |
2.5711(4) |
Br(2)–Ni–Br(1) |
175.626(13) |
Ni–Br(2) |
2.5063(4) |
N(3)–Ni–Se(2) |
82.33(5) |
Ni–N(1) |
2.0830(18) |
Se(1)–Ni–Se(2) |
163.335(13) |
Ni–N(3) |
2.1038(18) |
|
|
Ethylene oligomerization studies
Nickel complexes Ni1–Ni5 were tested as precatalysts in ethylene oligomerization reactions using methylaluminoxane (MAO) (20 wt% TMA) as cocatalyst. In this case, MAO was chosen taking into account the good catalytic performance of similar pyrazolyl–ether complexes in terms of activity as well as selectivity for production of 1-butene.8a,c Moreover, the use of other alkyl aluminium cocatalysts such as EASC8b and DEAC8c afforded poor selectivity catalyst systems. The reactions were carried out at 30 °C, and using 20 bar of ethylene pressure (kept constant) and 6.5 μmol of precatalyst in 100 mL of toluene. Table 3 summarizes the results of the oligomerization reactions. All experiments were performed at least in duplicate, yielding reproducible results within ±5%. All nickel complexes were found to generate active systems for the oligomerization of ethylene, with turnover frequencies (TOFs) varying from 6.2 to 23.0 × 103 (mol ethylene) per (mol Ni) per h.
Table 3 Catalytic data for complexes Ni1–Ni5 using MAO as cocatalysta
Entry |
Cat. |
[Al/Ni] |
T (°C) |
Time (min) |
Olig. (g) |
TOFb (103) |
Selectivity (mass%) |
C4 (α-C4) |
C4 (cis) |
C4 (trans) |
C6 |
Reaction conditions: [Ni] = 6.5 μmol; toluene = 100 mL; P(ethylene) = 20 bar (kept constant); MAO as cocatalyst. The results shown are representative of at least duplicated experiments. (mol of ethylene converted) per (mol of Ni) per h as determined by quantitative gas–liquid chromatography. |
1 |
Ni1 |
400 |
30 |
20 |
0.5 |
10.0 |
97.7 (92.1) |
4.1 |
1.5 |
2.3 |
2 |
Ni2 |
400 |
30 |
20 |
1.3 |
23.0 |
96.5 (87.2) |
5.5 |
3.8 |
3.5 |
3 |
Ni3 |
400 |
30 |
20 |
0.3 |
6.2 |
97.9 (93.3) |
2.5 |
2.1 |
2.1 |
4 |
Ni4 |
400 |
30 |
20 |
1.0 |
19.3 |
95.9 (91.8) |
2.4 |
1.7 |
4.1 |
5 |
Ni5 |
400 |
30 |
20 |
0.8 |
15.4 |
98.9 (94.1) |
3.0 |
1.8 |
1.1 |
6 |
Ni2 |
800 |
30 |
20 |
1.8 |
33.2 |
99.5 (91.1) |
5.5 |
2.9 |
0.5 |
7 |
Ni2 |
1600 |
30 |
20 |
2.2 |
40.1 |
99.3 (91.8) |
4.9 |
2.6 |
0.7 |
8 |
Ni2 |
2400 |
30 |
20 |
2.8 |
52.5 |
95.7 (86.9) |
5.7 |
3.1 |
4.3 |
9 |
Ni2 |
400 |
30 |
10 |
0.4 |
7.5 |
99.6 (91.4) |
5.1 |
3.1 |
0.4 |
10 |
Ni2 |
400 |
30 |
30 |
1.3 |
14.4 |
94.1 (84.2) |
5.6 |
4.3 |
5.9 |
11 |
Ni2 |
400 |
40 |
20 |
5.9 |
109.2 |
95.5 (85.4) |
6.0 |
4.1 |
4.5 |
12 |
Ni2 |
400 |
50 |
20 |
2.3 |
42.1 |
94.8 (83.4) |
6.8 |
4.6 |
5.2 |
The ligand environment, i.e. the substituent on the arylselenyl and pyrazolyl units, as well as the length of the backbone chain, influenced the catalytic performance of the nickel precatalysts. Among the catalytic systems studied herein, Ni2/MAO was found to give the highest activity [TOF = 23.0 × 103 (mol ethylene) per (mol Ni) per h] with good selectivity towards the production of 1-butene (87.2%). The correlation between the catalytic performance and the length of the backbone chain can be observed by comparing the catalytic activities of Ni1 and Ni2. Thus, L2 directs the formation of a five-membered ring around the nickel centre, leading to a higher activity for Ni2 as compared with the Ni1/MAO system [TOF = 10.0 × 103 (mol ethylene) per (mol Ni) per h]. This result is in accordance with the catalytic activities presented by nickel complexes based on tridentate bis(pyrazolyl) ligands, where changing the six-membered chelate ring to a five-membered one increased the catalytic activity of the complexes, probably due to the formation of a more rigid and stable structure.8c On the other hand, the lower activity of Ni3 [TOF = 6.2 × 103 (mol ethylene) per (mol Ni) per h] compared with Ni2 can be tentatively associated with the lower electron-donating ability of the pyrazolyl unit when compared with methyl substituents.14 This observation is in agreement with previously reported ethylene oligomerization results using MAO-activated nickel complexes bearing pyrazolyl ligands.8a Moreover, the presence of an electron-donating (Ni5) or withdrawing group (Ni4) on the arylselenyl unit did not exert any significant influence on the TOF. On the other hand, the arylselenyl–pyrazolyl ligands have no significant influence on the selectivity for production of 1-butene attaining the values between 87.2 and 94.1% with formation of only a minimal amount of hexenes (1.1–4.1%). In this case, the electronic and/or structural modification on the ligand framework did not provide any substantial impact on the product distribution.
On the basis of these preliminary results, complex Ni2 was selected to investigate the effect of the [Al]/[Ni] molar ratio, temperature and oligomerization time on the TOF and selectivity towards the production of 1-butene. When activated with higher loading of MAO (800 equiv.), Ni2 gave higher activity [TOF = 33.2 × 103 (mol ethylene) per (mol Ni) per h], which continued to increase until using 2400 equiv. of MAO [TOF = 52.5 × 103 (mol ethylene) per (mol Ni) per h]. At the same time, increasing the amount of MAO to 2400 equiv. led to a slight reduction in the selectivity for 1-butene (86.9 wt%), probably due to the exothermic character of the reaction.1b,4e
Studies of the effect of oligomerization temperature have revealed that Ni2 is quite sensitive to variation of this parameter. For instance, elevating the temperature from 30 °C to 40 °C led to a substantial increase in the activity [TOF = 109.2 × 103 (mol ethylene) per (mol Ni) per h]. This effect can be attributed to the higher solubility of Ni2 in toluene at 40 °C, which promotes the formation of larger amounts of active species. It is noteworthy to mention that at 40 °C, the Ni2/MAO catalytic system was much more active than a nickel precatalyst based on a pyrazolyl–ether ligand, NiCl2{3,5-dimethyl-1-(3-phenoxypropyl)-1H-pyrazole} [TOF = 65.1 × 103 (mol ethylene) per (mol Ni) per h], suggesting that selenium-based ligands are a good alternative for generating highly active catalyst systems.8a Elevating the temperature to 50 °C led to a reduction in the activity [TOF = 42.1 × 103 (mol ethylene) per (mol Ni) per h], suggesting a partial decomposition of the active catalytic sites. At the same time, increasing the temperature from 30 °C led to a slight decrease of the 1-butene selectivity.
The catalyst activities and selectivities were also monitored versus time by using the Ni2/MAO catalytic system with an [Al]/[Ni] molar ratio of 400 at 30 °C. At 10 min, the Ni2/MAO catalyst system showed lower activity, which may be attributable to the poor solubility of the complex in toluene. However, we cannot rule out the explanation that MAO has a low ability to activate Ni2 in 10 min. As the oligomerization time increased from 20 min to 30 min, the TOF decreased from 23.0 × 103 to 14.4 × 103 (mol ethylene) per (mol Ni) per h. In this case, the generation of the same amount of products (1.3 g) at 20 and 30 min indicates the complete deactivation of Ni2 after 20 min. However, the reaction time did not substantially affect the selectivity for 1-butene, which varied from 91.4% (10 min) to 84.2% (30 min).
Conclusions
A new series of nickel(II) complexes (Ni1–Ni5) based on bidentate arylselenyl–pyrazolyl ligands were synthesized and characterized. The molecular structure of Ni4 showed an octahedral environment around the nickel metal centre, with the existence of selenium–nickel bonds. All the nickel complexes proved to be active catalytic systems for the oligomerization of ethylene upon activation with MAO. The ligand environment, i.e. the substituents on the arylselenyl and pyrazolyl units, as well as the length of the backbone chain, influenced the catalytic performance of the catalysts. Thus, the presence of methyl groups at the 3- and 5-positions of the pyrazolyl ring and the formation of five-membered nickel complexes afforded more highly active systems. Both the activity and the selectivity towards the production of 1-butene can be controlled by varying the reaction conditions. The nickel complexes bearing selenium–pyrazolyl bidentate ligands introduced in this paper demonstrate high potential to act as effective oligomerization catalysts. Further studies are underway in order to expand this class of nickel catalysts, evaluating mainly the influence of the steric and electronic properties of the selenium-based ligands on the activity and selectivity towards the production of 1-butene.
Experimental section
General
All manipulations involving air- and/or water-sensitive compounds were carried out in an MBraun glovebox or under dry argon using standard Schlenk techniques in a vacuum line. Solvents were purified and dried by standard procedures and freshly distilled prior to use. NiBr2(DME) (DME = 1,2-dimethoxyethane), 1H-pyrazole and 3,5-dimethyl-1H-pyrazole were purchased from Sigma-Aldrich and used as received. 1-(2-Chloroethyl)-3,5-dimethyl-1H-pyrazole,15 1-(chloromethyl)-3,5-dimethyl-1H-pyrazole16 and 1-(chloromethyl)-1H-pyrazole16 were synthesized following the literature procedures. Ethylene (White Martins Co.) and argon were deoxygenated and dried through BTS columns (BASF) and activated molecular sieves prior to use. MAO (Witco, 5.21 wt% Al solution in toluene, 20% TMA) was used as received. Infrared spectra were performed on an FT-IR Bruker Alpha Spectrometer. NMR spectra of the ligands were recorded on a Varian MR 400 MHz spectrometer operating at 25 °C. 1H and 13C chemical shifts are reported in ppm vs. SiMe4 and were determined by reference to the residual solvent peaks. Elemental analysis was performed by the Analytical Central Service of the Institute of Chemistry-UFRGS (Brazil) and is the average of two independent determinations. High-resolution mass spectra (HRMS) of Ni(II) complexes (Ni1–Ni5) were obtained by electrospray ionization (ESI) in the positive mode in CH3OH solutions using a Micromass Waters® Q-Tof spectrometer. Quantitative gas chromatographic analysis of the ethylene oligomerization products was performed on an Agilent 7890A instrument with a Petrocol DH capillary column (methyl silicone, 100 m length, 0.25 mm i.d. and 0.5 μm film thickness) operating at 36 °C for 15 min followed by heating at 5 °C min−1 until 250 °C; cyclohexane was used as the internal standard.
3,5-Dimethyl-1-(2-(phenylselenyl)ethyl)-1H-pyrazole (L1). To a solution of (PhSe)2 (0.468 g, 1.5 mmol) in ethanol/THF (v/v = 1
:
3, 20 mL) was added NaBH4 (0.126 g, 3.3 mmol) and the reaction was stirred until colourless. Then, 1-(2-chloroethyl)-3,5-dimethyl-1H-pyrazole (0.317 g, 2 mmol) in ethanol (5 mL) was added, and the mixture was further stirred for 24 h at 70 °C. The reaction was extracted with dichloromethane (3 × 20 mL) and washed with H2O (2 × 30 mL). The organic phase was dried over MgSO4, filtered, and the solvent was evaporated, resulting in a dark yellow oil. After purification by chromatographic column on silica gel (hexane/ethyl acetate, 90
:
10), L1 was obtained as a light yellow oil (0.335 g, 60%). 1H NMR (CDCl3, 298 K, 400 MHz): δ 2.12 (s, 3H, CH3), 2.18 (s, 3H, CH3), 3.25 (t, 3JHH = 8 Hz, 2H, CH2), 4.19 (t, 3JHH = 12 Hz, 2H, CH2), 5.72 (s, 1H, Hpyr), 7.25 (m, 3H, Haro), 7.49 (m, 2H, Haro). 13C NMR (CDCl3, 298 K, 100 MHz): δ 11.03 (CH3), 13.57 (CH3), 27.14 (CH2), 48.64 (CH2), 105.04 (C–Hpyr), 127.32 (Caro), 129.07 (Caro), 129.27 (Caro), 132.90 (Caro), 138.91 (C–Npyr), 147.81 (C–Npyr). IR (ATR, cm−1): ν 3053 (w), 2917 (w), 2863 (m), 1578 (m), 1552 (m), 1477 (m), 1459 (m), 1436 (m), 1421 (m), 1384 (m), 1299 (m), 1252 (w), 1072 (w), 1021 (m), 776 (m), 732 (s), 689 (s), 668 (m), 636 (w), 614 (m). Anal. calc. for C13H16N2Se: C, 55.92; H, 5.78; N, 10.03. Found: C, 56.67; H, 5.61; N, 9.99.
3,5-Dimethyl-1-((phenylselenyl)methyl)-1H-pyrazole (L2). This product was prepared by following a similar procedure to that described above for L1, starting from (PhSe)2 (0.936 g, 3 mmol), NaBH4 (0.256 g, 6.6 mmol) and 1-(chloromethyl)-3,5-dimethyl-1H-pyrazole (0.576 g, 4 mmol). After purification by chromatographic column on silica gel (hexane/ethyl acetate, 70
:
30), L2 was obtained as a light yellow oil (0.870 g, 82%). 1H NMR (CDCl3, 298 K, 400 MHz): δ 1.86 (s, 3H, CH3), 2.19 (s, 3H, CH3), 5.39 (s, 2H, CH2), 5.74 (s, 1H, Hpyr), 7.29 (m, 3H, Haro), 7.49 (m, 2H, Haro). 13C NMR (CDCl3, 298 K, 100 MHz): δ 10.82 (CH3), 13.69 (CH3), 45.11 (CH2), 106.29 (C–Hpyr), 128.30 (Caro), 128.46 (Caro), 129.17 (Caro), 135.78 (Caro), 139.72 (C–Npyr), 148.74 (C–Npyr). IR (ATR, cm−1): ν 3053 (w), 2921 (w), 2859 (m), 1557 (m), 1455 (m), 1415 (m), 1377 (m), 1292 (m), 1234 (m), 1146 (w), 1069 (w), 1022 (m), 973 (m), 784 (m), 736 (s), 689 (m), 661 (s), 583 (m). Anal. calc. for C12H14N2Se: C, 54.34; H, 5.32; N, 10.56. Found: C, 54.99; N, 11.08; H, 5.73.
1-((Phenylselenyl)methyl)-1H-pyrazole (L3). This product was prepared by following a similar procedure to that described above for L1, starting from (PhSe)2 (0.936 g, 3 mmol), NaBH4 (0.256 g, 6.6 mmol) and 1-(chloromethyl)-1H-pyrazole (0.464 g, 4 mmol). After purification by chromatographic column on silica gel (hexane/ethyl acetate, 70
:
30), L3 was obtained as a light yellow oil (0.642 g, 68%). 1H NMR (CDCl3, 298 K, 400 MHz): δ 5.53 (s, 2H, CH2), 6.19 (t, 3JHH = 2 Hz, 1H, Hpyr), 7.21 (d, 3JHH = 2.4 Hz, 1H, Hpyr), 7.29 (m, 3H, Haro), 7.48 (m, 2H, Haro), 7.53 (d, 2JHH = 1.6 Hz, 1H, Hpyr). 13C NMR (CDCl3, 298 K, 100 MHz): 47.86 (CH2), 106.38 (C–Hpyr), 128.29 (Caro), 128.33 (Caro), 129.17 (Caro), 129.28 (C–Npyr), 134.82 (Caro), 140.21 (C–Npyr). IR (ATR, cm−1): ν 3054 (w), 2922 (w), 2852 (m), 1576 (w), 1513 (m), 1476 (m), 1436 (m), 1406 (w), 1389 (m), 1280 (m), 1239 (m), 1208 (m), 1132 (w), 1085 (m), 1042 (m), 1021 (m), 998 (w), 962 (m), 915 (m), 879 (w), 837 (w), 735 (s), 709 (m), 688 (s), 623 (s), 585 (m). Anal. calc. for C10H10N2Se: C, 50.64; H, 4.25; N, 11.81. Found: C, 49.41; H, 3.88; N, 10.86.
1-(((4-Chlorophenyl)selenyl)methyl)-3,5-dimethyl-1H-pyrazole (L4). This product was prepared by following a similar procedure to that described above for L1, starting from (p-Cl-PhSe)2 (1.146 g, 3 mmol), NaBH4 (0.256 g, 6.6 mmol) and 1-(chloromethyl)-3,5-dimethyl-1H-pyrazole (0.576 g, 4 mmol). After purification by chromatographic column on silica gel (hexane/ethyl acetate, 75
:
25), L4 was obtained as a light yellow oil (0.683 g, 57%). 1H NMR (CDCl3, 298 K, 400 MHz): δ 1.91 (s, 3H, CH3), 2.16 (s, 3H, CH3), 5.35 (s, 2H, CH2), 5.74 (s, 1H, Hpyr), 7.19 (dd, J = 2 Hz and 6.4 Hz, 2H, Haro), 7.37 (dd, J = 2 Hz and 6.4 Hz, 2H, Haro). 13C NMR (CDCl3, 298 K, 100 MHz): δ 11.16 (CH3), 13.84 (CH3), 45.27 (CH2), 106.68 (C–Hpyr), 126.42 (Caro), 129.46 (Caro), 135.11 (Caro), 137.30 (Caro), 139.81 (C–Npyr), 148.93 (C–Npyr). IR (ATR, cm−1): ν 3047 (w), 2920 (m), 2852 (m), 1558 (m), 1467 (m), 1416 (m), 1378 (m), 1292 (m), 1234 (s), 1146 (w), 1086 (s), 1011 (m), 973 (m), 813 (s), 783 (s), 729 (m), 663 (m), 582 (m). Anal. calc. for C12H13ClN2Se: C, 48.10; H, 4.37; N, 9.35. Found: C, 50.66; H, 4.25; N, 9.21.
1-(((4-Methoxyphenyl)selenyl)methyl)-3,5-dimethyl-1H-pyrazole (L5). This product was prepared by following a similar procedure to that described above for L1, starting from (p-OMe-PhSe)2 (1.12 g, 3 mmol), NaBH4 (0.256 g, 6.6 mmol) and 1-(chloromethyl)-3,5-dimethyl-1H-pyrazole (0.576 g, 4 mmol). After purification by chromatographic column on silica gel (hexane/ethyl acetate, 75
:
25), L5 was obtained as a light yellow oil (0.909 g, 77%). 1H NMR (CDCl3, 298 K, 400 MHz): δ 1.81 (s, 3H, CH3), 2.17 (s, 3H, CH3), 3.76 (s, 3H, O–CH3), 5.28 (s, 2H, CH2), 5.71 (s, 1H, Hpyr), 6.75 (dd, J = 1.2 Hz and 8.8 Hz, 2H, Haro), 7.35 (dd, J = 1.3 Hz and 8.8 Hz, 2H, Haro). 13C NMR (CDCl3, 298 K, 100 MHz): δ 10.74 (CH3), 13.63 (CH3), 45.30 (CH2), 55.29 (O–CH3), 106.05 (C–Hpyr), 114.69 (Caro), 118.16 (Caro), 137.95 (Caro), 139.65 (C–Npyr), 148.55 (C–Npyr), 160.21 (O–Caro). IR (ATR, cm−1): ν 3060 (w), 2922 (w), 2856 (m), 2836 (m), 1588 (m), 1557 (m), 1489 (s), 1417 (m), 1379 (m), 1285 (m), 1241 (w), 1172 (m), 1100 (m), 1025 (m), 822 (m), 790 (m), 659 (m), 598 (m), 516 (m). Anal. calc. for C13H16N2OSe: C, 52.89; H, 5.46; N, 9.49. Found: C, 53.56; H, 5.75; N, 8.84.
NiBr2{bis(3,5-dimethyl-1-(2-(phenylselenyl)ethyl)-1H-pyrazole)} (Ni1). To a solution of NiBr2(DME) (0.165 g, 0.53 mmol) in THF (15 mL) was added a solution of L1 (0.310 g, 1.11 mmol) in THF (5 mL). The reaction mixture was stirred for 24 h at room temperature. Then, the solvent was removed under vacuum resulting in a purple solid residue that was washed with Et2O (3 × 10 mL). Complex Ni1 was obtained as a purple solid (0.199 g, 48%). IR (ATR, cm−1): ν 3051 (m), 2922 (m), 2860 (m), 1720 (w), 1577 (m), 1551 (s), 1465 (s), 1435 (s), 1384 (s), 1301 (m), 1253 (m), 1197 (w), 1152 (m), 1020 (m), 852 (w), 794 (m), 734 (s), 689 (m), 668 (m), 614 (w). Anal. calc. for C26H32Br2N4NiSe2: C, 40.19; H, 4.15; N, 7.21. Found: C, 39.07; H, 4.07; N, 6.54. ESI-HRMS (CH3OH, m/z): 696.9516 [M − Br]+ (calc. for C26H32BrN4NiSe2: 696.9494).
NiBr2{bis(3,5-dimethyl-1-((phenylselenyl)methyl)-1H-pyrazole)} (Ni2). This product was prepared in a similar manner as described above for Ni1, starting from NiBr2(DME) (0.087 g, 0.28 mmol) in THF (15 mL) and L2 (0.165 g, 0.62 mmol) in THF (5 mL) to give Ni2 as a dark green solid. (0.156 g, 74%). IR (ATR, cm−1): ν 3053 (w), 2922 (m), 2863 (m), 1715 (w), 1661 (w), 1552 (m), 1460 (m), 1415 (s), 1378 (m), 1285 (w), 1235 (m), 1041 (m), 998 (m), 794 (m), 738 (s), 665 (m), 661 (m), 609 (w). Anal. calc. for C24H28Br2N4NiSe2: C, 38.49; H, 3.77; N, 7.48. Found: C, 38.66; H, 4.01; N, 7.19. ESI-HRMS (CH3OH, m/z): 668.9186 [M − Br]+ (calc. for C24H28BrN4NiSe2: 668.9181).
NiBr2{bis(1-((phenylselenyl)methyl)-1H-pyrazole)} (Ni3). This product was prepared in a similar manner as described above for Ni1, starting from NiBr2(DME) (0.098 g, 0.32 mmol) in THF (15 mL) and L3 (0.165 g, 0.70 mmol) in THF (5 mL) to give Ni3 as a light green solid. (0.177 g, 81%). IR (ATR, cm−1): ν 3047 (w), 2938 (w), 2854 (w), 1574 (w), 1479 (m), 1430 (m), 1333 (w), 1241 (m), 1154 (m), 1055 (m), 987 (m), 902 (m), 772 (m), 732 (s), 685 (m), 661 (m), 597 (m). Anal. calc. for C20H20Br2N4NiSe2: C, 34.67; H, 2.91; N, 8.09. Found: C, 34.70; H, 3.65; N, 7.08. ESI-HRMS (CH3OH, m/z): 612.8555 [M − Br]+ (calc. for C20H20BrN4NiSe2: 612.8555).
NiBr2{bis(1-(((4-chlorophenyl)selenyl)methyl)-3,5-dimethyl-1H-pyrazole)} (Ni4). This product was prepared in a similar manner as described above for Ni1, starting from NiBr2(DME) (0.077 g, 0.25 mmol) in THF (15 mL) and L4 (0.165 g, 0.55 mmol) in THF (5 mL) to give Ni4 as a dark green solid. (0.135 g, 66%). IR (ATR, cm−1): ν 3050 (m), 2921 (m), 2856 (m), 2364 (w), 1554 (m), 1467 (m), 1416 (m), 1381 (m), 1285 (w), 1235 (m), 1088 (s), 1042 (m), 1005 (m), 806 (s), 726 (m), 663 (s), 612 (m). Anal. calc. for C24H26Br2Cl2N4NiSe2: C, 35.25; H, 3.20; N, 6.85. Found: C, 34.76; H, 3.80; N, 5.91. ESI-HRMS (CH3OH, m/z): 738.8393 [M − Br]+ (calc. for C24H26BrCl2N4NiSe2: 738.8381).
NiBr2{bis(1-(((4-methoxyphenyl)selenyl)methyl)-3,5-dimethyl-1H-pyrazole)} (Ni5). This product was prepared in a similar manner as described above for Ni1, starting from NiBr2(DME) (0.078 g, 0.25 mmol) in THF (15 mL) and L5 (0.165 g, 0.56 mmol) in THF (5 mL) to give Ni5 as a dark green solid. (0.149 g, 73%). IR (ATR, cm−1): ν 3051 (m), 2916 (m), 2836 (m),1717 (w), 1625 (w), 1585 (m), 1554 (m), 1489 (s), 1458 (m), 1416 (m), 1384 (m), 1287 (m), 1247 (s), 1175 (m), 1104 (w), 1020 (m), 809 (m), 657 (w), 597 (w), 518 (w). Anal. calc. for C26H32Br2N4NiO2Se2: C, 38.60; H, 3.99; N, 6.93. Found: C, 37.83; H, 3.94; N, 5.67. ESI-HRMS (CH3OH, m/z): 728.9393 [M − Br]+ (calc. for C26H32BrN4NiO2Se2: 728.9323).
Ethylene oligomerization
All ethylene oligomerization tests were performed in a 300 mL double-walled stainless Parr reactor equipped with mechanical stirring, internal temperature control and continuous feed of ethylene. The Parr reactor was dried in an oven at 120 °C for 5 h prior to each run, and then placed under vacuum until at room temperature. A typical reaction was performed by introducing toluene (90 mL) and an appropriate amount of the cocatalyst into the reactor under an ethylene atmosphere. After 10 min, the toluene catalyst solution (10 mL, [Ni] = 6.5 μmol) was injected into the reactor under a stream of ethylene and then the reactor was immediately pressurized. Ethylene was continuously fed in order to maintain the ethylene pressure. After the desired time, the reaction was stopped by cooling the system to −60 °C and depressurizing. An exact amount of cyclohexane was introduced, as internal standard, and the mixture was analysed by quantitative GLC.
Single-crystal X-ray diffraction of Ni4·PhMe
Single crystals of Ni4·PhMe suitable for X-ray analysis were obtained from concentrated toluene solutions at room temperature. A Bruker CCD X8 Kappa APEX II diffractometer outfitted with a graphite monochromator and Mo-Kα radiation (λ = 0.71073 Å) was used to collect X-ray data for the structural analysis of Ni4·PhMe. A combination of ϕ and ω scans was carried out to obtain at least one unique data set. The crystal structure of the compound was solved using direct methods in the SHELXS program. The final structures were refined using SHELXL, where the remaining atoms were located from difference Fourier synthesis in which anisotropic displacement parameters were applied to all non-hydrogen atoms, followed by full-matrix least-squares refinement based on F2. All hydrogen atoms were placed in ideal positions and refined as riding atoms with relative isotropic displacement parameters. Additional structural information is provided in Table 1. Selected bond lengths and angles for Ni4·PhMe are presented in Table 2.
Acknowledgements
This work was supported in part by the Petrobras S/A. L. C. Dresch and Bruno B. de Araújo gratefully acknowledge CAPES and FAPERGS for the fellowship.
Notes and references
-
(a) S. M. Pillai, M. Ravindranathan and S. Sivaram, Chem. Rev., 1986, 86, 353 CrossRef CAS;
(b) J. Skupinska, Chem. Rev., 1991, 91, 613 CrossRef CAS;
(c) S. Wang, W.-H. Sun and C. Redshaw, J. Organomet. Chem., 2014, 751, 717 CrossRef CAS;
(d) P.-A. R. Breuil, L. Magna and H. Olivier-Bourbigou, Catal. Lett., 2015, 145, 173 CrossRef CAS;
(e) A. M. Al-Jarallah, J. A. Anabtawi, M. A. B. Siddiqui, A. M. Aitani and A. W. Al-Sa'doun, Catal. Today, 1992, 14, 1 CrossRef.
-
(a) P. W. N. N. Van Leeuwen, Homogeneous Catalysis, Kluwer Academic, Inc., Dordrecht, 2004, p. 175 Search PubMed;
(b) D. Vogt, Applied Homogeneous Catalysis with Organometallic Compounds, Wiley-VCH, Weinheim, Germany, 2000, p. 245 Search PubMed.
-
(a) C. Bianchini, L. Gonsalvi, W. Oberhauser, D. Sémeril, P. Brüggeller and R. Gutmann, Dalton Trans., 2003, 3869 RSC;
(b) N. A. Cooley, S. M. Green, D. F. Wass, K. Heslop, A. G. Orpen and P. G. Pringle, Organometallics, 2001, 20, 4769 CrossRef CAS;
(c) J. N. L. Dennett, A. L. Gillon, K. Heslop, D. J. Hyett, J. S. Fleming, C. E. Lloyd-Jones, A. G. Orpen, P. G. Pringle, D. F. Wass, J. N. Scutt and R. H. Weatherhead, Organometallics, 2004, 23, 6077 CrossRef CAS.
-
(a) S. Zhang, R. Pattacini, S. Jiea and P. Braunstein, Dalton Trans., 2012, 379 RSC;
(b) A. Mukherjee, U. Subramanyam, V. G. Puranik, T. P. Mohandas and A. Sarkar, Eur. J. Inorg. Chem., 2005, 1254 CrossRef CAS;
(c) W. Keim, S. Killat, C. F. Nobile, G. P. Suranna, U. Englert, R. Wang, S. Mecking and D. L. Schröder, J. Organomet. Chem., 2002, 662, 150 CrossRef CAS;
(d) Z. Guan and W. J. Marshall, Organometallics, 2002, 21, 3580 CrossRef CAS;
(e) F. Speiser, P. Braunstein and L. Saussine, Organometallics, 2004, 23, 2625 CrossRef CAS;
(f) F. Speiser, P. Braunstein and L. Saussine, Organometallics, 2004, 23, 2633 CrossRef CAS;
(g) A. Kermagoret and P. Braunstein, Organometallics, 2008, 27, 88 CrossRef CAS;
(h) J. Flapper, H. Kooijman, M. Lutz, A. L. Spek, P. W. N. M. van Leeuwen, C. J. Elsevier and P. C. J. Kamer, Organometallics, 2009, 28, 1180 CrossRef CAS;
(i) L. O. de la Tabla, I. Matas, P. Palma, E. Álvarez and J. Cámpora, Organometallics, 2012, 31, 1006 CrossRef.
-
(a) Z. J. A. Komon, X. Bu and G. C. Bazan, J. Am. Chem. Soc., 2000, 122, 12379 CrossRef CAS;
(b) J. Heinicke, M. Köhler, N. Peulecke, M. He, M. K. Kindermann, W. Keim and G. Fink, Chem.–Eur. J., 2003, 9, 6093 CrossRef CAS PubMed;
(c) S. D. Ittel, L. K. Johnson and M. Brookhart, Chem. Rev., 2000, 100, 1169 CrossRef CAS PubMed;
(d) P. Kuhn, D. Sémeril, D. Matt, M. J. Chetcuti and P. Lutz, Dalton Trans., 2007, 515 RSC;
(e) J. Heinicke, M. He, A. Dal, H.-F. Klein, O. Hetche, W. Keim, U. Flörke and H.-J. Haupt, Eur. J. Inorg. Chem., 2000, 431 CrossRef CAS.
-
(a) X. Tang, W.-H. Sun, T. Gao, J. Hou, J. Chen and W. Chen, J. Organomet. Chem., 2005, 690, 1570 CrossRef CAS;
(b) E. Nelkenbaum, M. Kapon and M. S. Eisen, Organometallics, 2005, 24, 2645 CrossRef CAS.
-
(a) C. Carlini, M. Isola, V. Liuzzo, A. M. R. Galletti and G. Sbrana, Appl. Catal., A, 2002, 231, 307 CrossRef CAS;
(b) S. Wu and S. Lu, Appl. Catal., A, 2003, 246, 295 CrossRef CAS;
(c) J. Hou, W.-H. Sun, D. Zhang, L. Chen, W. Li, D. Zhao and H. Song, J. Mol. Catal. A: Chem., 2005, 231, 221 CrossRef CAS;
(d) L. Wang, W.-H. Sun, L. Han, Z. Li, Y. Hu, C. He and C. Yan, J. Organomet. Chem., 2002, 650, 59 CrossRef CAS.
-
(a) A. H. D. P. S. Ulbrich, R. R. Campedelli, J. L. S. Milani, J. H. Z. dos Santos and O. L. Casagrande Jr, Appl. Catal., A, 2013, 453, 280 CrossRef CAS;
(b) A. H. D. P. S. Ulbrich, A. L. Bergamo and O. L. Casagrande Jr, Catal. Commun., 2011, 16, 245 CrossRef CAS;
(c) L. L. Oliveira, R. R. Campedelli, M. C. A. Kuhn, J.-F. Carpentier and O. L. Casagrande Jr, J. Mol. Catal. A: Chem., 2008, 288, 58 CrossRef;
(d) N. Ajellal, M. C. A. Kuhn, A. D. G. Boff, M. Hörner, C. M. Thomas, J.-F. Carpentier and O. L. Casagrande Jr, Organometallics, 2006, 25, 1213 CrossRef CAS;
(e) L. L. Oliveira, R. R. Campedelli, A. L. Bergamo, A. H. D. P. Santos and O. L. Casagrande, J. Braz. Chem. Soc., 2010, 21, 1318 CrossRef.
- A. C. Pinheiro, A. H. Virgili, T. Roisnel, E. Kirillov, J.-F. Carpentier and O. L. Casagrande Jr, RSC Adv., 2015, 5, 91524 RSC.
-
(a) A. S. Sigeev, A. S. Peregudov, A. V. Cheprakov and I. P. Beletskaya, Adv. Synth. Catal., 2015, 357, 417 CrossRef CAS;
(b) Q. Yao, E. P. Kinney and C. Zheng, Org. Lett., 2004, 6, 2997 CrossRef CAS PubMed;
(c) A. Kumar, M. Agarwal and A. K. Singh, Polyhedron, 2008, 27, 485 CrossRef CAS;
(d) F. Saleem, G. K. Rao, A. Kumar, S. Kumar, M. P. Singh and A. K. Singh, RSC Adv., 2014, 4, 56102 RSC.
- B. J. Khairnar, S. Dey, V. K. Jain and B. M. Bhanage, Tetrahedron Lett., 2014, 55, 716 CrossRef CAS.
-
(a) A. Budhai, B. Omondi, S. O. Ojwach, C. Obuah, E. Y. Osei-Twum and J. Darkwa, Catal. Sci. Technol., 2013, 3, 3130 RSC;
(b) N. Baho and D. Zargarian, Inorg. Chem., 2007, 46, 7621 CrossRef CAS PubMed;
(c) S. M. Nelana, J. Darkwa, I. A. Guzei and S. F. Mapolie, J. Organomet. Chem., 2004, 689, 1835 CrossRef CAS;
(d) M. K. Ainooson, S. O. Ojwach, I. A. Guzei, L. C. Spencer and J. Darkwa, J. Organomet. Chem., 2011, 696, 1528 CrossRef CAS;
(e) S. O. Ojwach, I. A. Guzei, L. L. Benade, S. F. Mapolie and J. Darkwa, Organometallics, 2009, 28, 2127 CrossRef CAS.
- A. Panda, S. C. Menon, H. B. Singh, C. P. Morley, R. Bachman, T. M. Cocker and R. J. Butcher, Eur. J. Inorg. Chem., 2005, 1114 CrossRef CAS.
- S. Trofimenko, Scorpionates: The Coordination Chemistry of Polypyrazolylborate Ligands, Imperial College Press, London, 1999 Search PubMed.
- T. N. Sorrell and M. R. Malachowski, Inorg. Chem., 1983, 22, 1883 CrossRef CAS.
-
(a) S. Julia, C. Martinez-Martorell and J. Elguero, Heterocycles, 1986, 24, 2233 CrossRef CAS;
(b) A. R. Katritzky and J. N. Lam, Can. J. Chem., 1989, 67, 1144 CrossRef CAS.
- G. M. Sheldrick, Acta Crystallogr., Sect. A: Found. Crystallogr., 2008, 64, 112 CrossRef CAS PubMed.
Footnote |
† Electronic supplementary information (ESI) available: 1H and 13C NMR spectra of the prepared ligands; HRMS of the Ni complexes; CIF file for Ni4·PhMe. CCDC 1474431. For ESI and crystallographic data in CIF or other electronic format see DOI: 10.1039/c6ra18987c |
|
This journal is © The Royal Society of Chemistry 2016 |
Click here to see how this site uses Cookies. View our privacy policy here.