DOI:
10.1039/C6RA12161F
(Review Article)
RSC Adv., 2016,
6, 69282-69300
Strategies for formulation development of andrographolide
Received
10th May 2016
, Accepted 28th June 2016
First published on 6th July 2016
Abstract
Andrographolide (AN), a diterpenoid lactone, is one of the prime phytoconstituents of Andrographis paniculata. AN possesses various therapeutic benefits such as hepatoprotective, analgesic, anti-inflammatory, anti-tumor, anti-hyperglycemic, anti-oxidant activities. However, AN has not reached its milestone therapeutic potential as clinical studies have suggested that a conventional dosage form of AN showed low oral bioavailability (2.67%). The factors attributed to low bioavailability included specific site absorption, hydrolysis in weak alkaline environments, first pass metabolism, P-gp excretion in the terminal intestine, biliary excretion and poor aqueous solubility (3.29 μg mL−1). A successful AN therapy warrants an apt delivery system that will enhance its bioavailability. Current approaches for developing an improved delivery system for AN are mainly focused on overcoming solubility and toxicity issues. The path to identify new molecules with better therapeutic efficacy will continue to be an integral part of health care systems but here the authors have emphasized on ‘better delivery of drugs’, which is going to further refine therapy. The different formulation approaches investigated so far have shown much promise experimentally in improving the bioavailability of AN. However, a product for human use is still far away. Since its isolation and characterisation in 1911 several developments related to AN have occurred. This review is the first comprehensive account of the pharmaceutical aspects of AN with special emphasis on its delivery that have occurred over the last century.
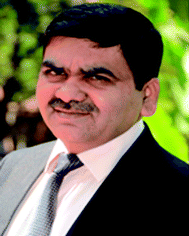 Atmaram Pawar | Atmaram Pawar is currently employed as a Professor of Pharmaceutics and Vice-Principal for Department of Pharm D at Bharati Vidyapeeth University, Pune, Maharashtra, India. He acquired graduate, post graduate and PhD degrees from Bharati Vidyapeeth University, Pune, Maharashtra, India. With an academic teaching experience of 25 years, he is also the author of approximately 75 peer-reviewed publications, twelve textbooks and three Indian patents. He is a referee for more than 25 pharmaceutical international journals, including Langmuir, JCR, DDIP, IJP, AAPS, J. Microcap., J. Pharm. And Pharmacology, Journal of Macromolecular Science, Chem. Engg. and Communication. |
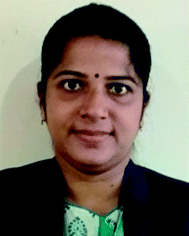 S. Rajalakshmi | S. Rajalakshmi is currently employed as an Assistant Professor of Pharmaceutics at Padmashree Dr D. Y. Patil College of Pharmacy, Akurdi, Pune-411044. Maharashtra, India. She pursued her post graduate course from Bharati Vidyapeeth University, Maharashtra, India. She has published articles related to her expertise in several peer reviewed journals of international repute. She is actively involved in crystal modification such as co-crystals for some poorly water soluble drugs to improve their biopharmaceuticals properties and development of some novel drug delivery systems for phytomedicines. |
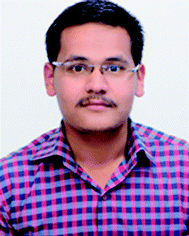 Piyush Mehta | Piyush Mehta is currently employed as a Research Associate in Respiratory Formulations at the Cipla R & D, Mumbai, Maharashtra, India. He has pursued graduate and post graduate courses from the Bharati Vidyapeeth University, Pune, Maharashtra, India. He has published more than 10 articles related to his proficiency in several peer reviewed journals of international repute. He is actively involved in the development of dry powder inhalers, phytopharmaceuticals and various complementary alternative therapies. |
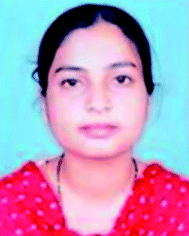 Karimunnisa Shaikh | Karimunnisa Shaikh is currently employed as an Associate Professor of Pharmaceutics at Modern College of Pharmacy, University of Pune, Maharashtra, India. She acquired a PhD degree from Bharati Vidyapeeth University, Maharashtra, India. She was a recipient of National Doctoral Fellowship from AICTE, India. She pursued her graduate and post graduate courses from University of Pune, India. She has been contributing to academia for 11 years. She has published articles related to her expertise in several peer reviewed journals of international repute. She is actively involved in development of novel drug delivery systems, specifically nanoparticles, liposomes, niosomes, ethosomes and phytopharmaceuticals. |
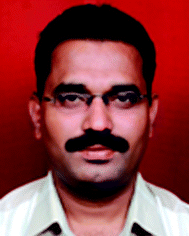 Chellampillai Bothiraja | C. Bothiraja is an Associate Professor of Pharmaceutics at Bharati Vidyapeeth University, Maharashtra, India. He acquired a PhD from Bharati Vidyapeeth University, Maharashtra, India. He has a rich experience of 15 years academic teaching. He has published 38 papers in international peer reviewed journals. He is a referee for more than fifteen pharmaceutical international journals, including RSC Advances, Exp. Opi. Drug. Deliv., Colloids Surface B Interface., Food and Chemical Toxicology., J. Microencap., Pharm. Dev. Technol., and Int. J. Nanomedi. He is actively involved in the development of novel drug delivery systems, specifically targeted nanoparticles, nanocomposites, microsponges, liposomes, nanomicelles, nanocochleates and phytopharmaceuticals. |
Introduction
Herbs have been used for centuries to improve the health and well-being of mankind. Mainstream modern medicine has begun to re-focus on herbs and natural plant therapies as a primary means to deal with health issues, also said as ‘Return to Nature’. Herbal medicines are being used by about 80% of the world population. Mainly in the developing countries, wherein access to hospital and health care is limited and herbal medicines often are the only general form of medicine available. Moreover, herbal medicines are non-toxic with lesser side effects, possess wide biological activities, cultural acceptability and easy availability at affordable prices. Moreover, the chemical constituents are a part of the physiological functions of living flora and therefore they are believed to have better compatibility with the human body as compared to synthetic drugs.1,2 According to the World Health Organization (WHO), the use of herbal remedies throughout the world exceeds conventional drugs by a factor two to three times.3
Herbalists generally use unpurified plant extracts containing several different constituents. Often several different herbs are used together where practitioner's claim the principles of synergy and buffering apply to combinations of plants, combining herbs improves efficacy and reduces adverse effect. This is in contrast with conventional practice, wherein poly-pharmacy is generally avoided whenever possible.4 The pharmacological action of the desired “active” component in herbal medicaments in the form of crude extract and galenicals preparation may be modified due to other phytoconstituents that are present. Moreover, some secondary metabolites that are present in the plant may produce undesirable side effects. The use of major “active” phytoconstituents as a phytomedicine can be strengthened by proper identification, isolation, elucidation of the biopharmaceutical properties and pharmacological action. The isolation of the active principles from the plants and herbs began in the early 1800 s using various techniques such as silica gel column chromatography, preparative high performance thin layer chromatography (HPTLC), supercritical carbon dioxide extraction, high-speed counter-current chromatography and cold maceration.5
The use of phytoconstituents is marred due to problems related to its physical nature, solubility, lipophilicity and stability, which are main causes of low bioavailability. Techniques, such as co-precipitation, pro-drugs, salt formation, complexation and particle size reduction, are used to improve the bioavailability of phytoconstituents.6 For example, the oral bioavailability of reserpine, an anti-hypertensive phytoconstituent, was increased 2 to 3 fold when it was co-precipitated with polyvinyl pyrrolidine (PVP).7 Solubility, bioavailability, chemical and photo stability of curcumin were improved by complexation with gelatin,8 polysaccharides, protein,9 cyclodextrin10 and solid dispersion with PVP.11
Novel drug delivery systems for phytoconstituents
As mentioned in the preceding section, poor solubility, chemical complexity, rapid metabolism and poor bioavailability have limited the success of phytoconstituents in clinical settings. The quest to maximise the therapeutic potential of phytoconstituents has led drug delivery researchers to focus on novel drug delivery systems (NDDS) for phytomedicines. The NDDS are expected to fulfill two prime requisites. First, they should deliver the drug at a rate directed by the needs of the body over the period of treatment. Second, they should channel the active entity to the site of action. Conventional dosage forms are unable to meet these demands. Developing various novel dosage forms, such as polymeric nanoparticles, nanocapsules, liposomes, solid lipid nanoparticles, phytosomes and nano-emulsions, have offered a number of advantages for phytomedicines, including enhancement of solubility, bioavailability, pharmacological activity, stability, tissue macrophage distribution, sustained delivery and lowering of the toxicity.12 Several potential phytoconstituents have been successfully delivered using NDDS.
Andrographolide
Andrographis paniculata Wall. ex Nees (Acanthaceae) grows widely in various Asian countries and has a very strong history of therapeutic usage in culture specific Indian and Oriental medicine.13–15 Due to its extremely bitter properties, it is commonly known as “king of bitters”. In traditional Chinese medicine, it is an important “cold property” herb used to rid the body of heat, as in fevers and to dispel toxins from the body. Traditionally, it is used as a remedy against various disease conditions such as the common cold, dysentery, fever, tonsillitis, diarrhoea, liver diseases, inflammation and herpes.16–18 A. paniculata (AP) is a predominant constituent of about 26 Ayurvedic formulations used to treat liver disorders.19 AP is a source of number of active principles, including diterpene lactones, flavonoids and polyphenols.20,21 Andrographolide (AN), a diterpenoid lactone, is the prime constituent responsible for the therapeutic properties of AP (Fig. 1).22 According to Indian Pharmacopoeia, AP comprises dried leaves and tender shoots, which yield not less than 1% AN on a dry-weight basis.19 AP also contains various other chemical constituents. They are listed in the Table 1 along with their molecular weights and melting points.23 AN was first isolated in 1911 and characterized in the same year as a trihydroxy lactone by Gorter.24 Subsequent studies of Schwyzer et al., 1951; Kleipool and Kostermans, 1951; Kleipool, 1952; Chakravarti and Chakravarti, 1952; Kondo and Ohno, 1958; Cava and Weinstein, 1959; Chan et al., 1960; and Cava et al., 1962, 1963 and 1965 led to the assignment of the carbon skeleton with almost all the stereochemical centres except for the geometry of the C(12)–C(13) olefinic bond and the relative configuration of the C(14) allylic hydroxyl substituent.25–35 The crystal structure of AN was determined and the absolute configuration at C-14 was established as R in 1982.36 AN exhibited various activities such as anti-microbial,37 analgesic,38 hypotensive,39 anti-hyperglycemic,40 oxygen radical scavenging,41 atherosclerotic,42 anti-HIV,43 anti-platelet aggregation,44 hepatic lipid peroxidation protective,45 choleretic effect,46 and anti-periodic.47 It also used in the treatment of myocardial ischemia,48 pharyngotonsillitis,49 respiratory tract infections50 and the common cold.51 Since it was first isolated and characterized in 2011, tremendous research developments have occurred with respect to its pharmacological activity, therapeutic usage and formulation. The authors present a centennial review of the AN chemistry, structure active relationship, mechanism of action, metabolism, toxicology, analytical method development, NDDS and patents. The review also discusses the challenges ahead in the future developments of AN.
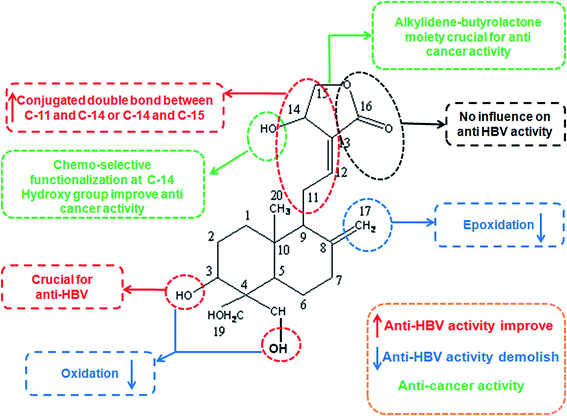 |
| Fig. 1 Schematic of the andrographolide structural activity relationship. | |
Table 1 Chemical constituents of A. Paniculata
Name of compounds |
Molecular formula |
Melting point °C |
Andrographolide |
C20H30O5 |
230–239 |
14-Deoxy-andrographolide |
C20H30O4 |
175 |
14-Deoxy-11-oxoandrographolide |
C20H28O5 |
98–100 |
14-Deoxy-11,12-didehydro andrographolide |
C20H30O4 |
203–204 |
Homo andrographolide |
C22H32O9 |
115 |
Andrographosterol |
C23H38O |
135 |
Neo-andrographolide (non bitter) |
C26H40O8 |
167–168 |
Andrographone |
C32H64O |
85 |
Andrographane |
C40H82 |
67–68 |
Andrographolide chemistry
The chemical name of AN is 3-[2-{decahydro-6-hydroxy-5-(hydroxymethyl)-5,8a-dimethyl-2-ethylene-1-naphthalenyl}ethylidene] dihydro-4-hydroxy,2(3H)-furanone with a molecular weight of 350.46. In native form, AN occur as a white crystalline powder with a bitter taste. The melting point, boiling point and pKa are of 229–232 °C, 557 °C and 12.32, respectively. AN is relatively insoluble in water but dissolves in methanol, ethanol, pyridine, acetic acid and acetone. AN is unstable at a neutral to basic pH wherein it gets hydrolyzed to an inactive product.52 When subjected to ultra-violet spectrophotometric investigation, AN displays an absorption maxima of 223 nm in methanol.
Andrographolide structural activity relationship
A small library of 3,14,19-modified derivatives of AN were synthesized and tested for in vitro inhibitory activities to cancer cell growth and proliferation and in vivo toxicities against zebrafish embryo development. The study revealed that the property of a substituent, substituted positions and 14-stereochemistry together determined a compound's in vitro anti-proliferative activity against cancer cells, the in vivo toxicity to zebrafish and the selectivity between MDA-MB-231 and A549 cells.53
Nanduri et al., synthesized and analyzed the different analogues of AN for their anticancer activity. They found that (a) the intact α-alkylidene γ-butyrolactone moiety of AN, (b) the Δ12(13) double bond, (c) the C-14 hydroxyl or its ester moiety and (d) the Δ8(17) double bond or epoxy moiety are responsible for the cytotoxic activity.54 The presence of the alkylidene part attached to the butyrolactone ring is crucial for cytotoxicity. Possibly, the activity of AN and its unsaturated analogues is associated with their ability to promote the alkylation of biological nucleophiles (e.g. enzymes) through a Michael addition to the alkylidene–butyrolactone moiety. Increase in lipophilicity of AN by protection of 3,19-hydroxyl groups has detrimental anticancer effects.55
Chen et al., synthesized and evaluated 48 derivatives of dehydro AN for their anti-hepatitis B virus (HBV) activity. They found that (a) 19-O-substituted compounds with the free hydroxyl group at C-3 could provide higher anti-HBV activity than other analogues, (b) 3,4,5-trimethoxycinnamoyl, nicotinoyl, 2-furoyl and 2-thenoyl groups are favourable to enhance anti-HBV activity, (c) the double bond between C-8 and C-17, and the conjugated double bonds between C-11 and C-14 or C-12 and C-15 are necessary for maintaining anti-HBV activity, decreasing cytotoxicity and (d) the methylene at C-15 is necessary for anti-HBV activity.56 Schematic of AN structural activity relationship is shown in Fig. 1.
Based upon the discovery of the natural compound AN as a non-steroidal farnesoid X receptor (FXR) antagonist, a series of AN derivatives were synthesized and the structural activity relationship (SAR) studies demonstrated that 14-phenoxy AN scaffold was an excellent structural pharmacophore for FXR antagonists. Remarkably, 14b-compounds of 12b, 12f and 10g were found to be the most potent FXR antagonists. Structural docking discovered that the phenoxy substitution at the 14-position and the modification at 3,19-positions altered the putative binding positions of small FXR ligands, resulting in an FXR antagonistic activity discrepancy.57
Andrographolide mechanism of action
AN has a wide spectrum of activity ranging from anti-inflammatory to anticancer. Table 2 is a comprehensive account of the biological activity of AN. Its mechanism of action is illustrated in Fig. 2 and 3.
Table 2 Biological activities and mechanism of action of andrographolidea
Biological activities |
Mechanism of action |
References |
AN: andrographolide, NO: nitric oxide, Cox-2: cyclooxygenase-2, MAPK: mitogen-activated protein kinases, ACTH: adrenocorticotropic hormone, CD4: cluster of differentiation 4, CDK2: cyclin-dependent kinase 2, HIV: human immunodeficiency virus and E. coli: Escherichia coli. |
Hepatoprotective |
Interaction with GSH ↑ BNF induced CYP1A1 mRNA expression in B6 mouse |
58–61 |
↑ GSTP expression is mediated by the PI3k/Akt pathway |
↑ CYP1A1 and CYP1A2 mRNA expression levels |
The schematic representation of the hepatoprotective activity of AN shown in Fig. 2 |
Anti-inflammatory and anti-pyretic |
(i) Inhibits iNOS and Cox-2 expression through inhibiting p38 MAPKs activation |
62 |
(ii) Inhibits neutrophil adhesion and transmigration |
(iii) Increases cortisol (natural anti-inflammatory) level through ACTH signals |
Anti-cancer |
Cell-cycle arrest at G1/G0 phase by the induction of cell-cycle inhibitory proteins p16, p21, p27 and concomitant (a) decrease in the expression of cyclin A, cyclin D, CD4 (dependent kinase) and CDK2, required for G1 to S transition and (b) inhibition of DNA topoisomerase II. The schematic representation of anti-cancer activity of AN shown in Fig. 3 |
63 |
Anti-hyperglycemic |
(a) Increases glucose utilization to lower plasma glucose in diabetic rats lacking insulin and |
62 |
(b) Inhibits oxidative stress |
Anti-HIV |
(a) Prevents transmission of the virus to other cells |
64 |
(b) Stops progress of the disease by modifying cellular signal transduction |
(c) Inhibits C-mos-kinase involved in HIV propagation |
Anti-diarrhoeal |
Inhibition of the intestinal secretory response induced by heat-labile E. coli enterotoxins through the stimulation of adenylate cyclase/activation of guanylate cyclase |
65 |
Anti-malarial |
In vitro and in vivo inhibition of Plasmodium berghei growth |
66 |
Anti-leishmaniasis |
AN react with the active cysteine residues, effectively inducing extrusion of cellular glutathione and converting the intracellular reactive oxygen. AN triggers an alternation of intracellular reactive oxygen species and eventually induces collapse of mitochondrial membrane potential |
67 |
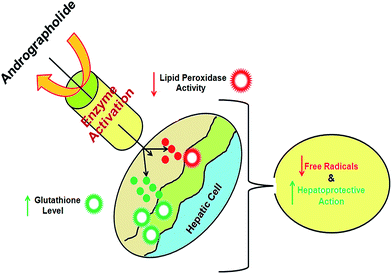 |
| Fig. 2 Schematic of the hepatoprotective activity of andrographolide. | |
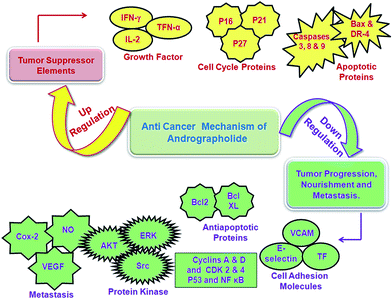 |
| Fig. 3 Anti-cancer effect of AN. AN modulates various biochemical pathways of cancer cells thereby inhibiting the tumor growth. The compound exerts cytotoxic effect on various cancer cell types in a time and dose dependent manner. | |
Metabolism of andrographolide
Pharmacokinetic studies showed that AN is quickly absorbed and extensively metabolised in rat and humans.68 He et al., isolated ten sulfonated AN metabolites from rat urine, feces and the contents of the small intestine after the drug was orally administered to the rat.69,70 Among them, one metabolite, 14-deoxy-12(R)-sulfo AN, was found to be identical to an injectable anti-inflammatory drug (Lianbizhi) currently in clinical use in China.71 Cui et al., identified 12 new AN metabolites in human urine after oral administration, three of which were characterized as 3-O-sulfate conjugates, one as a 3-O-sulfate-12-S-cysteine conjugate and the remaining 8 as 19-O-β-D-glucuronide.72,73 Cui et al., also demonstrated that AN was extensively metabolized through a variety of pathways to form a large number of metabolites in humans. The pathways include oxidation, hydrolysis, isomerization, glucuronidation, sulfation and the conjugation of urea, cysteine, creatinine and hydrazine. Zhao et al., studied comparative in vitro metabolic profiles of AN using pooled human (HLMs), dog (DLMs) and rat (RLMs) liver microsomes (Fig. 4). Eight phase I (M1-1 to M1-8) and five phase II (M2-1 to M2-5) metabolites resulted from dehydration, deoxygenation, hydrogenation and glucuronidation were identified by accurate mass measurement and MS/MS fragmentation behavior. A dehydration reaction was detected in all liver microsome incubation systems. Deoxy-AN and the related glucuronide metabolites were observed in HLMs only.74
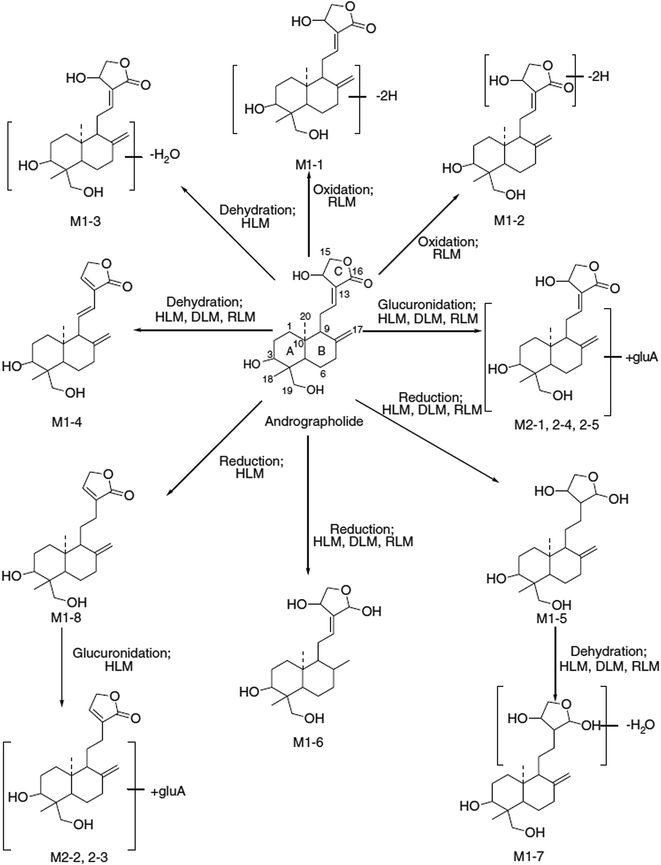 |
| Fig. 4 The possible metabolic pathways of andrographolide in human (HLMs), dog (DLMs) and rat (RLMs) liver microsomes. Reprinted (adapted) from Zhao et al.,74 with permission from John Wiley & Sons. | |
Andrographolide toxicity
Wang et al., reported a lethal dose (LD50) of 13.4 g kg−1 for total diterpene lactones, >20 g kg−1 for deoxy-, neo- or dehydro-AN and >40 g kg−1 for AN in mice.75 While traditional doses of 9–15 g of powdered herb once a day provided 90–150 mg AN per day, clinical trials have used much higher doses of 2.25–22.5 mg kg−1 or over 1500 mg per day in a 70 kg subject.76 AN (200, 600 and 2000 mg kg−1) cannot induce progesterone-mediated termination of pregnancy in rats.77 No testicular toxicity was found with the treatment of 20, 200 and 100 mg kg−1 of AP in male Sprague Dawley rats.78 However, two cases of urticaria have been reported.79 AN proved to affect spermatogenesis in rats by preventing cytokinesis of the dividing spermatogenic cell lines with the appearance of sertoli cell damage and spermatotoxic effects.78,80
Analytical methods for andrographolide
Various analytical techniques have been developed for AN that include gravimetric,81 chemical titration,82 spectrophotometric,83 thin layer chromatography (TLC),84 capillary electrophoresis chromatography (CE),85,86 high performance liquid chromatography (HPLC),87–89 HPTLC,90 chemiluminescence,91 high-performance liquid chromatography with a UV detector and a mass detector (HPLC-UV, HPLC-MS),92 microemulsion electrokinetic chromatography (MEEKC),93 high-performance liquid chromatography-photodiode array detection-electro spray mass spectrometry (HPLC-DAD-ESI/MS),94 micellar electrokinetic chromatography (MEKC)95 and micellar electrokinetic capillary chromatography (MECC).96 Few analytical chemists have validated the bio analytical methods for AN, predominantly by HPLC in rabbit serum97 and plasma,98 HPLC in rat blood,99 high performance liquid chromatography/electrospray-mass spectrometry (HPLC/ESI-MS) in human plasma100 and HPLC in human plasma.101
Andrographolide delivery systems
AN has a low oral bioavailability (2.67%) leading to a poor therapeutic application.102 The factors attributed to poor oral bioavailability include site specific absorption from the upper part of the GI tract, pH dependent hydrolysis in neutral or weak alkaline environments of the intestine, rapid metabolism to an impermeable hydrophilic sulphate metabolite (14-deoxy-12-sulfo-AN) in the intestine (duodenum and jejunum), first pass metabolism in both the intestine and the liver, P-gp (P-glycoprotein) excretion in the terminal intestine (ileum and colon), biliary excretion and high lipophilicity (log
P 2.632 ± 0.135) with a low aqueous solubility (3.29 ± 0.73 μg mL−1). Considering these limitations, researchers have designed various drug delivery systems to enhance its therapeutic efficacy (Fig. 5).103 Each of these systems has been discussed in detail in the following sections.
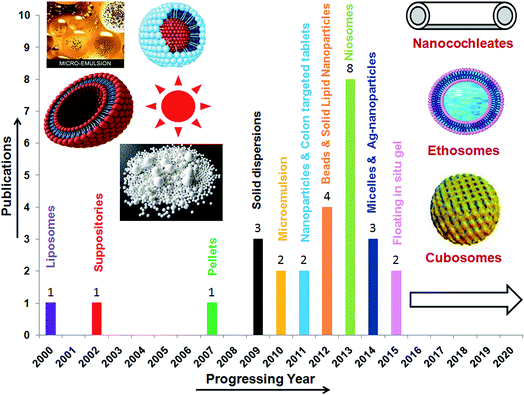 |
| Fig. 5 Chronological developments in andrographolide drug delivery systems. | |
Liposomes
Liposomes are small spherical shape lipid vesicles fabricated from cholesterol and various natural phospholipids. They help to improve the solubility, bioavailability, intracellular uptake of components, in vitro and in vivo stability, the pharmacokinetics and bio-distribution pattern of active moieties.104,105 Biocompatibility, biodegradability, site-specificity, low toxicity and the ability to entrap both the hydrophobic and hydrophilic molecules are the striking features of liposomes that make them highly favoured in the cosmetic and pharmaceutical industries. Literature revealed that numerous liposomal products are available on the market and many more are in various stages of clinical trials.106 Apart from above mentioned reasons, flexibility and compatibility were the driving force for the development of liposomal delivery systems that were expected to overcome the problems associated with therapeutic potential of AN.
AN intercalated multi-lamellar liposomes were prepared using phosphatidylethanolamine
:
cholesterol
:
dicetyl phosphate
:
AN in the ratio of 7
:
1
:
1
:
1 by a film hydration method. The NH2-group of phosphatidylethanolamine was coupled with p-amino phenyl-a-D-mannopyranoside using glutaraldehyde as the coupling agent to form mannosylated liposomes. The mannosylated liposomes improved the efficacy of AN and an 86% reduction of the splenic parasitic (Leishmania donovani amastigotes) burden was achieved. It was concluded that the developed liposomes selectively bound to mannosyl-fucosyl receptors and demonstrated better efficacy. It also reduced the hepatic and renal toxicity. In addition, mannosylated AN-loaded liposome-treated animals showed a normal blood image and splenic tissue histoarchitecture when compared with those treated with free drug or conventional liposomes.107 Herbosomes were prepared by hydrating an AN–soya-phosphatidylcholine (SPC) complex film with cholesterol in a phosphate buffer saline. The AN–herbosomes (448.12 ± 11.24 nm) showed better absorption as compared to pure AN in the everted rat intestinal sac model. The amphiphilic nature of the AN–SPC complex greatly enhanced the water and lipid solubility of AN leading to an increased absorption of AN through the intestine and improved the hepatoprotective activity.108
Niosomes
Niosomes are nonionic surfactant based vesicles formed by the self assembly of nonionic amphiphiles in aqueous media resulting in closed bilayer structures. Niosomes have been prepared from different classes of nonionic surfactants such as polyoxyethylene alkyl ethers, sorbitan monoesters (Span 20, 40, 60 and 80) and polyoxyethylene sorbitan monoesters (Tween 20, 60, 61 and 80).109 Cholesterol added to the nonionic surfactant provide rigidity and orientation order to the niosomal bilayer. Niosomes have many advantages such as better patient compliance and therapeutic effects than conventional oily formulations, high capability of entrapping hydrophilic, lipophilic as well as amphiphilic drugs and more stable than liposomes. Moreover, it can also protect the drugs from biological enzymes and deliver targeting drugs to the certain organs.110 AN-loaded niosomes were prepared using span-60 and cholesterol by film hydration method. The prepared niosomes showed 72.36%, 5.90% and 206 nm of drug entrapment efficiency, drug-loading and average particle size, respectively. Biological activity and tissue distribution study revealed that the niosomes reduced AN elimination and caused a greater accumulation in the liver as compared to free AN, indicating its potential for liver targeting. In addition, niosomes maintained the anti-hepatocellular carcinoma (HCC) activity of AN in HepG2 cell.111
Nano-particulate systems
Nanotechnology has provided a unique platform in the field of drug delivery. Nanoparticles were developed around 1970 and since then there has been considerable interest in developing nanoparticles as effective drug delivery carriers.112 Nanoparticles are solid, colloidal particles comprising macromolecular substances that vary in size from 10 nm to 1000 nm in which the active principle is dissolved, entrapped or encapsulated and/or to which the active principle is adsorbed or attached, which can be administered in fluid form with a liquid carrier.113 Nanotechnological drug delivery modalities include nanoparticles, nanoemulsions, polymeric nanoparticles, solid lipid nanoparticles (SLNs), nanocapsules, nanospheres, and polymeric micelles. The prime idea is to control particle size, surface properties and the release of pharmacologically active agents to achieve site-specific action at the therapeutically optimal rate and dose regimen. Nano-particulate systems also offer an enhancement in solubility, bioavailability and penetration through biological barriers. This technology also helps to reduce the dose and improve the absorption potential of phytoconstituents.114,115
Solid lipid nanoparticles
Solid lipid nanoparticles (SLNs) are colloidal carriers made up of lipids that remain solid at room and body temperature and also offer unique properties such as small size (50–500 nm), favourable zeta potential (responsible for its stability) and less toxicity. The SLN matrix is composed of physiologically tolerated lipid components, which decreases the potential for acute and chronic toxicity.116 Some other properties of SLNs are pseudo-zero order kinetics and the prolonged/sustained release of drugs.117
AN-loaded SLNs were formulated by a cold high-pressure homogenization method using glyceryl behenate (Compritol ATO888) and glyceryl-monostearate (GMS) as lipid carriers, lecithin and Tween-80 as surfactant. AN-SLN showed high drug loading (3.49%), drug-entrapment efficiency (91%) with an average diameter of 286.1 nm and zeta potential of −20.8 mV. The AN–SLNs exhibited a 2.41 times improvement in oral bioavailability of AN as compared to a pure AN suspension. The enhancement of bioavailability and anti-hyperlipidemic activity of AN by SLNs was attributed to its increase in solubility, absorption of AN by passive transport or endocytosis, prevention of duodenal metabolism (14-deoxy-12-sulfo-AN) and P-glycoprotein efflux transport in the colon.116 Moreover, AN-loaded SLNs were prepared by a solvent injection method using cetyl alcohol as a lipid carrier, Tween 80 as a surfactant, polyvinyl alcohol as a stabilizer and ethyl alcohol as an organic solvent. These SLNs (154.1 ± 10.7 nm) demonstrated sustained release (77.89% w/v) of AN up to 36 h. They exhibited a 3.4 and 3.9-fold improvement in oral AUC and Cmax, respectively, in rats leading to more drug availability at the site of action over a prolonged period, which reflected in an improvement in the anticancer activity against EAC tumor bearing mice. It also helped to reduce the variability in systemic drug levels with dose reduction.117
Polymeric nanoparticles
Polymeric nanoparticles are solid colloidal particles prepared from variety of biodegradable polymers and their co-polymers. Commonly used polymers are chitosan and collagen or non-biodegradable polymers such as poly(lactic acid) (PLA) and poly(lactic co-glycolic acid) (PLGA). These multi-functionalized polymeric material yield micelles, nanocapsules, nanospheres, dendrimers, core–shells, and nanoparticle incorporated polymer matrices. Their small size (50–300 nm) allows the particles to penetrate capillaries and to be taken up by cells, increasing the accumulation of the drug at the target site of action.118
Our research group prepared the AN-loaded pH sensitive polymeric nanoparticles by nanoprecipitation technique using Eudragit® EPO (cationic poly methacrylate copolymer) and Pluronic® F-68 as the stabilizer. The nanoparticles showed a high-encapsulation efficiency (93.8% ± 0.67%) with a particle size and zeta potential of 255 ± 9 nm and of 29.3 ± 3.4 mV, respectively. An in vivo study in rats exhibited a 2.2 and 3.2-fold increase in oral AUC and Cmax, respectively, with a 121.53% increase in its relative bioavailability (Table 3).102 The improvement in bioavailability was reflected in the hepatoprotectivity against CCl4-induced hepatotoxicity in rats, which may be due to its reduced particle size, increased surface area and reduced diffusion layer thickness. In another study, our group formulated a sustained release injectable AN-loaded biodegradable poly(ε-caprolactone) (PCL) nanoparticle suspension by a solvent displacement process using poly(ethylene oxide)–poly(propylene oxide)–poly(ethylene oxide) (PEO–PPO–PEO) triblock polymeric stabilisers (Pluronic® F-68/Pluronic® F-127). The nanoparticles showed a controlled zero order drug release up to 35 h that followed diffusion mechanism.119 Another group prepared AN-loaded biodegradable poly(D,L-lactide-co-glycolic acid) (PLGA) nanoparticles by an emulsion solvent evaporation technique using polyvinyl alcohol as a stabilizer. These nanoparticles were 5 and 8 times more efficacious than pure AN and sodium stibogluconate in monocyte–macrophage cells infected with the leishmanial pathogenic amastigote.67 Zhang et al., formulated a AN-loaded biodegradable poly(D,L-lactide-co-glycolide)-b-poly(ethylene glycol(-b-poly(D,L-lactide-co-glycolide) (PLGA–PEG–PLGA) amphiphilic triblock co-polymeric micelles by a solvent evaporation method. The micelles represented stable mono dispersed suspension with suitable particle size (124.3 ± 6.4 nm) and high encapsulation efficiency (92%). In vitro cytotoxicity study of micelles revealed higher proliferation inhibition, cell cycle arrest at the G2/M phase and pro-apoptosis effects in MAD-MB-231 cells attributable to higher efficiency of cellular uptake and intracellular transport. Furthermore, micelles showed a 2.7 and 2.5-fold increase in bioavailability and mean residence time of AN in rat plasma as compared to raw AN suspension.120 Moreover, the nanoparticulate system exhibited three fold improvements in anticancer potency. Furthermore, chitosan coated AN–PLGA nanoparticulates accentuated cellular localization, induced G1 cell cycle arrest and increased cellular toxicity and apoptosis in MCF-7 cells (Fig. 6). The charge modulation by chitosan led to a more efficient traverse through the cytoplasm and accumulation in the nucleus. An in vivo study confirmed that the nanoparticles reduced tumor weight by 68.21% as compared to 24.7% by pure AN and increased the life span of mice infected with Ehrlich ascites carcinoma (EAC) by 78.08% as compared to 23.5% for AG alone.121 Heparin functionalized AN-loaded PLGA nanoparticles were formulated using a facile emulsion solvent evaporation technique. Low amounts of heparin on the nanoparticles surface helped with localisation in the liver and the consequent efficient hepato-protection in paracetamol induced acute liver failure.122
Table 3 Pharmacokinetic parameters of andrographolide after a single oral dose (10 mg kg−1) administration of andrographolide-loaded pH-sensitive nanoparticle suspension and pure andrographolide in rat serum. Reprinted (adapted) from Chellampillai and Pawar with permission from Springera102
Parameters |
Pure andrographolide |
Andrographolide nanoparticles |
Each value represents the mean ± S.D. of six rats. AUC: area under the serum concentration–time curve from 0 h to ∞, AUMC: area under the first moment time curve from 0 h to ∞, Cmax: peak concentration, Tmax: time to reach peak concentration, t1/2Ke: elimination half-life of andrographolide, Ke: elimination rate constant, Cl/F: apparent oral clearance, Vd/F: apparent volume of distribution, MRT0–∞: mean retention time, Fr: relative bioavailability. *P < 0.05 significantly and **P < 0.05 no significantly different from pure drug. |
Cmax (μg mL−1) |
0.83 ± 0.05 |
2.67 ± 0.13* |
Tmax (h) |
1.00 ± 0.06 |
0.25 ± 0.02* |
AUC0–∞ (μg h mL−1) |
2.169 ± 0.143 |
4.807 ± 0.261* |
AUMC0–∞ (μg h mL−1) |
4.099 ± 0.061 |
8.941 ± 0.070* |
t1/2Ke (h) |
1.33 ± 0.04 |
1.30 ± 0.09** |
Ke (h−1) |
0.522 ± 0.017 |
0.533 ± 0.023** |
Cl/F (mL h−1 kg−1) |
4610.53 ± 80.45 |
2080.29 ± 54.38* |
Vd/F (mL kg−1) |
8713.23 ± 122.37 |
3868.32 ± 80.39* |
MRT0–∞ (h) |
1.89 ± 0.43 |
1.86 ± 0.27** |
Fr (%) |
— |
221.53 |
R2 |
0.996 |
0.997** |
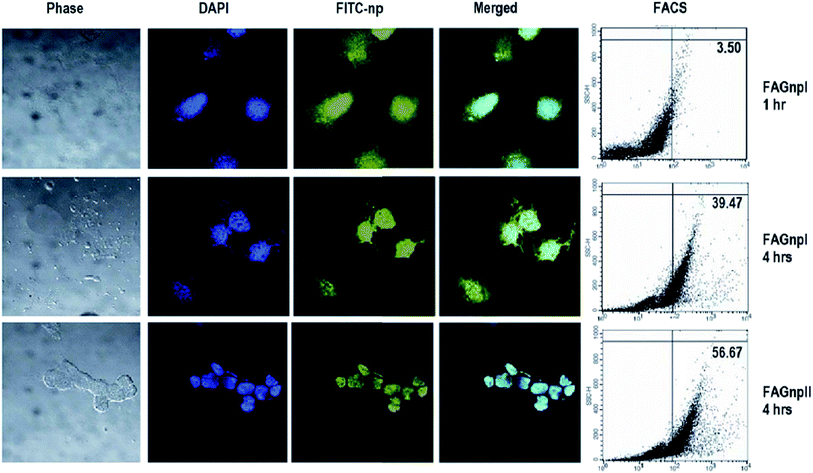 |
| Fig. 6 Uptake of FITC-tagged AG nanoparticles in human breast cancer cells. MCF-7 cells were incubated with FITC-tagged AGnps and CAGnps for 1–4 hours, washed and counter-stained with DAPI, and visualized under a confocal microscope. Alternately, cells were harvested and subjected to FACS analysis for quantitation of FITC-tagged nanoparticle accumulation within the cells. Reprinted (adapted) from Roy et al.,121 with permission from Bentham Science. | |
AN loaded PLGA (50
:
50) nanoparticles (ANnps) stabilized by P-gp efflux inhibitor vitamin E TPGS (D-α-tocopheryl polyethyleneglycol 1000 succinate) were prepared for delivery into macrophage cells infested with sensitive and drug resistant amastigotes of Leishmania parasites. Antileishmanial activity was found to be significant for ANnps with TPGS in about one-tenth of the dosage for the free AN and one-third of the dosage for the ANnp without TPGS. Similar observations were also found in the case of in vitro generated drug resistant and field isolated resistant strains of Leishmania. Macrophage uptake of ANnps was almost complete within one hour. The low-selectivity of AN in in vitro generated drug resistant and field isolated resistant strains was improved in the case of AN nanomedicines designed with vitamin E TPGS (Fig. 7).123 Das et al. investigated the effectiveness of polylactide-co-glycolide (PLGA) nanocapsulated AN (NA) against arsenic induced liver damage in mice. Protective efficiency of NA was about five times more than that of AN. Administration of NA to arsenic-treated mice caused signs of improvement in liver tissue architecture.124
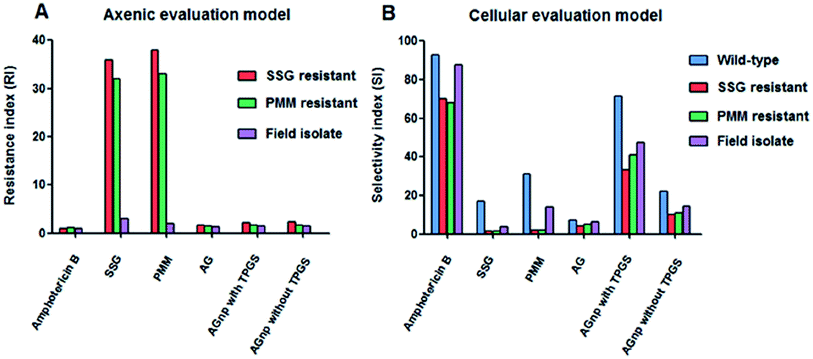 |
| Fig. 7 Characterization of the resistance index (RI) in axenic L. donovani amastigotes and selectivity index (SI) in cellular L. donovani amastigotes. Panel (A) nature of resistance indexes (RIs) of amphotericin B, SSG, PMM, AG, AGnp with TPGS and AGnp without TPGS in axenic amastigites of SSG resistant, PMM resistant and field isolate L. donovani. RI is defined, as given in Table 2. Panel (B) nature of selectivity indexes (SIs) of amphotericin B, SSG, PMM, AG, AGnp with TPGS and AGnp without TPGS in cellular amastigotes of wild-type, SSG resistant, PMM resistant and field isolate L. donovani. SI is defined as CC50/IC50. Reprinted (adapted) from Mondal et al.,123 with permission from PLoS One. | |
GA (glycyrrhetinic acid) and PHIS (poly(L-histidine)) were conjugated to form poly(ethylene glycol)–poly(lactic-co-glycolic acid) (GA–PEG–PHIS–PLGA, GA–PPP) micelles by a grafting reaction between the active terminal groups, AN was encapsulated to GA–PPP to make AN/GA–PPP to target HCC (hepatocellular carcinoma) that have GA binding receptors and release its encapsulated AN in the acidic microenvironment of HCC. The encapsulated AN released faster in vitro at pH 5.0. The AN/GA–PPP micelle uptake was more efficient in human Hep3B liver cells than that of human MDA-MB-231 breast cancer cells. GA–PPP micelles accumulated specifically in the liver and possessed long retention time in vivo. AN/GA–PPP micelles significantly inhibited tumor growth and provided better therapeutic outcomes compared to free AN and AN/PEG–PLGA (AN/PP) micelles without GA and PHIS decoration (Fig. 8).125 The incorporation of AN with different poly(D,L-lactide-co-glycolide) (PLGA) nanoformulations enhanced the antileishmanial activity of AN against axenic and intracellular amastigote forms of L. donovani. Among the formulations tested, the 175 nm AN-loaded nanoparticles exhibited the best antileishmanial activity (IC50 = 36 and 28 μM for axenic and intracellular amastigotes, respectively).126 Interestingly, particle size also proved to be an important factor for drug delivery efficacy. In fact, nanoparticles in a size range below 200 nm have been associated with an increase in phagocytosis by Leishmania-infected macrophages.
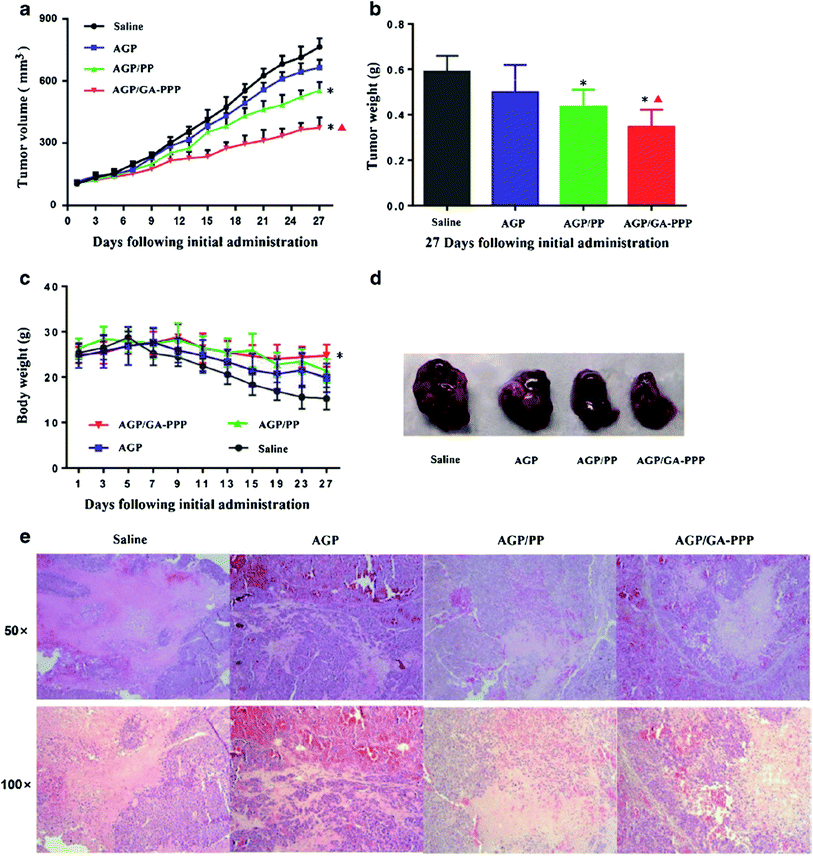 |
| Fig. 8 In vivo anticancer activity of AGP/GA–PPP micelles. (a) The tumour volume growth of xenografts in all groups; (b) the tumour weight of mice in all groups after last administration; (c) the body weight of mice in all groups during the treatment period; (d) representative tumour tissues in all groups after treatment; (e) representative images of tumour tissue after last treatment by H&E staining for histopathological analysis. Reprinted (adapted) from Zhang et al.,125 with permission from Springer. | |
Microparticulate systems
Microparticles are small solid particles within the size range of 1–200 μm. Depending upon the method of preparation, the drug is dissolved, entrapped and encapsulated into the microparticle matrix. The microparticles offer a variety of opportunities such as protection and masking of entrapped dug, reduced dissolution rate, facilitation of handling and spatial targeting of the active ingredient.127 From last few years, polymeric microparticles with different geometries have gained numerous interesting applications in biomedical science, especially drug delivery. Usually, microparticles comprise a heterogeneous distribution of different polymers together with encapsulated agents integrated inside a polymeric shell layer. Based on the ultimate application, different hydrophobic and hydrophilic polymers can be utilized during the fabrication process.128
A sustained-release injectable formulation containing AN-loaded poly(D,L-lactide-co-glycolide acid) (PLGA) microspheres was prepared by solid-in-oil-in-water (s/o/w) emulsion solvent evaporation method. AN-microspheres were spherical with smooth surface and showed 75.79% ± 3.02% and 47.06% ± 2.18% entrapment efficiency and drug loading capacity, respectively. Moreover, encapsulation of AN into PLGA helped in drug loading to overcomes the defects of conventional oral dosage forms of AN (tablets and dripping pills), which included low bioavailability and frequent administration. After a single intramuscular injection in rats, the microspheres maintained a relatively high sustained plasma concentration of AN over one week with a 40 fold improved mean retention time as compared to a pure AN solution (Fig. 9).129 Our research group formulated a AN-loaded floating micro-particulate system by adsorbing AN onto the low-density porous Cavilink™ (prepared by suspension polymerization by high internal phase emulsion (HIPE) technique using porous styrene and divinylbenzene) bead through solvent evaporation method. Beads showed high encapsulation efficiency (52% to 96%), excellent buoyancy (13 h) and controlled zero order drug release up to 10 h.103 This floating micro-particulate system could improve solubility and absorption of AN in upper part of the GIT, which can reduce dosing regimens, increase compliance and further enhance the therapeutic effect of AN.
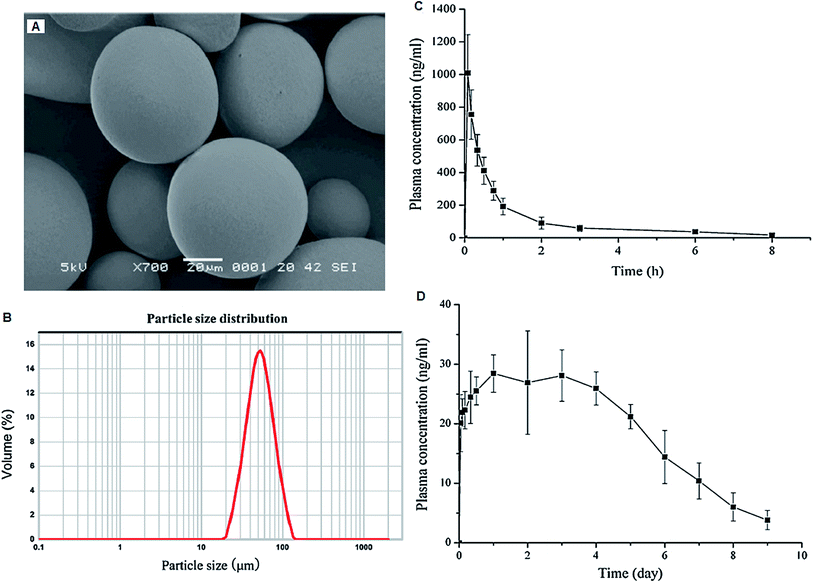 |
| Fig. 9 SEM image (A) and particle size distribution (B) of AG loaded PLGA microspheres. Mean plasma concentration–time profiles of AG in rats following (C) i.v. administration of pure AG solution at a single dose of 2 mg kg−1 and (D) i.m. administration of AG loaded PLGA microspheres at a single dose of 10 mg kg−1 (mean ± SD, n = 6). Reprinted (adapted) from Jiang et al.,129 with permission from Elsevier. | |
Cyclodextrin complexes
Cyclodextrins (CDs) are cyclic oligosaccharides with a hydrophilic outer shell and a lipophilic cavity in the center, with α, β and γ being the three most commonly used forms of CDs. The bio-adaptability and multi-functional properties of CDs help to improve the solubility, bioavailability, tolerability and also to eliminate the negative side-effects of drugs.130–132 CD complexes can act as hosts for a wide variety of lipophilic drugs and are a versatile platform for novel drug delivery systems.133
AN–hydroxypropyl–β-cyclodextrin (HP–β-CD) complex was prepared by solvent evaporation method. An oral suspension of AN–HP–β-CD complex exhibited a 1.6-fold increment in AUC as compared to plain AN.134 β-CD helped to wrap the entire decalin ring of AN moiety in inclusion complex of AN–β-CD (1
:
1). The intermolecular hydrogen bonding between AN and β-CD helped to improve the stability and solubility of AN.135 In another study, AN–β-CD complex was prepared by a saturated aqueous solution method for topical delivery. It showed 72.42% encapsulation with improved solubility, in vitro dissolution and percutaneous absorption of AN.136 An AN–β-CD complex in molar ratio of 1
:
2 was prepared using a spray drying technique. It showed better dissolution performance and stability at different pH's as compared to pure AN. It also showed better stabilizing effects on the degradation of AN in comparison to the AN–γ-CD complex.137
Emulsion
An emulsion is a non-homogenous dispersion of two or more immiscible liquids stabilized by surfactant, sub-surfactant and emulsifiers. Drug packaging in the inner core of emulsion facilitates sustained release of drug over a long period of time.138 The size of the emulsion droplets play a significant role in the targeted distribution of drugs. Emulsions help to concentrate the lipophilic drug moieties into the liver, spleen and kidney and hydrophilic drugs into lymphatic system via intramuscular or subcutaneous injection.11 An AN-loaded O/W microemulsion was prepared using an oil phase of isopropyl myristate (2.5% w/w), a surfactant phase of Tween 80 (25% w/w), a co-surfactant phase of alcohol (50% w/w) and water (22.5% w/w). The microemulsion exhibited a higher capacity of solubilization for AN (8.02 mg mL−1) as compared to intrinsic solubility of AN (74 μg mL−1) in water which was reflected in the improvement of anti-inflammatory activity in rats as well as higher biological availability in rabbits as compared to AN tablets.139 In order to avoid first pass metabolism, stable AN loaded O/W microemulsion was prepared by using EO (ethylene oxide)/Tween-80/PEG-600/water for a sustained release transdermal delivery of AN.140
Pellets/beads
Pellets/beads are small, spherical, free-flowing granules with a narrow size distribution, typically varying between 500 and 1500 μm. These are particulates that are formulated from agglomerates of fine powders or granules of bulk drugs and excipients for pharmaceutical applications. Pellets help to control the variations in gastric emptying rates and overall transit times associated with single-unit regimens.141 Pellets also provide a stable plasma level; the lower local drug concentration reduces the irritation of GIT. Improvement in bioavailability is attributed to the equal distribution of the drug in GIT and the increased contact between the drug and body surface.142 AN-loaded bitterless micro pellets were prepared by an ionotropic gelation technique using sodium alginate and calcium chloride. AN-pellets showed a good entrapment efficiency and released 85% of the drug away from the stomach. Problems, such as vomiting, loss of appetite and nausea on regurgitation, were avoided by this gastric resistant formulation.143
Gel
In situ gel delivery system is a process of gel formation at the site of action after the formulation has been applied. The gelation occurs due to one or a combination of different stimuli such as pH change, temperature modulation, presence of ions and ultra violet irradiation. They are mainly administered by oral, ocular, rectal, vaginal, injectable and intraperitoneal routes. The in situ gel forming polymeric formulations offer several advantages such as sustained and prolong action in comparison to conventional drug delivery systems.144
Our research group designed AN-loaded floating in situ gel (ANFIG) using gellan gum and calcium carbonate for the treatment of gastric ulcers. The formulation gelled within 2 s and floated for more than 24 h in simulated gastric fluid. It exhibited an initial burst release of 11.7% ± 0.9% to 32.4% ± 2.1% till 1 h followed by a sustained release up to 24 h. ANFIG showed lower acid and protein levels in the gastric content, high haemoglobin level and negligible ulcer index in rats compared to pure AN and ranitidine. The gel improved anti-ulcer activity and preserved the integrity and histological aspects of the gastric mucosa (Fig. 10). Improved anti-ulcer activity of ANFIG was credited to a longer residence time of AN in the stomach, which improved the activity of myelo-peroxidase, lipid peroxide, mucin content and glutathione peroxidase on gastric mucosal surface leading to protection from alcohol induced erosion in rats.145 Silica xerogel and its polyethylene glycol (PEG) assisted derivatives were prepared by a sol–gel method for controlled release of AN. Drug release from the matrix increased with increasing percentage of PEG and followed a biphasic pattern. The in vitro release profile followed zero-order kinetics with erosion of the matrix for the first six hours followed by Higuchi model with the diffusion mechanism. The pharmacokinetic data obtained after intraperitoneal administration revealed sustained release of the drug from silica-drug composite with higher elimination half-life than the AN. Silica gel and PEG assisted derivatives could be used as a biocompatible and sustained release device for AN.146
 |
| Fig. 10 Antiulcer activity of AN-loaded floating in situ gel. Figure shows changes in morphological appearance of stomach treated with control (a), 80% ethanol (b), pure AG (c), AGFIG (d) and ranitidine (e). AG andrographolide, AGIFG andrographolide floating in situ gel. Reprinted (adapted) from Bothiraja et al.,145 with permission from the Royal Society of Chemistry. | |
Solid dispersion
An array of methods is available to improve the dissolution profiles of poorly water soluble drugs, including salt formation, micronization, and hot melt extrusion. Solid dispersion (SD) is one of the approaches used to improve the dissolution profile of poorly water soluble drugs. The term “solid dispersion” is defined as a dispersion of a drug in a solid matrix where the matrix is either a small molecule or polymer. The dispersed state includes many forms such as eutectic mixtures, crystalline/glass solutions and amorphous/crystalline suspensions. Currently three methods of preparing SDs have been successfully used in commercial production: (a) melt extrusion, (b) spray drying and (c) co-precipitation. SDs provide a unique platform to improve oral bioavailability of poorly soluble active pharmaceutical ingredients.147–149
Our research group prepared AN-SD with polyvinyl pyrrolidine (PVP-K-30) by a spray-drying technique. The microphotographs showed that pure AN comprised plate-shaped oblong crystals with fully developed corners; a few were cylindrical rods (Fig. 11). The spray-dried powder comprised spherical-shaped microparticles with a narrow particle size distribution and smooth surfaces. SD exhibited a five-fold increase in saturation solubility and dissolution of AN in a 0.1 M HCl, phosphate buffer at pH 6.8 and distilled water (Fig. 12). Enhanced drug dissolution and bioavailability was due to complete amorphization and intermolecular hydrogen bonding between AN and PVP-K-30.6 AN–cyclodextrin SD prepared by an ultrafine grinding method exhibited improvement in solubility and dissolution of AN. Furthermore, AN dispersible tablets formulated with AN–cyclodextrin SD ultra-fine powder too demonstrated an improvement in the dissolution rate of AN with the increase in the surfactant concentration of dissolution media.150 AN–hydroxyapatite SD (1
:
8) was formulated via solvent evaporation method. The prepared SD showed 93% drug release in 45 min. After the accelerated stability test for three months, the drug dissolution and AN content in SD showed no significant change.151
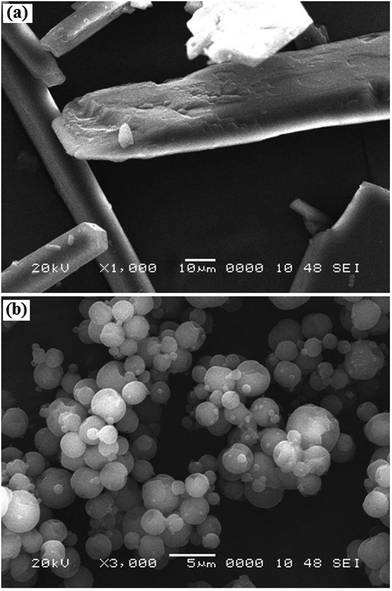 |
| Fig. 11 Scanning electron microscopy photographs of andrographolide (a) and solid dispersion with PVP K30 (b). Reprinted (adapted) from Bothiraja et al.,6 with permission from Wiley Online Library. | |
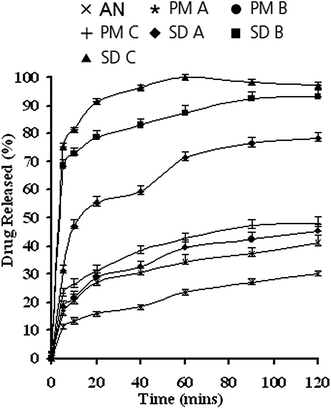 |
| Fig. 12 Dissolution profiles of isolated andrographolide, physical mixtures and solid dispersions in 0.1 M HCl. IAG, isolated andrographolide; PM, physical mixture; SD, solid dispersion. Reprinted (adapted) from Bothiraja et al.,6 with permission from Wiley Online Library. | |
Miscellaneous
The pH-dependent AN tablets were formulated with Eudragit S100 (coating material) and diethyl phthalate (elasticizer) for colon targeting. Prepared tablets did not release any drug in gastric juice (2 h) and artificial small intestinal juice (4 h), whereas 80% cumulative drug release was observed in an artificial colon within 2 h.152 AN oral disintegrating tablet was formulated using microcrystalline cellulose and cross-linked polyvinylpyrrolidone, which disintegrated within one minute and followed all other specifications according to the limit of Chinese Pharmacopoeia (2005 edition).153
Our research group prepared AN–humic acid (HA) complexes by a solvent evaporation method, which demonstrated improvement in dissolution and permeation across a rat intestine. In addition, this complex also showed better hepatoprotection action against carbon tetrachloride-induced liver toxicity of AN compared to free AN in rats.154 AN-loaded rectal suppositories were prepared by using PEG 4000 and 6000 base. Suppositories melted at body temperature in 15–16 min, were stable up to one month at various temperature conditions and did not exhibit any alteration in in vitro release.155 Du et al., compared the bioavailability of AN from microemulsions and pills in rabbit plasma. They observed a shorter Tmax (27.08 min) and higher biological availability (AUC 1406.72 μg mL−1) from oral AN microemulsions compared to pills (Tmax 61.04 min; AUC 877.37 μg mL−1).156
Wang et al. prepared AN and polyethylene glycol (PEG) 6000 composite particles by a mechanical crushing method. AN and PEG 6000 gave rise to coated composite particle structures with the decrease in the crystallinity of AN. The in vitro dissolution rate of the composite particles was significantly obvious than that of its raw materials, ultrafine powder and their physical mixtures.157
Crystal-engineering principles and hydrogen-bonding rules are the primary design elements for the preparation of co-crystals with predictable and robust supramolecular synthons.158 Studies on co-crystals with tailor-made properties have found immediate applications in pharmaceuticals.159 The ability to modify the physicochemical properties of active pharmaceutical ingredients (APIs)160 in co-crystals without altering the chemical structure of the active component has generated significant interest over the past decade, as highlighted by the solubility and stability improvement of drugs.161 Other applications of pharmaceutical co-crystals are improvement in the tableting behavior,162 color, photostability163 and hydration control.164 The abundance of co-formers in the GRAS (generally regarded as safe) list165 (over 1000) allows the crystal engineer to control the hydrogen bonding with the drug to obtain co-crystals with predictable crystal structures and molecular packing for modulating a given property.
As discussed previously, a significant decrease in the bioavailability of AN was due to the transformation of four metabolites isolated from humans and rats. Three of the AP metabolites are isomers of 14-deoxy-12-sulfoandrographolide and the fourth product is the S-conjugate (sulfated derivative) of AN. AN co-crystals were prepared with co-formers such as vanillin, vanillic acid, salicylic acid, resorcinol and guaiacol by liquid-assisted grinding methods to solve the chemical instability and poor solubility problems of AN. All co-crystals were iso-structural and the physicochemical properties were quite different. The AN–salicylic acid co-crystal completely inhibited the chemical transformation of AN to its inactive sulfate (AN–SO3H) metabolite. The acidic nature of the ortho-hydroxy acid in salicylic acid and lower pKa were reasoned as the cause for this inhibitory effect. The 12-fold improvement in solubility and the three-times-faster dissolution rate of AN–salicylic acid is an added benefit for oral formulation.166 Various novel drug delivery systems for AN and their key findings are summarized in Table 4.
Table 4 Various novel drug delivery systems of AN along with their key findings
Delivery system |
Key finding |
References |
AN-loaded mannosylated liposomes |
Reduced splenic parasitic burden |
107 |
AN–soya-phosphatidylcholine liposomes |
Enhanced oral absorption and cumulative release of AN |
108 |
AN loaded niosomes |
Improvement (2.14-fold) in liver targeting |
111 |
AN-loaded SLNs |
Enhancement in anti-hyperlipidemic activity by increasing the solubility and stability of AN in the intestine |
117 |
AN-loaded pH sensitive polymeric nanoparticles |
2.2 and 3.2-fold increase in oral AUC and Cmax respectively with a 121.53% increase in relative bioavailability |
102 |
AN-loaded poly(ε-caprolactone) nanoparticles |
Controlled zero order drug release up to 35 h |
119 |
AN–PLGA nanoparticles |
5 and 8 times more efficacious than pure AN in monocyte–macrophage cells infected with the leishmanial pathogenic amastigote |
67 |
AN-loaded PLGA–PEG–PLGA micelles |
Improved cellular uptake and intracellular transport |
120 |
AN-loaded PLGA nanoparticles |
3-Fold improvements in anticancer potency |
121 |
Heparin functionalized AN-loaded PLGA nanoparticles |
Improved antioxidant capacity and hepatic glutathione storage |
122 |
AN–PLGA microspheres i.m. injection |
Improved in retention time (40-fold) and sustained release |
129 |
AN-floating micro-particulates |
Controlled zero-order drug release |
103 |
AN–HP–β-CD complex |
Improvement (1.66-fold) in bioavailability |
134 |
AN–β-CD complex |
Improved solubility and stability, percutaneous absorption and |
135–137 |
AN–O/W microemulsion |
Improvement (1.60-fold) in AUC |
139 |
AN–O/W microemulsion |
Sustained transdermal release |
140 |
AN loaded micro pellets |
Taste masking of AN |
143 |
AN-loaded floating in situ gel |
Improved anti-ulcer activity of AN |
145 |
AN–PVP-K30 solid dispersion |
Improved solubility and anti-inflammatory activity |
6 |
AN–cyclodextrin solid dispersion |
Improved solubility and dissolution |
150 |
AN–hydroxyapatite solid dispersion |
Improved solubility and stability |
151 |
pH-dependent AN tablets |
Colon targeted |
152 |
AN–humic acid complexes |
Improved hepato protective action |
154 |
AN-loaded rectal suppositories |
Avoids hepatic first pass metabolism and improves bioavailability |
155 |
Andrographolide patents
Nanduri et al., patented the novel derivatives of AN, their stereoisomers, polymorphs; their pharmaceutically acceptable salts and their pharmaceutically acceptable solvates with an objective of treating cancer, HSV (herpes simplex virus), HIV, psoriasis, restonosis, atherosclerosis, cardiovascular disorders and metabolic disorders. They claimed that these derivatives were potent at lower doses and had better efficacy and lower toxicity.167 A novel oral composition comprising AN and α-lipoic acid for prevention or treatment of chronic fatigue syndrome, over fatigue, physical fatigue, mental fatigue and organ fatigue has been reported.168 The AN derivatives were synthesized by different substituents selected from hydrogen, substituted or un-substituted organic acid radicals, inorganic acid radicals, alkyl and aryl or heteroaryl. It was claimed that the derivatives have good antitumor effect, can induce apoptosis of tumor cells, directly kill Gram-positive bacteria (Staphylococcus aureus) and drug resistance bacteria (MRSA5676 and MRSA5677), inhibit the QS-system of Gram-negative bacteria (Pseudomonas aeruginosa), can inhibit and destroy the formation of biofilm of Pseudomonas aeruginosa and exhibits a significant hypoglycemic effect. It can be used in the manufacture of medicaments for the treatment of cancers, inflammation, diabetes, and bacterial and viral infections.169 Another lipophilic complexation between AN with phospholipids (herbosome) was patented. Herbosomes equivalent to 25 and 50 mg kg−1 of AN showed Cmax of 9.64 μg mL−1 and 6.79 μg mL−1, respectively, in rat plasma after oral administration. The resulting improvement in the pharmacokinetic parameters suggested that the AN loaded herbosomes can be used advantageously in the treatment of acute or chronic liver diseases.170
Challenges and future perspectives
It is clear that further development of AN will be dictated by the development of formulations that can address its bitter taste, low aqueous solubility, extensive hepatic first-pass metabolism and poor oral bioavailability, while not compromising the patient acceptability. Sublingual dissolving tablets provide a quick onset of action, because sublingual mucosa is rich in blood supply. They are especially suitable for drugs that undergo rapid hepatic first pass effect; one of the problems associated with AN. However, the bitter taste of AN becomes a hurdle. There is a need to develop taste masked sublingual formulations.
Mucoadhesive drug delivery systems can also be explored to enhance the efficacy of the AN. If a drug molecule is made to locate itself around its absorption window, it leads to an enhancement of its bioavailability. Absorption of AN across various mucosal sites needs to be assessed to develop mucoadhesive systems for its delivery.
The progress in NDDS has already boosted the use of phytoconstituents for various therapeutic aliments. Many novel drug delivery systems have been utilized to enhance the efficacy of AN. However, novel polymer synthesis, introduction of natural carriers, progress in particle engineering and superior understanding of the structure–function association have to be exploited to enable targeting of AN to various cells. This may be challenged by maintenance of its structural integrity, better stability in biological fluids, biocompatibility, ease and safety of production, targeting ability, reduced side effects and improved efficacy. For localized targeting, ligand based AN loaded carriers appear to have lot of potential to reach the clinic in the near future. Nanocochleates and ethosomes formulation are alternatives to maximise the potential of AN. Limitations such as low amounts of AN delivered and rapid clearance can be overcome by improving the encapsulation efficiency and use of long-circulating carriers, respectively. The optimistic view expressed by the author is based on the ever increasing numbers of formulations under clinical trials and advances observed in the last decade in AN formulation technology.
Conflict of interest
The authors declare that they have no conflicts of interest concerning this study.
Acknowledgements
The authors would like to acknowledge the support from Bharati Vidyapeeth Deemed University, Poona College of Pharmacy, Padmashree Dr D. Y. Patil College of Pharmacy, as well as the Modern College of Pharmacy, Pune, India.
Notes and references
- A. Kalia, Text Book of Industrial Pharmacognosy, Oscar publication, 1st edn, 2005 Search PubMed.
- P. Mehta, R. Shah, L. Sathiyanarayanan and K. R. Mahadik, Journal of Traditional and Complementary Medicine, 2015, 5, 207 CrossRef PubMed.
- S. Pal and Y. Shukla, Asian Pacific Journal of Cancer Prevention: APJCP, 2033, 4, 281 Search PubMed.
- A. Vickers and C. Zollman, BMJ 319, 1999, 1050–1053 CrossRef CAS.
- D. Newman, G. Cragg and K. Snader, Nat. Prod. Rep., 2000, 17, 215 RSC.
- C. Bothiraja, M. Shinde, S. Rajalakshmi and A. Pawar, J. Pharm. Pharmacol., 2009, 61, 1465 CrossRef CAS PubMed.
- I. Elliott, A. Howard and R. Theodore, J. Pharmacokinet. Pharmacodyn., 1974, 2, 511 CrossRef.
- J. Schranz, US Pat., 4368208A, 1983 Jan 11.
- P. Todd, US Pat., 4999205, 1991 Mar 12.
- H. Tonnesen, M. Masson and T. Loftsson, Int. J. Pharm., 2002, 244, 127 CrossRef CAS PubMed.
- A. Paradkar, A. Anshuman, K. Bhimrao and K. Mahadik, Int. J. Pharm., 2004, 271, 281 CrossRef CAS PubMed.
- A. Saraf, Fitoterapia, 2010, 81, 680 CrossRef PubMed.
- K. Sabu, P. Padmesh and S. Seeni, J. Med. Aromat. Plant Sci., 2000, 23, 637 Search PubMed.
- A. Woo, M. Waye, S. Tsui, S. Yeung and C. Cheng, J. Pharmacol. Exp. Ther., 2008, 325, 226–235 CrossRef CAS PubMed.
- N. Rao, Iran. J. Pharmacol. Ther., 2006, 5, 47 Search PubMed.
- C. Patarapanich, S. Laungcholatan, N. Mahaverawat, C. Chaichantipayuth and S. Pummangura, Thai J. Pharm. Sci., 2007, 31, 91 CAS.
- A. Panossian, T. Davtyan, N. Gukassyan, G. Gukasova, G. Mamikonyan, E. Gabrielian and G. Wikman, Phytomedicine, 2002, 9, 598 CrossRef CAS PubMed.
- S. Mishra, N. Sangwan and R. Sangwan, Pharmacogn. Rev., 2007, 1, 283 CAS.
- C. Khare, Indian Medicinal Plants: An Illustrated Dictionary, Springer, Berlin, Germany, 1st edn, 2007 Search PubMed.
- W. Li, X. Xu, H. Zhang, C. Ma, H. Fong, R. Van Breemen and J. Fitzloff, Pharm. Bull., 2007, 55, 455 CrossRef CAS.
- M. Rajani, N. Shrivastava and M. Ravishankara, Pharm. Biol., 2000, 38, 204 CrossRef CAS PubMed.
- C. Bothiraja, A. Pawar, V. Shende and P. Joshi, Comp. Clin. Pathol., 2013, 22, 1123 CrossRef CAS.
- A. Niranjan, S. Tewari and A. Lehri, Indian J. Nat. Prod. Resour., 2010, 1, 125 CAS.
- M. K. Gorter, Recl. Trav. Chim. Pays-Bas, 1911, 30, 151 CrossRef.
- R. Schwyzer, H. G. Biswas and P. Karrer, Helv. Chim. Acta, 1951, 34, 652 CrossRef CAS.
- R. J. C. Kleipool and D. G. F. R. Kostermans, Recl. Trav. Chim. Pays-Bas, 1951, 70, 1085 CrossRef CAS.
- R. J. C. Kleipool, Nature, 1952, 169, 33 CrossRef CAS.
- D. Chakravarti and R. N. Chakravarti, J. Chem. Soc., 1952, 1697 RSC.
- H. Kondo and A. Ohno, Annu. Rep. Itsuu Lab., 1958, 9, 38 CAS.
- M. P. Cava and B. Weinstein, Chem. Ind., 1959, 851 CAS.
- W. R. Chan, L. J. Haynes and L. F. Johnson, Chem. Ind., 1960, 22 CAS.
- M. P. Cava, W. R. Chan, L. J. Haynes and L. F. Johnson, Tetrahedron, 1962, 18, 397 CrossRef CAS.
- M. P. Cava, B. Weinstein, W. R. Chan, L. J. Haynes and L. F. Johnson, Chem. Ind., 1963, 167 CAS.
- A. Balmain and J. D. Connolly, J. Chem. Soc., Perkin Trans. 1, 1973, 1, 1247 RSC.
- M. P. Cava, W. R. Chan, R. P. Stein and C. R. Willis, Tetrahedron, 1965, 21, 2617 CrossRef CAS.
- A. B. Smith, B. H. Toder, P. Carrol and J. Donohue, J. Crystallogr. Spectrosc. Res., 1982, 12, 309 CrossRef CAS.
- K. Prajjal, S. Singha and S. Dey, Fitoterapia, 2003, 74, 692 CrossRef.
- J. Batkhun, K. Hattori and F. Takano, Biol. Pharm. Bull., 2002, 25, 1169 Search PubMed.
- C. Zhang and B. Tan, Clin. Exp. Pharmacol. Physiol., 1996, 23, 675 CrossRef CAS PubMed.
- M. Borhanuddin, M. Shamsuzzoha and A. Hussain, Bangladesh Med. Res. Counc. Bull., 1994, 20, 24 CAS.
- Y. Shen, C. Chen and W. Chioys, Br. J. Pharmacol., 2002, 135, 399 CrossRef CAS PubMed.
- D. Wang and H. Zhao, Chin. Med. J., 1994, 107, 464 CAS.
- C. Calabrese, S. Berman, J. Babish, X. Ma, L. Shinto, M. Dorr, K. Wells, C. Wenner and L. Standish, Phytother. Res., 2000, 14, 333 CrossRef CAS PubMed.
- E. Amroyan, E. Gabrielain and A. Panossian, Phytomedicine, 1999, 6, 27 CrossRef CAS PubMed.
- B. Choudhury and M. Poddar, Methods Find. Exp. Clin. Pharmacol., 1984, 6, 481 CAS.
- B. Shukla, P. Visen, G. Patnaik and B. Dhawan, Planta Med., 1992, 58, 146 CrossRef CAS PubMed.
- P. Rojanapanthu, W. Gritsanapan, M. Sirirat and C. Amornchat, US Pat., 7135164 B2, 2006 Nov 14.
- Z. Guo, H. Zhao and X. Zheng, J. Tongji Med. Univ., 1995, 15, 205 CrossRef CAS PubMed.
- V. Thamlikitkul, T. Dechatiwongse and S. Theerapong, J. Med. Assoc. Thailand, 1991, 74, 437 CAS.
- J. Coon and E. Ernst, Planta Med., 2004, 70, 293 CrossRef CAS PubMed.
- J. Melchior, Phytomedicine, 1996, 3, 315 CrossRef.
- D. Zhao, K. Liao, X. Ma and X. Yan, J. Inclusion Phenom., 2002, 43, 259 CrossRef CAS.
- Y. Peng, J. Li, Y. Sun, J. Chan, D. Sheng, K. Wang, P. Wei, P. Ouyang, D. Wang, S. Lee and G. Zhou, RSC Adv., 2015, 5, 22510 RSC.
- S. Nanduri, V. Nyavanandi, S. Rao, T. Kasu, M. Pallerla, P. Ram, S. Rajagopal, R. Kumar, R. Ramanujam, J. Babu, K. Vyas, A. Devi, G. Reddy and V. Akella, Bioorg. Med. Chem. Lett., 2004, 14, 4711 CrossRef CAS PubMed.
- B. Das, C. Chowdhury, D. Kumar, R. Sen, R. Roy, P. Das and M. Chatterje, Bioorg. Med. Chem. Lett., 2010, 20, 6947 CrossRef CAS PubMed.
- H. Chen, Y. Ma, X. Huang, C. Geng, Y. Zhao, L. Wang, R. Guo, W. Liang, X. Zhang and J. Chen, Bioorg. Med. Chem. Lett., 2014, 24, 2353 CrossRef CAS PubMed.
- Z. Liu, W. Law, D. Wang, X. Nie, D. Sheng, G. Song, K. Guo, P. Wei, P. Ouyang, C. Wong and G. Zhou, RSC Adv., 2014, 4, 13533 RSC.
- S. Handa and A. Sharma, Indian J. Med. Res., 1990, 92, 284 CAS.
- A. Rana and Y. Avadhoot, Arch. Pharmacal Res., 1991, 14, 93 CrossRef CAS.
- A. Kapil, L. Koul, S. Banerjee and B. Gupta, Biochem. Pharmacol., 1993, 46, 182 CrossRef CAS PubMed.
- P. Visen, B. Shukla, B. Pantnaik and B. Dhawan, J. Ethnopharmacol., 1993, 40, 131 CrossRef CAS PubMed.
- K. Maiti, A. Gantait, K. Mukherjee, B. Saha and P. Mukherjee, J. Nat. Rem., 2006, 6, 1 CAS.
- S. Rajagopal, A. kumar, S. Dhanvanthri, S. Chitkala and R. Rajagopalan, J. Exp. Ther. Oncol., 2003, 3, 147 CrossRef CAS PubMed.
- G. Tripathi and Y. Tripathi, Phytother. Res., 1991, 5, 176 CrossRef CAS.
- S. Gupta, J. Yadava and J. Tandon, Pharm. Biol., 1993, 31, 198 CrossRef.
- P. Mishra, N. Pal, P. Guru, J. Kativar and J. Tandon, Pharm. Biol., 1991, 29, 19 CrossRef.
- P. Roy, S. Das, T. Bera, S. Mondol and A. Mukherjee, Int. J. Nanomed., 2010, 5, 1113 CAS.
- A. Panossian, A. Hovhannisyan, G. Mamikonyan, H. Abrahamian, E. Hambardzumyan, E. Gabrielian, G. Goukasova, G. Wikman and H. Wagner, Phytomedicine, 2000, 7, 351 CrossRef CAS PubMed.
- X. He, J. Li, H. Gao, F. Qiu, X. Cui and X. Yao, Chem. Pharm. Bull., 2003a, 51, 586 Search PubMed.
- X. He, J. Li, H. Gao, F. Qiu, K. Hu, X. Cui and X. Yao, Drug Metab. Dispos., 2003b, 31, 983 Search PubMed.
- Z. M. Meng, Acta Pharmacol. Sin., 1981, 16, 571 CAS.
- L. Cui, F. Qiu, N. Wang and X. Yao, Chem. Pharm. Bull., 2004, 53, 772 CrossRef.
- L. Cui, F. Qiu and X. Yao, Drug Metab. Dispos., 2005, 33, 555 CrossRef CAS PubMed.
- H. Zhao, H. Hu and Y. Wang, Rapid Commun. Mass Spectrom., 2013, 27, 1 CrossRef CAS PubMed.
- Y. Wang, The pharmacology and application of traditional chinese medicine, People's Health Press, Beijing, 1st edn, 1983 Search PubMed.
- V. Thamlikitkul, T. Dechatiwongse, S. Theerapong, C. Chantrakul, P. Boonroj, W. Punkrut, W. Ekpalakorn, N. Boontaeng, S. Taechaiya and S. Petcharoen, J. Med. Assoc. Thailand, 1991, 74, 437 CAS.
- A. Panossian, A. Kochikian and E. Gabrielian, Phytomedicine, 1999, 6, 157 CrossRef CAS PubMed.
- M. Akbarsha and P. Murugaian, Phytother. Res., 2000, 14, 432 CrossRef CAS PubMed.
- J. Melchior, Phytomedicine, 1996, 3, 315 CrossRef.
- S. Pramanick, S. Banerjee, B. Achari, B. Das, A. Sen, S. Mukhopadhyay, A. Neuman and T. Prange, J. Nat. Prod., 2006, 69, 403 CrossRef CAS PubMed.
- Pharmacopoeia of India, Governament of India Press, Calcutta.cvvvv, 1995.
- V. S. Bhat and D. D. Nanavati, Indian Drugs, 1978, 3, 187 Search PubMed.
- W. Tang and G. Eisenbrand, Chinese drug of plant origin chemistry, Springer-Verlag, Berlin, 1992, p. 97 Search PubMed.
- S. Saxena, D. C. Jain, M. M Gupta, R. S. Bhakuni, H. O. Mishra and R. P. Sharma, Phytochem. Anal., 2000, 11, 34 CrossRef CAS.
- Y. Zhao, Y. Ming, H. Zhang, X. Luo, L. Chen and Y. Li, Chromatographia, 2005, 62, 611 CAS.
- Z. Yanfang, L. Xingping, Z. Zongde, C. Liren and L. Yongmin, J. Pharm. Biomed. Anal., 2006, 40, 157 CrossRef PubMed.
- N. Pholphana, N. Rangkadilok, S. Thongnest, S. Ruchirawat, M. Ruchirawat and J. Satyavivad, Phytochem. Anal., 2004, 15, 365 CrossRef CAS PubMed.
- Q. Du, G. Jerz and P. Wiinterhalter, J. Chromatogr. A, 2003, 984, 147 CrossRef CAS.
- D. C. Jain, M. M. Gupta, S. Saxene and S. Kumar, J. Pharm. Biomed. Anal., 2000, 22, 705 CrossRef CAS PubMed.
- A. G. Akowuah, I. Zhari, I. Norhayati and A. Mariam, J. Food Compos. Anal., 2006, 19, 118 CrossRef.
- B. Q. Wang, Z. G. Pang and C. Y. Wang, Huazue. Tongbo, 1994, 7, 50 Search PubMed.
- S. C. Wang, T. Y. Tseng, C. M. Huang and T. H. Tsai, J. Chromatogr. B: Anal. Technol. Biomed. Life Sci., 2004, 812, 193 CrossRef CAS.
- Y. Zhao, Y. Ming, H. Zhang, X. Luo, L. Chen and Y. Li, Chromatographia, 2005, 62, 611 CAS.
- D. Li, B. L Xu, T. Fei, B. Y. Jin, G. Manli and Z. Y. Shou, Talanta, 2008, 74, 1344 CrossRef PubMed.
- H. Y. Cheung, C. S. Cheung and C. K. Kong, J. Chromatogr. A, 2001, 28, 171 CrossRef.
- J. Zhao, G. Yang, H. Liu, D. Wang, X. Song and Y. Chen, Phytochem. Anal., 2002, 13, 222 CrossRef CAS PubMed.
- K. S. Kumaran, P. Thirugnanasambamtham, S. Viswanathan and M. Sree Ramamurthy, Indian J. Pharmacol., 2003, 35, 109 CAS.
- L. Chen, H. Jin, L. Ding, H. Zhang, X. Wang, Z. Wang, J. Li, C. Qu, Y. Wang and H. Zhang, J. Chromatogr. A, 2007, 1140, 71 CrossRef CAS PubMed.
- X. B. Suo, H. Zhang and Y. Q. Wang, Biomed. Chromatogr., 2007, 21, 730 CrossRef CAS PubMed.
- Y. Gua, M. Jinyu, L. Yali, C. Bo and Y. Shouzhuo, J. Chromatogr. B: Anal. Technol. Biomed. Life Sci., 2007, 854, 328 CrossRef PubMed.
- J. Wangboonskul, D. Supawadee, J. Kanokwan and S. Bungorn, Thai Pharmaceutical and Health Science Journal, 2006, 1, 209 Search PubMed.
- C. Bothiraja and A. Pawar, Eur. J. Drug Metab. Pharmacokinet., 2011, 35, 123 CrossRef PubMed.
- C. Bothiraja, A. Pawar, P. Munde and K. Shaikh, Nanosci. Nanotechnol. Lett., 2012, 4, 445 CrossRef CAS.
- A. Akbarzadeh, R. Rezaei-Sadabady, S. Davaran, S. Joo, N. Zarghami, Y. Hanifehpour, M. Samiei, M. Kouhi and K. Nejati-Koshki, Nanoscale Res. Lett., 2013, 8, 102 CrossRef PubMed.
- Y. L. Xiao and B. Li, Chin. Tradit. Herb. Drugs, 2002, 33, 385 CAS.
- T. Allen and P. Cullis, Adv. Drug Delivery Rev., 2013, 65, 36 CrossRef CAS PubMed.
- J. Sinha, S. Mukhopadhyay, N. Das and M. Basu, Drug Delivery, 2000, 7, 209 CrossRef CAS PubMed.
- P. Jain, N. Khurana, Y. Pounikar, A. Gajbhiye and M. Kharya, J. Liposome Res., 2013, 23, 110 CrossRef CAS PubMed.
- K. Kazi, A. Mandal, N. Biswas, A. Guha, S. Chatterjee, M. Behera and K. Kuotsu, J. Adv. Pharm. Technol. Res., 2010, 4, 374 Search PubMed.
- A. Sankhyan and P. Pawar, Appl. Pharm. Sci., 2012, 2, 20 Search PubMed.
- Y. Tu, D. Sun, J. Zhang, Z. Jiang, Y. Chen, X. Zeng, D. Huang and N. Yao, J. Microencapsulation, 2014, 31, 307 CrossRef CAS PubMed.
- E. Allemann, R. Gurny and E. Doelker, Eur. J. Pharm. Biopharm., 1993, 39, 173 CAS.
- P. Couvreur, C. Dubernet and F. Puisieux, Eur. J. Pharm. Biopharm., 1995, 41, 2 CAS.
- I. Brigger, C. Dubernet and P. Couvreur, Adv. Drug Delivery Rev., 2002, 54, 631 CrossRef CAS PubMed.
- F. Alexis, P. Basto, E. Levy-Nissenbaum, A. Radovic-Moreno, L. Zhang, E. Pridgen, A. Wang, S. Marein, K. Westerhof, L. Molnar and O. Farokhzad, ChemMedChem, 2008, 3, 1839 CrossRef CAS PubMed.
- T. Yang, H. Sheng, N. Feng, H. Wei, Z. Wang and C. Wang, J. Pharm. Sci., 2013, 12, 4414 CrossRef PubMed.
- R. Parveen, F. Ahmad, Z. Iqbal and S. Ahmad, Planta Med., 2013, 79, 79 Search PubMed.
- M. Diaz and P. Vivas-Mejia, Pharmaceuticals, 2013, 6, 1361 CrossRef PubMed.
- C. Bothiraja and A. Pawar, Int. J. Nanotechnol., 2011, 8, 764 CrossRef.
- J. Zhang, Y. Li, W. Gao, M. Repka, Y. Wang and M. Chen, Expert Opin. Drug Delivery, 2014, 11, 1367 CrossRef CAS PubMed.
- P. Roy, S. Das, A. Mondal, U. Chatterji and A. Mukherjee, Curr. Pharm. Biotechnol., 2012, 13, 2669 CAS.
- P. Roy, S. Das, R. Auddy, A. Saha and A. Mukherjee, J. Pharma Res., 2013, 30, 1252 CrossRef CAS PubMed.
- S. Mondal, P. Roy, S. Das, A. Halder, A. Mukherjee and T. Bera, PLoS One, 2013, 8, e81492 Search PubMed.
- S. Das, G. K. Pradhan, S. Das, D. Nath and K. Das Saha, Chem.-Biol. Interact., 2015, 242, 281 CrossRef CAS PubMed.
- J. Zhang, M. Zhang, J. Ji, X. Fang, X. Pan, Y. Wang, C. Wu and M. Chen, Pharm. Res., 2015, 32, 3376 CrossRef CAS PubMed.
- P. Roy, S. Das, T. Bera, S. Mondol and A. Mukherjee, Int. J. Nanomed., 2010, 5, 1113 CAS.
- I. Chandiran, B. Kumar and K. Jayaveera, Drug Invent. Today, 2013, 5, 235 CrossRef CAS.
- P. Davoodi, F. Feng, Q. Xu, W. Yan, Y. Tong, M. Srinivasan, V. Sharma and C. Wang, J. Controlled Release, 2015, 205, 70 CrossRef CAS PubMed.
- Y. Jiang, F. Wang, H. Xu, H. Liu, Q. Meng and W. Liu, Int. J. Pharm., 2014, 20, 475 CrossRef PubMed.
- A. Nasir, S. Harikumar and A. Kaur, Int. Res. J. Pharm., 2012, 3, 44 Search PubMed.
- K. Uekama, F. Hirayama and T. Irie, Drug Targeting Delivery, 1994, 3, 411 CAS.
- G. Tiwari, R. Tiwari and A. Rai, J. Pharm. BioAllied Sci., 2010, 2, 72 CrossRef CAS PubMed.
- A. Rasheed, A. Kumar and V. Sravanthi, Sci. Pharm., 2008, 76, 567 CrossRef CAS.
- K. Ren, Z. Zhang, Y. Li, J. Liu, D. Zhao, Y. Zhao and T. Gong, Pharmazie, 2009, 64, 515 CAS.
- H. Zhou, W. Lai, Z. Zhang, W. Li and H. Cheung, J. Comput.-Aided Mol. Des., 2009, 23, 153 CrossRef CAS PubMed.
- B. Q. Li and Y. Z. Ding, China Papers, 2010, 8, 1 Search PubMed.
- W. P. Lai, 2009, http://lib.cityu.edu.hk/record=b2367225.
- M. Lu, Y. Cheng, L. Li and J. Wu, Mater. Rev., 2005, 19, 108 Search PubMed.
- H. Du, X. Yang, H. Li, L. Han, X. Li, X. Dong, Q. Zhu, M. Ye, Q. Feng and X. Niu, J. Microencapsulation, 2012, 29, 657 CrossRef CAS PubMed.
- Z. Z. Yu and D. Z. Ying, China Papers, 2010, 4, 1 Search PubMed.
- M. Ibrahim and G. Shazly, Acta Pol. Pharm., 2014, 5, 821 Search PubMed.
- M. Marvola, P. Nykanen, S. Rautio, N. Isonen and A. Autere, Eur. J. Pharm. Sci., 1999, 7, 259 CrossRef CAS PubMed.
- S. Arshia, P. Manna, K. Paranjothy and M. Manjula, Pak. J. Pharm. Sci., 2007, 20, 1 Search PubMed.
- J. Kumar, S. Muralidharan and S. Dhanaraj, J. Pharm. Pharm. Sci., 2013, 2, 1 Search PubMed.
- C. Bothiraja, V. Kumbhar, A. Pawar, K. Shaikh and R. Kamble, RSC Adv., 2015, 5, 28848 RSC.
- S. Chakraborty, S. Biswas, B. Sa, S. Das and R. Dey, Colloids Surf., A, 2014, 455, 111 CrossRef CAS.
- Y. Huang and W. Dai, Acta Pharm. Sin. B, 2014, 4, 18 CrossRef PubMed.
- S. Chan, Y. Chung, X. Cheah, E. Tan and J. Quah, Asian J. Pharm. Sci., 2015, 10, 372 CrossRef.
- R. Kamble, P. Palve and P. Mehta, J. Adv. Pharm. Educ. Res., 2014, 4, 65 Search PubMed.
- Z. Tong and W. Kang, China Papers, 2011, 2, 1 Search PubMed.
- S. Hu, Z. Zhang and X. Jia, China J. Chin. Mater. Med., 2013, 38, 341 CAS.
- C. Y. Shan, W. Chen and Y. J. Wang, J. Xinjiang Med. Univ., 2011, 1, 1 CrossRef.
- L. I. Jun and W. Cheng, Natural Product R&D, 2033, 4, 1 Search PubMed.
- A. Thingale, K. Shaikh, P. Channekar, U. Galgatte, P. Chaudhari and C. Bothiraja, Drug Delivery, 2015, 22, 117 CrossRef CAS PubMed.
- K. Senthil, P. Thriuganasambantham, S. Viswanathan and S. Muthy, Indian Drugs, 2002, 39, 648 Search PubMed.
- H. Du, X. Niu, F. Q. Jin and L. M. Ye, Tradit. Chin. Drug Res. Clin. Pharmacol., 2007, 18, 217 CAS.
- X. P. Wang, L. Han, G. L. Ren, D. K. Zhang, Z. F. Wu, M. Yang and Z. P. Guo, Zhongguo Zhongyao Zazhi, 2014, 39, 657 CAS.
- G. R. Desiraju, J. J. Vittal and A. Ramanan, Crystal Engineering. A Textbook, World Scientific Publishing, Singapore, 2011 Search PubMed.
- T. Kawasaki, K. Jo, H. Igarashi, I. Sato, M. Nagano, H. Koshima and K. Soai, Angew. Chem., 2005, 44, 2774 CrossRef CAS PubMed.
- N. R. Goud, S. Gangavaram, K. Suresh, S. Pal, S. G. Manjunatha, S. Nambiar and A. Nangia, J. Pharm. Sci., 2012, 101, 664 CrossRef CAS PubMed.
- M. R. Cheney, N. Shan, E. R. Healey, M. Hanna, L. Wojtas, M. J. Zaworotko, V. Sava, S. Song and J. R. Sanchez-Ramos, Cryst. Growth Des., 2010, 10, 394 CAS.
- P. M. Bag, M. Chen, C. Calvin and C. M. Reddy, CrystEngComm, 2012, 14, 3865 RSC.
- N. J. Babu, P. Sanphui and A. Nangia, Chem.–Asian J., 2012, 7, 2274 CrossRef CAS PubMed.
- S. F. Chow, M. Chen, L. Shi, A. H. L. Chow and C. C. Sun, Pharm. Res., 2012, 29, 1854 CrossRef CAS PubMed.
- The Generally Regarded as Safe Chemicals list may be accessed at: http://www.accessdata.fda.gov/scripts/fcn/fcnNavigation.cfm?rpt=scogsListing%26displayAll=true.
- K. Suresh, N. R. Goud and A. Nangia, Chem.–Asian J., 2013, 12, 3032 CrossRef PubMed.
- S. Nanduri, S. Rajagopal, S. Pothukuchi, S. Pillai and R. Chakrabarti, US Pat., 6, 410, 590 B1, 2002.
- S. Asami, K. Saito, A. Maeda and N. Tateishi, US Pat., 8,507, 547 B2, 2013.
- J. Jiang, X. Jiang, Y. Wang, Z. Yang, P. Yu and Z. Zhang, WO 2009018780 A1, 2009.
- P. K. Mukherjee, Indian Patent, App No: 00056/KOL/2006 A, 2006.
|
This journal is © The Royal Society of Chemistry 2016 |
Click here to see how this site uses Cookies. View our privacy policy here.