DOI:
10.1039/C6RA12165A
(Paper)
RSC Adv., 2016,
6, 62866-62875
Reactivity of (poly)fluorobenzamides in palladium-catalysed direct arylations†
Received
10th May 2016
, Accepted 21st June 2016
First published on 22nd June 2016
Abstract
The influence of fluoro-substituents on secondary and tertiary benzamides on the regioselectivity of palladium-catalysed direct arylations was studied. With most (poly)fluoro-substituted tertiary benzamides, the arylations proceed very regioselectively at ortho-positions of the fluoro substituents using 1 mol% of air-stable palladium catalysts and PivOK/DMA as the reaction conditions. With the 3,5-difluoro-substituted secondary benzamides, quite regioselective arylations at C4-positions were observed. For these reactions, a variety of substituents on the aryl bromide, such as ester, propionyl, acetyl, formyl, nitro, nitrile, trifluoromethyl, chloro, fluoro or methyl, was tolerated. These results reveal that under our reaction conditions, fluoro substituents act as better directing groups than amides in the palladium-catalysed direct arylations.
Introduction
Several bioactive compounds contain both amide and fluoro substituents on arenes. For example, vidofludimus is an oral immunomodulator inhibiting dihydroorotate dehydrogenase, radezolid is an antibiotic developed for the treatment of multi-drug-resistant infections and flumazenil is used to treat benzodiazepine overdoses (Fig. 1). Therefore, the discovery of general simple routes for access to amide-substituted fluorobenzene derivatives has potential for medicinal chemistry.
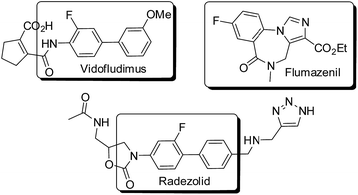 |
| Fig. 1 Examples of bioactive amide containing fluorobenzenes. | |
Pd-catalysed reactions such as Suzuki or Negishi couplings allow the formation of a wide variety of fluoro- or amide-substituted biaryls.1 However, such couplings require the preliminary synthesis of organometallic derivatives. Moreover, they provide a stoichiometric amount of metallic salts as side-products. Fagnou et al. reported in 2006 the Pd-catalysed direct arylation2 of polyfluorobenzenes,3 using aryl halides as coupling partners (Scheme 1a). Since these results, the palladium-catalysed direct arylation of polyfluorobenzenes with aryl halides has proved to be a powerful method for the synthesis of a variety of polyfluoro-substituted biaryls.4 On the other hand, Miura et al. reported in 2000 the Pd-catalysed C2-arylation of benzamides (Scheme 1b).5 Then, several groups extended the C2-arylation of benzamides.6 The reports of Fagnou and Miura revealed that both fluoro and amide-substituents on benzenes act as directing groups for such couplings. However, little is known on the reactivity of fluoro-substituted benzamides in palladium-catalysed direct arylation. Chatani et al. reported in 2014 that the Ni-catalysed direct arylation of a 4-fluorobenzamide containing an 8-aminoquinoline moiety proceeds at ortho-position of the amide group (Scheme 1c).7 An α-amino ester (or acid) moiety also acts as directing group for selective Pd-catalysed ortho-C–H activation of fluorobenzamides (Scheme 1d and e).8 Weinreb amide was also successfully employed as directing group to promote the C2-arylation of a 4-fluorobenzamide derivative (Scheme 1f).9 Finally, Ackermann et al. reported in 2015 Co-catalysed direct C2-arylation of a 4-fluorobenzamide (Scheme 1g).10 It should be mentioned that to our knowledge, so far no examples of direct arylation at ortho-position of fluoro-substituents of fluorobenzamides has been reported. Therefore, the discovery of a procedure allowing a general access with a high regioselectivity to such biaryls is desirable.
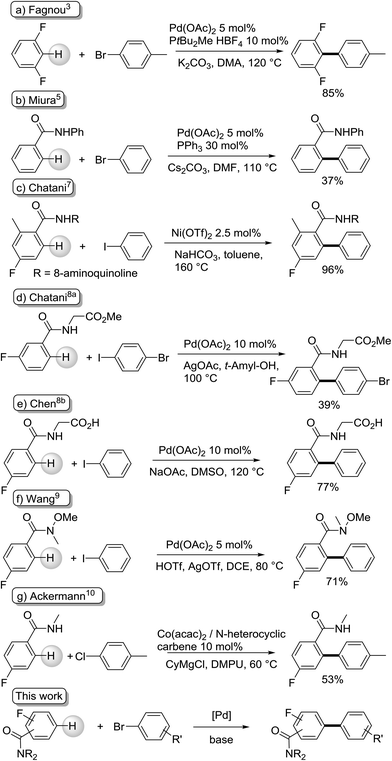 |
| Scheme 1 Metal-catalysed direct arylation of fluoro- and/or amide-substituted benzenes. | |
Herein, starting from a set of (poly)fluorobenzamides as reactants, we report (i) on the reactivity and regioselectivity of benzene derivatives containing both fluoro and secondary or tertiary amide substituents; (ii) on the access to a variety of amide-substituted (poly)fluorobiphenyls using a variety of aryl bromides as coupling partners (Scheme 1, bottom).
Results and discussion
We first examined the reaction of N,N-diethylfluorobenzamides with 4-bromonitrobenzene (Scheme 2). N,N-Diethyl-4-fluorobenzamide and 4-bromonitrobenzene in the presence of 1 mol% PdCl2 using PivOK as base/ligand, gave regioselectively 1, revealing a better directing effect of the fluoro-substituent than that of the tertiary amide substituent (Scheme 2, top). It should be mentioned that when the fluoro substituent on N,N-diethyl-4-fluorobenzamide was replaced by chloro- or methoxy-substituents, under the same conditions using again 4-bromonitrobenzene as coupling partner, only traces (<5%) of coupling products were detected by GC/MS analysis of the crude mixtures. These results confirm the strong directing and activating effect of a fluoro substituent on benzenes. From a mixture of N,N-diethyl-3-fluorobenzamide and 4-bromonitrobenzene, under the same reaction conditions, only the coupling product 2, arising from coupling at para-position from the amide substituent was isolated (Scheme 2, middle). N,N-Diethyl-2-fluorobenzamide and 4-bromonitrobenzene was also arylated regioselectively at the ortho-position of the fluoro-substituent affording 3 in 28% yield (Scheme 2, bottom). These three reactions gave poor yields due to partial conversions of the aryl bromides, however they clearly demonstrate that, under these conditions, fluoro-substituents act as better directing groups than tertiary amides in the Pd-catalysed direct arylation of fluorobenzamides. According to Gorelsky,11c the fluorine substituents on arenes, especially at the ortho-position, favour a Concerted Metallation Deprotonation (CMD) transformation.11
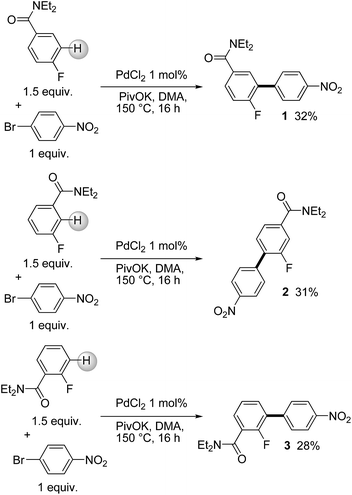 |
| Scheme 2 Regioselectivity of palladium-catalysed direct arylation of a set of N,N-diethylfluorobenzamides. | |
The use of N,N-diethyl-3,5-difluorobenzamide and 4-bromobenzonitrile as the coupling partners in the presence of 1 mol% Pd(OAc)2 catalyst and AcOK as the base at 150 °C in DMA selectively gave the coupling product 4a, arising from a regioselective coupling at C4 position, which is flanked by the two fluoro substituents, in 63% yield with 100% conversion of the aryl bromide (Table 1, entry 1). It is known that the presence of additional fluoro substituents on the arene amplifies the acidity of the C–H bond at their ortho-position and leads to a lower CMD activation energy.11c The influence of some reaction conditions was investigated. The use of PivOK as the base for the reaction with 4-bromobenzonitrile allowed to increase the yield in 4a from 63% to 77% by reduction of 4-bromobenzonitrile homo-coupling side-product formation (Table 1, entry 2). On the contrary, the use of K2CO3 as base, which had been found to be very effective by Fagnou and co-workers for the arylation of 1,3-difluorobenzene using 5 mol% Pd(OAc)2 associated to 10 mol% PtBu2Me·HBF4 as catalyst (Scheme 1a), gave 4a in only 24% yield due to a poor conversion of 4-bromobenzonitrile (Table 1, entry 3).3 Then, we examined the influence of several other reaction conditions for this coupling. The use of 1 mol% PdCl2 catalyst instead of Pd(OAc)2 gave 4a in a slightly higher yield of 80% (Table 1, entry 4). On the other hand, the use of both DMF and NMP as the solvents, with PivOK as the base, afforded 4a in lower yields, and both xylene and pentan-1-ol were ineffective for this reaction (Table 1, entries 5–8). A lower catalyst loading of 0.5 mol% of PdCl2 was also employed using DMA as the solvent, affording 4a in 79% yield (Table 1, entry 9). In all cases, no formation of the other regioisomer 4b was detected by GC/MS analysis of the crude mixtures; whereas, some 4-bromobenzonitrile homo-coupling side-product formation was observed.
Table 1 Influence of the reaction conditions for the palladium-catalysed direct arylation of N,N-diethyl-3,5-difluorobenzamide with 4-bromobenzonitrilea
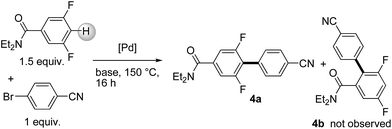
|
Entry |
Catalyst (mol%) |
Base |
Solvent |
Conv. (%) |
Yield in 4a (%) |
4-Bromobenzonitrile (1 equiv.), N,N-diethyl-3,5-difluorobenzamide (1.5 equiv.), base (2 equiv.), 16 h, 150 °C. |
1 |
Pd(OAc)2 (1) |
AcOK |
DMA |
100 |
63 |
2 |
Pd(OAc)2 (1) |
PivOK |
DMA |
100 |
77 |
3 |
Pd(OAc)2 (1) |
K2CO3 |
DMA |
46 |
24 |
4 |
PdCl2 (1) |
PivOK |
DMA |
100 |
80 |
5 |
PdCl2 (1) |
PivOK |
DMF |
100 |
58 |
6 |
PdCl2 (1) |
PivOK |
NMP |
100 |
67 |
7 |
PdCl2 (1) |
PivOK |
Xylene |
<10 |
<5 |
8 |
PdCl2 (1) |
PivOK |
Pentan-1-ol |
85 |
<5 |
9 |
PdCl2 (0.5) |
PivOK |
DMA |
100 |
79 |
In order to assess the full scope of the aryl bromide coupling partner for reaction with N,N-diethyl-3,5-difluorobenzamide, we undertook a systematic study with a set of (hetero)aryl bromides (Scheme 3). The electron-deficient para-substituted aryl bromides, 4-bromopropiophenone, 4-bromonitrobenzene, ethyl 4-bromobenzoate and 4-trifluoromethylbromobenzene regioselectively gave the desired products 5–8 in 81–88% yields. A high yield of 83% in 9 was also obtained using 4-chlorobromobenzene as reaction partner. Moreover, no cleavage of the C–Cl bond was observed, allowing further transformations. Even 4-fluorobromobenzene was successfully coupled with N,N-diethyl-3,5-difluorobenzamide to provide 10 in 76% yield. meta-Substituted aryl bromide, 3-nitrobenzene gave 11 in 88% yield. Both 2-bromonaphthalene and 2-bromobenzonitrile also gave the desired products 12 and 13 in high yields. We also employed two heteroaryl bromides. From 3-bromopyridine and 3-bromoquinoline, regioselective reactions were also observed affording 14 and 15 in 61% and 87% yields, respectively. Electron-rich aryl bromide, 4-bromotoluene also led to a good yield of 78% in the biaryl derivative 16.
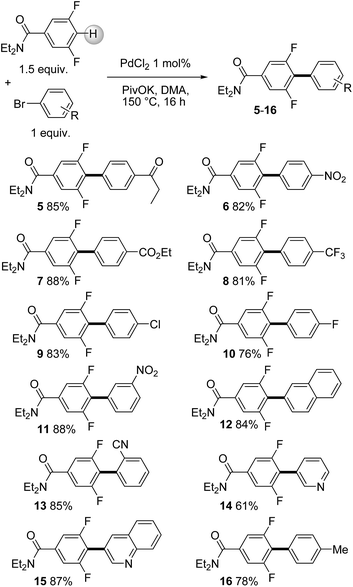 |
| Scheme 3 Palladium-catalysed direct 4-arylation of N,N-diethyl-3,5-difluorobenzamide. | |
We then studied the reactivity of N,N-diethyl-2,4-difluorobenzamide and N,N-diethyl-2,4,5-trifluorobenzamide (Scheme 4). Again, regioselective arylations at the C–H bonds flanked by the two fluorine atoms was observed. The two electron-deficient aryl bromides, 4-bromonitrobenzene and 4-bromobenzonitrile were successfully employed with N,N-diethyl-2,4-difluorobenzamide, affording 17 and 18 in 79% and 81% yields, respectively. N,N-Diethyl-2,4,5-trifluorobenzamide was regioselectively arylated by 4-bromobenzonitrile, 2-(4-bromophenyl)acetonitrile and 3-bromoquinoline at C3-position to provide 19–21 in 53–81% yields. An additional atom at C5-position of the benzamide derivative exhibits a minor effect on the reactivity and has no effect on the regioselectivity, as C6-arylated benzamide was not detected.
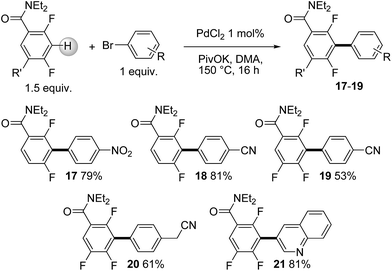 |
| Scheme 4 Palladium-catalysed direct 3-arylation of N,N-diethyl-2,4-difluorobenzamide and N,N-diethyl-2,4,5-trifluorobenzamide. | |
The arylation of N,N-diethyl-2,3-difluorobenzamide led to a regioselective arylation in favour of the C–H bond flanked by one fluorine atom (Scheme 5). From 4-bromoacetophenone and 4-bromonitrobenzene, products 22 and 23 were obtained in 61% and 64% yields, respectively. Surprisingly, N,N-diethyl-3,4-difluorobenzamide was regioselectively arylated at C5 affording 24 in low yield. These results confirm that a tertiary amide substituent on benzene acts as a poor directing group compared to fluoro substituents.
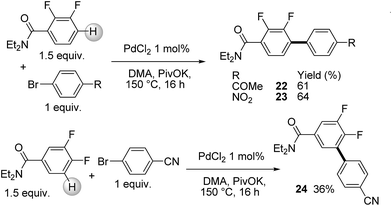 |
| Scheme 5 Palladium-catalysed direct arylation of N,N-diethyl-2,3-difluorobenzamide and N,N-diethyl-3,4-difluorobenzamide. | |
In order to compare the reactivity of tertiary and secondary amides, the arylation of both N-n-butyl-3,5-difluorobenzamide and N-tert-butyl-3,5-difluorobenzamide was explored (Table 2 and Scheme 6). With both substrates, the formation of mixtures of C2- and C4-arylated benzamides was observed. The regioselectivity partially depends on the reaction conditions. The reaction of N-n-butyl-3,5-difluorobenzamide with 4-bromobenzonitrile using PivOK as base in DMA gave a mixture of 25a and 25b in 70
:
30 ratio (Table 2, entry 1). Under these conditions, the C–H bond flanked by two fluorine atoms was the most reactive. The use of a mixture of PivOK and Cs2CO3 as base in DMA led to products 25a and 25b in 10
:
90 ratio, but with a low conversion of 4-bromobenzonitrile. Less polar solvents such as xylene or chlorobenzene afforded larger amounts of product 25b than the reactions performed in DMA (Table 2, entries 7 and 10). These results seem to indicate that the formation of regioisomer 25b comes from a coordination assisted mechanism due to amide deprotonation by the base;12 whereas, the formation of 25a certainly proceeds via a concerted-metallation–deprotonation mechanism (CMD).
Table 2 Influence of the reaction conditions for the palladium-catalysed direct arylation of N-n-butyl-3,5-difluorobenzamide with 4-bromobenzonitrilea
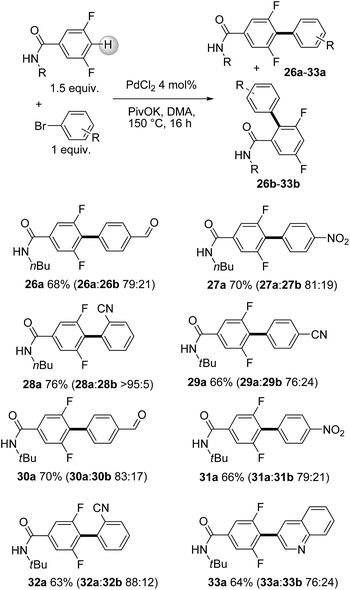 |
| Scheme 6 Palladium-catalysed direct 4-arylation of N-n-butyl-3,5-difluorobenzamide and N-tert-butyl-3,5-difluorobenzamide. | |
Then, we studied the reactivity of N-n-butyl-3,5-difluorobenzamide with a set of aryl bromides (Scheme 6). In all cases, mixtures of C2- and C4-arylated products were obtained. The highest selectivity was obtained with quite congested 2-bromobenzonitrile, as 28a was produced in 95% regioselectivity and in 76% yield. The use of N-tert-butyl-3,5-difluorobenzamide led to quite similar regioselectivities than N-n-butyl-3,5-difluorobenzamide. For example, it's coupling with 4-bromobenzonitrile gave 29a and 29b in 76
:
24 ratio. In the presence of 2-bromobenzonitrile, a regioselectivity of 88% in 32a was obtained.
It should be mentioned that, under the same reaction conditions, the primary benzamide 3,5-difluorobenzamide was found to be completely unreactive, as no formation of 34 was detected (Scheme 7). The Hammet constants14 of amide substituents on benzenes (Scheme 7) seems to reveal that the difference of reactivity of primary and secondary difluorobenzamides does not come from electronic factors, but more likely from the poisoning of the catalyst.
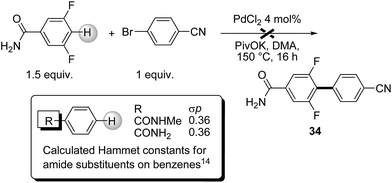 |
| Scheme 7 Attempt of palladium-catalysed direct arylation of 3,5-difluorobenzamide. | |
To better understand the amine substituent influence on the catalyst for such couplings, we performed a competition reaction (Scheme 8). From an equimolar mixture of 3,5-difluorobenzamide and N,N-diethyl-3,5-difluorobenzamide using 4-bromobenzonitrile as the coupling partner, in the presence of 4 mol% PdCl2, only the formation of 2a arising from coupling with N,N-diethyl-3,5-difluorobenzamide was detected in very low yield. The partial conversion of 4-bromobenzonitrile reveals that the non-reactivity of 3,5-difluorobenzamide is certainly due to a partial poisoning of the catalyst by 3,5-difluorobenzamide.
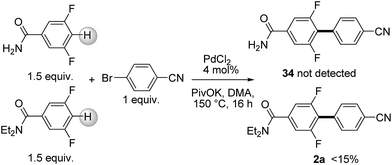 |
| Scheme 8 Competition reaction using an equimolar mixture of 3,5-difluorobenzamide and N,N-diethyl-3,5-difluorobenzamide. | |
Conclusions
In summary, the Pd-catalysed direct arylation of 3,5-difluoro-substituted secondary benzamides, affords mixtures of regioisomers; and a 3,5-difluoro-substituted primary benzamide was found to be unreactive. On the other hand, fluoro substituents act as better directing groups than tertiary amides in the palladium-catalysed direct arylation of (poly)fluorobenzamides. With tertiary benzamides, high regioselectivities in favour of arylations at the C–H bond flanked by the fluorine atoms were obtained. To our knowledge these are the first examples of metal-catalysed direct arylations at ortho-position of fluoro-substituents of (poly)fluorobenzamides. These arylations were performed using only 1 mol% of a commercially available air-stable catalyst. Moreover, a variety of substituents on the aryl bromide such as ester, propionyl, acetyl, formyl, nitro, nitrile, trifluoromethyl, chloro, fluoro or methyl was tolerated. The major by-products of such couplings are a base associated to HBr; moreover the method avoids the preliminary preparation of organometallic derivatives reducing the number of steps to prepare these compounds. For these reasons, this process gives an simple and environmentally attractive access to arylated (poly)fluorobenzamides.
Experimental
DMA (N,N-dimethylacetamide) (99%) was purchased from Acros. PdCl2 (99%) was purchased from Aldrich. These compounds were not purified before use.
General procedure for the synthesis of the (poly)fluorobenzamides. The reaction of the (poly)fluorobenzoyl chloride (10 mmol) and primary or secondary amine (10 mmol) at 25 °C during 16 h in NEt3 (15 mL) and CH2Cl2 (15 mL) affords the (poly)fluorobenzamide after evaporation of the solvent and filtration on silica gel.
N,N-Diethyl-2,4,5-trifluorobenzamide. The reaction of 2,4,5-trifluorobenzoyl chloride (1.94 g, 10 mmol) and diethylamine (0.730 g, 10 mmol) affords N,N-diethyl-2,4,5-trifluorobenzamide in 78% (1.80 g) yield. 1H NMR (400 MHz, CDCl3, 25 °C): δ = 7.14 (td, J = 6.0, 5.0 Hz, 1H), 6.94 (dd, J = 6.2, 6.0 Hz, 1H), 3.51 (q, J = 7.6 Hz, 2H), 3.18 (q, J = 7.6 Hz, 2H), 1.22 (t, J = 7.6 Hz, 3H), 1.07 (t, J = 7.6 Hz, 3H). 13C NMR (100 MHz, CDCl3, 25 °C): δ = 163.9, 153.2 (ddd, J = 247.9, 19.5, 2.8 Hz), 150.5 (ddd, J = 254.0, 14.4, 12.0 Hz), 147.0 (ddd, J = 248.0, 12.8, 3.8 Hz), 121.3 (dt, J = 21.2, 5.7 Hz), 116.7 (ddd, J = 19.1, 5.7, 1.4 Hz), 106.1 (dd, J = 27.8, 21.0 Hz), 43.1, 39.5, 14.0, 12.7.
N,N-Diethyl-3,4-difluorobenzamide. The reaction of 3,4-difluorobenzoyl chloride (1.76 g, 10 mmol) and diethylamine (0.730 g, 10 mmol) affords N,N-diethyl-2,4,5-trifluorobenzamide in 72% (1.53 g) yield. 1H NMR (400 MHz, CDCl3, 25 °C): δ = 7.22–7.05 (m, 2H), 3.46 (q, J = 7.6 Hz, 2H), 3.22 (q, J = 7.6 Hz, 2H), 1.25–1.00 (m, 6H). 13C NMR (100 MHz, CDCl3, 25 °C): δ = 168.8, 151.7 (dd, J = 248.0, 12.0 Hz), 150.1 (dd, J = 250.7, 11.9 Hz), 134.0 (m), 122.8 (dd, J = 6.6, 4.0 Hz), 117.4 (d, J = 17.5 Hz), 116.1 (d, J = 18.4 Hz), 43.3, 39.5, 13.9, 12.7.
General procedure for the synthesis of 1–33. The reaction of the aryl bromide (1 mmol), (poly)fluorobenzamide derivative (1.5 mmol) and PivOK (0.248 g, 2 mmol) at 150 °C during 16 h in DMA (3 mL) in the presence of PdCl2 (1.8 mg, 0.01 mmol or 7.1 mg 0.04 mmol) (see schemes) under argon affords the arylation product after evaporation of the solvent and filtration on silica gel.
N,N-Diethyl-6-fluoro-4′-nitrobiphenyl-3-carboxamide (1). From 4-bromonitrobenzene (0.202 g, 1 mmol) and N,N-diethyl-4-fluorobenzamide (0.292 g, 1.5 mmol), 1 was obtained in 32% (0.101 g) yield. 1H NMR (400 MHz, CDCl3, 25 °C): δ = 8.33 (d, J = 8.2 Hz, 2H), 7.74 (d, J = 8.2 Hz, 2H), 7.53 (dd, J = 7.3, 2.1 Hz, 1H), 7.48–7.42 (m, 1H), 7.25 (dd, J = 10.3, 8.2 Hz, 1H), 3.56 (m, 2H), 3.34 (m, 2H), 1.50–1.00 (m, 6H). 13C NMR (100 MHz, CDCl3, 25 °C): δ = 169.7, 159.8 (d, J = 252.4 Hz), 147.5, 141.5, 134.1 (d, J = 4.0 Hz), 129.9 (d, J = 3.4 Hz), 129.1 (d, J = 3.2 Hz), 128.7 (d, J = 8.8 Hz), 127.1 (d, J = 13.6 Hz), 123.9, 116.6 (d, J = 23.3 Hz), 43.3, 39.5, 14.2, 12.9. Elemental analysis: calcd (%) for C17H17FN2O3 (316.32): C 64.55, H 5.42; found: C 64.40, H 5.37.
N,N-Diethyl-2-fluoro-4′-nitrobiphenyl-4-carboxamide (2). From 4-bromonitrobenzene (0.202 g, 1 mmol) and N,N-diethyl-3-fluorobenzamide (0.292 g, 1.5 mmol), 2 was obtained in 31% (0.098 g) yield in 90% purity. 1H NMR (400 MHz, CDCl3, 25 °C): δ = 8.34 (d, J = 8.2 Hz, 2H), 7.74 (d, J = 8.2 Hz, 2H), 7.53 (t, J = 7.7 Hz, 1H), 7.35–7.22 (m, 2H), 3.59 (bs, 2H), 3.35 (bs, 2H), 1.30–1.18 (m, 6H). 13C NMR (100 MHz, CDCl3, 25 °C): δ = 169.1, 159.3 (d, J = 251.7 Hz), 147.5, 141.5, 139.6 (d, J = 7.5 Hz), 130.8 (d, J = 3.1 Hz), 129.8 (d, J = 3.3 Hz), 127.6 (d, J = 13.2 Hz), 123.8, 122.7 (d, J = 3.7 Hz), 114.8 (d, J = 24.3 Hz), 43.4, 39.5, 14.3, 12.9.
N,N-Diethyl-2-fluoro-4′-nitrobiphenyl-3-carboxamide (3). From 4-bromonitrobenzene (0.202 g, 1 mmol) and N,N-diethyl-2-fluorobenzamide (0.292 g, 1.5 mmol), 3 was obtained in 28% (0.088 g) yield. 1H NMR (400 MHz, CDCl3, 25 °C): δ = 8.33 (d, J = 8.2 Hz, 2H), 7.74 (d, J = 8.2 Hz, 2H), 7.51 (td, J = 7.5, 1.8 Hz, 1H), 7.41 (td, J = 7.5, 1.8 Hz, 1H), 7.34 (t, J = 7.6 Hz, 1H), 3.63 (m, 2H), 3.29 (q, J = 7.6 Hz, 2H), 1.30 (t, J = 7.6 Hz, 3H), 1.14 (t, J = 7.6 Hz, 3H). 13C NMR (100 MHz, CDCl3, 25 °C): δ = 165.6, 154.9 (d, J = 250.1 Hz), 147.5, 141.6, 131.1 (d, J = 2.8 Hz), 129.9 (d, J = 3.3 Hz), 128.9 (d, J = 4.4 Hz), 127.4 (d, J = 13.5 Hz), 126.5 (d, J = 20.0 Hz), 125.1 (d, J = 4.1 Hz), 123.8, 43.1, 39.3, 14.1, 12.8. Elemental analysis: calcd (%) for C17H17FN2O3 (316.32): C 64.55, H 5.42; found: C 64.69, H 5.57.
4′-Cyano-N,N-diethyl-2,6-difluorobiphenyl-4-carboxamide (4a). From 4-bromobenzonitrile (0.182 g, 1 mmol) and N,N-diethyl-3,5-difluorobenzamide (0.320 g, 1.5 mmol), 4a was obtained in 80% (0.251 g) yield. 1H NMR (400 MHz, CDCl3, 25 °C): δ = 7.76 (d, J = 8.1 Hz, 2H), 7.59 (d, J = 8.1 Hz, 2H), 7.05 (d, J = 7.8 Hz, 2H), 3.55 (q, J = 7.6 Hz, 2H), 3.33 (q, J = 7.6 Hz, 2H), 1.25 (t, J = 7.6 Hz, 3H), 1.20 (t, J = 7.6 Hz, 3H). 13C NMR (100 MHz, CDCl3, 25 °C): δ = 167.9, 159.5 (dd, J = 252.4, 6.8 Hz), 139.6 (t, J = 9.0 Hz), 133.3, 132.1, 131.0 (t, J = 2.0 Hz), 118.4, 117.4 (t, J = 18.1 Hz), 112.4, 110.3 (d, J = 27.6 Hz), 43.3, 39.5, 14.2, 12.8. Elemental analysis: calcd (%) for C18H16F2N2O (314.33): C 68.78, H 5.13; found: C 68.68, H 5.21.
N,N-Diethyl-2,6-difluoro-4′-propionylbiphenyl-4-carboxamide (5). From 4-bromopropiophenone (0.213 g, 1 mmol) and N,N-diethyl-3,5-difluorobenzamide (0.320 g, 1.5 mmol), 5 was obtained in 85% (0.293 g) yield. 1H NMR (400 MHz, CDCl3, 25 °C): δ = 8.06 (d, J = 8.1 Hz, 2H), 7.58 (d, J = 8.1 Hz, 2H), 7.04 (d, J = 7.2 Hz, 2H), 3.56 (m, 2H), 3.34 (m, 2H), 3.05 (q, J = 7.6 Hz, 2H), 1.30–1.15 (m, 9H). 13C NMR (100 MHz, CDCl3, 25 °C): δ = 200.2, 168.2, 159.7 (dd, J = 251.8, 7.1 Hz), 138.9 (t, J = 9.0 Hz), 136.7, 133.1, 130.5, 127.9, 118.3 (t, J = 18.5 Hz), 110.2 (d, J = 27.8 Hz), 43.3, 39.6, 31.9, 14.3, 12.8, 8.2. Elemental analysis: calcd (%) for C20H21F2NO2 (345.38): C 69.55, H 6.13; found: C 69.30, H 6.04.
N,N-Diethyl-2,6-difluoro-4′-nitrobiphenyl-4-carboxamide (6). From 4-bromonitrobenzene (0.202 g, 1 mmol) and N,N-diethyl-3,5-difluorobenzamide (0.320 g, 1.5 mmol), 6 was obtained in 82% (0.274 g) yield. 1H NMR (400 MHz, CDCl3, 25 °C): δ = 8.32 (d, J = 8.1 Hz, 2H), 7.67 (d, J = 8.1 Hz, 2H), 7.08 (d, J = 7.7 Hz, 2H), 3.56 (m, 2H), 3.34 (m, 2H), 1.25 (m, 3H), 1.20 (m, 3H). 13C NMR (100 MHz, CDCl3, 25 °C): δ = 167.9, 159.5 (dd, J = 252.4, 6.8 Hz), 147.7, 139.8 (t, J = 9.0 Hz), 135.2, 131.3 (t, J = 2.1 Hz), 123.5, 117.1 (t, J = 18.1 Hz), 110.4 (d, J = 27.6 Hz), 43.3, 39.6, 14.3, 12.8. Elemental analysis: calcd (%) for C17H16F2N2O3 (334.32): C 61.07, H 4.82; found: C 61.31, H 4.67.
Ethyl 4′-(diethylcarbamoyl)-2′,6′-difluorobiphenyl-4-carboxylate (7). From ethyl 4-bromobenzoate (0.229 g, 1 mmol) and N,N-diethyl-3,5-difluorobenzamide (0.320 g, 1.5 mmol), 7 was obtained in 88% (0.318 g) yield. 1H NMR (400 MHz, CDCl3, 25 °C): δ = 8.16 (d, J = 8.1 Hz, 2H), 7.70 (d, J = 8.1 Hz, 2H), 7.04 (d, J = 7.2 Hz, 2H), 4.40 (t, J = 7.6 Hz, 2H), 3.56 (m, 2H), 3.34 (m, 2H), 1.42 (t, J = 7.6 Hz, 3H), 1.28–1.17 (m, 6H). 13C NMR (100 MHz, CDCl3, 25 °C): δ = 168.2, 166.1, 159.7 (dd, J = 251.8, 7.1 Hz), 138.9 (t, J = 9.0 Hz), 133.0, 130.5, 130.2 (t, J = 1.8 Hz), 129.5, 118.4 (t, J = 18.5 Hz), 110.2 (d, J = 27.8 Hz), 61.2, 43.3, 39.5, 15.2, 14.3, 12.8. Elemental analysis: calcd (%) for C20H21F2NO3 (361.38): C 66.47, H 5.86; found: C 66.70, H 5.99.
N,N-Diethyl-2,6-difluoro-4′-(trifluoromethyl)biphenyl-4-carboxamide (8). From 4-trifluoromethylbromobenzene (0.225 g, 1 mmol) and N,N-diethyl-3,5-difluorobenzamide (0.320 g, 1.5 mmol), 8 was obtained in 81% (0.289 g) yield. 1H NMR (400 MHz, CDCl3, 25 °C): δ = 7.75 (d, J = 8.1 Hz, 2H), 7.61 (d, J = 8.1 Hz, 2H), 7.04 (d, J = 7.3 Hz, 2H), 3.56 (m, 2H), 3.34 (m, 2H), 1.30–1.18 (m, 6H). 13C NMR (100 MHz, CDCl3, 25 °C): δ = 168.2 (t, J = 2.4 Hz), 159.7 (dd, J = 151.9, 7.0 Hz), 139.2 (t, J = 9.0 Hz), 132.2, 130.7, 130.6 (q, J = 32.6 Hz), 124.0 (q, J = 272.2 Hz), 125.3 (q, J = 3.7 Hz), 117.9 (t, J = 18.4 Hz), 110.3 (d, J = 19.9 Hz), 43.3, 39.5, 14.2, 12.8. Elemental analysis: calcd (%) for C18H16F5NO (357.32): C 60.50, H 4.51; found: C 60.34, H 4.32.
4′-Chloro-N,N-diethyl-2,6-difluorobiphenyl-4-carboxamide (9). From 4-bromochlorobenzene (0.191 g, 1 mmol) and N,N-diethyl-3,5-difluorobenzamide (0.320 g, 1.5 mmol), 9 was obtained in 83% (0.268 g) yield. 1H NMR (400 MHz, CDCl3, 25 °C): δ = 7.47 (d, J = 8.1 Hz, 2H), 7.42 (d, J = 8.1 Hz, 2H), 7.04 (d, J = 7.3 Hz, 2H), 3.56 (m, 2H), 3.34 (m, 2H), 1.30–1.18 (m, 6H). 13C NMR (100 MHz, CDCl3, 25 °C): δ = 168.3, 159.7 (dd, J = 251.2, 7.1 Hz), 138.5 (t, J = 9.0 Hz), 134.7, 131.5 (t, J = 1.8 Hz), 128.6, 126.8, 118.1 (t, J = 18.5 Hz), 110.1 (d, J = 28.0 Hz), 43.3, 39.5, 14.2, 12.8. Elemental analysis: calcd (%) for C17H16ClF2NO (323.76): C 63.06, H 4.98; found: C 63.24, H 5.18.
4′-Fluoro-N,N-diethyl-2,6-difluorobiphenyl-4-carboxamide (10). From 4-bromofluorobenzene (0.175 g, 1 mmol) and N,N-diethyl-3,5-difluorobenzamide (0.320 g, 1.5 mmol), 10 was obtained in 76% (0.233 g) yield. 1H NMR (400 MHz, CDCl3, 25 °C): δ = 7.50–7.40 (m, 2H), 7.16 (t, J = 8.1 Hz, 2H), 7.04 (d, J = 7.3 Hz, 2H), 3.56 (m, 2H), 3.34 (m, 2H), 1.30–1.18 (m, 6H). 13C NMR (100 MHz, CDCl3, 25 °C): δ = 168.4 (t, J = 2.4 Hz), 162.3 (d, J = 248.5 Hz), 159.7 (dd, J = 250.8, 7.2 Hz), 138.3 (t, J = 9.0 Hz), 132.0 (dt, J = 8.2, 1.9 Hz), 124.3 (d, J = 3.4 Hz), 118.3 (t, J = 18.5 Hz), 115.5 (d, J = 21.7 Hz), 110.2 (d, J = 28.2 Hz), 43.3, 39.5, 14.2, 12.8. Elemental analysis: calcd (%) for C17H16F3NO (307.31): C 66.44, H 5.25; found: C 66.57, H 5.17.
N,N-Diethyl-2,6-difluoro-3′-nitrobiphenyl-4-carboxamide (11). From 3-bromonitrobenzene (0.202 g, 1 mmol) and N,N-diethyl-3,5-difluorobenzamide (0.320 g, 1.5 mmol), 11 was obtained in 88% (0.294 g) yield. 1H NMR (400 MHz, CDCl3, 25 °C): δ = 8.36 (s, 1H), 8.29 (d, J = 8.1 Hz, 1H), 7.81 (d, J = 7.7 Hz, 1H), 7.67 (t, J = 7.7 Hz, 1H), 7.08 (d, J = 7.4 Hz, 2H), 3.56 (m, 2H), 3.34 (m, 2H), 1.30–1.15 (m, 6H). 13C NMR (100 MHz, CDCl3, 25 °C): δ = 168.0, 159.6 (dd, J = 252.4, 6.8 Hz), 148.2, 139.5 (t, J = 9.0 Hz), 136.3, 130.1, 129.4, 125.3 (t, J = 2.1 Hz), 123.4, 116.7 (t, J = 18.1 Hz), 110.3 (d, J = 27.6 Hz), 43.3, 39.6, 14.2, 12.8. Elemental analysis: calcd (%) for C17H16F2N2O3 (334.32): C 61.07, H 4.82; found: C 61.27, H 4.80.
N,N-Diethyl-3,5-difluoro-4-(naphthalen-2-yl)benzamide (12). From 2-bromonaphthalene (0.207 g, 1 mmol) and N,N-diethyl-3,5-difluorobenzamide (0.320 g, 1.5 mmol), 12 was obtained in 84% (0.284 g) yield. 1H NMR (400 MHz, CDCl3, 25 °C): δ = 8.01 (s, 1H), 7.95 (d, J = 8.1 Hz, 1H), 7.93–7.87 (m, 2H), 7.59 (d, J = 8.1 Hz, 1H), 7.57–7.51 (m, 2H), 7.11 (d, J = 7.4 Hz, 2H), 3.56 (m, 2H), 3.34 (m, 2H), 1.30–1.15 (m, 6H). 13C NMR (100 MHz, CDCl3, 25 °C): δ = 168.4, 159.9 (dd, J = 252.4, 6.8 Hz), 138.2 (t, J = 9.0 Hz), 132.9, 129.8, 128.2, 127.8, 127.6, 127.4, 126.6, 126.3, 125.8, 119.3 (t, J = 18.1 Hz), 110.0 (d, J = 27.6 Hz), 43.3, 39.5, 14.1, 12.7. Elemental analysis: calcd (%) for C21H19F2NO (339.38): C 74.32, H 5.64; found: C 74.17, H 5.47.
2′-Cyano-N,N-diethyl-2,6-difluorobiphenyl-4-carboxamide (13). From 2-bromobenzonitrile (0.182 g, 1 mmol) and N,N-diethyl-3,5-difluorobenzamide (0.320 g, 1.5 mmol), 13 was obtained in 85% (0.267 g) yield. 1H NMR (400 MHz, CDCl3, 25 °C): δ = 7.83 (d, J = 8.1 Hz, 1H), 7.71 (t, J = 7.8 Hz, 1H), 7.56 (t, J = 7.7 Hz, 1H), 7.49 (d, J = 8.1 Hz, 1H), 7.08 (d, J = 7.4 Hz, 2H), 3.56 (m, 2H), 3.34 (m, 2H), 1.35–1.15 (m, 6H). 13C NMR (100 MHz, CDCl3, 25 °C): δ = 168.0, 159.6 (dd, J = 252.4, 6.8 Hz), 140.7 (t, J = 8.8 Hz), 133.1, 132.7, 132.4, 131.7, 129.2, 117.4, 115.9 (t, J = 19.7 Hz), 113.9, 110.3 (d, J = 27.3 Hz), 43.4, 39.5, 14.2, 12.8. Elemental analysis: calcd (%) for C18H16F2N2O (314.33): C 68.78, H 5.13; found: C 68.89, H 5.04.
N,N-Diethyl-3,5-difluoro-4-(pyridin-3-yl)benzamide (14). From 3-bromopyridine (0.158 g, 1 mmol) and N,N-diethyl-3,5-difluorobenzamide (0.320 g, 1.5 mmol), 14 was obtained in 61% (0.177 g) yield. 1H NMR (400 MHz, CDCl3, 25 °C): δ = 8.72 (s, 1H), 8.84 (d, J = 4.9 Hz, 1H), 7.82 (d, J = 8.0 Hz, 1H), 7.42 (dd, J = 8.0, 4.9 Hz, 1H), 7.08 (d, J = 7.4 Hz, 2H), 3.56 (m, 2H), 3.32 (m, 2H), 1.35–1.15 (m, 6H). 13C NMR (100 MHz, CDCl3, 25 °C): δ = 168.0, 159.6 (dd, J = 252.4, 6.8 Hz), 150.3, 149.2, 139.4 (t, J = 8.7 Hz), 127.8, 125.0, 123.4, 115.7 (t, J = 19.7 Hz), 110.3 (d, J = 27.6 Hz), 43.3, 39.6, 14.2, 12.8. Elemental analysis: calcd (%) for C16H16F2N2O (290.31): C 66.20, H 5.56; found: C 66.00, H 5.68.
N,N-Diethyl-3,5-difluoro-4-(quinolin-3-yl)benzamide (15). From 3-bromoquinoline (0.208 g, 1 mmol) and N,N-diethyl-3,5-difluorobenzamide (0.320 g, 1.5 mmol), 15 was obtained in 87% (0.296 g) yield. 1H NMR (400 MHz, CDCl3, 25 °C): δ = 9.00 (s, 1H), 8.32 (s, 1H), 8.19 (d, J = 8.5 Hz, 1H), 7.89 (d, J = 8.1 Hz, 1H), 7.79 (t, J = 8.2 Hz, 1H), 7.61 (t, J = 7.4 Hz, 1H), 7.11 (d, J = 7.4 Hz, 2H), 3.57 (m, 2H), 3.35 (m, 2H), 1.35–1.15 (m, 6H). 13C NMR (100 MHz, CDCl3, 25 °C): δ = 168.0, 159.6 (dd, J = 252.4, 6.8 Hz), 150.7, 147.2, 139.4 (t, J = 8.7 Hz), 137.7, 130.4, 129.1, 128.1, 127.5, 127.2, 122.0, 115.9 (t, J = 19.7 Hz), 110.4 (d, J = 27.6 Hz), 43.3, 39.5, 14.2, 12.8. Elemental analysis: calcd (%) for C20H18F2N2O (340.37): C 70.58, H 5.33; found: C 70.29, H 5.47.
N,N-Diethyl-2,6-difluoro-4′-methylbiphenyl-4-carboxamide (16). From 4-bromotoluene (0.171 g, 1 mmol) and N,N-diethyl-3,5-difluorobenzamide (0.320 g, 1.5 mmol), 16 was obtained in 78% (0.236 g) yield. 1H NMR (400 MHz, CDCl3, 25 °C): δ = 7.36 (d, J = 7.8 Hz, 2H), 7.28 (d, J = 7.8 Hz, 2H), 7.01 (d, J = 6.3 Hz, 2H), 3.54 (m, 2H), 3.33 (m, 2H), 2.41 (s, 3H), 1.30–1.18 (m, 6H). 13C NMR (100 MHz, CDCl3, 25 °C): δ = 168.9, 159.9 (dd, J = 250.4, 7.5 Hz), 138.5, 137.8 (t, J = 9.0 Hz), 130.0, 129.1, 125.4, 119.3 (t, J = 18.7 Hz), 110.0 (d, J = 28.5 Hz), 43.3, 39.5, 21.3, 14.3, 12.8. Elemental analysis: calcd (%) for C18H19F2NO (303.35): C 71.27, H 6.31; found: C 71.30, H 6.50.
N,N-Diethyl-2,6-difluoro-4′-nitrobiphenyl-3-carboxamide (17). From 4-bromonitrobenzene (0.202 g, 1 mmol) and N,N-diethyl-2,4-difluorobenzamide (0.320 g, 1.5 mmol), 17 was obtained in 79% (0.264 g) yield. 1H NMR (400 MHz, CDCl3, 25 °C): δ = 8.33 (d, J = 8.1 Hz, 2H), 7.67 (d, J = 8.1 Hz, 2H), 7.40 (ddd, J = 8.5, 7.3, 6.1 Hz, 1H), 7.08 (dd, J = 9.0, 1.4 Hz, 1H), 3.58 (q, J = 7.6 Hz, 2H), 3.26 (q, J = 7.6 Hz, 2H), 1.25 (t, J = 7.6 Hz, 3H), 1.13 (t, J = 7.6 Hz, 3H). 13C NMR (100 MHz, CDCl3, 25 °C): δ = 164.8, 159.7 (dd, J = 254.3, 6.2 Hz), 155.2 (dd, J = 155.3, 8.1 Hz), 147.7, 135.2, 131.2, 129.1 (dd, J = 10.4, 6.2 Hz), 123.5, 122.4 (dd, J = 20.6, 4.2 Hz), 116.7 (t, J = 18.0 Hz), 112.5 (dd, J = 24.5, 3.1 Hz), 43.2, 39.4, 14.1, 12.8. Elemental analysis: calcd (%) for C17H16F2N2O3 (334.32): C 61.07, H 4.82; found: C 61.34, H 4.53.
4′-Cyano-N,N-diethyl-2,6-difluorobiphenyl-3-carboxamide (18). From 4-bromobenzonitrile (0.182 g, 1 mmol) and N,N-diethyl-2,4-difluorobenzamide (0.320 g, 1.5 mmol), 18 was obtained in 81% (0.254 g) yield. 1H NMR (400 MHz, CDCl3, 25 °C): δ = 7.77 (d, J = 8.1 Hz, 2H), 7.60 (d, J = 8.1 Hz, 2H), 7.37 (ddd, J = 8.5, 7.3, 6.1 Hz, 1H), 7.10 (dd, J = 9.0, 1.4 Hz, 1H), 3.58 (q, J = 7.6 Hz, 2H), 3.26 (q, J = 7.6 Hz, 2H), 1.25 (t, J = 7.6 Hz, 3H), 1.13 (t, J = 7.6 Hz, 3H). 13C NMR (100 MHz, CDCl3, 25 °C): δ = 164.8, 159.8 (dd, J = 246.3, 6.2 Hz), 155.1 (dd, J = 254.0, 6.6 Hz), 133.4, 132.6, 131.1, 128.9 (dd, J = 10.3, 6.2 Hz), 125.5, 122.3 (dd, J = 20.4, 4.0 Hz), 118.4, 117.0 (t, J = 18.0 Hz), 112.3 (t, J = 23.0 Hz), 43.1, 39.4, 14.0, 12.8. Elemental analysis: calcd (%) for C18H16F2N2O (314.33): C 68.78, H 5.13; found: C 68.75, H 5.03.
4′-Cyano-N,N-diethyl-2,5,6-trifluorobiphenyl-3-carboxamide (19). From 4-bromobenzonitrile (0.182 g, 1 mmol) N,N-diethyl-2,4,5-trifluorobenzamide (0.347 g, 1.5 mmol), 19 was obtained in 53% (0.176 g) yield. 1H NMR (400 MHz, CDCl3, 25 °C): δ = 7.77 (d, J = 8.1 Hz, 2H), 7.61 (d, J = 8.1 Hz, 2H), 7.25–7.17 (m, 1H), 3.58 (q, J = 7.6 Hz, 2H), 3.27 (q, J = 7.6 Hz, 2H), 1.24 (t, J = 7.6 Hz, 3H), 1.15 (t, J = 7.6 Hz, 3H). 13C NMR (100 MHz, CDCl3, 25 °C): δ = 163.6, 150.1 (d, J = 246.0 Hz), 147.5 (ddd, J = 253.0, 14.5, 6.8 Hz), 147.3 (ddd, J = 248.7, 13.8, 3.4 Hz), 132.4, 132.2, 130.9 (m), 121.8 (dm, J = 22.2 Hz), 118.8 (dd, J = 20.5, 14.9 Hz), 118.2, 115.8 (dd, J = 9.2, 4.4 Hz), 113.0, 43.2, 39.5, 14.0, 12.7. Elemental analysis: calcd (%) for C18H15F3N2O (332.32): C 65.06, H 4.55; found: C 62.17, H 4.58.
4′-(Cyanomethyl)-N,N-diethyl-2,5,6-trifluorobiphenyl-3-carboxamide (20). From 2-(4-bromophenyl)acetonitrile (0.196 g, 1 mmol) N,N-diethyl-2,4,5-trifluorobenzamide (0.347 g, 1.5 mmol), 20 was obtained in 61% (0.211 g) yield. 1H NMR (400 MHz, CDCl3, 25 °C): δ = 7.50–7.46 (m, 4H), 7.20–7.15 (m, 1H), 3.84 (s, 2H), 3.59 (q, J = 7.6 Hz, 2H), 3.27 (q, J = 7.6 Hz, 2H), 1.24 (t, J = 7.6 Hz, 3H), 1.15 (t, J = 7.6 Hz, 3H). 13C NMR (100 MHz, CDCl3, 25 °C): δ = 13C NMR (100 MHz, CDCl3, 25 °C): δ = 164.0, 150.5 (d, J = 246.0 Hz), 148.0 (ddd, J = 253.0, 14.5, 6.8 Hz), 147.5 (ddd, J = 248.7, 13.7, 3.6 Hz), 130.9, 128.2, 127.7, 121.5 (dm, J = 18.0 Hz), 119.8 (dd, J = 21.0, 15.3 Hz), 117.4, 114.9 (dd, J = 20.7, 5.6 Hz), 43.2, 39.5, 23.5, 14.0, 12.7. Elemental analysis: calcd (%) for C19H17F3N2O (346.34): C 65.89, H 4.95; found: C 65.77, H 4.98.
N,N-Diethyl-2,4,5-trifluoro-3-(quinolin-3-yl)benzamide (21). From 3-bromoquinoline (0.208 g, 1 mmol) and N,N-diethyl-2,4,5-trifluorobenzamide (0.347 g, 1.5 mmol), 21 was obtained in 81% (0.290 g) yield. 1H NMR (400 MHz, CDCl3, 25 °C): δ = 9.01 (s, 1H), 8.33 (s, 1H), 8.19 (d, J = 8.1 Hz, 1H), 7.91 (d, J = 8.1 Hz, 1H), 7.82 (t, J = 7.8 Hz, 1H), 7.65 (t, J = 7.8 Hz, 1H), 7.26 (m, 1H), 3.61 (q, J = 7.6 Hz, 2H), 3.31 (q, J = 7.6 Hz, 2H), 1.28 (t, J = 7.6 Hz, 3H), 1.17 (t, J = 7.6 Hz, 3H). 13C NMR (100 MHz, CDCl3, 25 °C): δ = 163.7, 150.8 (dd, J = 246.3, 6.2 Hz), 150.5, 148.3 (dd, J = 246.3, 6.2 Hz), 147.7, 147.6 (dd, J = 246.3, 13.5 Hz), 137.7, 130.6, 129.4, 128.2, 127.4, 127.3, 121.8 (dt, J = 22.7, 5.1 Hz), 121.3, 117.5 (dd, J = 21.5, 15.2 Hz), 115.7 (dd, J = 19.3, 4.5 Hz), 43.2, 39.5, 14.0, 12.7. Elemental analysis: calcd (%) for C20H17F3N2O (358.36): C 67.03, H 4.78; found: C 67.24, H 5.00.
4′-Acetyl-N,N-diethyl-2,3-difluorobiphenyl-4-carboxamide (22). From 4-bromoacetophenone (0.199 g, 1 mmol) and N,N-diethyl-2,3-difluorobenzamide (0.320 g, 1.5 mmol), 22 was obtained in 61% (0.202 g) yield. 1H NMR (400 MHz, CDCl3, 25 °C): δ = 8.07 (d, J = 8.4 Hz, 2H), 7.66 (d, J = 8.4 Hz, 2H), 7.35–7.20 (m, 2H), 3.61 (q, J = 7.6 Hz, 2H), 3.30 (q, J = 7.6 Hz, 2H), 2.67 (s, 3H), 1.30 (t, J = 7.6 Hz, 3H), 1.17 (t, J = 7.6 Hz, 3H). 13C NMR (100 MHz, CDCl3, 25 °C): δ = 197.5, 164.1 (d, J = 2.5 Hz), 147.8 (dd, J = 253.8, 13.7 Hz), 147.0 (dd, J = 250.5, 14.5 Hz), 138.7, 136.8, 130.5 (d, J = 10.2 Hz), 129.1 (d, J = 3.1 Hz), 128.6, 127.0 (d, J = 15.7 Hz), 125.4, 122.8, 43.1, 39.4, 26.7, 14.1, 12.9. Elemental analysis: calcd (%) for C19H19F2NO2 (331.36): C 68.87, H 5.78; found: C 69.04, H 5.79.
N,N-Diethyl-2,3-difluoro-4′-nitrobiphenyl-4-carboxamide (23). From 4-bromonitrobenzene (0.202 g, 1 mmol) and N,N-diethyl-2,3-difluorobenzamide (0.320 g, 1.5 mmol), 23 was obtained in 64% (0.214 g) yield. 1H NMR (400 MHz, CDCl3, 25 °C): δ = 8.35 (d, J = 7.8 Hz, 2H), 7.74 (d, J = 7.8 Hz, 2H), 7.35–7.20 (m, 2H), 3.62 (q, J = 7.6 Hz, 2H), 3.31 (q, J = 7.6 Hz, 2H), 1.30 (t, J = 7.6 Hz, 3H), 1.17 (t, J = 7.6 Hz, 3H). 13C NMR (100 MHz, CDCl3, 25 °C): δ = 164.1 (d, J = 2.5 Hz), 147.7, 147.6 (dd, J = 253.8, 13.8 Hz), 147.0 (dd, J = 250.5, 14.3 Hz), 140.5, 129.8, 129.4 (d, J = 10.1 Hz), 127.7 (d, J = 15.8 Hz), 125.5, 124.0, 123.1, 43.2, 39.4, 14.1, 12.8. Elemental analysis: calcd (%) for C17H16F2N2O3 (334.32): C 61.07, H 4.82; found: C 61.18, H 4.99.
4′-Cyano-N,N-diethyl-5,6-difluorobiphenyl-3-carboxamide (24). From 4-bromobenzonitrile (0.182 g, 1 mmol) and N,N-diethyl-3,4-difluorobenzamide (0.320 g, 1.5 mmol), 24 was obtained in 36% (0.113 g) yield. 1H NMR (400 MHz, CDCl3, 25 °C): δ = 7.78 (d, J = 8.3 Hz, 2H), 7.68 (d, J = 8.3 Hz, 2H), 7.33–7.22 (m, 2H), 3.55 (bs, 2H), 3.35 (bs, 2H), 1.30–1.10 (m, 6H). 13C NMR (100 MHz, CDCl3, 25 °C): δ = 168.4, 150.7 (dd, J = 248.0, 12.0), 148.1 (dd, J = 250.0, 11.9), 138.4, 134.0 (m), 132.5, 129.6, 123.4, 118.4, 115.8 (d, J = 18.8 Hz), 112.5, 43.4, 39.6, 14.0, 12.8. Elemental analysis: calcd (%) for C18H16F2N2O (314.33): C 68.78, H 5.13; found: C 69.02, H 4.94.
N-n-Butyl-4′-cyano-2,6-difluorobiphenyl-4-carboxamide (25a). From 4-bromobenzonitrile (0.182 g, 1 mmol) and N-butyl-3,5-difluorobenzamide (0.320 g, 1.5 mmol), 25a was obtained in 61% (0.191 g) yield. 1H NMR (400 MHz, CDCl3, 25 °C): δ = 7.79 (d, J = 8.1 Hz, 2H), 7.62 (d, J = 8.1 Hz, 2H), 7.45 (d, J = 7.8 Hz, 2H), 6.10 (s, 1H), 3.50 (q, J = 7.6 Hz, 2H), 1.65 (quint., J = 7.6 Hz, 2H), 1.45 (sext., J = 7.6 Hz, 2H), 1.00 (t, J = 7.6 Hz, 3H). 13C NMR (100 MHz, CDCl3, 25 °C): δ = 164.5, 159.8 (dd, J = 252.0, 6.8 Hz), 137.2 (t, J = 8.6 Hz), 133.1, 132.1, 131.0 (t, J = 2.3 Hz), 119.3, 118.4, 112.6, 110.7 (d, J = 27.4 Hz), 40.1, 31.6, 20.1, 13.7. Elemental analysis: calcd (%) for C18H16F2N2O (314.33): C 68.78, H 5.13; found: C 68.89, H 5.24. 25b was also isolated in low yield: 1H NMR (400 MHz, CDCl3, 25 °C): δ = 7.75 (d, J = 8.1 Hz, 2H), 7.53 (d, J = 8.1 Hz, 2H), 7.21 (d, J = 7.8 Hz, 1H), 7.03 (t, J = 8.0 Hz, 1H), 5.31 (s, 1H), 3.19 (q, J = 7.6 Hz, 2H), 1.26 (quint., J = 7.6 Hz, 2H), 1.15 (sext., J = 7.6 Hz, 2H), 0.85 (t, J = 7.6 Hz, 3H).
N-n-Butyl-2,6-difluoro-4′-formylbiphenyl-4-carboxamide (26a). From 4-bromobenzaldehyde (0.185 g, 1 mmol) and N-butyl-3,5-difluorobenzamide (0.320 g, 1.5 mmol), 26a was obtained in 68% (0.215 g) yield. 1H NMR (400 MHz, CDCl3, 25 °C): δ = 10.10 (s, 1H), 8.00 (d, J = 8.3 Hz, 2H), 7.66 (d, J = 8.3 Hz, 2H), 7.46 (d, J = 7.8 Hz, 2H), 6.29 (s, 1H), 3.50 (q, J = 7.6 Hz, 2H), 1.65 (quint., J = 7.6 Hz, 2H), 1.45 (sext., J = 7.6 Hz, 2H), 1.01 (t, J = 7.6 Hz, 3H). 13C NMR (100 MHz, CDCl3, 25 °C): δ = 191.7, 164.6, 159.8 (dd, J = 251.7, 6.9 Hz), 137.0 (t, J = 8.6 Hz), 136.2, 134.6, 131.0, 129.6, 120.0 (t, J = 18.3 Hz), 110.6 (d, J = 27.7 Hz), 40.1, 31.6, 20.2, 13.8. Elemental analysis: calcd (%) for C18H17F2NO2 (317.33): C 68.13, H 5.40; found: C 68.41, H 5.24.
N-n-Butyl-2,6-difluoro-4′-nitrobiphenyl-4-carboxamide (27a). From 4-bromonitrobenzene (0.202 g, 1 mmol) and N-butyl-3,5-difluorobenzamide (0.320 g, 1.5 mmol), 27a was obtained in 70% (0.234 g) yield. 1H NMR (400 MHz, CDCl3, 25 °C): δ = 8.35 (d, J = 8.3 Hz, 2H), 7.68 (d, J = 8.3 Hz, 2H), 7.47 (d, J = 7.8 Hz, 2H), 6.20 (s, 1H), 3.50 (q, J = 7.6 Hz, 2H), 1.65 (quint., J = 7.6 Hz, 2H), 1.45 (sext., J = 7.6 Hz, 2H), 1.01 (t, J = 7.6 Hz, 3H). 13C NMR (100 MHz, CDCl3, 25 °C): δ = 164.1, 159.8 (dd, J = 252.3, 6.7 Hz), 147.5, 137.1 (t, J = 8.2 Hz), 134.7, 130.9 (t, J = 2.3 Hz), 123.2, 118.7 (t, J = 18.3 Hz), 110.4 (d, J = 27.5 Hz), 39.8, 31.3, 19.8, 13.4. Elemental analysis: calcd (%) for C17H16F2N2O3 (334.32): C 61.07, H 4.82; found: C 61.30, H 4.64.
N-n-Butyl-2′-cyano-2,6-difluorobiphenyl-4-carboxamide (28a). From 2-bromobenzonitrile (0.182 g, 1 mmol) and N-butyl-3,5-difluorobenzamide (0.320 g, 1.5 mmol), 28a was obtained in 76% (0.239 g) yield. 1H NMR (400 MHz, CDCl3, 25 °C): δ = 7.84 (d, J = 7.8 Hz, 1H), 7.74 (t, J = 7.8 Hz, 1H), 7.59 (t, J = 7.8 Hz, 1H), 7.51 (d, J = 7.8 Hz, 1H), 7.48 (d, J = 7.2 Hz, 2H), 6.21 (s, 1H), 3.50 (q, J = 7.6 Hz, 2H), 1.65 (quint., J = 7.6 Hz, 2H), 1.45 (sext., J = 7.6 Hz, 2H), 1.01 (t, J = 7.6 Hz, 3H). 13C NMR (100 MHz, CDCl3, 25 °C): δ = 164.8, 159.7 (dd, J = 252.0, 6.6 Hz), 138.2 (t, J = 8.5 Hz), 133.1, 132.7, 132.4, 131.5, 129.3, 117.8 (t, J = 19.7 Hz), 117.3, 113.9, 110.6 (d, J = 27.7 Hz), 40.1, 31.6, 20.1, 13.7. Elemental analysis: calcd (%) for C18H16F2N2O (314.33): C 68.78, H 5.13; found: C 68.70, H 5.00.
N-tert-Butyl-4′-cyano-2,6-difluorobiphenyl-4-carboxamide (29a). From 4-bromobenzonitrile (0.182 g, 1 mmol) and N-tert-3,5-difluorobenzamide (0.320 g, 1.5 mmol), 29a was obtained in 66% (0.207 g) yield. 1H NMR (400 MHz, CDCl3, 25 °C): δ = 7.77 (d, J = 8.1 Hz, 2H), 7.60 (d, J = 8.1 Hz, 2H), 7.40 (d, J = 7.8 Hz, 2H), 6.00 (s, 1H), 1.48 (s, 9H). 13C NMR (100 MHz, CDCl3, 25 °C): δ = 163.9, 159.7 (dd, J = 251.8, 7.7 Hz), 138.4 (t, J = 8.4 Hz), 133.3, 132.0, 131.0 (m), 119.0 (t, J = 18.2 Hz), 118.4, 112.5, 110.7 (d, J = 27.4 Hz), 52.2, 28.7. Elemental analysis: calcd (%) for C18H16F2N2O (314.33): C 68.78, H 5.13; found: C 68.64, H 5.02.
N-tert-Butyl-2,6-difluoro-4′-formylbiphenyl-4-carboxamide (30a). From 4-bromobenzaldehyde (0.185 g, 1 mmol) and N-tert-3,5-difluorobenzamide (0.320 g, 1.5 mmol), 30a was obtained in 70% (0.222 g) yield. 1H NMR (400 MHz, CDCl3, 25 °C): δ = 10.10 (s, 1H), 8.02 (d, J = 8.1 Hz, 2H), 7.66 (d, J = 8.1 Hz, 2H), 7.40 (d, J = 8.0 Hz, 2H), 5.97 (s, 1H), 1.51 (s, 9H). 13C NMR (100 MHz, CDCl3, 25 °C): δ = 191.7, 163.9, 158.7 (dd, J = 251.7, 6.9 Hz), 138.1 (t, J = 8.5 Hz), 136.2, 134.6, 131.0, 129.6, 119.7 (t, J = 18.3 Hz), 110.5 (d, J = 27.7 Hz), 52.2, 28.8. Elemental analysis: calcd (%) for C18H17F2NO2 (317.33): C 68.13, H 5.40; found: C 68.02, H 5.54.
N-tert-Butyl-2,6-difluoro-4′-nitrobiphenyl-4-carboxamide (31a). From 4-bromonitrobenzene (0.202 g, 1 mmol) and N-tert-3,5-difluorobenzamide (0.320 g, 1.5 mmol), 31a was obtained in 66% (0.220 g) yield. 1H NMR (400 MHz, CDCl3, 25 °C): δ = 8.34 (d, J = 8.1 Hz, 2H), 7.67 (d, J = 8.1 Hz, 2H), 7.42 (d, J = 8.0 Hz, 2H), 5.99 (s, 1H), 1.50 (s, 9H). 13C NMR (100 MHz, CDCl3, 25 °C): δ = 163.8 (t, J = 2.4 Hz), 159.7 (dd, J = 252.2, 6.7 Hz), 147.8, 138.6 (t, J = 8.5 Hz), 135.1, 131.3 (t, J = 2.2 Hz), 123.6, 118.7 (t, J = 18.3 Hz), 110.7 (d, J = 27.5 Hz), 52.3, 28.8. Elemental analysis: calcd (%) for C17H16F2N2O3 (334.32): C 61.07, H 4.82; found: C 61.02, H 4.99.
N-tert-Butyl-2′-cyano-2,6-difluorobiphenyl-4-carboxamide (32a). From 2-bromobenzonitrile (0.182 g, 1 mmol) and N-tert-3,5-difluorobenzamide (0.320 g, 1.5 mmol), 32a was obtained in 63% (0.198 g) yield. 1H NMR (400 MHz, CDCl3, 25 °C): δ = 7.82 (d, J = 8.0 Hz, 1H), 7.74 (t, J = 7.8 Hz, 1H), 7.57 (t, J = 7.8 Hz, 1H), 7.50 (d, J = 8.0 Hz, 1H), 7.40 (d, J = 7.8 Hz, 2H), 6.06 (s, 1H), 1.48 (s, 9H). 13C NMR (100 MHz, CDCl3, 25 °C): δ = 164.0, 159.7 (dd, J = 251.7, 6.9 Hz), 139.4 (t, J = 8.2 Hz), 133.1, 132.7, 132.4, 131.5, 129.3, 117.5 (t, J = 19.9 Hz), 117.4, 113.8, 110.5 (d, J = 27.3 Hz), 52.2, 28.7. Elemental analysis: calcd (%) for C18H16F2N2O (314.33): C 68.78, H 5.13; found: C 68.87, H 5.24.
N-tert-Butyl-3,5-difluoro-4-(quinolin-3-yl)benzamide (33a). From 3-bromoquinoline (0.208 g, 1 mmol) and N-tert-3,5-difluorobenzamide (0.320 g, 1.5 mmol), 33a was obtained in 64% (0.218 g) yield. 1H NMR (400 MHz, CDCl3, 25 °C): δ = 9.01 (s, 1H), 8.32 (s, 1H), 8.18 (d, J = 8.0 Hz, 1H), 7.90 (d, J = 8.0 Hz, 1H), 7.80 (t, J = 7.8 Hz, 1H), 7.62 (t, J = 7.8 Hz, 1H), 7.45 (d, J = 7.8 Hz, 2H), 6.03 (s, 1H), 1.51 (s, 9H). 13C NMR (100 MHz, CDCl3, 25 °C): δ = 163.9, 160.1 (dd, J = 251.7, 7.0 Hz), 150.9, 147.5, 138.2 (t, J = 8.2 Hz), 137.6, 130.5, 129.3, 128.2, 127.5, 127.2, 122.0, 117.7 (t, J = 19.9 Hz), 110.6 (d, J = 27.3 Hz), 52.2, 28.8. Elemental analysis: calcd (%) for C20H18F2N2O (340.37): C 70.58, H 5.33; found: C 70.40, H 5.21.
Acknowledgements
We thank the Centre National de la Recherche Scientifique and “Rennes Metropole” for providing financial support.
Notes and references
-
(a) J. J. Li and G. W. Gribble, Palladium in Heterocyclic Chemistry, Pergamon, Amsterdam, 2000 Search PubMed;
(b) Handbook of Organopalladium Chemistry for Organic Synthesis, ed. E. Negishi, Wiley-Interscience, New York, 2002, Part III, p. 213 Search PubMed;
(c) L. Ackermann, Modern arylation methods, Wiley, 2009 CrossRef.
- For reviews on metal-catalysed direct arylation:
(a) T. Satoh and M. Miura, Chem. Lett., 2007, 36, 200–205 CrossRef CAS;
(b) B.-J. Li, S.-D. Yang and Z.-J. Shi, Synlett, 2008, 949–957 CAS;
(c) F. Bellina and R. Rossi, Tetrahedron, 2009, 65, 10269–10310 CrossRef CAS;
(d) L. Ackermann, R. Vicente and A. Kapdi, Angew. Chem., Int. Ed., 2009, 48, 9792–9826 CrossRef CAS PubMed;
(e) X. Chen, K. M. Engle, D.-H. Wang and J.-Q. Yu, Angew. Chem., Int. Ed., 2009, 48, 5094–5115 CrossRef CAS PubMed;
(f) J. Roger, A. L. Gottumukkala and H. Doucet, ChemCatChem, 2010, 2, 20–40 CrossRef CAS;
(g) T. Satoh and M. Miura, Synthesis, 2010, 3395–3409 CrossRef CAS;
(h) C.-L. Sun, B.-J. Li and Z.-J. Shi, Chem. Commun., 2010, 46, 677–685 RSC;
(i) N. Kuhl, M. N. Hopkinson, J. Wencel-Delord and F. Glorius, Angew. Chem., Int. Ed., 2012, 51, 10236–10254 CrossRef CAS PubMed;
(j) B.-J. Li and Z.-J. Shi, Chem. Soc. Rev., 2012, 41, 5588–5598 RSC;
(k) M. C. White, Synlett, 2012, 23, 2746–2748 CAS;
(l) S. I. Kozhushkov and L. Ackermann, Chem. Sci., 2013, 4, 886–896 RSC;
(m) J. Wencel-Delord and F. Glorius, Nat. Chem., 2013, 5, 369–375 CrossRef CAS PubMed;
(n) M. He, J.-F. Soulé and H. Doucet, ChemCatChem, 2014, 6, 1824–1859 CrossRef CAS;
(o) R. Rossi, F. Bellina, M. Lessi and C. Manzini, Adv. Synth. Catal., 2014, 356, 17–117 CrossRef CAS;
(p) M. Zhang, Y. Zhang, X. Jie, H. Zhao, G. Li and W. Su, Org. Chem. Front., 2014, 1, 843–895 RSC;
(q) K. Yuan, J.-F. Soulé and H. Doucet, ACS Catal., 2015, 5, 978–991 CrossRef CAS;
(r) C. B. Bheeter, L. Chen, J.-F. Soulé and H. Doucet, Catal. Sci. Technol., 2016, 6, 2005–2049 RSC.
- M. Lafrance, C. N. Rowley, T. K. Woo and K. Fagnou, J. Am. Chem. Soc., 2006, 128, 8754–8756 CrossRef CAS PubMed.
- For intermolecular palladium-catalysed direct arylations of (poly)fluorobenzenes:
(a) M. Lafrance, D. Shore and K. Fagnou, Org. Lett., 2006, 8, 5097–5100 CrossRef CAS PubMed;
(b) Y. Wei, J. Kan, M. Wang, W. Su and M. Hong, Org. Lett., 2009, 11, 3346–3349 CrossRef CAS PubMed;
(c) O. Rene and K. Fagnou, Org. Lett., 2010, 12, 2116–2119 CrossRef CAS PubMed;
(d) Y. Wei and W. Su, J. Am. Chem. Soc., 2010, 132, 16377–16379 CrossRef CAS PubMed;
(e) D. Lapointe, T. Markiewicz, C. J. Whipp, A. Toderian and K. Fagnou, J. Org. Chem., 2011, 76, 749–759 CrossRef CAS PubMed;
(f) H. Li, J. Liu, C.-L. Sun, B.-J. Li and Z.-J. Shi, Org. Lett., 2011, 13, 276–279 CrossRef CAS PubMed;
(g) H. Zhao, Y. Wei, J. Xu, J. Kan, W. Su and M. Hong, J. Org. Chem., 2011, 76, 882–893 CrossRef CAS PubMed;
(h) L. Ackermann and S. Fenner, Chem. Commun., 2011, 47, 430–432 RSC;
(i) M. Yu, Z. Liang, Y. Wang and Y. Zhang, J. Org. Chem., 2011, 76, 4987–4994 CrossRef CAS PubMed;
(j) B. Liu, Z. Wang, N. Wu, M. Li, J. You and J. Lan, Chem.–Eur. J., 2012, 18, 1599 CrossRef CAS PubMed;
(k) J. C. Bernhammer and H. V. Huynh, Organometallics, 2012, 31, 5121 CrossRef CAS;
(l) F. Chen, Q.-Q. Min and X. Zhang, J. Org. Chem., 2012, 77, 2992 CrossRef CAS PubMed;
(m) J. W. W. Chang, E. Y. Chia, C. L. L. Chai and J. Seayad, Org. Biomol. Chem., 2012, 10, 2289–2299 RSC;
(n) T. Yan, L. Chen, C. Bruneau, P. H. Dixneuf and H. Doucet, J. Org. Chem., 2013, 78, 4177–4183 CrossRef CAS PubMed;
(o) T. Yan, L. Zhao, M. He, J.-F. Soulé, C. Bruneau and H. Doucet, Adv. Synth. Catal., 2014, 356, 1586–1596 CrossRef CAS;
(p) D. Yuan and H. V. Huynh, Organometallics, 2014, 33, 6033–6043 CrossRef CAS;
(q) H.-H. Zhang, J. Dong and Q.-S. Hu, Eur. J. Org. Chem., 2014, 1327–1332 CrossRef PubMed;
(r) T. Miao and L. Wang, Adv. Synth. Catal., 2014, 356, 429–436 CrossRef CAS;
(s) O. Y. Yuen, M. Charoensak, C. M. So, C. Kuhakarn and F. Y. Kwong, Chem.–Asian J., 2015, 10, 857–861 CrossRef CAS PubMed.
- Y. Kametani, T. Satoh, M. Miura and M. Nomura, Tetrahedron Lett., 2000, 41, 2655–2658 CrossRef CAS.
- For intermolecular palladium-catalysed direct arylations of benzamides:
(a) D. Shabashov and O. Daugulis, Org. Lett., 2006, 8, 4947–4949 CrossRef CAS PubMed;
(b) J. Kim, M. Jo, W. So and Z. No, Tetrahedron Lett., 2009, 50, 1229–1235 CrossRef CAS;
(c) J. Kim, M. Jo, W. So and Z. No, Tetrahedron Lett., 2009, 50, 1229–1235 CrossRef CAS;
(d) B. V. S. Reddy, G. Revathi, A. S. Reddy and J. S. Yadav, Synlett, 2011, 2374–2378 CrossRef CAS;
(e) J. Karthikeyan and C.-H. Cheng, Angew. Chem., Int. Ed., 2011, 50, 9880–9883 CrossRef CAS PubMed;
(f) X. Wang, D. Leow and J.-Q. Yu, J. Am. Chem. Soc., 2011, 133, 13864–13867 CrossRef CAS PubMed;
(g) F. Péron, C. Fossey, T. Cailly and F. Fabis, Org. Lett., 2012, 14, 1827–1829 CrossRef PubMed;
(h) D.-D. Li, T.-T. Yuan and G.-W. Wang, J. Org. Chem., 2012, 77, 3341–3347 CrossRef CAS PubMed;
(i) C. Arroniz, J. G. Denis, A. Ironmonger, G. Rassias and I. Larrosa, Chem. Sci., 2014, 5, 3509–3514 RSC;
(j) Z. Yang, F.-C. Qiu, J. Gao, Z.-W. Li and B.-T. Guan, Org. Lett., 2015, 17, 4316–4319 CrossRef CAS PubMed.
- A. Yokota, Y. Aihara and N. Chatani, J. Org. Chem., 2014, 79, 11922–11932 CrossRef CAS PubMed.
-
(a) L. C. M. Castro and N. Chatani, Chem.–Eur. J., 2014, 20, 4548–4553 CrossRef PubMed;
(b) X. Zhou, Q. Wang, W. Zhao, S. Xu, W. Zhang and J. Chen, Tetrahedron Lett., 2015, 56, 851–855 CrossRef CAS.
- Y. Wang, K. Zhou, Q. Lan and X.-S. Wang, Org. Biomol. Chem., 2015, 13, 353–356 Search PubMed.
- J. Li and L. Ackermann, Chem.–Eur. J., 2015, 21, 5718–5722 CrossRef CAS PubMed.
-
(a) D. Garcia-Cuadrado, P. de Mendoza, A. A. C. Braga, F. Maseras and A. M. Echavarren, J. Am. Chem. Soc., 2007, 129, 6880–6886 CrossRef CAS PubMed;
(b) S. I. Gorelsky, D. Lapointe and K. Fagnou, J. Org. Chem., 2012, 77, 658–668 CrossRef CAS PubMed;
(c) S. I. Gorelsky, Coord. Chem. Rev., 2013, 257, 153–164 CrossRef CAS.
-
(a) T. Okazawa, T. Satoh, M. Miura and M. Nomura, J. Am. Chem. Soc., 2002, 124, 5286–5287 CrossRef CAS PubMed;
(b) A. Yokooji, T. Okazawa, T. Satoh, M. Miura and M. Nomura, Tetrahedron, 2003, 59, 5685–5689 CrossRef CAS.
- T. Cantat, E. Génin, C. Giroud, G. Meyer and A. Jutand, J. Organomet. Chem., 2003, 687, 365–376 CrossRef CAS.
- C. Hansch, A. Leo and R. W. Taft, Chem. Rev., 1991, 91, 165–195 CrossRef CAS.
Footnote |
† Electronic supplementary information (ESI) available. See DOI: 10.1039/c6ra12165a |
|
This journal is © The Royal Society of Chemistry 2016 |
Click here to see how this site uses Cookies. View our privacy policy here.