DOI:
10.1039/C6RA16232K
(Paper)
RSC Adv., 2016,
6, 79900-79909
Janus films with stretchable and waterproof properties for wound care and drug delivery applications†
Received
22nd June 2016
, Accepted 21st July 2016
First published on 18th August 2016
Abstract
A commercial bandage provides a quick solution to small cuts and bruises by absorbing excess blood and covering the wound. In this study, we propose a type of medical dressing using a polydimethylsiloxane (PDMS) membrane with significant potential to improve wound healing conditions. A thin soft Janus PDMS film with opposing porous and nonporous faces is fabricated and tested for qualities applicable to improve current bandages, such as the porosity, stretchability, and water-wettability. The PDMS film can be stretched to 150% of its original length to allow the bending of fingers or joints. The nonporous face of the PDMS film provides a natural waterproofing barrier against intrusion by microbes and contaminants. The porous face of the bandage with pore size between 100 μm and 400 μm provides abundant void space for the preloading of pharmaceuticals and allows a relatively quick drug release (over 50% in 5 min, over 80% in 30 min), while simultaneously improving water vapor permeability. Blood absorbed could be distributed within the film's pore network, reducing the chances of forming a large clot and hardened scab that may break the wound bed upon removal. All these improvements demonstrate that the Janus PDMS film will provide the performance needs as a new bandage for wound care.
1. Introduction
Skin trauma resulting from physical or thermal damage is hard to avoid in everyday life. Minor trauma, such as small cuts or mild burns, can heal on their own or through simple treatment with wound care products like band-aids, ointments or hydrogels. Major skin damage that involves wounds with large surface areas or deep injuries and chronic wounds, including diabetic foot ulcers,1 burns2 and post-operative wounds, generally require more careful management. Postponed and improper wound treatment may result from nutritional deficiency3 or poor circulation4 and bring about severe infection by microorganism intrusion,5,6 all of which may impede wound healing and turn acute wounds into chronic, non-healing wounds. This will further increase management costs, negatively affect the patient's quality of life and even cause life threatening complications.7–9
Wound bandages or dressings are kinds of engineered materials or composites applied on the wound area, which are widely used in wound treatment to create an appropriate environment for healing.10–12 Wound dressings should not only form a barrier for protecting the injury site from further exterior harm, but further help achieve a rapid tissue repair by providing pharmaceuticals such as antibiotics, growth factors, vitamins and other beneficial agents that play an active role in the healing process. Generally, dressings are composed of two parts: the one which directly contacts the wound surface is referred to as the primary dressing while secondary dressings cover the primary dressing to support and hold it in place.10 Primary dressings are basically materials with the ability to absorb liquid and can also release preloaded drugs when applied. Hydrocolloids,13,14 alginates15 and hydrogels16 are alternative dressing materials that can be used for wounds with low to high exudate, according to their different absorbent capabilities and moisture-retaining properties. However, since most of these dressings are viscous and have low mechanical strength, they usually require a secondary covering.10,15 This may make such dressings difficult to handle and partly affects patient compliance.
Polymeric matrices with porous structures, such as porous polyurethane,17–19 are one type of promising dressing material, also referred to as a foam or sponge dressing. Due to the highly connected porous structure, foam/sponge dressings are good absorbents and the absorbency can be adjusted by manipulating sponge properties such as pore size and thickness. The open pore structure also facilitates moisture vapor transmission, making it suitable for most wounds with different amounts of exudate. When compared to gel dressings, foam/sponge dressings have a higher mechanical strength and resilience, which allows them to cushion and protect the wound area from further damage. The good strength also proves useful in negative pressure wound therapy.20,21 Nevertheless, a secondary covering such as a hydrophobic backing is usually necessary to prevent exterior leakage of medicine with exudate and serve as a barrier against bacteria and contaminants.
Considering the above issues, we proposed an engineered dressing that incorporates both a porous polymeric matrix and a non-porous layer into a single film sheet to potentially improve dressing performance, comfort and practicality in wound care. Composite materials with similar bilayer structure that are composed of an outer silicone layer and an inner collagen layer were reported as a long-term skin substitute.22,23 In our work, silicone elastomer polydimethylsiloxane (PDMS) was selected as a base material of the dressing. As a commercial elastomer, PDMS is commonly used in biotechnology due to its biocompatibility and physical properties, providing a safe, non-biodegradable and reliable substrate for use in biomaterials and their applications.24–26 Examples of biomedical PDMS applications include mammalian aorta repair,27 improvement of Schwann cell migration in nerve repair,28 and long term medical implants like breast implants.29,30 PDMS-based silicones have been used as drug delivery31,32 and release platforms33 due to their ability to hold several types of antibiotics both within and on the polymer surface.34 PDMS also provides other advantageous properties. For example, the mechanical properties of PDMS such as elasticity can be modified by changing the silicone base and curing agent ratio. This makes PDMS a stretchable material that can expand and contract with the natural bends of a finger or joints, contrary to typical woven-cloth bandages. Additionally, the hydrophobic property of PDMS, created through the repulsion of siloxane groups and water molecules, provides a durable waterproofing feature.35 Furthermore, PDMS is known to be permeable to oxygen and water vapor.36,37 These qualities together make PDMS a suitable and reliable bandage platform for use in wound care.
In this study, Janus PDMS films containing a porous structure on one face and a flat, non-porous structure on the other face were easily fabricated through a simple template method. The unique structure of the PDMS films created dressings with suitable properties for wound care applications. For this goal, relevant investigations on the PDMS films were carried out.
2. Results and discussion
2.1 Fabrication of Janus PDMS film
Fig. 1 schematically illustrated the fabrication process of the Janus PDMS film. To create pores in the thin film, a template was made by firmly pressing salt crystals and forming a relatively even distribution on the adhesive side of tape. PDMS prepolymers of a 10
:
1 mixing ratio (elastomer base to curing agent) were poured and spread onto the template. After being degassed under a vacuum, the prepolymer was cured at 75 °C for 2 h. The solidified PDMS film was then peeled off from the tape and immersed in water to dissolve the embedded salts. Finally, the Janus film, with one porous face and one non-porous face, could be obtained after drying in oven. By using this method, an integrated Janus film could be simply and reproducibly fabricated and the thickness of the porous structure could be controlled by adjusting thickness of the salt layer. In this work, PDMS films with thicknesses of 0.7 mm and 1.5 mm were fabricated.
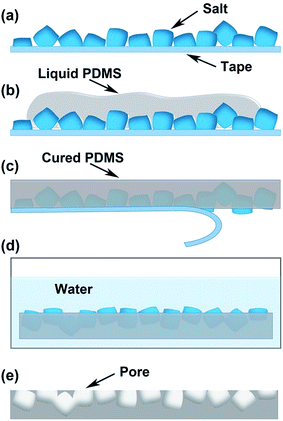 |
| Fig. 1 Schematic illustration of the fabrication process of Janus PDMS films, showing how the porous and non-porous surfaces of the film are formed. | |
2.2 Characterization of Janus PDMS film
2.2.1 Morphology and porosity. Morphologies of both thin (0.7 mm) and thick (1.5 mm) films were observed using a scanning electron microscope (SEM). As shown in Fig. 2a, there were pores of different sizes and various apertures distributed on one surface for both thin and thick films, whereas the contrary surfaces are flat and non-porous. The pore sizes ranged from 100 μm to 400 μm. Deeper morphologies of the films underneath the surface were also shown from the cross sectional view. It could be seen that the porous network within the thick film (the porous layer was about 1.32 mm thick) was about three-fold compared to that of the thin one (the porous layer was about 0.43 mm thick). These pores appeared to be interconnected, forming a sponge-like structure.
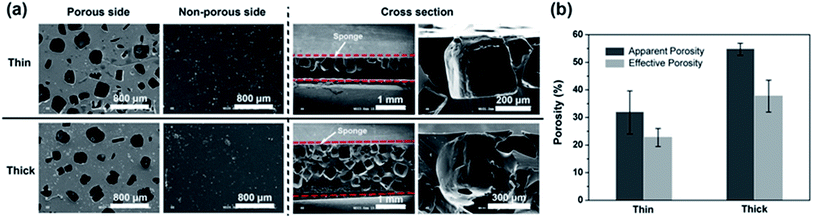 |
| Fig. 2 (a) Structural observation of Janus PDMS films by SEM. (b) Porosity characterization of Janus PDMS films. The thickness is 0.7 mm for thin films and 1.5 mm for thick ones. | |
To quantify the pore volume in the films and further characterize the extent of pore interconnectivity, the apparent porosity and the effective porosity (Fig. 2b) of the films were determined through a water saturation method. Porosities of the thin film and the thick film were 31.8% and 54.7% respectively, indicating that almost one third volume in the thin film or more than half of the total volume in the thick film was void space of pores. However, not all the void space could be used as reservoirs for drug loading due to the incomplete pore-interconnectivity and incomplete air exclusion of the films. Effective porosity, representing the fraction of “accessible void” in films, was about 22.8% for the thin film and 37.7% for the thick one. The sponge-like structure in the Janus PDMS films provided enough room to incorporate materials of interest like therapeutics.
2.2.2 Stretchability. Unlike conventional woven and nonwoven textiles used in band-aids, the PDMS films in this work exhibited high flexibility and stretchability because of the elastic nature of PDMS polymer itself and the sponge structure. Fig. 3a showed the images of different PDMS films at relaxation and stretched states. All the PDMS strips could readily be stretched. A higher stretchability could be obtained by decreasing the ratio of curing agent (comparing thin 10
:
1 with thin 20
:
1). While at the same curing agent ratio, the thick film exhibited a stretchability comparable to the thin film (comparing thick 10
:
1 with thin 10
:
1). That could be attributed to the higher proportion of loosened porous structure in the thick film.
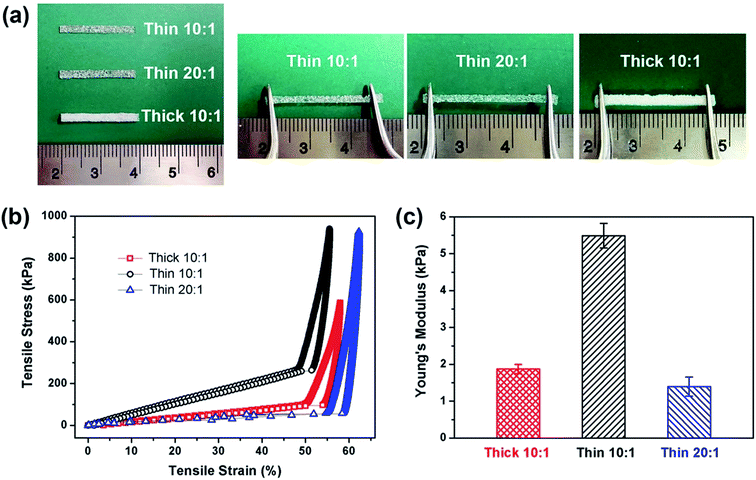 |
| Fig. 3 Tensile properties of Janus PDMS films. (a) Images of Janus films at relaxation (first image on left) and at stretching states (three smaller images). (b) Stress vs. strain curves for Janus films subjected to tension tests. (c) Young's moduli of Janus films based on tension tests. | |
Cycled stress–strain curves of different films and their corresponding Young's moduli were measured using a thermochemical analyzer (TMA, Q400 from TA Instruments) and were presented in Fig. 3b and c. It was observed that all the film samples could easily reach an elongation of about 150% with a low tensile stress and recover to their original states after the stress was released. It was noted that there were inflection points in the stress–strain curves, indicating that the stress increased sharply when the films were stretched to a certain extent. This was probably ascribed to the double-layer structure of Janus films which generated a non-uniform stress–strain state. Before the inflection point, the force was mainly loaded to the nonporous layer because the porous layer was more elastic and deformed more easily, but once the porous layer reached its limit of free deformation, both layers started to bear the force and caused a stress increase. Similar results were found in materials consisting of different components or structures.38,39 The Young's moduli of samples were about 1.9 kPa for thick 10
:
1, 5.5 kPa for thin 10
:
1 and 1.4 kPa for thin 20
:
1. Interestingly, the thick PDMS film with a high curing agent ratio possessed a lower Young's modulus than the thin PDMS with low curing agent ratio. This demonstrated that the high proportion of pores in the thick film caused a large stretching strain at a low tensile stress.
2.2.3 Water-wettability. Janus structures can create distinct properties on different surfaces in one material.40,41 The unique structure of the obtained PDMS film made its property changeable including surface water-wettability. As shown in Fig. 4a, the non-porous surface of the film was water-resistant, owing to the inherent hydrophobic properties of PDMS. The porous face was able to load various drugs and also able to generate distinct water wettability on its surface. To investigate the surface wettability of the film, water contact angles (CAs) were measured before and after incorporation of a model antiseptic benzalkonium chloride (BAC) into the porous surface. Fig. 4b showed the CA images on both film surfaces with and without BAC. For the non-porous surface, BAC incorporation did not alter its wettability, and all the CAs were above 105° (Fig. 4c).
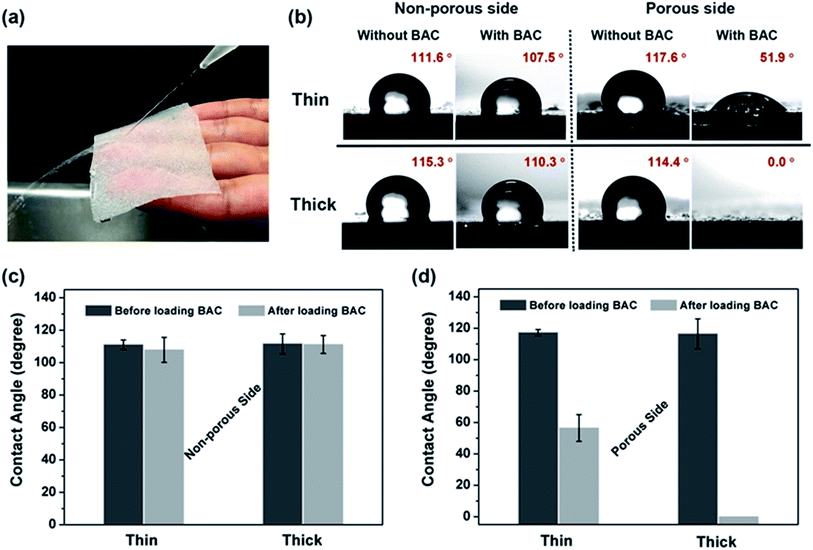 |
| Fig. 4 Surface water wettability of Janus PDMS films. (a) Optical image of a jet of water bouncing off the non-porous surface of a Janus film. (b) Water contact angle measurements on Janus films before and after incorporation of benzalkonium chloride (BAC). (c & d) Values of contact angles according to the measurement in (b). | |
However, on the porous face, the wettability was significantly improved after loading BAC (Fig. 4b and d). Before drug loading, the porous face exhibited high unwettability with the CA value slightly higher than that of the non-porous face. This was likely attributed to the intensified the hydrophobicity42 by increased surface roughness resulted from the porous structure, as opposed to the flat nonporous surface. Water drops on the surface might be on the Cassie state because of the inherent hydrophilicity of PMDS and the air-containing pores.43,44 After being loaded with BAC, the porous surface changed into water-wettable, wherein BAC played a critical role. BAC is a cationic surfactant and has been widely used as a biocide. It is also a useful excipient to aid drug dissolution and increase drug penetration. When BAC was loaded in PDMS film, its hydrophobic part was prone to adsorbing onto pore walls through hydrophobic interactions, leaving its hydrophilic part extending on PDMS surface.45,46 This facilitated the subsequent water infiltration into the pores and water spreading on the surface which consequently led to the change of surface property from hydrophobic to hydrophilic in appearance. And the wetting state accordingly turned to the Wenzel-state.43,44 The CA on the porous side with BAC was about 56° for the thin film but 0° for the thick one as the whole water droplet was absorbed into its thicker porous layer (Fig. 4b and d).
2.3 Drug incorporation and release
To further investigate drug loading and release capability of the film, methylene blue (MB) was introduced into the pores for visual observation. Both thin and thick films with or without MB were observed under an optical microscope. As shown in Fig. 5a, MB could absorb onto the pore walls and be separated evenly in the pores. The release of incorporated MB was observed according to the color change on gelatin hydrogels with time. Gelatin was chose for the test because it was widely used to mimic skin tissue and extracellular matrix in tissue engineering47,48 (Fig. 5b). It was noted that the blue color intensified from 5 min to 2 hours after placing the MB-containing film on the gels, indicating MB was continuously released and permeated into the gel matrix with time.
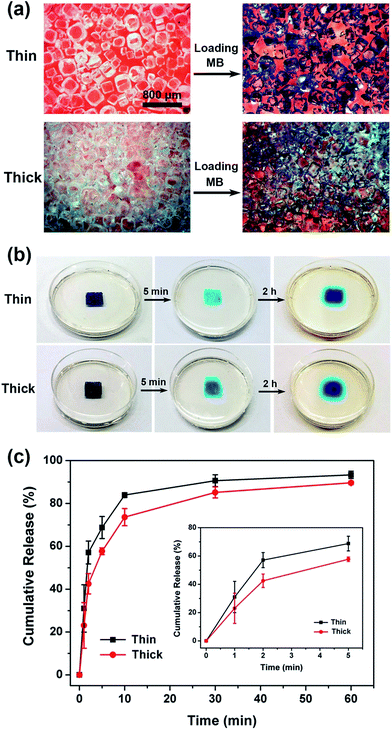 |
| Fig. 5 Investigation of Janus PDMS films for drug loading and release. (a) Optical images of Janus films before and after incorporation of methylene blue (MB). (b) Release observation of MB-contained Janus films on gelatin hydrogels. (c) BAC release from Janus films in PBS solution (the insert is the enlarged BAC release profiles during the first five min). | |
In addition to the direct observation of drug release, BAC release behaviors in phosphate buffered saline (PBS) were also characterized at room temperature. The cumulative release profiles of BAC shown in Fig. 5c indicated that both thin and thick films released over 50% of the loaded BAC in 5 min and over 80% after 30 min. The rapid release of antiseptics like BAC is needed in wound dressing applications to ensure a “clean” environment for wound healing.
The porous structure in the Janus film could offer abundant room and specific surface for incorporation of not only pure drugs as shown above but also formulated drugs. Additionally, PDMS surface can be easily functionalized by chemical modification to bond drugs via different mechanisms,49,50 even other small drug carriers like liposomes, polymeric micelles or nanoparticles.51 Other materials such as hydrogels52,53 are also able to be introduced into the pores to encapsulate drugs or increase moisture. The great potential of surface functionalization for PDMS may impart more possibilities to the Janus films to load and release different drugs according to practical needs.
2.4 In vitro antimicrobial activity
Antibacterial performances of the PDMS film with different amounts of BAC were next evaluated using the Kirby–Bauer disc diffusion assay. As test bacterium, we evaluated the Gram-positive Staphylococcus aureus (S. aureus) and Gram negative Escherichia coli (E. coli) that are a common cause of wound associated infections. Discs made from the PDMS Janus films were loaded with varying amounts of BAC and placed in contact with agar plates inoculated with the corresponding bacterium (Fig. 6a). The positive controls were the filter paper discs loaded with either BAC or antibiotics kanamycin (Kan) or ampicillin (Amp).
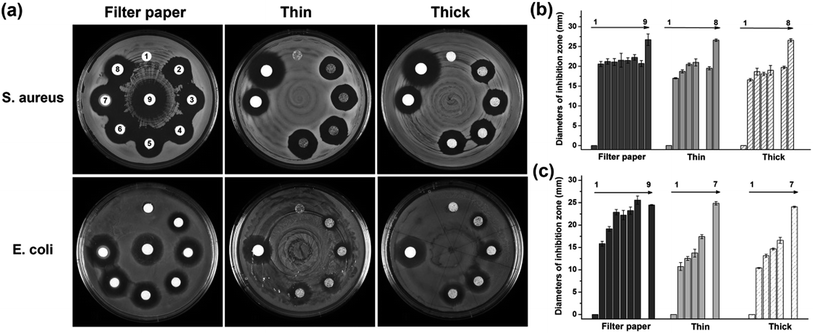 |
| Fig. 6 Antibacterial evaluation of BAC-containing Janus PDMS films against S. aureus and E. coli. (a) Representative images of agar plates seeded with either S. aureus or E. coli and incubated with drug-loaded filter paper and Janus film discs. The diameter comparison of inhibition zones produced, presented as dark circles, after 24 hours, for (b) S. aureus and (c) E. coli. Generally, position 1 is control (no drug was contained) with increasing amounts of BAC located in clockwise positions (details in ESI Table S1†). | |
The inhibition of bacterial growth as shown in Fig. 6a was observed for all drug-containing discs after 24 hours of incubation. The inhibitory effect was assessed and compared by the diameters of those inhibition zones, which were presented in Fig. 6b and c. The BAC-containing PDMS films demonstrated an efficient suppression of S. aureus growth and presented a comparable inhibition zone size to that of BAC-loaded filter paper discs. There was no inhibition for blank discs, indicating the filter paper had no antibacterial activity. The discs with the lowest quantity of BAC appeared to be equally effective at inhibiting S. aureus, as the zone of inhibition did not markedly increase with dose on either thin or thick PDMS films. In contrast, the inhibitory effect of BAC against E. coli appeared to be proportional to the BAC dosage. When compared with the filter paper controls, the discs of BAC-containing PDMS film exhibited a relatively slower, but still proficient drug release properties. This demonstrated that an effective antibacterial performance could be achieved with the PDMS Janus films.
2.5 Janus PDMS film as bandage materials
In order to assess the Janus PDMS film as a bandage or dressing material, we compared the performance of the PDMS film with commercially available adhesive-type bandages in practical flex/stretchability, moisture permeability and blood clot characteristics.
2.5.1 Bending test at finger joint. Common adhesive bandages usually consist of a cotton-gauze pad on an adhesive tape made of woven textile. When in use, the cotton pad is to be applied over the wound area and held in place by the tape. However, most adhesive bandages are inelastic and have a limited stretchability. When being applied on the skin, traditional bandages do not comply well with the skin deformation during muscle movement, especially around fingers or other joints, and impacting upon patients' comfort to some extent.In this part, finger motilities with a commercial bandage and the PDMS film were estimated by a qualitative finger flexion test (Fig. 7a and b). The subject reported that the finger covered with a traditional bandage encountered significant resistance during the flex test, while the same finger covered with the PDMS film could bend freely. The enhanced joint flexibility by the soft and elastic PDMS bandages may highly improve the compliance of patients and their ability to perform daily activities.
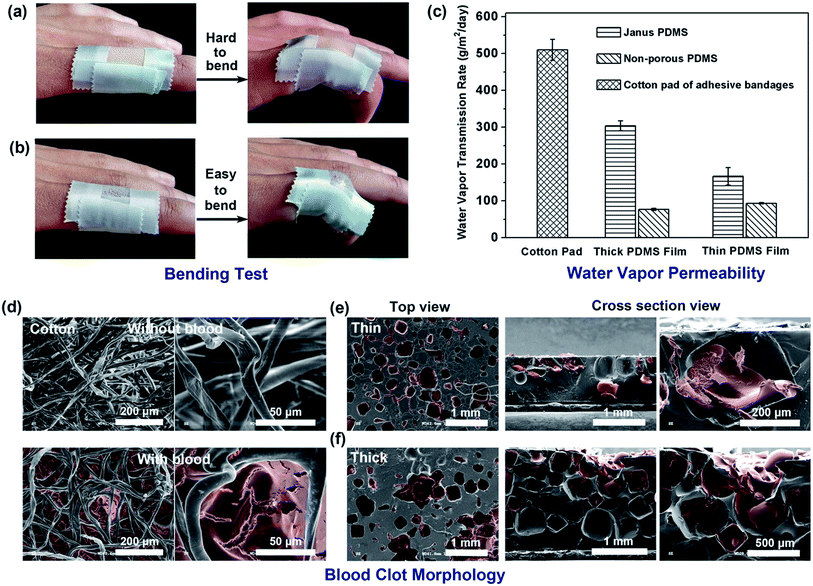 |
| Fig. 7 Assessment of Janus PDMS films as a bandage material by comparison with commercialized band-aid bandages. (a & b) Bending test on different bandages at finger joints. (c) Moisture vapor permeability of the cotton pad in a band-aid bandage and different PDMS films. (d–f) Morphologies of rabbit blood clots on cotton pads and PDMS films (the blood clots were stained in red). | |
2.5.2 Water vapor permeability. A moist wound environment has positive effects on promoting wound healing because it facilitates the formation of renewed skin instead of a scab that may lead to scarring. A desirable wound dressing should enable control of water loss and provide adequate moisture at the wound site to prevent wound dehydration. Therefore, the water vapor transmission rates (WVTRs) were next compared among the Janus PDMS films, commercialized adhesive bandages and PDMS films with the same thickness.As shown in Fig. 7c, the cotton pad, from an adhesive bandage, which has a fully opened fibrous network possessed the highest WVTR of about 510 g per m2 per day, followed by thick and thin Janus PDMS films with a WVTR of 304 and 167 g per m2 per day, respectively, whereas non-porous PDMS films showed limited WVTRs of about 76 g per m2 per day for thick ones and 93 g per m2 per day for thin ones. This indicated that the sponge-like structures in the Janus PDMS films could significantly enhance water vapor permeability due to the interconnected pores that can decrease the material barriers for vapor transport and facilitate the lateral diffusion of water molecules. That was why the thick Janus film possessed a higher WVTR than the thin film. Since different wounds may have different exudation rates, and even for same wounds the exudation rate may vary at different healing stages, a wound dressing with a proper WVTR has to be considered according to the wound type and conditions to create an optimal healing environment in practical applications.
2.5.3 Blood clot morphology on cotton and Janus PDMS film. Wound dressings or bandages made from cotton have a high affinity with blood components that may lead to formation of large, integrated clots due to excess blood absorbed in cotton pads. Furthermore, the shape and texture of the cotton-based dressing would be changed by the drying clots when they shrink and become stiff. Importantly, the dressing fibers would become entangled with the dried clot and adhere to the wound bed, resulting in further bleeding and tissue damage upon dressing removal.Fig. 7d–f showed the morphologies of rabbit blood clots within cotton pads and PDMS porous films. As presented in Fig. 7d, the cotton fiber network was fully filled by the blood clots, forming an entire bulk.
However, the Janus PDMS film is different from conventional cotton or gauze dressings. With a sponge structure, the PDMS film can absorb and disperse excess blood into its pores to avoid forming large clots. Besides, the PDMS film can keep it shape and softness even with dried blood clots because it has a higher rigidity than cotton fibers. Finally, the risk of stickiness between wound tissue and dressing can also be decreased due to the low surface energy of PDMS. As shown in Fig. 7e and f, on the porous PDMS films, the blood clots were separated into pores and the blood clot pieces were prone to detachment and peeling from the pore wall. The low affinity between PDMS and blood or wound exudate may impart a non-stick ability and provide an easier, less painful removal for the porous PDMS film to better serve as a wound dressing.
3. Conclusion
Janus elastic and stretchable PDMS films of different thicknesses were produced through a facile, chemical solvent free template method. The Janus films include the major advantages of some other bandages with similar effects. Porous faces of the films can not only provide cavities for incorporation of therapeutic agents and absorption of blood/wound exudate but mediate moisture vapor permeability of the film as well. Non-porous faces can act as a barrier to resist water and possibly the invasion of microorganisms. Meanwhile the low surface energy of PDMS may also make the film nonadherent at the wound surface. These attractive properties of the PDMS film may pave the way for its application in wound care, especially as dressings for wounds with light to moderate exudates.
4. Experimental section
4.1 Preparation of Janus PDMS films
A piece of scotch tape was affixed to a glass slide with the adhesive face exposed. Sodium chloride (NaCl, Fisher Scientific) and granulated sugar were mixed at a 100
:
1 ratio with 1 μL distilled water per 1 g NaCl/sugar mixture and spread evenly over the tape. The spread NaCl/sugar mixture was firmly pressed and formed a thin layer on the tape surface after scraping off any excess mixture. Sylgard 184 silicone elastomer prepolymer was mixed with a curing agent at the weight ratio of 10
:
1 and poured over the glass slide until a 0.6 mm thin film of the PDMS was distributed evenly onto the crystal layer. Thick PDMS films were created using the same method listed above with the exception that 2 μL water per 1 g NaCl/sugar mixture was added during the mixing process. The height of the NaCl/sugar layer on the glass slide was about 1.4 mm.
The slide/PDMS assembly was then degassed under a vacuum and cured at 75 °C for 2 hours. After curing, the PDMS film was separated from the tape and set in water to dissolve the NaCl and sugar to obtain a Janus film with one flat and one porous surface.
In the film fabrication, NaCl was used to form pores with proper size and sugar was used as adhesive after mixing with water to bind NaCl particles together and create connected porous structure. Similar methods have also been used to create porous polymeric scaffolds.54
4.2 Porosity measurement
Samples of both the thin and thick PDMS films were cut into 20 × 20 mm2 pieces and weighed (Md). Weights of non-porous PDMS films with the same volume as the thin and the thick samples were calculated theoretically and recorded as Mt. The porosity of the porous film was determined as (Mt − Md)/Mt × 100%. The dried porous PDMS films were filled with distilled water in a vacuum and the water-saturated samples were weighed after gently wiping away any excess water on the surface. The effective porosity then could be determined by the volume of filled water (Vw) in the film and the relevant apparent volume of the film (Va) as Vw/Va × 100%.
4.3 Scanning electron microscope observation
A field emission scanning electron microscopy (SEM, Hitachi S-4800, Japan) was employed to observe the morphology of the Janus PDMS film. The morphologies of rabbit blood clots on cotton pads and PDMS films were also examined under SEM. All the samples were first coated with gold in vacuum and then observed using SEM. Blood of rabbits was provided by Vancouver General Hospital (Vancouver, Canada). The blood drawing protocol was approved by the UBC Animal Care Committee.
4.4 Cyclic tension test
Samples of the thin and thick PDMS films were cut into rectangular strips of 1 mm × 20 mm and tested using a Q400 Thermochemical Analyzer (TA Instruments). All samples were manipulated with an initial force of 0 N, a ramp force up to a maximum of 1 N by 0.2 N min−1, and a ramp force down to 0 N by 0.2 N min−1. The Young's modulus was calculated from the linear stress–strain region by fitting a straight line between 5 and 15% strain.
4.5 Contact angle test
The images of contact angles (CAs) on both sides of the PDMS film were taken before and after drug (benzalkonium chloride, BAC) loading using a stereo microscope (Olympus SZ61, Basler Vision Technologies). The values of CAs were then calculated using image analysis software ImageJ. All the samples were measured at ambient humidity and temperature. For each measurement, 6 μL of distilled water was dropped onto the sample surface and an image was taken after 10 s. The average CA value for each sample was obtained based on 5 values of CA at different locations.
4.6 Drug release on gelatin hydrogel and in PBS
The gelatin hydrogels with released MB were recorded by taking photos after different time intervals. For preparation of gelatin hydrogel, 4.5 g gelatin (type A from porcine skin) was dissolved in 50 mL distilled water and poured into 60 mm diameter Petri dishes at heights of 3 mm. The hydrogel was then obtained after solidification at room temperature. Thin and thick films were cut into 1 × 1 cm2 pieces for drug loading and release. Samples loaded with methylene blue (MB) were first observed under a stereo microscope, and then put onto wet gelatin hydrogels for a qualitative analysis of dye release behavior.
For quantitative analysis of drug release, samples were loaded with 0.15 and 0.30 mg benzalkonium chloride (BAC, Alfa Aesar), for the thin and thick films, respectively. BAC loaded samples were set in 1.4 mL of PBS for drug release. At different time points, 0.7 mL of the test solution was removed for analysis and 0.7 mL of fresh PBS was supplemented for continuous release. The UV absorbance at 250 nm of the PBS with released BAC was recorded using a UV-visible spectrophotometer (Varian Cary 50 Bio) and the amount of released BAC was calculated using the standard curve of BAC. The release process was observed and tested at room temperature about 23 °C.
Both MB and BAC were first dissolved in ethanol and then dispersed into the porous surface of the PDMS films. After ethanol was evaporated, the drug-loaded films were obtained. The amounts of substance in the film were controlled through the concentration and volume used.
4.7 In vitro Kirby–Bauer disk antibacterial analysis
Thin and thick films were cut into 0.8 mm diameter round discs and loaded with different amounts of BAC dissolved in ethanol. For the thin films, each disc contained 0, 0.05, 0.10, 0.15, or 0.3 mg BAC while for the thick films, each disc contained 0, 0.10, 0.20, 0.30, or 0.60 mg BAC. The ethanol was evaporated, and the BAC loaded discs were placed porous-face down onto Luria–Bertani (LB) agar plates inoculated by spreading with Staphylococcus aureus (S. aureus) or Escherichia coli (E. coli). The agar plates were incubated for 24 h at 37 °C and images taken to evaluate the zones of inhibition. As controls, filter paper discs (Whatman 3 MM chr) were loaded with 0, 0.05, 0.10, 0.15, 0.20, 0.30, 0.60 mg BAC or with 0.10 mg kanamycin (Kan, Sigma) or 0.01 mg ampicillin (Amp, Sigma).
4.8 Water-vapor transmission evaluation
Commercially available adhesive bandages (American White Cross) and thin and thick PDMS films with and without pores were used to seal vials with an opening diameter of 1.6 cm. Each vial contained 1 mL distilled water. The amount of water loss over time was measured by weighing the residual water to determine the moisture permeability of the different films. The porous surface was orientated toward the water containing chamber.
Acknowledgements
This work has been supported by an NSERC/CIHR CHRP Grant (CHRP 414087-12), NSERC Discovery Grant, Canada Foundation for Innovations and UBC's Institute for Computing, Information and Cognitive Systems (ICICS).
References
- W. J. Jeffcoate and K. G. Harding, Lancet, 2003, 361, 1545 CrossRef
. - D. Church, S. Elsayed, O. Rei, B. Winston and R. Lindsay, Clin. Microbiol. Rev., 2006, 19, 403 CrossRef PubMed
. - R. Greif, O. Akça, E. P. Horn, A. Kurz and D. I. Sessler, N. Engl. J. Med., 2000, 342, 161 CrossRef CAS PubMed
. - A. R. Siddiqui and J. M. Bernstein, Clin. Dermatol., 2010, 28, 519 CrossRef PubMed
. - D. Mackay and A. L. Miller, Alternative Med. Rev., 2003, 8, 359 Search PubMed
. - A. Gosain and L. DiPietro, World J. Surg., 2004, 28, 321 CrossRef PubMed
. - C. K. Sen, G. M. Gordillo, S. Roy, R. Kirsner, L. Lambert, T. K. Hunt, F. Gottrup, G. C. Gurtner and M. T. Longaker, Wound Repair Regen., 2009, 17(6), 763 CrossRef PubMed
. - L. M. Teague and J. L. Mahoney, Wound Care, 2004, 2(1), 32 Search PubMed
. - A. C. Tricco, E. Cogo, W. Isaranuwatchai, P. A. Khan, G. Sanmugalingham, J. Antony, J. S. Hoch and S. E. Straus, BMC Med., 2015, 13, 90 CrossRef PubMed
. - J. S. Boateng, K. H. Matthews, H. N. E. Stevens and G. M. Eccleston, J. Pharm. Sci., 2008, 97, 2892 CrossRef CAS PubMed
. - P. Zahedi, I. Rezaeian, S.-O. Ranaei-Siadat, S.-H. Jafari and P. Supaphol, Polym. Adv. Technol., 2010, 21, 77 CAS
. - J. G. Powers, L. M. Morton and T. J. Phillips, Dermatology and Therapy, 2013, 26, 197 CrossRef PubMed
. - S. G. Jin, K. S. Kim, A. M. Yousaf, D. W. Kim, S. W. Jang, M.-W. Son, Y. H. Kim, C. S. Yong, J. O. Kim and H.-G. Choi, Int. J. Pharm., 2015, 490, 240 CrossRef CAS PubMed
. - H.-E. Thu, M. H. Zulfakar and S.-F. Ng, Int. J. Pharm., 2012, 434, 375 CrossRef CAS PubMed
. - K. Y. Lee and D. J. Mooney, Prog. Polym. Sci., 2012, 37, 106 CrossRef CAS PubMed
. - L. Fan, H. Yang, J. Yang, M. Peng and J. Hu, Carbohydr. Polym., 2016, 146, 427 CrossRef CAS PubMed
. - L. A. Dessy, F. Serratore, F. Corrias, P. Parisi, M. Mazzocchi and B. Carlesimo, Int. Wound J., 2015, 12, 132 CrossRef PubMed
. - B. Li, K. V. Brown, J. C. Wenke and S. A. Guelcher, J. Controlled Release, 2010, 145, 221 CrossRef CAS PubMed
. - R. Ceesala, N. Bar, N. R. Dhoke, P. Basak and A. Das, Biomaterials, 2016, 77, 1 CrossRef PubMed
. - P. A. Blume, J. Walters, W. Payne, J. Ayala and J. Lantis, Diabetes Care, 2008, 31, 631 CrossRef PubMed
. - C. Huang, T. Leavitt, L. R. Bayer and D. P. Orgill, Curr. Probl. Surg., 2014, 51, 301 CrossRef PubMed
. - S. Suzuki, K. Kawai, F. Ashoori, N. Morimoto, Y. Nishimura and Y. Ikada, Br. J. Plast. Surg., 2000, 53, 659 CrossRef CAS PubMed
. - S. Aoki, T. Takezawa, S. Ikeda, Y. Narisawa, A. Oshikata-Miyazaki, S. Miyauchi, H. Hirayama, T. Sawaguchi, T. Chimuro and S. Toda, Wound Repair Regen., 2015, 23, 819 CrossRef PubMed
. - H. Zhang and M. Chiao, J. Med. Biol. Eng., 2015, 35, 143 CrossRef PubMed
. - A. Mata, A. J. Fleischman and S. Roy, Biomed. Microdevices, 2005, 7(4), 281 CrossRef CAS PubMed
. - F. N. Pirmoradi, J. K. Jackson, H. M. Burt and M. Chiao, Lab Chip, 2011, 11, 3072 RSC
. - X. Wang, M. P. Wolf, R. B. Keel, R. Lehner and P. R. Hunziker, J. Biomed. Opt., 2012, 17(7), 076006 Search PubMed
. - E. Jacchetti, I. Tonazzini, S. Meucci, F. Beltram and M. Cecchini, Microelectron. Eng., 2014, 124, 26 CrossRef CAS
. - A. U. Daniels, Silicone breast implant materials, Swiss Med. Wkly., 2012, 142, w13614 CAS
. - X. Thomas, in Silicones in Industrial Applications in Inorganic Polymers, ed. R. De Jaeger and M. Gleria, Nova Science Publishers, 2007, ch. 2 Search PubMed
. - M. Kajihara, T. Sugie, M. Mizuno, N. Tamura, A. Sano, K. Fujioka, Y. Kashiwazaki, T. Yamaoka, S. Sugawara and Y. Urabe, J. Controlled Release, 2000, 66, 49 CrossRef CAS PubMed
. - F. N. Pirmoradi, J. K. Jackson, H. M. Burt and M. Chiao, Lab Chip, 2011, 11, 2744 RSC
. - W. Kohnen, C. Kolbenschlag, S. Teske-Keiser and B. Jansen, Biomaterials, 2003, 24, 4865 CrossRef CAS PubMed
. - C. Tsai and C. Chang, J. Mater. Chem. B, 2013, 1, 5963 RSC
. - D. Yu, Y. Zhao, H. Li, H. Qi, B. Li and X. Yuan, Prog. Org. Coat., 2013, 76, 1435 CrossRef CAS
. - Y. Zhang, M. Ishida, Y. Kazoe, Y. Sato and N. Miki, IEEJ Trans. Electr. Electron. Eng., 2009, 4, 442 CrossRef CAS
. - T. C. Merkel, V. I. Bondar, K. Nagai, B. D. Freeman and I. Pinnau, J. Polym. Sci., Polym. Phys., 2000, 38, 415 CrossRef CAS
. - A. Danpinid, J. Luo, J. Vappou, P. Terdtoon and E. E. Konofagou, Ultrasonics, 2010, 50, 654 CrossRef CAS PubMed
. - T. Khamdaeng, J. Luo, J. Vappou, P. Terdtoon and E. E. Konofagou, Ultrasonics, 2012, 52, 402 CrossRef CAS PubMed
. - J. Du and R. K. O'Reilly, Chem. Soc. Rev., 2011, 40, 2402 RSC
. - H. Zhang, R. Hao, J. K. Jackson, M. Chiao and H. Yu, Chem. Commun., 2014, 50, 14843 RSC
. - L. Feng, S. Li, Y. Li, H. Li, L. Zhang, J. Zhai, Y. Song, B. Liu, L. Jiang and D. Zhu, Adv. Mater., 2002, 14(24), 1857 CrossRef CAS
. - L. Cao, T. P. Price, M. Weiss and D. Gao, Langmuir, 2008, 24, 1640 CrossRef CAS PubMed
. - C. Luo, M. Xiang, X. Liu and H. Wang, Microfluid. Nanofluid., 2011, 10, 831 CrossRef CAS
. - H. Sütterlin, R. Alexy and K. Kümmerer, Ecotoxicol. Environ. Saf., 2008, 71(2), 498 CrossRef PubMed
. - P. Wang and A. A. Keller, Environ. Toxicol. Chem., 2008, 27(6), 1237 CrossRef CAS PubMed
. - A. Karimi and M. Navidbakhsh, Biomed. Tech., 2014, 59(6), 479 CAS
. - J. Mao, L. Zhao, K. de Yao, Q. Shang, G. Yang and Y. Cao, J. Biomed. Mater. Res., Part A, 2003, 64(2), 301 CrossRef PubMed
. - J. Zhang, Q. Wang and A. Wang, Acta Biomater., 2010, 6, 445 CrossRef CAS PubMed
. - E. J. Anglin, L. Cheng, W. R. Freeman and M. J. Sailor, Adv. Drug Delivery Rev., 2008, 60, 1266 CrossRef CAS PubMed
. - J. S. Son, M. Appleford, J. L. Ong, J. C. Wenke, J. M. Kim, S. H. Choi and D. S. Oh, J. Controlled Release, 2011, 153, 133 CrossRef CAS PubMed
. - H. Zhang, C. Bian, J. K. Jackson, F. Khademolhosseini, H. M. Burt and M. Chiao, ACS Appl. Mater. Interfaces, 2014, 6, 9126 CAS
. - H. Zhang, J. K. Jackson, C. Bian, H. M. Burt and M. Chiao, Adv. Mater. Interfaces, 2015, 2, 1500154 CrossRef
. - X. Zhao, L. Li, B. Li, J. Zhang and A. Wang, J. Mater. Chem. A, 2014, 2, 18281 CAS
.
Footnote |
† Electronic supplementary information (ESI) available. See DOI: 10.1039/c6ra16232k |
|
This journal is © The Royal Society of Chemistry 2016 |