DOI:
10.1039/C6RA12364C
(Review Article)
RSC Adv., 2016,
6, 71827-71851
Recent developments in the chemistry of bicyclic 6-6 systems: chemistry of pyrido[4,3-d]pyrimidines
Received
12th May 2016
, Accepted 22nd July 2016
First published on 22nd July 2016
Abstract
The present review provides a study on the structure features, reactions and synthetic methodologies of pyrido[4,3-d]pyrimidines. 5,6,7,8-Tetrahydropyrido[4,3-d]pyrimidine and related compounds have been used as starting materials for the multi-step synthesis of tetrahydropteroic acid derivatives. The aim of this review is to give an overview of the diverse methodologies that have been reported on the chemistry of pyrido[4,3-d]pyrimidines. The different synthetic routes have been grouped according to the way the pyrido[4,3-d]pyrimidine moiety has been created. Thus, the investigated compounds were obtained from pyridine and pyrimidine derivatives or from multicomponent synthesis. The mechanistic pathways of the reactions and the important biological applications are discussed.
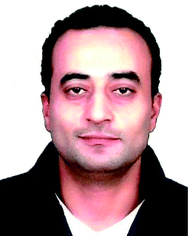 Khaled M. Elattar | Khaled M. Elattar was born in Menyet Sammanoud, Aga, El-dakahlia, Egypt (1979). He received his B.Sc. 2002 from Faculty of Science, Mansoura University, Egypt and M.Sc. 2006 from Faculty of Science, Benha University, Benha, Egypt. He obtained his PhD in organic chemistry 2011 from Faculty of Science, Mansoura University, Egypt (PhD thesis advisor: Prof. A. A. Fadda and Prof. A. S. Fouda). He is a Lecturer of Organic Chemistry, Faculty of Education, Sert University, Sert, Libya since 2012. He is a member of the Egyptian Chemical Society. He is a reviewer for some of international journals. His main research interests are in the field of organic synthesis of heterocyclic compounds of pharmaceutical interest, medicinal chemistry and synthesis of new porphyrins derivatives. |
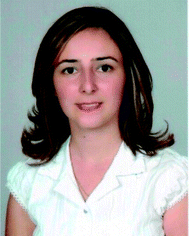 Başak Doğru Mert | Başak Doğru Mert was born in 1982 in Adana, Turkey. She received her B.Sc. 2004 from Chemistry Department of Science and Letters Faculty, Çukurova University, Turkey, M.Sc. 2007 and PhD 2012 in field of physical chemistry from Science and Letters Faculty of Çukurova University. She has been researcher in Çukurova University since 2006. Her research interests are anodizing, electrochemical synthesis and characterization of conducting polymers, investigation of corrosion inhibition effect of organic compounds on various metals, preparation of electro-catalytic electrode materials by electrochemical deposition method and applications for alkaline water electrolysis also electro-oxidation of organic molecules. |
1. Introduction
Pyrido[4,3-d]pyrimidines display various valuable biological activities, such as fungicidal, antiviral, anti-inflammatory, and antimicrobial properties.1–6 Some compounds have also been discovered as EGFr-TK inhibitors,7–9 or DHFR inhibitors,10 which stimulated our interest intensely. As is known so far, the reported syntheses of pyrido[4,3-d]pyrimidines usually involved the formation of a pyrimidine ring from a suitable substituted pyridine ring.11–13 However, the preparation of the substituted pyridine or the formation of the pyrimidine ring required relatively strict reaction conditions and long reaction times. A protocol via the aza-Wittig reaction has been developed for the construction of pyrido[4,3-d]pyrimidin-4-ones in order to find compounds with some particular biological functions.14 Recently, a highly efficient regioselective intramolecular ring closure reaction was also developed and utilized successfully for the synthesis of bioactive 4-methylene pyrido[4,3-d]pyrimidines.15–17 Pyrido[4,3-d]pyrimidines exhibited some interesting antitumor activity,18 which attracted our attention. As literatures described,9,11 this class of pyrido[4,3-d]pyrimidines are most likely to be the EGFr inhibitors. The reports ever pointed out that electron-donating groups in ortho-position of pyridine ring would contribute to the potency.8 So here we designed and synthesized a series of 4-methylene pyrido[4,3-d]pyrimidines, in which the electron-donating methoxy group was introduced to the ortho-position of pyridine and a variety of the substituents were introduced to the pyrimidine ring.
Propelled by our interest in the synthesis of medicinally active heterocyclic molecules,19–26 and in continuation of our previous reviews in the chemistry of pyridopyrimidines,27,28 we report herein this deep and schematic study about the chemistry and biological importance of pyrido[4,3-d]pyrimidine heterocyclic systems.
2. Spectroscopy
2.1. X-ray investigations
The single-crystal X-ray diffraction of 3-(4-fluorophenyl)-5,8,9-trimethyl-2-(p-tolyloxy)thieno [3′,2′:5,6]pyrido[4,3-d]pyrimidin-4(3H)-one showed that the C–S bond lengths are 1.730(2) Å and 1.744(2) Å, respectively.29 As expected for a nonprotonated ring system, the C1–N1–C9 angle of 117.17(16)° is smaller than 120°.30 The torsion angles C4–C3–C9–N1 and C9–C8–C5–S1 are 179.41(17)° and 179.89(13)°, respectively, showing the essential planarity of the tricyclic system. There is no intermolecular non-bonded interaction with fluorine or sulfur atom from the data and the crystal packing is stabilized by π–π stacking interactions and van der Waals forces.31
Recently, C27H22N3O2BrI2 and C27H19N3O2Br4I2·2CHCl3·2H2O salts were prepared by the iodination of 1,3-dimethyl-2,4-dioxo-5,6,7-R-1H,2H,3H,4H-pyrido[4,3-d]pyrimidinium bromides 1 and 2 (Fig. 1) with an equimolar amount of iodine. The structure of diiodine-bromide is composed of separate almost liner BrI2− and 1,3-dimethyl-2,4-dioxo-5,6,7-thriphenyl-1H,2H,3H,4H-pyrido[4,3-d]pyrimidinium cation. The crystal structure of 2 is built up of alternate layers of (C27H19N3O2Br3+)(I3−) and (Br2I−)(CHCl3), which are connected with each other by hydrogen bonds: Br(Br2I−)⋯H(C27H19N3O2Br3+). The formation of the ions I3− and Br2I− occurs via the disproportionation of I2Br− under the action of solvent on C27H19N3O2Br4I2·2CHCl3·2H2O crystallization.32
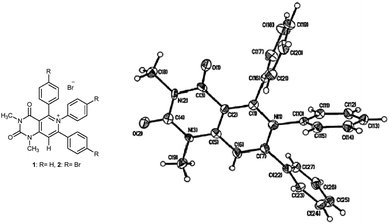 |
| Fig. 1 Molecular structure of pyrido[4,3-d]pyrimidinium salts. | |
2.2. Mass spectroscopy
The mass spectra of salts 2 are characterized by the cation in positive mode [ESI(+)-MS m/z, Ir (%) 657.89 [M]+ (100)]. Isotope cluster of the molecular ion is represented by four peaks, namely, m/z 653.89/655.89/657.89/659.89 (33/97/97/32). Negative electrospray mode led to cleavage of anion: ESI(−)-MS m/z Ir (%): 126.90 [I]− (98).32
2.3. NMR spectroscopy
Structural elucidation of the pyridopyrimidine-2-thiones was accomplished from 1D and 2D NMR spectroscopic data as described for 6-benzyl-8-benzylidene-4-(2,4-dichlorophenyl)-3,4,5,6,7,8-hexahydropyrido[4,3-d]pyrimidine-2(1H)-thione (3) as a representative case. The 1H NMR spectrum of 3 has a singlet at δ 5.46 ppm due to H-4. The H-4 showed H,H-COSY correlations with the doublets at δ 2.82 and 3.11 ppm (J = 16.8 Hz) enabling their assignment to H-5a and H-5b. The other doublets at δ 3.28 and 3.44 ppm (J = 13.8 Hz) attributable to H-7a and H-7b. The 1H singlet at δ 6.61 ppm is due to H-9 and the 2H singlet at δ 3.49 ppm corresponding to CH2 of NCH2Ph. One of the NH protons of the pyrimidine ring appeared as a singlet signal at δ 7.80 ppm, while the other merges with the signal of the aromatic protons in the region 6.99–7.42 ppm. The assignment of signals of carbon bearing hydrogen atoms has been done from the chemical shifts of hydrogen atoms and C,H-COSY correlations (Fig. 2).33
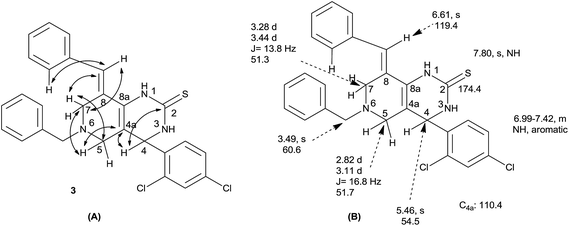 |
| Fig. 2 (A) HMBCs and (B) 1H and 13C chemical shifts of 6-benzyl-8-benzylidene-4-(2,4-dichlorophenyl)-3,4,5,6,7,8-hexahydropyrido[4,3-d]pyrimidine-2(1H)-thione. | |
3. Reactivity
3.1. Reactivity of substituents attached to ring carbon atoms
Compounds 7 and 8 were prepared by simple replacement reaction of the dichloride 4 with appropriate nucleophiles. The first replacement reaction with the corresponding benzenesulfonamides (5) proceeded completely at position 4 of the pyrimidine ring. Compound 4 is commercially available or can be prepared easily according to a synthetic methods in the literature.34 Catalytic reduction of the benzyl (Bn) group in compound 7, followed by reductive amination using formaldehyde and NaBH(OAc)3 afforded the N-methylated compound 8 (Scheme 1).35
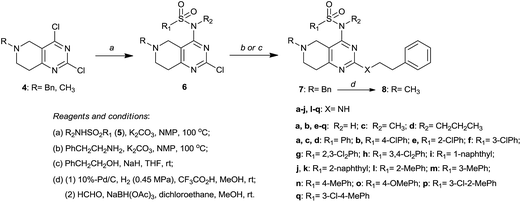 |
| Scheme 1 Reactivity of 2,4-dichloro-6-substituted-5,6,7,8-tetrahydropyrido[4,3-d]pyrimidines. | |
Because methylthio groups on the pyrimidine rings can be removed only using harsh conditions, the trans-functionalization of 9 required a prior oxidation to form a better leaving group such as methylsulfonyl group. Thus, the reaction of 9 with m-CPBA afforded disulfone 10 in good yield. The reaction of 10 with sodium methoxide gave dimethoxy derivative 11 in good yield, where only the two sulfonyl groups were displaced leaving the N-Tf group unchanged. In contrast, the reaction of 10 with sodium hydroxide afforded a mixture of the uracil derivatives 12 and 13. In this case, compound 13 resulted from the hydrolysis of the sulfonyl groups (Scheme 2).36
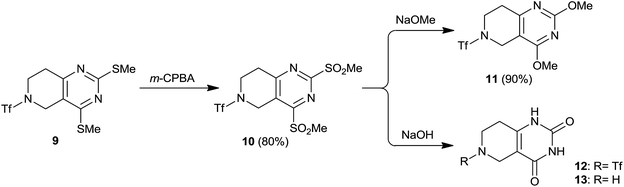 |
| Scheme 2 Synthesis of 5,6,7,8-tetrahydropyrido[4,3-d]pyrimidine and its dione derivatives. | |
Chlorination of 14 with phosphorus oxychloride in refluxing acetonitrile containing DIPEA as a base gave 15, which underwent nucleophilic substitution with a variety of anilines to yield compounds 16a–q in satisfactory yields. Deprotection of 16a–q in HBr and acetic acid yielded 4-amino-N-substituted-phenyl-5,6,7,8-tetrahydropyrido[4,3-d]pyrimidines 17a–q (Scheme 3).37
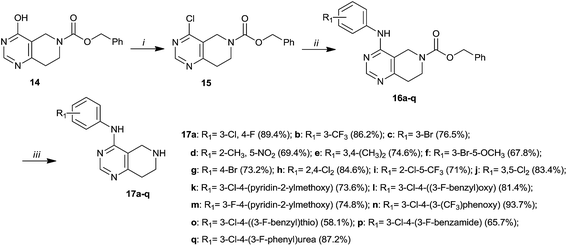 |
| Scheme 3 Reagents and conditions: (i) POCl3, DIPEA, CH3CN, reflux, 6 h; (ii) NH2ArR1, NaHMDS, THF, 0 °C, 2 h; (iii) HBr, acetic acid, 50 °C, 2 h. | |
3.2. Reactivity of NH group of pyridine ring
Pyrido[4,3-d]pyrimidines 18–23 were obtained in various conditions as depicted in Scheme 2. Compound 17a reacted with alkylsulfuryl chloride to obtain sulfonamides 18a and 18b, or underwent Michael addition or alkylation to get amines 19a and 19b. Also, compound 17a reacted with diethyl squarate and subsequently amines to afford compounds 20a–c or reacted with CDI and amines to prepare ureas 21a–c. The coupling reaction of 17a with chlorocarbonic ester or acyl chloride/aliphatic acid afforded carbamates 22a–d or amides 23a–u, respectively (Scheme 4).37
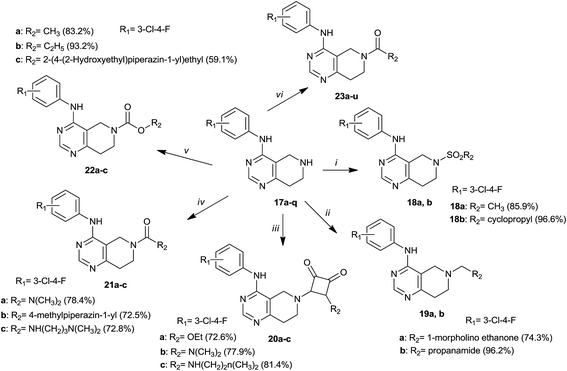 |
| Scheme 4 Reagents and conditions: (i) ClO2SR2, DIPEA, DCM, 0 °C, 2 h; (ii) ClCH2R2, DIPEA, DCM, 0 °C, 1 h or CH2CHR2, EtOH, 60 °C, 8 h; (iii) (1) diethyl squarate, EtOH, 60 °C, 4 h; (2) HNR1R2, EtOH, 60 °C, 4 h; (iv) (i) CDI, EtOH, reflux, 4 h; (ii) HNR1R2, EtOH, reflux, 12 h; (v) ClOCOR2, DIPEA, CH3CN, 0 °C, 1 h; (vi) ClOCR2, DIPEA, DCM, 0 °C, 1 h or HO2CR2 EDCI, HOBT, DIPEA, CH3CN, 60 °C, 6 h. | |
3.3. Ring transformation
The nucleophilic attack (24) of the nitrogen atom of the nicotinamido group on the carbonyl carbon of the 4-amido group was the rate-determining step in the cyclization to the pyrido[4,3-d]pyrimidines (25). Ring opening (26) and hydrolysis, presumably of the amidine (27), to 4-aminonicotinic acid was rapidly accomplished by warming the pyridopyrimidine (25; R1 = Me, R2 = H) with dilute hydrochloric acid (Fig. 3).38
 |
| Fig. 3 Ring opening of pyridopyrimidine. | |
3.4. Alkylation, heteroarylation and benzoylation reactions
The subsequent alkylation of the free amino group in the 4-position of 4-amino-5,6,7,8-tetrahydropyrido[4,3-d]pyrimidines 28 may be effected in known manner, for instance with the aid of an alkyl halide, a dialkyl sulfate or an alkyl ester of an aromatic sulfonic acid. In the event that it is desired to methylate the free amino group, this may also be accomplished by heating compound 28 with formic acid and formaldehyde (Fig. 4).39
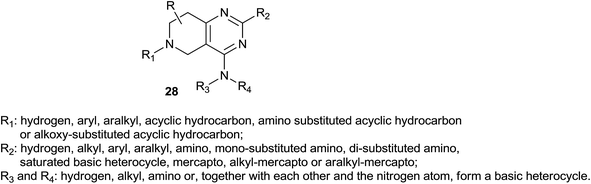 |
| Fig. 4 Chemical structures of 4-amino-5,6,7,8-tetrahydropyrido[4,3-d]pyrimidines. | |
Compound 29 underwent alkylation to give compound 30. After switching protecting groups at the 6 position, the PMB group was removed in the presence of aluminum chloride in CH2Cl2 and R3 was installed via a Mitsunobu displacement. The trifluoroacetate was removed from 32 and R1 installed at the 6 position by alkylation, acylation or reductive amination. The order of steps was modified depending on the desired point of diversity (Scheme 5).40
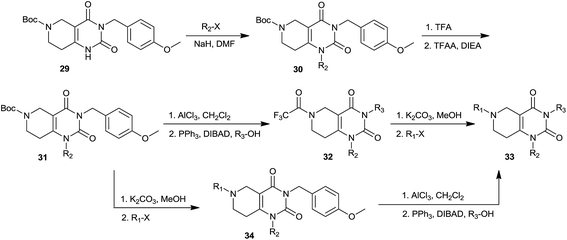 |
| Scheme 5 Synthesis of pyrido[3,4-d]pyrimidine-diones via a p-methoxybenzyl protecting group. | |
Treatment of 35 with morpholine led exclusively to the mono-substituted compound 36. The Suzuki reaction [step (d)] was performed with 3,4-dimethoxyphenylboronic acid under standard reaction conditions: potassium carbonate as a base and tetrakis triphenylphosphine as the catalyst. For the coupling with propargyl alcohol, a copper free version of the Sonagashira reaction [step (b)] was carried out using bis(triphenylphosphine)-palladium(II)acetate as the catalyst and triethylamine as the base. The Stille coupling [step (c)] was done with tributylphenyl stannane and tetrakis(triphenylphosphine)palladium(0) as the catalyst. The remaining chlorine of compounds 36 and 38 was reduced off by catalytic hydrogenation over palladium to produce 40 and 41 (Scheme 6).41
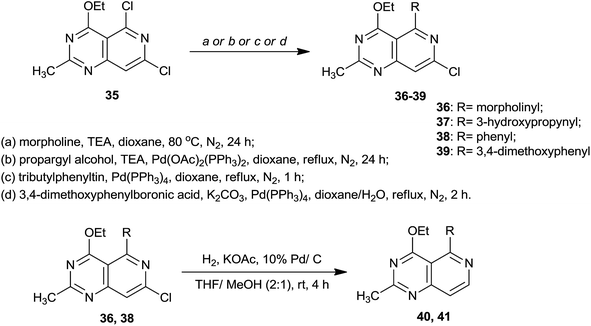 |
| Scheme 6 Alkylation of 5,7-dichloro-4-ethoxy-2-methylpyrido[4,3-d]pyrimidine. | |
The remaining chlorine at position 7 can be used for a subsequent SNAr or palladium-catalyzed cross-coupling reaction, as shown in Scheme 7. Treatment of 42 with morpholine furnished 5-morpholino derivative 43. The regiochemistry of this compound was proven by the reductive dehalogenation of chlorine, yielding 44. In the subsequent step, chlorine at position 7 was used for a Suzuki-type of coupling with 3,4-dimethoxyphenyl boronic acid, yielding the 5,7-disubstituted pyrido[4,3-d]pyrimidine analogue 45.41
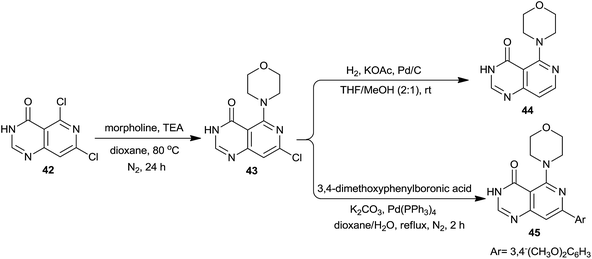 |
| Scheme 7 Synthesis of 5-morpholinopyrido[4,3-d]pyrimidin-4(3H)-ones. | |
Treatment of 5,6,7,8-tetrahydropyrido[4,3-d]pyrimidinedione 46 with POCl3 provided the desired dihalide 47, which was reduced by Zn to give 48. N-Aryl-piperazinyl 49 was obtained by the reaction of 47 with 1-(2-pyrimidinyl)piperazine. The benzyl group of 49 was removed by Pd/C to afford 50. Benzoylation of 50 by coupling with the appropriate substituted benzoic acid using standard EDCI/HOBt coupling conditions gave 51a–f. Compounds 51g and 51h were obtained from 52a and 52b in the same way as 51a–f (Scheme 8).42
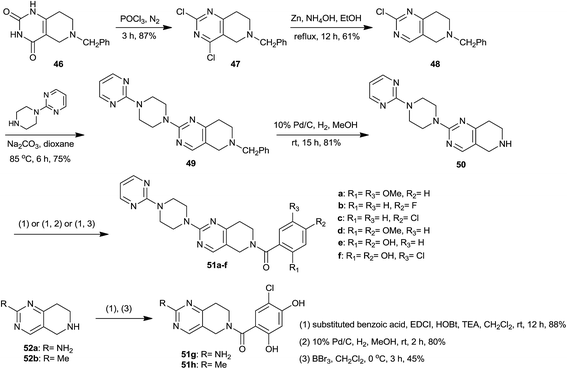 |
| Scheme 8 Arylation and benzoylation of 5,6,7,8-tetrahydropyrido[4,3-d]pyrimidines. | |
Compound 53 was alkylated at the N-1 position with various halides under basic conditions to give 54. Boc-protection instead of benzyl was necessary to keep the integrity of the amine at the 7 position during the allyl oxidation step. The allyl group of 54 was oxidized by treatment with OsO4/2,6-lutidine followed by NaIO4 to form the intermediate aldehyde 55. Reductive amination of 55 using standard conditions gave 56. Boc-deprotection followed by alkylation, acylation or reductive amination gave the final compounds 58 (Scheme 9).40
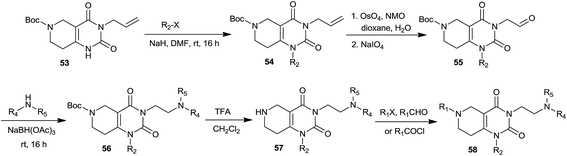 |
| Scheme 9 Alkylation of pyrido[3,4-d]pyrimidine-2,4-diones when R3 is an ethylamine. | |
4. Synthesis
4.1. Synthesis from pyridines
4.1.1. Synthesis from nicotinic acid. In order to synthesize the pyrido[4,3-d]pyrimidine scaffold from an appropriately substituted pyridine analogue, the amino group of 4-amino-2,6-dichloropyridine 59 was protected as a tert-butoxycarbonyl group (Boc). The formation of a carbanion by treatment with n-BuLi, in the presence of TMEDA and quenching by the addition of anhydrous carbon dioxide gas led to the formation of the nicotinic acid derivative 61. The Boc group was cleaved off under acidic conditions, yielding pyridine analogue 62. By refluxing compound 62 in thionylchloride, the carboxylic acid was converted to its corresponding acid chloride, which was subsequently treated with ammonia, affording amide 63. Condensation of 4-amino-2,6-dichloronicotinamide 63 with triethylortho-acetate gave 64 (38% yield), while heating of 63 in triethyl-orthoformate gave 42. The ethoxy compound 64 could be easily converted to its 4-hydroxy analogue by acidic or basic hydrolysis (Scheme 10).41
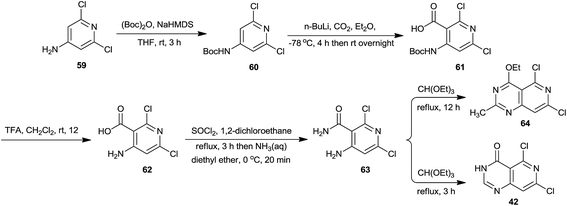 |
| Scheme 10 Synthesis of 5,7-dichloro-pyrido[4,3-d]pyrimidines. | |
The halogen in 2-chloro-4-aminonicotinic acid (65) provided a convenient handle for introducing substituents at C-5 and for further SAR exploration. Amidation of 65 with appropriate amines and reaction with either methyl- or benzyl-protected salicylaldehyde analogs followed by oxidation generated the corresponding pyrido[4,3-d]pyrimidin-4(3H)-one 66. The 5-chloro-analog could be further elaborated with nucleophiles to provide diverse C-5 substituents or O-deprotected to afford pyrido[4,3-d]pyrimidin-4-one derivatives 68 (Scheme 11).43
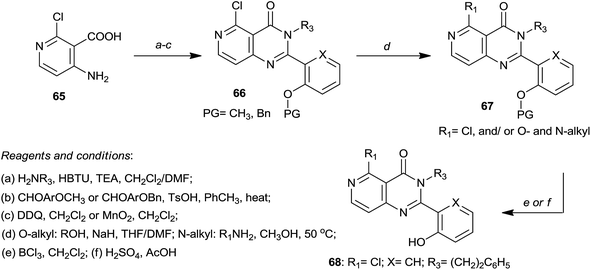 |
| Scheme 11 Synthesis of 3-phenethylpyrido[4,3-d]pyrimidin-4(3H)-ones. | |
4-Aminonicotinic acid (69) was prepared from 3-methyl-4-nitropyridine 1-oxide by oxidation to 4-nitronicotinic acid 1-oxide followed by catalytic reduction.44 The reaction of 2-methylpyrido[4,3-d][1,3]oxazin-4-one (70; R1 = Me) with ammonia, hydroxylamine or aniline yielded the corresponding pyrido[4,3-d]pyrimidin-4(3H)-ones (25; R1 = Me). 4-Amidonicotinates (73) were produced in excellent yields from the aminoester (71) and the appropriate acyl chloride in pyridine. Treatment of these amido-esters (73) with amines yielded the required 4-amidonicotinamides (72) (Scheme 12).38
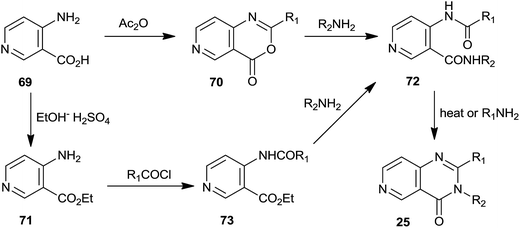 |
| Scheme 12 Synthesis of pyrido[4,3-d]pyrimidin-4(3H)-ones. | |
Irradiation of anthranilic acid 69 and DMF-DMA in DMF for 15 min at 100 °C gave enamine 74. Various amines could be used affording N3-alkylated quinazolin-4-ones 75a–f within a short period of time (Scheme 13).45
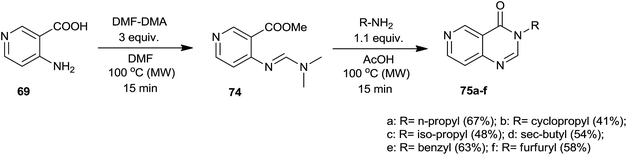 |
| Scheme 13 Optimized protocol for the one-pot synthesis of pyrido[4,3-d]pyrimidin-4(3H)-ones. | |
The mechanism of cyclization occurred via a first attack of the amine on the activated carbon of the amidine. The intermediate triamine species released dimethylamine and cyclized into the expected pyridopyrimidines 75a–f. In the specific case of 4-aminonicotinic acid 69, the low yields observed can be explained by a substantial contribution of a zwitterionic resonance form which could involve the instability of the intermediate triamine resulting from the condensation of the primary amine on the formimidamidoester 74. The elimination process turned in favor of the unexpected aminoester 76 (Scheme 14) which was isolated in 15–20% yield.45
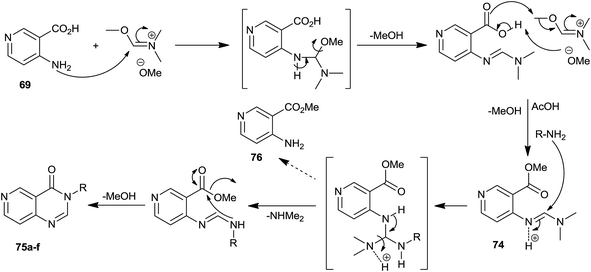 |
| Scheme 14 Proposed mechanism for the synthesis of pyrido[4,3-d]pyrimidin-4(3H)-ones. | |
4.1.2. Synthesis from ethyl nicotinate. The reaction of the dichloronicotinate 77 with p-methoxybenzyl amine provided the protected amino-nicotinate 78 which upon reaction with 4-trifluoromethylphenyl boronic acid under Suzuki reaction conditions provided 79. Subsequent hydrolysis of the ethyl nicotinate 79 followed by the EDC or BOP-mediated coupling reactions of the nicotinic acid 80 with ammonium chloride provided the nicotinamide 81. Then TFA mediated deprotection of the p-methoxybenzyl group provided the desired aminonicotinamide 82. The condensation of 82 with the corresponding aldehydes 83 followed by oxidative dehydrogenation provided pyrido[4,3-d]pyrimidin-4(3H)-ones 84 and 85 (Scheme 15).46
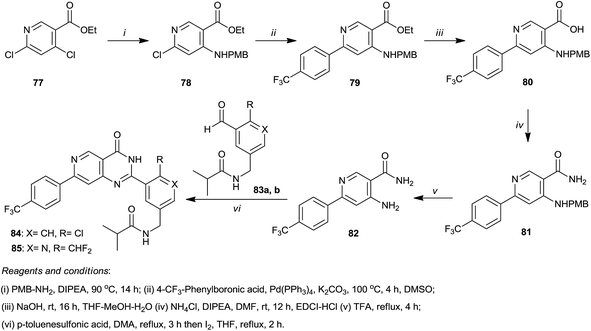 |
| Scheme 15 Synthesis of 2-substituted-7-(4-(trifluoromethyl)phenyl)pyrido[4,3-d]pyrimidin-4(3H)-ones. | |
To allow for more diversity at the 3 position, the p-methoxybenzyl (PMB) isocyanate was used as a protecting group. Compound 86 reacted with PMB isocyanate in refluxing toluene followed by treatment with sodium methoxide to give 87 (Scheme 16).40
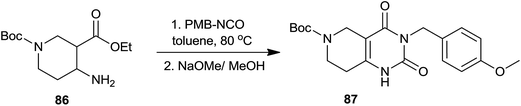 |
| Scheme 16 Synthesis of pyrido[3,4-d]pyrimidine-diones. | |
4.1.3. Synthesis from 4-amino-3-cyanopyridines. Treatment of 89 with triethyl orthoformate in the presence of acetic anhydride afforded imidate 90α (R = Et), which upon treatment with saturated methanolic gave the desired pyrido[4,3-d]pyrimidine 91α in poor yield together with a predominant amount of 89 produced by ammonolysis of the imidate ester. The use of trimethyl orthoformate to generate imidate 90α (R = Me), followed by ammonia treatment, provided 91α in better yield with only minor ammonolysis to 89. A similar sequence from 88 afforded the desired β-isomer 91β via 90β (R = Me). Both 91β and 91α could be readily deprotected (6% HCl in MeOH) to the corresponding pyrido[4,3-d]pyrimidine C-nucleosides 92 and 93, which were obtained in 55% and 41% overall yields from 88 and 89, respectively. Syntheses of the corresponding inosine analogs 96 and 97 were carried out using 2-aminonitriles 88 and 89, respectively. Annulation of the latter with triethyl orthoformate/acetic anhydride afforded pyrido[4,3-d]pyrimidine C-nucleoside 95α in excellent yield. Application of the same procedure to o-aminoamide 94β gave a similarly good yield of the desired blocked β C-nucleoside 95β. Deprotection of both isomers 95α and 95β by treatment with 6% HCl in methanol gave 97 and inosine analog 96 (in 65% and 66% overall yields from 89 and 88, respectively) (Scheme 17).47
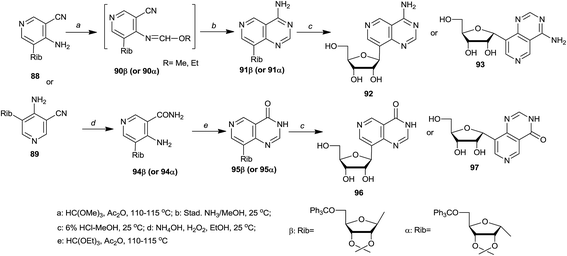 |
| Scheme 17 Synthesis of pyrido[4,3-d]pyrimidine C-nucleosides. | |
4.1.4. Synthesis from piperidin-4-one and pyridine-4-ones. 6-Benzyl-5,6,7,8-tetrahydropyrido[4,3-d]pyrimidine-2,4(1H,3H)-dione (46) was prepared by amination of ethyl 1-benzyl-4-oxopiperidine-3-carboxylate (98) with urea under basic conditions. Reaction of 99 with DMF-DMA at reflux afforded 100, which on treatment with guanidine carbonate or acetamidine hydrochloride in ethanol in the presence of sodium acetate provided 5,6,7,8-tetrahydropyrido[4,3-d]pyrimidines 52a, b (Scheme 18).42
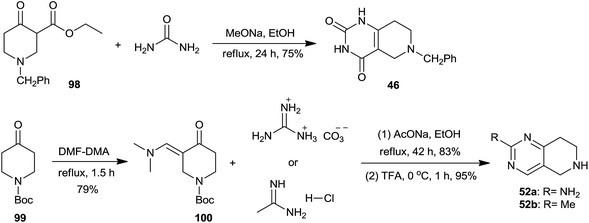 |
| Scheme 18 Synthesis of 5,6,7,8-tetrahydropyrido[4,3-d]pyrimidines. | |
The reaction of 1-benzylpiperidin-4-one (101) with different nitriles and Tf2O under mild conditions afforded pyrido[4,3-d]pyrimidines 9a–c in moderate yields (Scheme 19).36
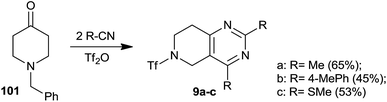 |
| Scheme 19 Synthesis of 2,4-disubstituted-pyrido[4,3-d]pyrimidines. | |
Multicomponent reaction of 102, 2 moles of different aromatic aldehydes and thiourea in alcoholic potassium hydroxide solution under reflux produced octahydropyrido[4,3-d]pyrimidine-2(1H)-thiones 103a–e (Scheme 20).48
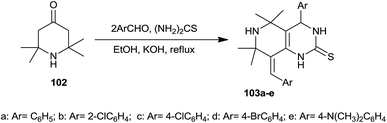 |
| Scheme 20 Synthesis of 3,4,5,6,7,8-hexahydropyrido[4,3-d]pyrimidine-2(1H)-thiones. | |
3-Cyano-piperidine (104) reacted with amidine (105) to give 4-amino-5,6,7,8-tetrahydropyrido[4,3-d]pyrimidines 106, the reaction is preferably carried out in the presence of an alkaline condensation agent at room temperature or moderately elevated temperatures, and advantageously in the presence of an inert solvent (Scheme 21).39
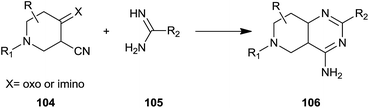 |
| Scheme 21 Synthesis of 4-amino-5,6,7,8-tetrahydropyrido[4,3-d]pyrimidines. | |
When R3 was a substituted ethyl amine, the 4-oxo-piperidine-3-carboxylic acid ethyl ester 107 was Boc-protected on nitrogen. Compound 108 was treated with ammonium acetate to give the corresponding enamine 109. Reaction of 109 with isocyanate followed by a ring-closure under basic conditions gave the pyrido[3,4-d]pyrimidine-2,4-dione 110 (Scheme 22).40
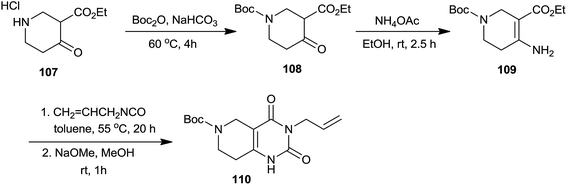 |
| Scheme 22 Synthesis of pyrido[3,4-d]pyrimidine-2,4-diones when R3 is an ethylamine. | |
Various alkyl, acid and sulphonyl halides reacted with 4-piperidone 111 to form N-substituted piperidin-4-one 112. Methoxycarbonylation of 112 in the presence of sodium hydride afforded the ketoester 113 which underwent base catalyzed reaction with guanidine to form substituted tetrahydropyridopyrimidine 114. Using various alkyl, aryl and hetroaryl acids, the acid amine coupling was then carried out with the aid of EDC and HOBT to form the amide derivatives of tetrahydropyrido-pyrimidines 115 (Scheme 23).49
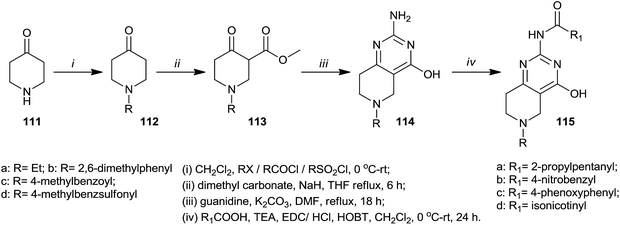 |
| Scheme 23 Synthetic route to substituted tetrahydropyridopyrimidines. | |
Catalytic hydrogenation of ethyl 1-benzyl-4-oxopiperidine-3-carboxylate (116) led to the intermediate 117, which was protected again with CbzCl to yield intermediate 118. Cyclization with formamidine acetate generated 14 efficiently (Scheme 24).37
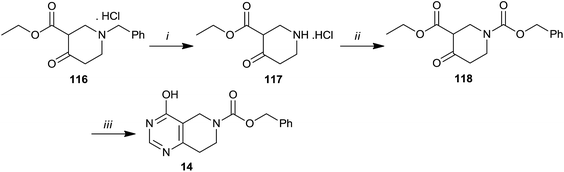 |
| Scheme 24 Reagents and conditions: (i) H2, Pd/C, 5 atm, HCl, ethanol, 40 °C, 8 h; (ii) CbzCl, DIPEA, DCM, rt, 2 h; (iii) formamidine acetate, ethanol, NaOCH3, 6 h, reflux. | |
4.1.5. Synthesis from 3-acetylpyridines. Dinitriles 119 were prepared by the reaction of malononitrile and carbon disulfide in the presence of KOH as base in acetonitrile, and then treated with ammonium hydroxide in methanol.50 4-Aminopyridines 120 were converted to the formamidates 121 in good yield via the reaction with triethyl orthofromate in the presence of acetic anhydride. Treated with amines, the formamidates 121 were cyclized easily and regioselectively to afford 122 in good yields (Scheme 25).51
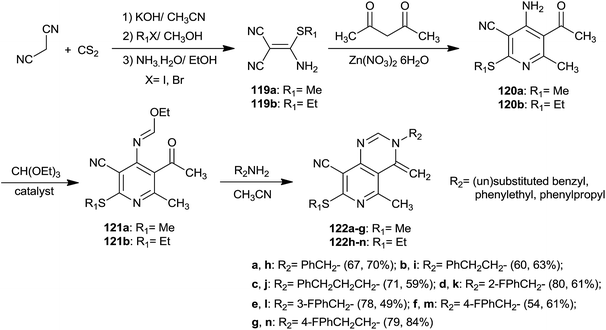 |
| Scheme 25 Synthesis of 3,4-dihydropyrido[4,3-d]pyrimidine-8-carbonitriles. | |
Substituted pyridine 120a,52 was readily oxidized by hydrogen peroxide to give intermediate 123, and then converted into 7-methoxy-4-amino-pyridine 124 by nucleophilic substitution reaction. The reaction of 124 with triethyl orthoformate in the presence of acetic anhydride afforded formamidate 125 in good yield. Cyclization of the formamidate 125 with alkyl amines or ammonia at mild condition afforded pyrido[4,3-d]pyrimidines 127 in moderate to good yields (Scheme 26).53
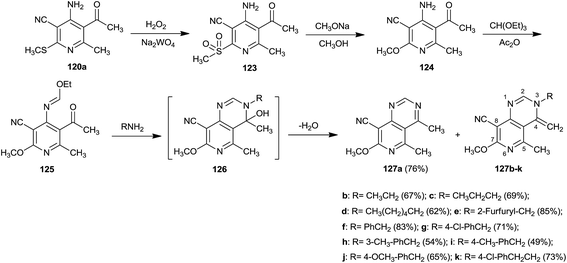 |
| Scheme 26 Synthesis of pyrido[4,3-d]pyrimidines. | |
4.2. Synthesis from pyrimidines
4.2.1. Synthesis from alkynyl-pyrimidines. Various 2,4-disubstituted 7-arylpyrido[4,3-d]pyrimidines 129a–t were prepared via the thermal reaction of 2,4-disubstituted 6-arylethynylpyrimidine-5-carbaldehydes 128a–t with tert-butylamine. When solutions of compounds 128a–h and tert-butylamine in DMF were irradiated in a microwave oven, after 10–60 min, 2,4-disubstituted 7-arylpyrido[4,3-d]pyrimidines 129a–h were obtained in high yields (Scheme 27).54
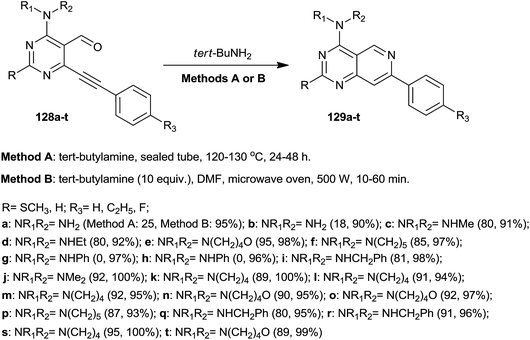 |
| Scheme 27 Synthesis of 2,4-disubstituted 7-arylpyrido[4,3-d]pyrimidines. | |
Pyrido[4,3-d]pyrimidines 129 were obtained via initial formation of the intermediate N-(tert-butyl)-N-{4-[(Z)-2-aryl-2-(tert-butylamino)vinyl]pyrimidin-5-yl}methyleneamines 130a, b followed by 1,6-electrocyclic reaction and aromatization (Scheme 28).54
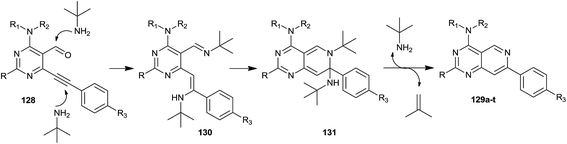 |
| Scheme 28 Mechanistic pathway to prepare 2,4-disubstituted 7-arylpyrido[4,3-d]pyrimidines. | |
4.2.2. Synthesis from pyrimidinones. Intramolecular Staudinger/aza-Wittig reaction of 5-acyl-4-(β-azidoalkyl)pyrimidines 132 promoted by PPh3 gave pyrido[4,3-d]pyrimidin-2-ones 134. The rate of this step depended on electrophilicity of carbonyl group and steric factors in iminophosphoranes 133 (Scheme 29).55
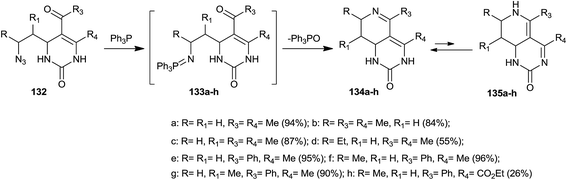 |
| Scheme 29 Synthesis of pyrido[4,3-d]pyrimidin-2-ones. | |
The pyrido[4,3-d]pyrimidine derivatives 138a–c were prepared from the reaction of pyrimidine derivatives 136 with amines 137 in an appropriate solvent such as absolute DMF for several tens of minutes to several hours under reflux (Scheme 30).56
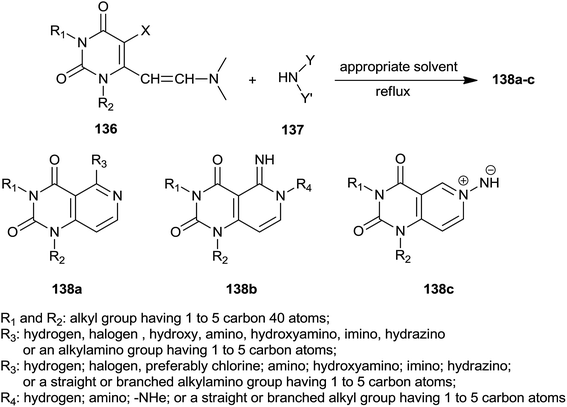 |
| Scheme 30 Synthesis of pyrido[4,3-d]pyrimidine derivatives. | |
4.2.3. Synthesis from halo-pyrimidines. 1-(4-Chloro-2-methylsulfanyl-pyrimidin-5-yl)ethanone 140 reacted with ethyl 3,3-diaminoprop-2-enoate 139 to produce the pyrido[4,3-d]pyrimidine 141 in 71% yield at room temperature in DMF. At elevated temperature (175 °C), the same reaction with chloropyrimidine 142 led in a few minutes to the bis-amino derivative 143 in good yield (Scheme 31).57–59
 |
| Scheme 31 Synthesis of pyrido[4,3-d]pyrimidines via an imine. | |
4.2.4. Synthesis from pyrimidine carboxylates. Reaction of the bromomethylpyrimidine 144 with potassium cyanide followed by treatment with arenediazonium salt afforded the corresponding hydrazone intermediate 147 which reacted with hydrazine derivatives to afford pyrido[4,3-d]pyrimidines 148a and 148b (Scheme 32).60
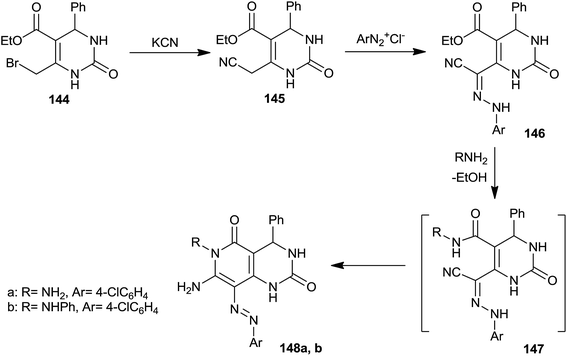 |
| Scheme 32 Synthesis of 3,4-dihydropyrido[4,3-d]pyrimidine-2,5(1H,6H)-diones. | |
When diester 149 is treated with primary amines i.e. benzylamine or butylamine, the CH2COOEt fragment selectively participates in the reaction to yield pyrimidinyl-acetamides 150a and 150b. Treatment of 150a and 150b with boiling solution of MeONa in MeOH led to the pyridine ring closure to form 6-R-4-amino-7-hydroxy-2-phenylpyrido[4,3-d]pyrimidine-5(6H)-ones 151a and 151b (Scheme 33).61
 |
| Scheme 33 Synthesis of 6-substituted-2-phenylpyrido[4,3-d]pyrimidin-5(6H)-ones. | |
4.3. Multicomponent synthesis
A microwave-promoted solvent- and catalyst-free aza-Diels–Alder reaction of uracil derivatives 152, aldehydes 153 and amines 154 at 550 W and 160 °C for 12 min gave 1,3-dimethyl-5,6-diaryl-5,6-dihydropyrido[4,3-d]pyrimidine-2,4(1H,3H)-diones 155 (Scheme 34).62
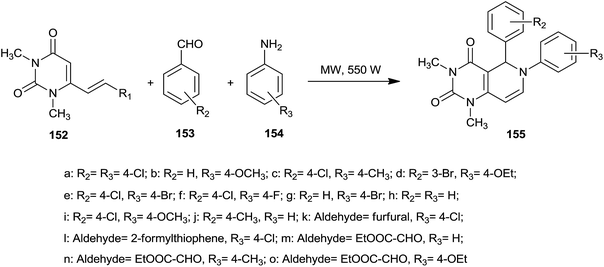 |
| Scheme 34 Synthesis of 1,3-dimethyl-5,6-diaryl-5,6-dihydropyrido[4,3-d]pyrimidine-2,4(1H,3H)-diones. | |
Pseudo four-component domino reactions of N-substituted-4-piperidones (156 and 157), aromatic aldehydes (158) and thiourea (159) under solvent-free conditions at ambient temperature afforded the pyrimidine-2-thiones 160 and 3 in almost quantitative yields (93–98%; Scheme 35).33,63
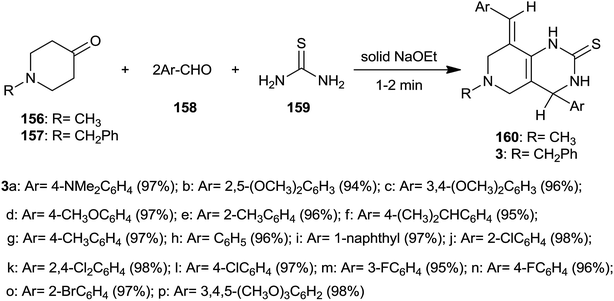 |
| Scheme 35 Synthesis of pyridopyrimidine-2-thiones. | |
A one-pot and catalyst-free reactions of aromatic aldehyde 158, 6-[2-(dimethylamino)prop-1-enyl]-1,3-dimethyluracil 161 and ammonium acetate 162 in ethanol under both ultrasound and thermal conditions gave 7-methyl-substituted pyrido[4,3-d]pyrimidines 163 (Scheme 36).64
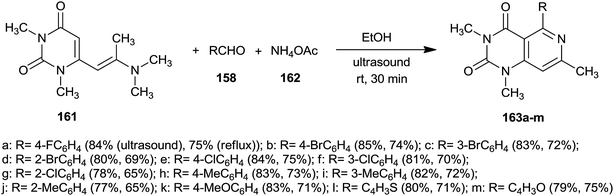 |
| Scheme 36 Multicomponent synthesis of pyrido[4,3-d]pyrimidines. | |
Thomas and Wilhelm,65 have reported the synthesis of 8,8-disubstituted-1,3,6-trimethyl-1,2,3,4,5,6,7,8-octahydropyrido[4,3-d]pyrimidines 164 from the reaction of a diphenyl propanone such as diphenyl acetone or a diphenyl propanone bearing a substituent on one or both of the phenyl groups, such as 1-phenyl-1-para-chlorophenyl-2-propanone with methyl amine and formaldehyde under basic conditions, e.g. in the presence of an organic base. Where, one or both of the substituted groups in the 8-position bears an additional substituent attached to the nuclear carbon atom and salts, where R1 and R2 each represents a nuclearly substituted or unsubstituted monocyclic 6-membered aryl group (Fig. 5).
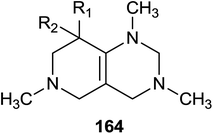 |
| Fig. 5 Chemical structure of 8,8-disubstituted-pyrido[4,3-d]pyrimidines. | |
4.4. Retrosynthesis of pyrido[4,3-d]pyrimidinones
Fesenko & Shutalev,55 have hypothesized that pyrido[4,3-d]pyrimidin-2-one scaffold 135 could be assembled from 5-acyl-4-(2-azidoalkyl)pyrimidines 165 using Staudinger/aza-Wittig sequence (Scheme 37). The synthesis of pyrimidines 165 could include ureidoalkylation of enolates of 1,3-diketones with N-(3-azido-1-tosylalkyl)ureas 166 followed by dehydration of the resulting products. Azides 166 could be obtained by three-component condensation of 3-azidoaldehydes 167, p-toluenesulfinic acid and ureas.
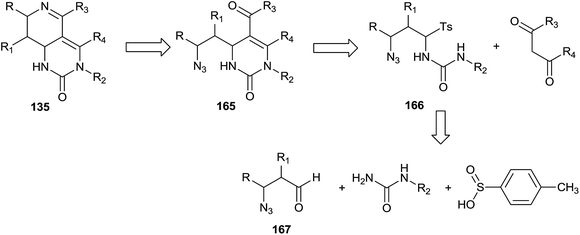 |
| Scheme 37 Retrosynthesis of pyrido[4,3-d]pyrimidin-2-ones via ureidoalkylation/Staudinger/aza-Wittig reactions. | |
4.5. Synthesis of binary heterocycles
Fusion of 168 with 2-aminopyridine in the presence of a catalytic amount of freshly fused sodium acetate afforded the amide derivative 169, which reacted with chloroacetyl chloride in chloroform at room temperature to give the chloro-acetyl derivative 170. Refluxing of 170 with acetic anhydride gave the corresponding pyrimidine 171, which gave the corresponding Mannich bases 172a and 172b upon reaction with methyl and ethyl amines at room temperature (Scheme 38).66
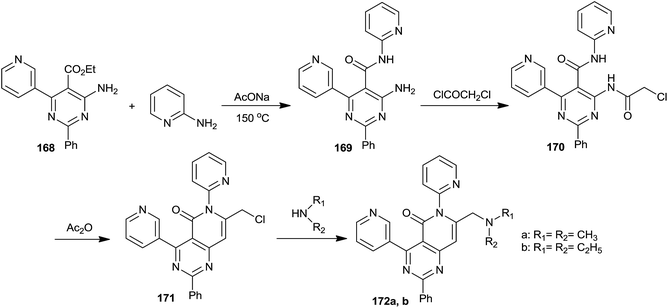 |
| Scheme 38 Synthesis of 6-(pyridin-2-yl)-4-(pyridin-3-yl)pyrido[4,3-d]pyrimidin-5(6H)-ones. | |
4.6. Synthesis of spiro heterocyclic systems
Treatment of 2,3,4,4a-tetrahydropyrido[4,3-d]pyrimidine-5,7(6H,8H)-diones 173 with two equivalents of bromine in acetic acid afforded the 8,8-dibromo derivatives 174. Heating of 174 in ethanol in presence of piperidine with either of ethylenediamine or thiosemicarbazide afforded the corresponding spiro compounds 175 and 176. Similarly, refluxing of 174 with o-phenylenediamine and o-aminothiophenol in ethanol in the presence of piperidine afforded the corresponding cyclized products 177a–d (Scheme 39).67
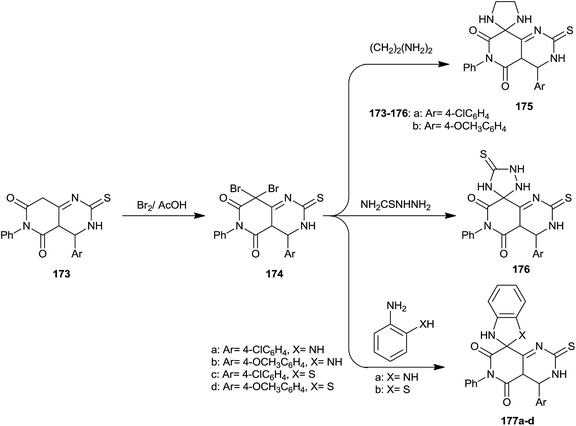 |
| Scheme 39 Reaction of dibromopyridopyrimidines with binucleophilic reagents. | |
Refluxing of 174 in ethanol/piperidine with either malononitrile (178a) or ethyl cyanoacetate (178b) afforded the corresponding arylidenes 179a–d. Heating of 179a–d with hydrazine hydrate in ethanol gave the spiro products 180a–d. Similar treatment of 179a–d with thiourea in ethanol/piperidine resulted in the formation of the spiro-pyrido[4,3-d]pyrimidines 181a–d (Scheme 40).67
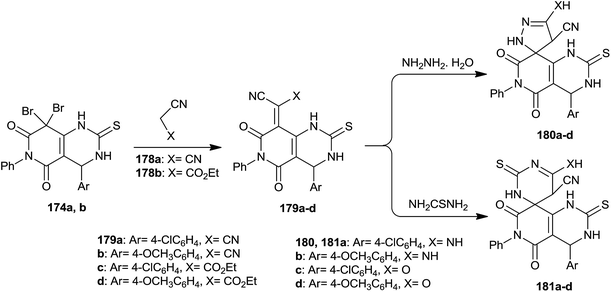 |
| Scheme 40 Reaction of dibromopyridopyrimidines with active methylene compounds followed by binucleophiles. | |
4.7. Synthesis of polycyclic systems
4.7.1. Synthesis of thienopyrido[4,3-d]pyrimidinones. Treatment of 183 with PPh3, hexachloroethane and TEA afforded iminophosphorane 184. Iminophosphorane 184 reacted with para-fluorophenyl isocyanate to give carbodiimide 185. The reaction of carbodiimide 185 with substituted phenols gave 186 under heating for 1–2 h in the presence of catalytic amount of K2CO3. The formation of 186 can be rationalized in terms of an initial nucleophilic addition of phenoxides to the carbodiimides 185 to give the intermediate 187 which cyclize to give 186a–j. Refluxing of 185 with primary or secondary amines in CH2Cl2 containing a catalytic amount of EtONa gave 2-(alkylamino)-thieno[3′,2′:5,6]pyrido[4,3-d]pyrimidin-4(3H)-ones 186k–p (Scheme 41).31
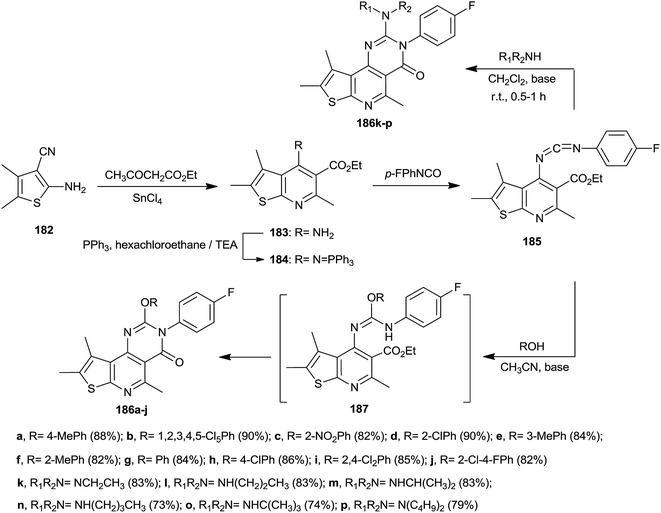 |
| Scheme 41 Synthesis of thieno[3′,2′:5,6]pyrido[4,3-d]pyrimidin-4(3H)-ones. | |
4.7.2. Synthesis of pyrido[4,3-d]thiazolo[3,2-a]pyrimidines. The corresponding pyrimidine thiones 189a–e were obtained from condensation of 188a–e with thiourea in ethanolic potassium hydroxide solution under reflux. The reaction of 188a–d, f with 2-amino-2-thiazoline in butanol/DMSO mixture afforded 190a–e in good yields. The reaction of 189a–e with chloroacetic acid or with 2-bromopropionic acid in the presence of sodium acetate in acetic acid/acetic anhydride mixture gave thiazolopyrimidenes 191a–e and 192a–e, respectively (Scheme 42).68
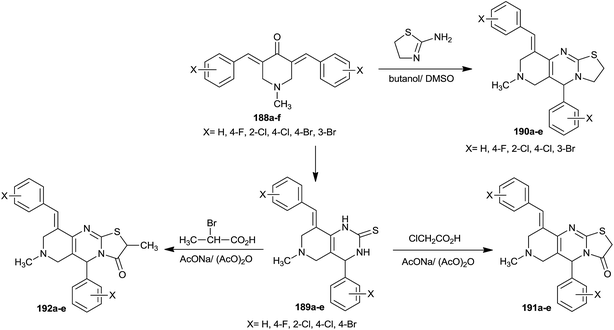 |
| Scheme 42 Synthesis of pyrido[4,3-d]thiazolo[3,2-a]pyrimidin-3(5H)-ones. | |
Compounds 191a, b, d contain an active methylene group, when these compounds reacted with aromatic aldehydes in the presence of acetic acid/acetic anhydride mixture, the corresponding arylmethylene thiazolopyrimidines 193a–e were obtained. The products 193a–e could be also obtained from reaction of 189a, b, d with chloroacetic acid, followed by treatment with aromatic aldehyde in the presence of sodium acetate in refluxing acetic acid/acetic anhydride mixture. Compounds 191a, b, d were coupled with aryldiazonium salts in the presence of pyridine to give arylazo-thiazolopyrimidines 194a–d (Scheme 43).68
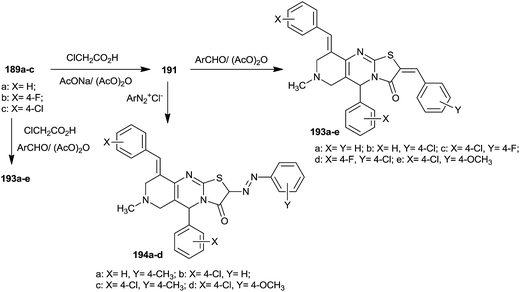 |
| Scheme 43 Synthesis of pyrido[4,3-d]thiazolo[3,2-a]pyrimidin-3(5H)-ones. | |
The reaction of 103a–e with chloroacetic acid gave pyrido[4,3-d]thiazolo[3,2-a]pyrimidin-3(5H)-ones 195a–c, which reacted with different aromatic aldehydes to produce pyrido[4,3-d]thiazolo[3,2-a]pyrimidin-3(5H)-ones 196a–c. Compound 196b was obtained also from the reaction of 102 with 3 moles of 2-chlorobenzaldehyde, thiourea and chloroacetic acid or from the reaction of 103b with one mole of 2-chlorobenzaldehyde and chloroacetic acid (Scheme 44).48
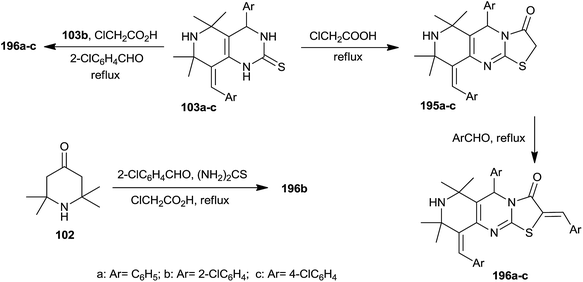 |
| Scheme 44 Synthesis of pyrido[4,3-d]thiazolo[3,2-a]pyrimidin-3(5H)-ones. | |
5. Biological applications
5,6,7,8-Tetrahydropyrido[4,3-d]pyrimidine and related compounds have been used as starting materials for the multi-step synthesis of tetrahydropteroic acid derivatives.69 On the other hand, substituted diamino-5,6,7,8-tetrahydropyrido[4,3-d]pyrimidines were tested due to the ability of the products to inhibit dihydrofolate reductase from Pneumocystis carinii and Toxoplasma gondii.70
5.1. Human CaR antagonism
Pyrido[4,3-d]pyrimidin-4(3H)-ones 68 were evaluated in vitro for human CaR antagonism. Introduction of a range of small alkyl, amino, and ether substituents resulted in compounds with diminished potency when compared with 68 (Fig. 6). The trifluoromethyl group at C-5 proved ideal, particularly in terms of its pharmacology and chemical stability. Orally-active 5-trifluoromethylpyrido[4,3-d]pyrimidin-4(3H)-one CaR antagonists with a short duration of action have been identified using a combination of PK/PD and in silico modeling of microsomal stability data, which greatly facilitated design of analogs. Additional safety and ADME profiling is currently underway for analogs (R)-68g and 68h in support of potential clinical studies.43
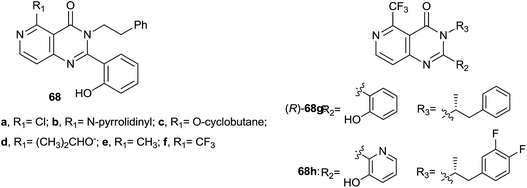 |
| Fig. 6 Human CaR antagonism agents. | |
5.2. ATPase activity
Malachite green assay for inorganic phosphate was initially used to measure the ATPase activity of Hsp90, it was a colorimetric assay which was well suited to the automation required for high throughput screening as a rough and fast evaluation methodology.71,72 Pyrido[4,3-d]pyrimidines 197–206 (Fig. 7) showed over 60% inhibition rate at 40 mM.42
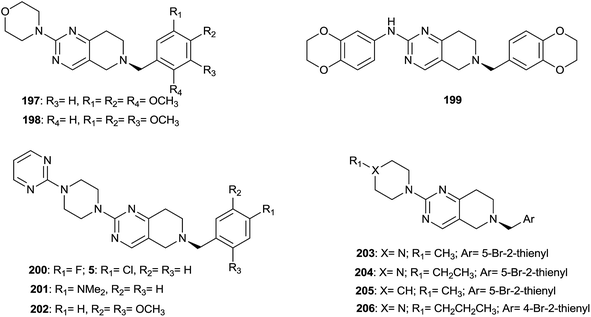 |
| Fig. 7 The structures of more potent compounds in the Malachite green assay. | |
5.3. Hepatic metabolism
Wuyts et al.,73 have reported the biopharmaceutical profile of pyrido[4,3-d]pyrimidines with different substitution pattern was determined at the intestinal and hepatic level. There were no toxicity issues toward Caco-2 and hepatocytes within a 6 h incubation. The intestinal solubility, permeability and hepatic metabolic stability were clearly structure dependent. A dimethoxyphenyl (207) and phenylhydrazido substituent (215) (Fig. 8) were unfavorable because of their low intestinal permeability and FaSSIF solubility, respectively. The 2-methoxyethoxy substituent of 211 resulted in the lowest Clint and ER of all pyrido[4,3-d]pyrimidines suggesting protective effects against hepatic metabolism. Conversely, apropenyl (213) or thienyl (208) group makes the analog more susceptible to hepatic metabolism. The compounds' PSA seems to be a key determinant in intestinal permeability and hepatic metabolism.
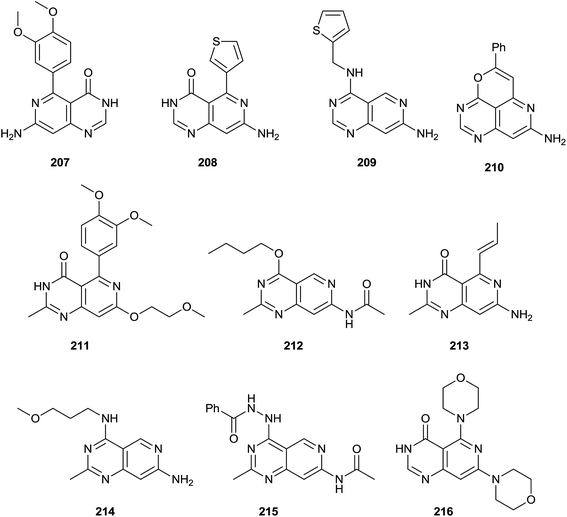 |
| Fig. 8 Chemical structure of the pyrido[4,3-d]pyrimidines. | |
5.4. Fungicidal activity
Compound 122a exhibited significant broad spectrum fungicidal activity, with nearly complete inhibition against F. oxysporium, B. cinereapers and G. zeae at dosage of 50 mg mL−1. Among compounds (R1 = Me), most of them showed significant activities. When fluorine was introduced to the compounds, the inhibition rates decreased obviously.51
5.5. Antitumor activity
Two representative compounds 127i: CNE2 (0.15), MGC-803 (0.59) and 127j: CNE2 (0.18), MGC-803 (0.67) displayed much higher antitumor activity against KB cell lines than positive control fluorouracil.53
5.6. Tyrosine kinase inhibitors
Pyrido[4,3-d]pyrimidines are known to be inhibitors of tyrosine kinases of the epidermal growth factor receptor family.74 Tetrahydropyrido[4,3-d]pyrimidine (THPP) core which have attracted considerable attention due to their biological and pharmaceutical properties such as PI3 K inhibitor 219,75 P2Y14 receptor antagonist 220,76 and other biological activities (TGR5 agonist 217,77 and PDE10 inhibitor 218,78), as shown in Fig. 9.37
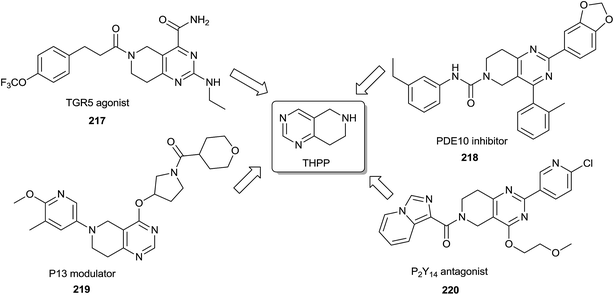 |
| Fig. 9 Structures of tyrosine kinase inhibitors. | |
The tyrosine kinase inhibitors 221 and 222 were competitive inhibitors with respect to ATP against purified epidermal growth factor (EGF) receptor tyrosine kinase and inhibited EGF receptor autophosphorylation in A431 human epidermoid carcinoma with IC50 values of 2085, 110 and 13 nM, respectively (Fig. 10).79
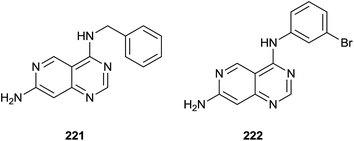 |
| Fig. 10 Chemical structures of the tyrosine kinase inhibitors. | |
5.7. Antimycobacterial activity
Compounds 3k, 3n, 3m and 160q exhibited in vitro antimycobacterial activity against Mycobacterium tuberculosis H37Rv (MTB) using agar dilution method and found to be 2.7, 2.2, 1.4 and 1.1 times, respectively, more active than ethambutol (MIC = 7.6 μM). In general, the N-benzylpyridopyrimidine-2-thiones 3 showed better activity than their N-methyl analogs 160 suggesting that lipophilicity could be an important factor for activity. In both the series 3 and 160, compounds with aryl ring bearing halogens exhibit enhanced activity.33
6. Concluding remarks
Pyrido[4,3-d]pyrimidine motif is widely found in many synthetic organic products and drug candidates with relevant biological activities. Furthermore, pyrido[4,3-d]pyrimidines are attractive candidates to synthetic chemists due to the ability of the motif to access a wide range of functional group transformations, including ring opening, reactivity of substituent attached to ring carbon atom, alkylation, arylation and benzoulation. Pyrido[4,3-d]pyrimidine compounds were obtained from pyridine derivatives such as 4-aminonicotinic acid, ethyl nicotinate, 4-amino-3-cyanopyridines, piperidin-4-one, pyridine-4-ones and 3-acetylpyridines. Pyrido[4,3-d]pyrimidines were prepared also from pyrimidine derivatives i.e. alkynyl-pyrimidines, pyrimidinones, halo-pyrimidines, pyrimidine carboxylates. Another route for the synthesis of pyrido[4,3-d]pyrimidines by applying multicomponent reaction of uracil derivatives, amines or N-substituted-4-piperidones, thiourea with aldehydes. The synthesis of binary, spiro and polycyclic heterocyclic systems incorporated pyrido[4,3-d]pyrimidine moiety were discussed. The biological importance of the investigated compounds were discussed in details, as well as, the reaction mechanisms.
A different investigations were carried out following the frequency with which papers belonging to different sections have been published during the past decade. The diagram in Fig. 11 shows that research until 1990 was focused on the synthesis of pyrido[4,3-d]pyrimidines from pyridines (Section 4.1.) and carrying out some reactions in order to evaluate their biological activity. The synthesis from pyrimidines (Section 4.2.) and multicomponet reactions (Section 4.3.) were used from 1990–2016, in which the depending on these reactions has a growth in the last decades. The synthesis of polycyclic systems (Section 4.7.) has appeared from 2005–2016 due to the valuable biological applications. Thirty five of the articles published on this moiety have evaluated the different biological activities as mentioned, in which the number of articles was duplicated in the last ten years (2005–2016). This revealed the valuable biological results of pyrido[4,3-d]pyrimidine ring system.
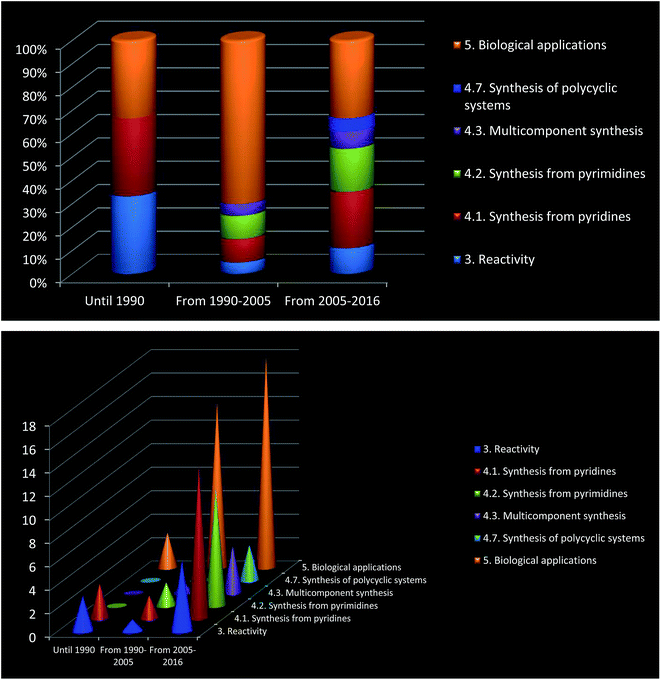 |
| Fig. 11 Occurrence of the major sections in the past decade. | |
7. Future overview
In 1957, Thomas and Wilhelm,65 have reported the synthesis of a series of 8,8-disubstituted-1,3,6-trimethyl-1,2,3,4,5,6,7,8-octahydropyrido[4,3-d]pyrimidines 164, from this time, a great numbers of proposals including the synthetic applications and reactions of pyrido[4,3-d]pyrimidines were reported. The structure studies, chemical properties, different synthetic pathways and biological importance of pyrido[4,3-d]pyrimidines, promote the researchers for the continuation of working in this field.
Abbreviations
1D and 2D NMR | 1st and 2nd dimensional nuclear magnetic resonance |
Bn | Benzyl group |
Ac | Acetyl group |
DIPEA | N,N-Diisopropylethylamine |
NaHMDS | Sodium hexamethyldisilazane |
CDI | 1,1′-Carbonyldiimidazole |
DCM or CH2Cl2 | Dichloromethane |
CH3CN | Acetonitrile |
EDCI | 1-Ethyl-3-(3-dimethylaminopropyl) carbodiimide hydrochloride |
HOBT | Hydroxybenzotriazole |
PMB group | p-Methoxybenzyl |
Zn | Zinc |
OsO4 | Osmium tetroxide |
BOC group | tert-Butyloxycarbonyl protecting group |
NaIO4 | Periodate |
TMEDA | N,N,N′,N′-Tetramethylethylenediamine |
n-BuLi | n-Butyllithium |
SAR | Structure activity relationship |
DMF-DMA | N,N-Dimethylformamide dimethyl acetal |
TFA | Trifluoacetic acid |
TEA | Triethyl amine |
Tf2O | Trifluoromethylsulfonic anhydride |
EDC | 1-Ethyl-3-[3-(dimethylamino)propyl] carbodiimide hydrochloride |
CbzCl | Carboxybenzyl chloride |
MW | Microwave |
DMF | N,N-Dimethyl formamide |
MeONa | Sodium methoxide |
K2CO3 | Potassium carbonate |
DMSO | Dimethyl sulfoxide |
CAR | The constitutive androstane receptor |
ADME profiling | Is an abbreviation in pharmacokinetics and pharmacology for “absorption, distribution, metabolism, and excretion” and describes the disposition of a pharmaceutical compound within an organism |
CNE2 | Carcinoma drug-resistant cell line |
MGC-803 | Apoptosis of human gastric cancer cell line |
MGC-803 | Gastric cancer cell line |
THPP | Tetrahydropyrido[4,3-d]pyrimidine |
References
- W. Y. Mo, Y. Y. Yao, Y. L. Shen, H. W. He and Y. C. Gu, J. Heterocycl. Chem., 2009, 46, 579–583 CrossRef CAS.
- R. E. Hacker and G. P. Jourdan, EP 0414386, 1991Chem. Abstr., 1991, 115, 71630j.
- H. Yamada, EP 0665224, 1994Chem. Abstr., 1994, 121, 230784e.
- E. F. Elselarge, J. Clarke and P. Jacob, J. Heterocycl. Chem., 1972, 9, 1113–1122 CrossRef.
- A. Rosowsky, C. E. Mota and S. F. Queener, J. Heterocycl. Chem., 1995, 32, 335–340 CrossRef CAS.
- E. S. Hussein, M. A. Z. Suhair and A. M. Mona, J. Med. Chem., 2000, 43, 2915–2921 CrossRef.
- A. M. Thompson, D. K. Murray, W. L. Elliott, D. W. Fry, J. A. Nelson, H. D. H. Showalter, B. J. Roberts, P. W. Vincent and W. A. Denny, J. Med. Chem., 1997, 40, 3915–3925 CrossRef CAS PubMed.
- D. W. Fry, J. A. Nelson, V. Slintak, P. R. Keller, G. W. Rewcastle, W. A. Denny, H. R. Zhou and A. J. Bridges, Biochem. Pharmacol., 1997, 54, 877–887 CrossRef CAS PubMed.
- J. B. Smaill, D. B. Palmer, G. W. Rewcastle, W. A. Denny, D. J. McNamara, E. M. Dobrusin, A. J. Brodges, H. R. Zhou, H. D. H. Showalter, R. T. Winters, D. W. Fry, J. M. Nelson, V. Slintak, W. L. Elliot, B. J. Roberts, P. W. Vincent and S. J. Patmore, J. Med. Chem., 1999, 42, 1803–1815 CrossRef CAS PubMed.
- A. Rosowsky, H. Bader and R. G. Moran, J. Heterocycl. Chem., 1989, 26, 509–516 CrossRef CAS.
- W. G. Rewcastle, D. B. Palmer, A. M. Thompson, A. J. Bridges, D. R. Cody, H. R. Zhou, D. W. Fry, A. McMichael and W. A. Denny, J. Med. Chem., 1996, 39, 1823–1835 CrossRef PubMed.
- A. M. Thompson, A. J. Bridges, D. W. Fry, A. J. Kraker and W. A. Denny, J. Heterocycl. Chem., 1995, 38, 3780–3788 CAS.
- M. C. Lanier, M. N. Feher, J. Ashweek, C. J. Loweth, J. K. Rueter, D. H. Slee, J. P. Williams, Y. F. Zhu, S. K. Sullivan and M. S. Brown, Bioorg. Med. Chem., 2007, 15, 5590–5603 CrossRef CAS PubMed.
- Q. Y. Ren, Z. P. Cui, H. W. He and Y. C. Gu, J. Fluorine Chem., 2007, 128, 1369–1379 CrossRef CAS.
- W. Y. Mo, G. H. Liao, T. Wang and H. W. He, J. Fluorine Chem., 2008, 129, 519–523 CrossRef CAS.
- H. W. He, T. Wang, G. H. Liao, W. Y. Mo and Q. Y. Ren, CN 1746170, 2006Chem. Abstr., 2006, 145, 124583.
- H. W. He, W. Y. Mo and Q. Y. Ren, CN 101323617, 2008Chem. Abstr., 2008, 149, 402379.
- Q. Y. Ren, Y. J. Liang, H. W. He, L. W. Fu and Y. C. Gu, Bioorg. Med. Chem. Lett., 2009, 19, 6713–6725 CrossRef CAS PubMed.
-
(a) K. M. Elattar, M. A. Abozeid, I. A. Mousa and A. El-Mekabaty, RSC Adv., 2015, 5, 106710–106753 RSC;
(b) K. M. Elattar, I. Youssef and A. A. Fadda, Synth. Commun., 2016, 46, 719–744, DOI:10.1080/00397911.2016.1166252.
- K. M. Elattar, M. A. Abozeid and H. A. Etman, Synth. Commun., 2016, 46, 93–117 CrossRef CAS.
- A. A. Fadda, E. Abdel-Galil and K. M. Elattar, Synth. Commun., 2015, 45(18), 2053–2082 CrossRef CAS.
- A. A. Fadda, S. A. El-Hadidy and K. M. Elattar, Synth. Commun., 2015, 45(24), 2765–2801 CrossRef CAS.
- A. A. Fadda, A. El-Mekabaty, I. A. Mousa and K. M. Elattar, Synth. Commun., 2014, 44(11), 1579–1599 CrossRef CAS.
- A. A. Fadda, A. El-Mekabaty and K. M. Elattar, Synth. Commun., 2013, 43(20), 2685–2719 CrossRef CAS.
- M. A. Gouda, M. A. Berghot, G. E. Abd El-Ghani, K. M. Elattar and A. M. Khalil, Turk. J. Chem., 2011, 35, 815–837 CAS.
- M. A. Gouda, A. A. Abu-Hashem, H. H. Saad and K. M. Elattar, Res. Chem. Intermed., 2016, 42, 2119–2162 CrossRef CAS.
- K. M. Elattar, R. Rabie and M. M. Hammouda, Synth. Commun., 2016 DOI:10.1080/00397911.2016.1211702.
- K. M. Elattar, Developments in the chemistry of pyrido[1,2-a]pyrimidines, LAP Lambert Academic Publishing GmbH & Co. KG, VDM Verlagsservicegesellschaft mbH, ISBN 978-3-659-82307-7, Editor, irusu, Heinrich-Böcking-Straße 6-8, D - 66121 Saarbrücken, 2015 Search PubMed.
- R. A. Bonham and F. A. Momany, J. Phys. Chem., 1963, 67, 2474–2477 CrossRef CAS.
- R. Ghosh and S. H. Simonsen, Acta Crystallogr., Sect. C: Cryst. Struct. Commun., 1993, 49, 1031–1032 CrossRef.
- Q. Ren, Z. Cui, H. He and Y. Gu, J. Fluorine Chem., 2007, 128, 1369–1375 CrossRef CAS.
- M. S. Chernovyants, I. V. Burykin, V. V. Kostrub, E. B. Tsupak, Z. A. Starikova and J. A. Kirsanova, J. Mol. Struct., 2012, 1010, 98–103 CrossRef CAS.
- S. M. Rajesh, R. S. Kumar, L. A. Libertsen, S. Perumal, P. Yogeeswari and D. Sriram, Bioorg. Med. Chem. Lett., 2011, 21, 3012–3016 CrossRef CAS PubMed.
- T. Sekiya, H. Hiranuma, T. Kanayama and S. Hata, Eur. J. Med. Chem., 1980, 15, 317–322 CAS.
- S. Asano, M. Komiya, N. Koike, E. Koga, S. Nakatani and Y. Isobe, Bioorg. Med. Chem. Lett., 2010, 20, 6696–6698 CrossRef CAS PubMed.
- A. Herrera, R. Martınez-Alvarez, R. Chioua and J. Almy, Tetrahedron Lett., 2006, 47, 5463–5465 CrossRef CAS.
- Y. Zhang, K. Zhang, M. Zhao, L. Zhang, M. Qin, S. Guo, Y. Zhao and P. Gong, Bioorg. Med. Chem., 2015, 23, 4591–4607 CrossRef CAS PubMed.
- A. G. lsmaii and D. G. Wibberley, J. Chem. Soc., 1967, 2613–2617 Search PubMed.
- O. Gerhard, US Pat., 3248395, 1966.
- M. C. Lanier, M. Feher, N. J. Ashweek, C. J. Loweth, J. K. Rueter, D. H. Slee, J. P. Williams, Y.-F. Zhu, S. K. Sullivan and M. S. Brown, Bioorg. Med. Chem., 2007, 15, 5590–5603 CrossRef CAS PubMed.
- M.-Y. Jang, S. De Jonghe, L.-J. Gaob and P. Herdewijn, Tetrahedron Lett., 2006, 47, 8917–8920 CrossRef CAS.
- H.-P. Sun, J.-M. Jia, F. Jiang, X.-L. Xu, F. Liu, X.-K. Guo, B. Cherfaoui, H.-Z. Huang, Y. Pan and Q.-D. You, Eur. J. Med. Chem., 2014, 79, 399–412 CrossRef CAS PubMed.
- M. T. Didiuk, D. A. Griffith, J. W. Benbow, K. K. C. Liu, D. P. Walker, F. C. Bi, J. Morris, A. Guzman-Perez, H. Gao, B. M. Bechle, R. M. Kelley, X. Yang, K. Dirico, S. Ahmed, W. Hungerford, J. DiBrinno, M. P. Zawistoski, S. W. Bagley, J. Li, Y. Zeng, S. Santucci, R. Oliver, M. Corbett, T. Olson, C. Chen, M. Li, V. M. Paralkar, K. A. Riccardi, D. R. Healy, A. S. Kalgutkar, T. S. Maurer, H. T. Nguyen and K. S. Frederick, Bioorg. Med. Chem. Lett., 2009, 19, 4555–4559 CrossRef CAS PubMed.
- W. Hertz and R. K. Murty, J. Org. Chem., 1961, 26, 122–125 CrossRef.
- E. Deau, D. Hédou, E. Chosson, V. Levacher and T. Besson, Tetrahedron Lett., 2013, 54, 3518–3521 CrossRef CAS.
- A. Banerjee, M. Y. Pawar, S. Patil, P. S. Yadav, P. A. Kadam, V. G. Kattige, D. S. Deshpande, P. V. Pednekar, M. K. Pisat and L. A. Gharat, Bioorg. Med. Chem. Lett., 2014, 24, 4838–4844 CrossRef CAS PubMed.
- K. V. B. Rao, R. S. Klein, M. S. P. Sarma and B. A. Otter, Nucleosides Nucleotides, 1992, 11(1), 61–83 CAS.
- H. A. Soliman, M. N. M. Yousif, M. M. Said, N. A. Hassan, M. M. Ali, H. M. Awad and F. M. E. Abdel-Megeid, Pharma Chem., 2014, 6(3), 394–410 Search PubMed.
- M. Sharma, V. Deekshith, A. Semwal, D. Sriram and P. Yogeeswari, Bioorg. Chem., 2014, 52, 69–76 CrossRef CAS PubMed.
- W. G. Zhao, S. H. Wang, W. Y. Wang and Z. M. Li, Huaxue Shiji, 2000, 22, 376–379 CAS.
- W. Mo, G. Liao, T. Wang and H. He, J. Fluorine Chem., 2008, 129, 519–523 CrossRef CAS.
- H. W. He, T. Wang, G. H. Liao, W. Y. Mo and Q. Y. Ren, CN 1746170, 2006Chem. Abstr., 2006, 145, 124583.
- W.-Y. Mo, Y.-J. Liang, Y.-C. Gu, L.-W. Fu and H.-W. He, Bioorg. Med. Chem. Lett., 2011, 21, 5975–5977 CrossRef CAS PubMed.
- I. Cikotiene, V. Kairys, R. Buksnaitiene, M. Morkunas, S. Rudys, A. Brukstus and M. X. Fernandes, Tetrahedron, 2009, 65, 5752–5759 CrossRef CAS.
- A. A. Fesenko and A. D. Shutalev, Tetrahedron, 2014, 70, 5398–5414 CrossRef CAS.
- G. Kouichiro, K. Yoshiyuki and K. Norihiko, EP 0244352 B1, 1993.
- F. Buron, J. Y. Merour, M. Akssira, G. Guillaumet and S. Routier, Eur. J. Med. Chem., 2015, 95, 76–95 CrossRef CAS PubMed.
- S. F. Yan, D. V. Dar’in, P. S. Lobanov and A. A. Potekhin, Chem. Heterocycl. Compd., 2008, 44, 457–460 CrossRef CAS.
- O. Y. Bakulina, E. M. Igumnova, D. V. Dar’in and P. S. Lobanov, Chem. Heterocycl. Compd., 2013, 49, 466–471 CrossRef CAS.
- N. A. Kheder, Y. N. Mabkhot and A. M. Farag, ARKIVOC, 2008, xvii, 107–116 Search PubMed.
- A. V. Komkov and V. A. Dorokhov, Russ. Chem. Bull., 2007, 56(11), 2290–2292 CrossRef CAS.
- M. M. Sarmah, R. Sarma, D. Prajapati and W. Hu, Tetrahedron Lett., 2013, 54, 267–271 CrossRef CAS.
- A. Basiri, V. Murugaiyah, H. Osman, R. S. Kumar, Y. Kia and M. A. Ali, Bioorg. Med. Chem., 2013, 21, 3022–3031 CrossRef CAS PubMed.
- M. M. Sarmah and D. Prajapati, Synlett, 2015, 26, 91–94 CAS.
- P. J. Thomas and W. Wilhelm, US Pat., 2802826, 1957.
- S. Abu-Melha, Chem. Process Eng., 2014, 20, 10–22 Search PubMed.
- R. M. Faty, M. S. Rashed and M. M. Youssef, Molecules, 2015, 20, 1842–1859 CrossRef PubMed.
- A. M. Mohamed, A.-G. E. Amr, M. A. Alsharari, H. R. M. Al-Qalawi, M. O. Germoush and M. A. Al-Omar, Am. J. Biochem. Biotechnol., 2011, 7(2), 43–54 CrossRef CAS.
- A. Rosowsky, H. Bader, R. G. Moran and J. H. Freisheim, J. Heterocycl. Chem., 1989, 26, 509–516 CrossRef CAS.
- A. Rosowsky, C. E. Mota and S. F. Queener, J. Heterocycl. Chem., 1995, 32, 335–340 CrossRef CAS.
- E. B. Cogan, G. B. Birrell and O. H. Griffith, Anal. Biochem., 1999, 271, 29–35 CrossRef CAS PubMed.
- J. Rumsfeld, K. Ziegelbauer and F. Spaltmann, Protein Expression Purif., 2000, 18, 303–309 CrossRef CAS PubMed.
- B. Wuyts, J. Keemink, S. De Jonghe, P. Annaert and P. Augustijns, Int. J. Pharm., 2013, 455, 19–30 CrossRef CAS PubMed.
- A. J. Bridges, W. A. Denny, D. Fry, A. Kraker, R. F. Meyer, G. W. Rewcastle and A. M. Thompson, US pat., 5654307, 1997.
- N. G. Cooke, G. S. Fernandes, A. Paulo, P. Furet, C. Hebach, K. Hoegenauer, G. Hollingworth, C. Kalis, I. Lewis, A. B. Smith and N. Soldermann, PCT Int. Appl. WO 2013088404, 2013.
- D. Guay, C. Beaulieu, M. Belley, S. N. Crane, J. DeLuca, Y. Gareau, M. Hamel, M. Henault, H. Hyjazie and S. Kargman, Bioorg. Med. Chem. Lett., 2011, 21, 2832–2835 CrossRef CAS PubMed.
- D. W. Piotrowski, K. Futatsugi, J. S. Warmus, S. T. M. Orr, K. D. Freeman-Cook, A. T. Londregan, L. Q. Wei, S. M. Jennings, M. Herr and S. B. Coffey, Med. Chem. Lett., 2013, 4, 63–68 CrossRef CAS PubMed.
- T. R. Izzat, J. B. Michael, F. Christine, F. Joy, H. Nicole, H. Sarah, K. Monika, H. K. Somang, M. Bennett, M. Georgia, J. R. John, D. S. John, S. Sujata, S. Sean, U. Jason, Y. W. Yan, J. C. Paul and D. C. Christopher, Bioorg. Med. Chem. Lett., 2012, 22, 5903–5908 CrossRef PubMed.
- D. W. Fry, J. M. Nelson, V. Slintak, P. R. Keller, G. W. Rewcastle, W. A. Denny, H. Zhou and A. J. Bridge, Biochem. Pharmacol., 1997, 54, 877–887 CrossRef CAS PubMed.
|
This journal is © The Royal Society of Chemistry 2016 |