DOI:
10.1039/C6RA10975F
(Paper)
RSC Adv., 2016,
6, 61418-61422
The use of ionic liquid as a mobile phase modifier in analytical supercritical fluid chromatography for the separation of flavonoids†
Received
28th April 2016
, Accepted 15th June 2016
First published on 16th June 2016
Abstract
Due to the low viscosity of carbon dioxide, supercritical-fluid chromatography (SFC) allows higher flow rates with lower back pressure. Generally, analytical SFC could provide much faster and more efficient separation compared to conventional chromatographic methods. However, the non-polar characteristic of supercritical carbon dioxide (scCO2) has limited the applicability of SFC in the separation of hydrophobic compounds. Ionic liquids (ILs), with attractive features including a wide range of solubility, miscibility, and low vapor pressure, may offer a possibility to improve SFC separation. In this work, an analytical SFC method using IL as mobile phase modifier was developed for the separation of six flavonoid aglycones, and 1-butyl-3-methyl imidazolium-based ILs were tested and compared as mobile phase additives. The results demonstrated the addition of ILs into SFC mobile phase could significantly improve the resolution of flavonoid aglycones, and the selectivity changed probably based on the hydrogen-bonding interaction.
1. Introduction
In recent years, supercritical fluid chromatography (SFC) has become recognized as one of the favourite and most powerful chromatographic techniques in many fields, such as pharmaceutical analysis and chiral separation.1,2 Compared to both liquid chromatography (LC) and gas chromatography (GC), SFC, which utilizes supercritical fluids as the mobile phase, exhibits special retention behaviours and selectivity of analytes.3–5 The potential benefits of analytical SFC has gradually attracted the widespread attention of analysts.
In SFC analysis, the mobile phase primarily consists of supercritical carbon dioxide (scCO2). The low viscosity and high solute diffusivity of carbon dioxide under supercritical conditions could result in superior separation.6,7 However, the non-polar characteristic of carbon dioxide has limited its elution range and ability, and many analytes could not be effectively eluted. Co-solvents, also named modifiers, were thus added to alter the mobile phase polarity through hydrogen bonds or dipole–dipole interactions.8 Some organic solvents such as short-chain alcohols, acetonitrile and chloroform are often used.
Ionic liquids (ILs) are a new class of organic salts consisting of organic cations and inorganic anions in a liquid state at room temperature. Due to their unique properties, including miscibility, wide liquid regions and favourable solvating properties for a range of polar and non-polar compounds, ILs have widespread applications in various analytical fields,9 especially being used as stationary phases in HPLC,10 GC,11 SFC,12 and using as mobile phase modifiers in HPLC.13 Recently, tuneable solvent systems composed of ILs and supercritical carbon dioxide have attracted increasing attention, since ILs could effectively modify the partitioning behaviour of organic compounds in supercritical fluids,14,15 and even provide favourable functionalities due to molecular interactions. Though ILs themselves are nearly insoluble in scCO2, the solubility of imidazolium-based ILs in scCO2 could be significantly enhanced by organic co-solvents.16 This could offer the potential of ILs to be used in analytical SFC.
In this study, the possibility of ILs as mobile phase modifiers in analytical SFC for the separation of six flavonoid aglycones with similar structures was investigated. To obtain the optimal separation, the analytical parameters including IL type, IL concentration, temperature and back pressure were optimized. The results showed that the addition of ILs into the mobile phase in SFC could significantly affect the peak shapes, and the retention behaviours of six analytes are close to the normal-phase model by using an EC-CN column. Collectively, our work has provided new application directions and developable concepts in using ILs in analytical fields.
2. Experimental
2.1 Chemicals and reagents
The following six flavonoid aglycones were used as probe compounds: acacetin (1), calycosin (2), diosmetin (3), apigenin (4), genistein (5) and luteolin (6) with purity above 96%, which were obtained from the National Institute for the Control of Pharmaceutical and Biological Product. Their structures are shown in Fig. 1. Mixed standard solutions were prepared as follows: accurately weigh 2.0 mg of the reference substances and that were dissolved in 100 μL of dimethylsulfoxide (DMSO), then dilute with methanol.
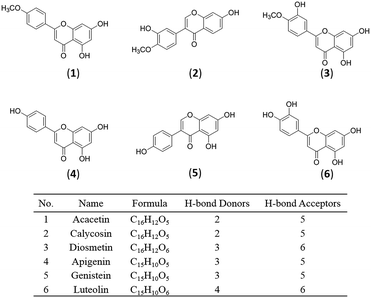 |
| Fig. 1 Chemical structures of the investigated flavonoid aglycones. | |
Five ionic liquids named 1-butyl-3-methylimidazolium tetrafluoroborate ([bmim][BF4]), 1-butyl-3-methylimidazolium hexafluorophosphate ([bmim][PF6]), 1-butyl-3-methylimidazolium nitrate ([bmim][NO3]), 1-butyl-3-methylimidazolium bis(trifluoromethylsulfonyl)imide ([bmim][NTF2]), and 1-butyl-3-methylimidazolium trifluoromethanesulfonate ([bmim][OTF]) were purchased from ChengJie Chemical Co. LTD. (Shanghai, China) and their purities were higher than 97%. Carbon dioxide (CO2) used was of high purity (99.999%). Methanol (HPLC grade) was obtained from Merck (Darmstadt, Germany).
2.2 Analytical supercritical-fluid chromatography parameters
An Agilent 1260 LC system, including a vacuum degasser, a binary pump, an auto-sampler with injection loop, a column compartment and diode array detector, with an Aurora A5 FusionTM SFC module was controlled by Agilent Openla CDS ChemStation (Agilent Technologies, CA, USA).
The chromatographic separation was performed on a Poroshell 120 EC-CN (3.0 × 100 mm, 2.7 μm) (Agilent Technologies, USA) with the flow rate at 2 mL min−1 and column temperature at 45 °C. The mobile phase consisted of (A) methanol with 20 mM [bmim][BF4] and (B) scCO2. The back pressure regulation (BPR) was set at 110 bar, and detection wavelength was 260 nm.
2.3 Chromatographic parameter calculation
The retention factor k′ in this study was calculated using the formula: k′ = (tR − t0)/t0, where tR is the solute retention time and t0 is the column dead time which was measured by the solvent peak. The resolution factor (Rs) was calculated from the software (Agilent ChemStation) and referred to the half-height method: Rs = 2(t2 − t1)/1.7(w0.5,1 − w0.5,2), where t1 and t2 are the retention times of the two peaks of interest, and w0.5,1 and w0.5,2 are the peak widths measured at half height. The number of plate counts (N) for each peak were also obtained from the software calculations.
3. Results and discussion
3.1 Selection of detection wavelength
The presence of ILs in the mobile phase could cause somewhat noticeable changes in the background signal when using a low detection wavelength, because ILs generally exhibit remarkable UV absorbance in the region between 200 and 240 nm. The entirely ultraviolet absorption of ILs used in present study was all less than 240 nm (see ESI, Fig. S1†), consistent with the previous reports.17 Since the maximal absorbances of flavonoids were at 260 nm and/or in the range between 330 and 360 nm (see ESI, Fig. S2†), the detection of flavonoids is not affected by using ILs as a co-solvent. To obtain the optimal UV response signals, the detection wavelength was selected to be 260 nm.
3.2 Selection of columns
SFC can operate as a normal-phase separation mode with polar stationary phases, or as a reversed-phase mode using non-polar bonded phases. These rules are verified for most carbon dioxide-based mobile phases in common use (CO2/methanol, CO2/acetonitrile or CO2/ethanol).5 In order to get a reference chromatographic behaviour of those flavonoids in SFC analysis, three common chromatographic column, i.e., Poroshell 120 EC-C18 (3.0 × 100 mm, 2.7 μm), Poroshell Phenyl Hexyl (3.0 × 100 mm, 2.7 μm), Poroshell EC-CN (3.0 × 100 mm, 2.7 μm) were tested with respect to the peak shape and resolution using methanol as an organic modifier. The stationary phases of the three columns are characterized as alkyl bonded phases, dual alkyl phases and polar phases, respectively. As Fig. 2 shows, the six flavonoid aglycones could not be separated on the EC-C18 and Phenyl Hexyl columns, but showed relative symmetric peak shapes on the Poroshell EC-CN column. This is probably because flavonoid aglycones have non-hydrophilic properties with few hydroxyl groups, which were well dissolved in the non-polar scCO2 and methanol. The difference indicated that the retention behaviours of flavonoid aglycones in SFC are close to the normal-phase model. So the Poroshell EC-CN column was selected to conduct the further experiments. While analysing on the EC-CN columns, the polarity range of the six analytes limited the content of methanol to between 5 and 20%.
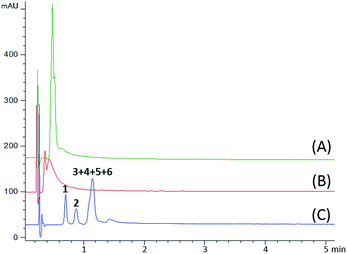 |
| Fig. 2 Chromatograms of 6 analytes obtained using a methanol modifier on various columns. (A) Poroshell 120 EC-C18 (3.0 × 100 mm, 2.7 μm) with 3% methanol; (B) Poroshell Phenyl Hexyl (3.0 × 100 mm, 2.7 μm) with 8% methanol; (C) Poroshell EC-CN (3.0 × 100 mm, 2.7 μm) with 8% methanol; flow-rates were kept constant at 2 mL min−1. | |
3.3 Effect of IL types and concentrations on flavonoid aglycone separation in SFC analysis
The six flavonoid aglycones with similar structures, namely acacetin (1), calycosin (2), diosmetin (3), apigenin (4), genistein (5) and luteolin (6), were not well separated using the common mobile phase system consisting of scCO2 and methanol. Obviously, compounds 3–6 were co-eluted in the absence of ILs (Fig. 2C). Five commercially available ILs, [bmim][NO3], [bmim][PF6], [bmim][BF4], [bmim][OTF] and [bmim][NTF2], with the same alkyl chain but different anions were thus tested as modifiers in the mobile phase. Noticeably, the addition of ILs, especially for [bmim][BF4], could change the retention behaviours of these flavonoids (see ESI, Fig. S3†). This might be a result from the hydrogen-bonding interaction between the anions of ILs and the hydroxyl group on the flavonoids.18–20
To achieve the optimum separation, the [bmim][BF4] concentration was evaluated in a range from 5 to 25 mM in the mobile phase. The ratio of co-solvent was set at 8% (92% scCO2), and the other analysis conditions were kept constant (temperature of 45 °C and flow rate of 2 mL min−1). In preliminary experiments (without IL additives), a poor separation efficiency of four analytes were observed (Fig. 3A). The addition of [bmim][BF4] at a low concentrations (5 mM, 10 mM) improved the resolution to some extent, but compounds 3, 4 and 5 still co-eluted with each other (Fig. 3B and C). When the IL concentration was increased to 15 mM, compound 3 achieved baseline separation from compound 4 (Fig. 3D). According to Fig. 3E, the introduction of [bmim][BF4] at a concentration of 20 mM was sufficient to separate the six tested analytes with good resolution. Increasing the [bmim][BF4] concentration to 25 mM significantly improved the separation of compounds 3, 4 and 5, but decreased the resolutions of compounds 2 and 3 (Fig. 3F). The resolutions (Rs) between compounds 2, 3, 4, 5 and 6 are shown as Fig. 4A. Obviously, the resolutions, except for compound 2 and 3, increased with the increase of the IL concentration.
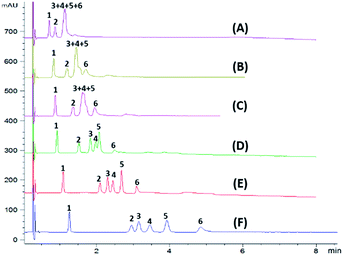 |
| Fig. 3 Effect of [bmim][BF4] concentration in methanol on the separation. (A) Without [bmim][BF4]; (B) 5 mM; (C) 10 mM; (D) 15 mM; (E) 20 mM; (F) 25 mM. | |
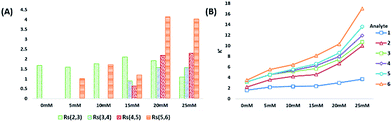 |
| Fig. 4 Plots of the (A) resolution (Rs) and (B) retention factor (k′) with respect to the concentration of [bmim][BF4] used as an additive for the separation of 6 analytes. | |
In short, with the IL concentration being increased, the interactions between the flavonoid aglycones and column were improved. The retention factors (k′) of the analytes are also displayed to further illustrate the effects of the IL concentration. As Fig. 4B shows, the retention factors increased upon increasing the IL concentrations. Moreover, the more hydroxyl groups the compounds have, the stronger the retention behaviours became. When the [bmim][BF4] concentration increased to 25 mM, the retention factors k′ of the compounds 3, 4, 5 and 6 were all greater than 10, which were consistent with the poor peak shape. It is noticed that the peak width of compound 6 was significantly increased with the [bmim][BF4] concentration increase from 15 to 25 mM. Considering compound 6 has the most hydroxy groups, the result indicated that the ILs probably interact with the phenolic hydroxyl of flavonoids.
Moreover, the elution order of six flavonoid aglycones were consistent with their acidity which is ranked as 1 < 2 < 3 < 4 ≈ 5 < 6, which are more regular than the separation by reversed-phase mode in the previous report.21 The acidity of flavonoid aglycones are dependent on the number and position of hydroxyl groups they have. Thus, the chromatographic retention behaviour of flavonoid aglycones in SFC with ILs as additives could be predicted based on their number and position of hydroxyl groups.
In summary, the undoubted changes in the partition of flavonoid aglycones between the mobile phase and the stationary phase of column were observed by adding ILs. It was speculated that there is a new hydrogen-bonding interaction between the analytes because of the hydrogen-bond acidity of flavonoid aglycones and the hydrogen-bond basicity of ILs.
3.4 Effect of other parameters
Analyte separation in SFC is dependent on the partition between the mobile phase and stationary phase. As is known, the temperature and pressure mainly act on the mobile phase density for super/subcritical CO2, and the effects of the retention mechanism were mainly in accordance with the density changes.
3.4.1 Effect of back pressure on separation. Back pressure regulation (BPR) is a uniquely strong influence on SFC separation. In general, the eluent strength of super/subcritical solvents was enhanced by higher pressure that reduces the retention time of analytes.22 The effect of the BPR on the separation was tested at a column temperature of 45 °C, in the presence of 8% MeOH (with 20 mM [bmim][BF4]) as a co-solvent with flow rate at 2 mL min−1. The retention times of all peaks shortened as the pressure increased. For the relative strongly retained analytes, such as compound 6, the elute time was shortened significantly (Fig. 5A). Moreover, the back pressure could change the selectivity of separation. For instance, compounds 4 and 5 could not be baseline separated when the BPR was 120 bar or higher. It can also be seen that the plate numbers of the analytes almost all increased when the pressure increased from 100 bar to 110 bar but decreased when the pressure sequentially increased to 150 bar according to the retention time change (Fig. 5B).
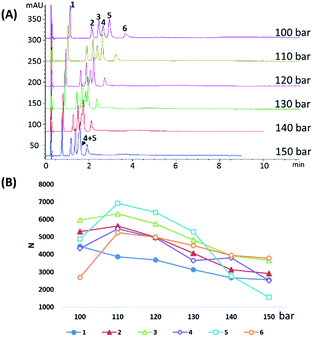 |
| Fig. 5 Effects of BPR pressure on the 6 analyte separation in SFC. (A) Chromatography; (B) plates. | |
3.4.2 Effect of temperature on separation. In addition to affecting the density of the mobile phase, a change in temperature also influences the retention times of analytes. Therefore, optimizing the operation temperature is an essential aspect of improving the separation. We investigated the effect of the temperature – varied between 25 °C and 50 °C – on the retention factor of the analytes, and BPR was kept constant at 110 bar. The results are shown in Fig. 6. Density generally decreases upon increasing the temperature, therefore, the retentions all increased upon increasing the temperature. It also seen that compounds 3, 4 and 5 could be separated by baseline when the temperature reaches 45 °C. However, when the temperature was raised to 50 °C, the tailing peaks appeared which resulted in the number of plates decreasing. In summary, the plate counts of most analytes were the highest at 45 °C (Fig. 6B).
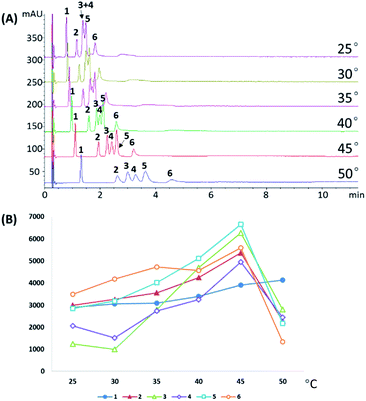 |
| Fig. 6 Effects of temperature on the 6 analyte separation in SFC. (A) Chromatography; (B) plates. | |
3.5 Method validation
Nine concentration levels of working solution were prepared by diluting the mixed standard solutions with methanol, then 3 μL of these were injected in triplicate to evaluate the linearity of analytical method. By plotting the peak areas of each analyte versus its concentration, the calibration curves were obtained and are listed in Table 1. All the calibration curves showed good linearity within r2 ≥ 0.9944 under the test range. Then the sensitivity was tested. The concentrations of the individual analytes determined at signal-to-noise ratios (S/N) of 3 and 10 were defined as the limit of detections (LODs) and limit of quantifications (LOQs), respectively. The peak area and S/N of the target analytes were calculated at maximum UV absorption wavelengths, i.e., 280 nm for compounds 1, 2 and 5, 330 nm for compounds 3 and 4, and 360 nm for compounds 6 and 7. The LOD of compound 6 was about 18 μg mL−1, and the others were in the range of 1.9–5.4 μg mL−1. The result indicated that SFC can provide comparable sensitivity with common HPLC methods.
Table 1 Calibration curves, LODs and LOQs of 6 analytes
Analyte |
Calibration curve |
r2 |
Test range (μg mL−1) |
LOQs (μg mL−1) |
LODs (μg mL−1) |
1 |
y = 3.9701x − 12.503 |
0.9976 |
1.95–500 |
4.0 |
1.9 |
2 |
y = 2.5242x − 9.0517 |
0.9992 |
10.94–1400 |
10.9 |
5.4 |
3 |
y = 2.3601x − 7.6791 |
0.9998 |
7.03–900 |
7.0 |
3.5 |
4 |
y = 4.3728x − 10.578 |
0.9999 |
5.47–700 |
5.4 |
2.7 |
5 |
y = 2.7685x − 15.205 |
0.9997 |
9.38–1200 |
9.3 |
4.6 |
6 |
y = 2.9793x − 6.1773 |
0.9944 |
34.3–1100 |
34.3 |
17.1 |
4. Conclusions
In this work, the performance of ILs as additives in SFC based on a normal-phase separation mode was evaluated in flavonoid analysis. The result showed that the addition of ILs could extremely improve the resolution of flavonoid algycones. Among the different ILs, [bmim][BF4] showed best performance with a concentration of 20 mM. The eluted order of the six flavonoid aglycones was mainly depended on the number and the position of hydroxyl groups, and the selectivity was probably according to the hydrogen-bonding interaction between the IL and the hydroxyl group on the flavonoids. ILs as an environment friendly additive can be used to improve and enrich the application of SFC in multiple compound analysis.
Acknowledgements
The authors thank Agilent Technologies (Shanghai, China) for loaning the SFC throughout this study. This work was financially supported by the Natural Science Foundation of Jiangsu Province (No. BK20140673), the National Natural Science Foundation of China (No. 81322051), the Qing Lan Project of Jiangsu Province and the Project Funded by the Priority Academic Program Development of Jiangsu Higher Education Institutions (PAPD).
References
- R. Q. Wang, T. T. Ong, W. Tang and S. C. Ng, TrAC, Trends Anal. Chem., 2012, 37, 83–100 CrossRef CAS.
- K. Kalíková, T. Šlechtová, J. Vozka and E. Tesařová, Anal. Chim. Acta, 2014, 821, 1–33 CrossRef PubMed.
- D. Spaggiari, F. Mehl, V. Desfontaine, A. Grand-Guillaume Perrenoud, S. Fekete, S. Rudaz and D. Guillarme, J. Chromatogr. A, 2014, 1371, 244–256 CrossRef CAS PubMed.
- A. J. Alexander, L. Zhang and T. F. Hooker, J. Pharm. Biomed. Anal., 2013, 78–79, 243–251 CrossRef CAS PubMed.
- E. Lesellier, Retention mechanisms in super/subcritical fluidchromatography on packed columns, J. Chromatogr. A, 2009, 1216, 1881–1890 CrossRef CAS PubMed.
- J. D. Pinkston, D. Wen, K. L. Morand, D. A. Tirey and D. T. Stanton, Anal. Chem., 2006, 78, 7467–7472 CrossRef CAS PubMed.
- L. Nováková, A. G.-G. Perrenoud, I. Francois, C. West, E. Lesellier and D. Guillarme, Anal. Chim. Acta, 2014, 824, 18–35 CrossRef PubMed.
- T. A. Berger and J. F. Deye, Anal. Chem., 1990, 62, 1181 CrossRef CAS.
- S. Pandey, Anal. Chim. Acta, 2006, 556, 38–45 CrossRef CAS PubMed.
- X. Z. Shi, L. Z. Qiao and G. W. Xu, J. Chromatogr. A, 2015, 1420, 1–15 CrossRef CAS PubMed.
- C. F. Poole and N. Lenca, J. Chromatogr. A, 2014, 1357, 87–109 CrossRef CAS PubMed.
- F. M. Chou, W. T. Wang and G. T. Wei, J. Chromatogr. A, 2009, 1216, 3594–3599 CrossRef CAS PubMed.
- M. C. García-Alvarez-Coque, M. J. Ruiz-Angel, A. Berthod and S. Carda-Broch, Anal. Chim. Acta, 2015, 883, 1–21 CrossRef PubMed.
- J. Planeta, P. Karásek, B. Hohnová, L. Št’avíková and M. Roth, J. Chromatogr. A, 2012, 1250, 54–62 CrossRef CAS PubMed.
- M. Roth, J. Chromatogr. A, 2009, 1216, 1861–1880 CrossRef CAS PubMed.
- W. Wu, W. Li, B. Han, T. Jiang, D. Shen, Z. Zhang, D. Sun and B. Wang, J. Chem. Eng. Data, 2004, 49, 1597–1601 CrossRef CAS.
- J. Cao, H. B. Qu and Y. Y. Cheng, Electrophoresis, 2010, 31, 3492–3498 CrossRef CAS PubMed.
- M. G. Freire, P. J. Carvalho, A. M. S. Silva, L. M. N. B. F. Santos, L. P. N. Rebelo, I. M. Marrucho and J. A. P. Coutinho, J. Phys. Chem. B, 2009, 113, 202–211 CrossRef CAS PubMed.
- Y. F. Cao, H. B. Xing, Q. W. Yang, Z. B. Bao, B. G. Su, Y. W. Yang and Q. L. Ren, J. Agric. Food Chem., 2012, 60, 3432–3440 CrossRef CAS PubMed.
- J. A. Blackwell and R. W. Stringham, Anal. Chem., 1997, 69, 409–415 CrossRef CAS PubMed.
- M. Ganzera, J. Pharm. Biomed. Anal., 2015, 107, 364–369 CrossRef CAS PubMed.
- X. W. Lou, H. G. Janssen and C. A. Cramers, J. Chromatogr. A, 1997, 785, 57 CrossRef CAS.
Footnote |
† Electronic supplementary information (ESI) available. See DOI: 10.1039/c6ra10975f |
|
This journal is © The Royal Society of Chemistry 2016 |
Click here to see how this site uses Cookies. View our privacy policy here.