DOI:
10.1039/C6RA08026J
(Paper)
RSC Adv., 2016,
6, 56638-56646
Ni–S-methylisothiourea complexes supported on boehmite nanoparticles and their application in the synthesis of 5-substituted tetrazoles
Received
28th March 2016
, Accepted 7th June 2016
First published on 8th June 2016
Abstract
Boehmite nanoparticles are aluminum oxide hydroxide (γ-AlOOH) particles, which were prepared by a simple and inexpensive procedure and immobilized further with nickel–S-methylisothiourea complexes (Ni–SMTU@boehmite), and characterized by FT-IR spectroscopy, TGA, XRD, ICP-OES, EDS, SEM and TEM techniques. Finally, we investigated the catalytic activity of Ni–SMTU@boehmite as a new, heterogeneous, stable and highly reusable nanocatalyst for the synthesis of 5-substituted 1H-tetrazole derivatives in PEG-400 as a green solvent. This catalyst was recovered by simple filtration and reused several times without significant loss of its catalytic efficiency or nickel leaching. The heterogeneity of this catalyst has been studied using hot filtration and ICP-OES technique.
1 Introduction
Tetrazoles are an interesting class of nitrogen-rich heterocycles, and also have wide range of applications as drugs in pharmaceuticals, in coordination chemistry as ligands, in synthetic organic chemistry, in catalysis technology, in medicinal chemistry as stable surrogates for carboxylic acids, in the photographic industry, in organometallic chemistry as effective stabilizers of metallopeptide structures and in various materials science applications.1–6 Recently tetrazole moieties were widely used for binding aryl thiotetrazolylacetanilides with HIV-1 reverse transcriptase.7 Also, tetrazoles and their derivatives have been reported as analgesic, antiviral, anti-inflammatory, anti-proliferative, antibacterial, potential anti HIV drug candidates, antifungal, herbicidal and anticancer agents.8–12 Valsartan and losartan are two typical examples of the extensive application of these compounds as drugs, such as anti-hypertensive sartan family drugs.13 Conventional synthesis of 5-substituted 1H-tetrazoles is [3 + 2] cycloaddition of azide to the corresponding nitriles. Recently, many catalysts have attracted increasing interest in the context for synthesis of tetrazole derivatives.1–12 In order to combine the advantages of both homogeneous and heterogeneous catalysis, immobilization of homogeneous catalysts on heterogeneous nanomaterials have been widely used as efficient and reusable catalysts for synthesis of tetrazole derivatives.14 However, many supports such as zeolite,3,12 MCM-41,15 iron oxide,16 carbon nanotubes,17 graphene,18 heteropolyacids,19 ionic liquids20 or some polymers21 have been used as catalyst supports, which most of this supports are expensive or unstable. Also, synthesis of other nanoparticles requires inert atmosphere or high temperature for calcination or preparation.22–25 While, boehmite nanoparticles is not air or moisture sensitive, more importance boehmite nanoparticles can be prepared in water at room temperature using available materials such as NaOH and Al(NO3)3·9H2O.25 Boehmite is an aluminum oxide hydroxide (γ-AlOOH) particles including double sheets of octahedral with aluminum ions at their centers and the sheets themselves are composed of octahedral chains in cubic orthorhombic structure. The surface of boehmite nanoparticles are covered by many hydroxyl groups, which makes possible the modification of the surface of boehmite nanoparticles with other functional groups to supported catalysts such as metal complexes. Boehmite nanoparticles have several advantages such as non-toxicity, stability, high specific surface area (>120 m2 g−1), ease of surface modification due to many hydroxyl groups on its surface, inexpensive and easily and readily availability.26–28 Several methods such as sol–gel,29 hydrothermal30 and hydrolysis of aluminum31 have been reported for preparation of boehmite nanoparticles; but, most of them have focused on the effect of temperature, ultrasonic waves, time and Al/OH molar ratio on morphologies, chemical and physical properties of the prepared particles.33 Also, boehmite has been used as an optical material, absorbent, composite reinforcement material in ceramics, vaccine adjuvants, cosmetic products, coatings, starting material in the synthesis of alumina.32 Meanwhile, nano boehmite has been rarely used as support for catalyst.34–36 Therefore herein a nickel–S-methylisothiourea complex immobilized on boehmite nanoparticles (Ni–SMTU@boehmite) has been reported as organometallic nanocatalyst for the synthesis of 5-substituted 1H-tetrazole derivatives using nitriles and sodium azide in PEG-400.
2 Results and discussion
2.1 Catalyst preparation
In order to prepare Ni–SMTU@boehmite, initially the boehmite nanoparticles was prepared according to new reported procedure,37 then modified using (3-chloropropyl)trimethoxysilane (CPTES). In the next step, the terminal Cl was successfully replaced by S-methylisothiourea hemisulfate salt (SMTU) through substation reaction, which SMTU has been supported on boehmite nanoparticles (SMTU@boehmite). Finally nickel(II) particles have been coordinated with SMTU@boehmite (Scheme 1). The prepared catalyst has been characterized by FT-IR, XRD, TEM, SEM, EDS, TGA and ICP-OES analysis.
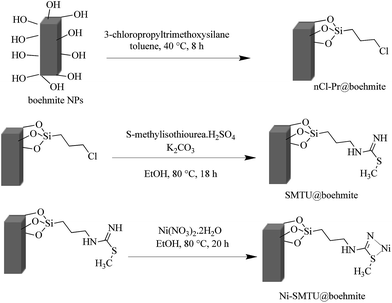 |
| Scheme 1 Synthesis of Ni–SMTU@boehmite. | |
2.2 Catalyst characterizations
The XRD pattern of the boehmite nanoparticles, shows several peaks at 2θ values at 14.40 (0 2 0), 28.39 (1 2 0), 38.57 (0 3 1), 46.46 (1 3 1), 49.54 (0 5 1), 51.94 (2 0 0), 56.01 (1 5 1), 59.35 (0 8 0), 65.07 (2 3 1), 65.56 (0 0 2), 68.10 (1 7 1), and 72.38 (2 5 1) corresponded to crystal planes of boehmite nanoparticles in orthorhombic unit cell (Fig. 1).34,35
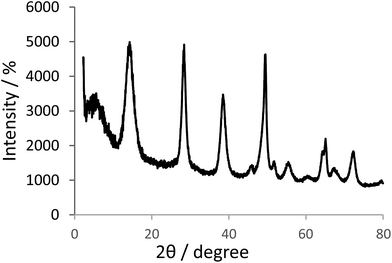 |
| Fig. 1 The XRD pattern of boehmite nanoparticles. | |
Scanning electron microscopy (SEM) was used to obtain direct information about the size and morphology of the prepared boehmite nanoparticles and Ni–SMTU@boehmite nanoparticles. SEM images show these nanoparticles was prepared with an average size about 20–40 nm (Fig. 2), were of uniform size, and showed good dispersity. Also the size and morphology of Ni–SMTU@boehmite was studied by TEM technique. As shown in Fig. 3, the unit cells of Ni–SMTU@boehmite was obtained in cubic orthorhombic structures with about 20–40 nm of size.
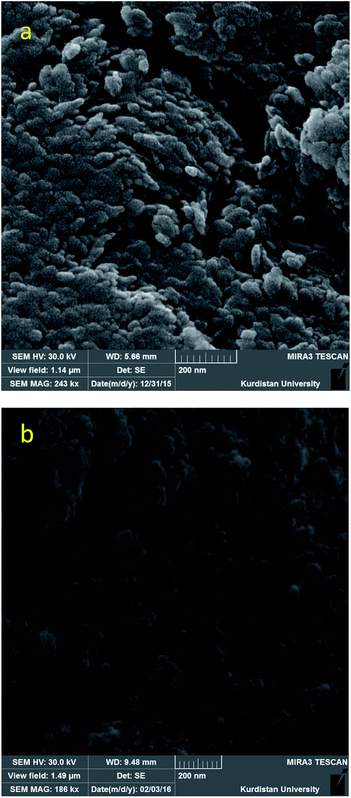 |
| Fig. 2 SEM images of boehmite nanoparticles (a) and Ni–SMTU@boehmite (b). | |
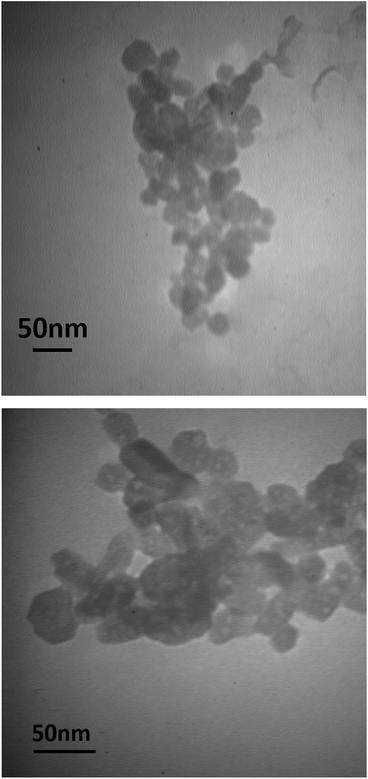 |
| Fig. 3 TEM images of Ni–SMTU@boehmite. | |
In order to show the presence elements in catalyst, the EDS analysis of Ni–SMTU@boehmite was performed. The EDS patterns of Ni–SMTU@boehmite is shown in Fig. 4, that EDS spectrum of this catalyst indicates the presence of Al, O, Si, C, S and N and Ni specie in the catalyst.
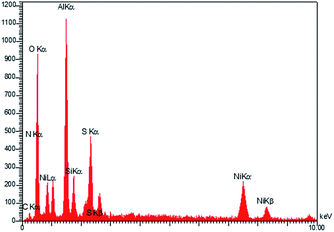 |
| Fig. 4 EDX spectrum of Ni–SMTU@boehmite. | |
Also, upon complexation of the modified boehmite nanoparticles with Ni, the exact amount of loaded metal was determined by inductively coupled plasma atomic emission spectroscopy (ICP-OES). Based on ICP, the nickel content of described catalyst was 1.64 × 10−3 mol g−1.
Successful functionalization of Ni-complex on boehmite nanoparticles was confirmed by FT-IR technique (Fig. 5). As shown in spectrum a, strong bands at 3086 and 3308 cm−1 are attributed to the symmetrical and asymmetrical modes of the hydroxyl groups on the surface of boehmite nanoparticles.37,38 In spectra a–d, several peaks at 480, 605 and 735 cm−1 are related to the Al–O bonds in boehmite structure.33 Also, the nitrate impurity and hydrogen bands (OH⋯OH) vibration are appeared at (1637) and (1164 and 1069) cm−1 respectively.25,32 In spectrum b, the modification of boehmite nanoparticles with chloropropyltrimethoxysilane is authenticated by C–H stretching vibrations (2955 cm−1) and O–Si stretching vibration (1073 cm−1). In spectrum c, the existence of the grafted S-methylisothiourea groups has been proved by C
N vibration peak (1638 cm−1).38
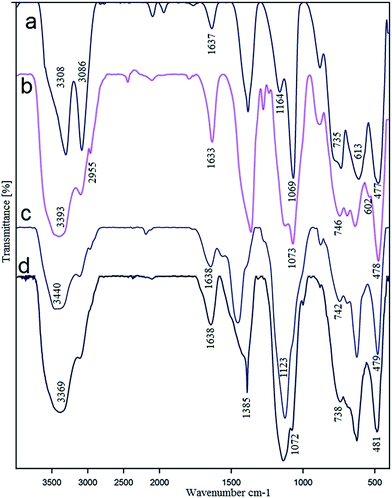 |
| Fig. 5 FT-IR spectra of boehmite nanoparticles (a), nCl–Pr@boehmite (b), SMTU@boehmite (c) and Ni–SMTU@boehmite (d). | |
In order to indicate the bond formation between the boehmite nanoparticles with the organic layers and Ni-complex, the TGA analysis was performed (Fig. 6). The TGA curve of the Ni–SMTU@boehmite shows the mass loss of the supported complex and organic functional groups as it decompose upon heating. In the all samples, the negligible weight loss below 200 °C is due to the attached hydroxyl groups or adsorbed solvent molecules on the surface of nanoparticles.24 But organic spacers and Ni-complex have been reported to desorb at temperatures above 260 °C. Based on TGA data, the weight losses of boehmite nanoparticles and SMTU@boehmite from 200 to 600 °C are 15% and 30%, respectively. While the weight loss of Ni–SMTU@boehmite is 47%. These results were confirmed that organic layers and Ni-complex have been anchored in the surface of boehmite nanoparticles.
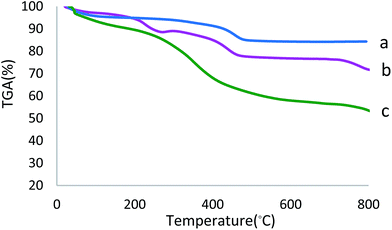 |
| Fig. 6 TGA diagram of boehmite nanoparticles (a), SMTU@boehmite (b) and Ni–SMTU@boehmite (c). | |
2.3 Catalytic study
We examined the activity of Ni–SMTU@boehmite as an efficient, stable, reusable and commercially available catalyst for the synthesis of 5-substituted 1H-tetrazole derivatives (Scheme 2).
 |
| Scheme 2 Synthesis of 5-substituted 1H-tetrazole derivatives in the presence of Ni–SMTU@boehmite. | |
In order to optimize the reaction conditions, the reaction of benzonitrile and sodium azide (NaN3) was selected as a model reaction. The effect of various parameters such as temperature, solvents, amount of catalyst and NaN3 were considered in the [2 + 3] cycloaddition reaction of benzonitrile with NaN3 (Table 1). When, 15 mg of catalyst was used to catalyze the model reaction, the product was obtained in 52% yield within 80 min at 120 °C. Increasing the amount of catalyst from 20 to 25 mg was led to increasing in yield of product from 69 to 94%. While, increasing the amount of catalyst (from 25 to 30 mg) had no significant effect on yield of product. In order to find the best solvent for this transformation, the model reaction was studied in PEG-400, DMSO, DMF, H2O and EtOH that the best results were obtained in PEG-400 (Table 1, entry 5). Also the effect of various amount of NaN3 were examined in the [2 + 3] cycloaddition reaction of benzonitrile with NaN3 (Table 1) and 1.4 mmol of NaN3 was found to be for completion of reaction within 80 min at 120 °C (Table 1, entry 5). Also, we found that the reaction yields were susceptible to temperature changes. Therefore, the effect of temperature were described (Table 1, entries 10–12) and the best results were obtained at 120 °C. As shown in Table 1, 0.025 g of Ni–SMTU@boehmite in PEG-400 at 120 °C using 1.4 mmol of NaN3 was found to be ideal the reaction conditions for the synthesis of 5-substituted 1H-tetrazole derivatives.
Table 1 Optimization of reaction conditions for synthesis of 5-substituted 1H-tetrazole derivatives in the presence of Ni–SMTU@boehmite

|
Entry |
Catalyst (mg) |
Solvent |
NaN3 (mmol) |
Temperature (°C) |
Time (min) |
Yielda (%) |
Isolated yield. |
1 |
30 |
PEG |
1.2 |
120 |
80 |
60 |
2 |
30 |
PEG |
1.3 |
120 |
80 |
73 |
3 |
30 |
PEG |
1.4 |
120 |
80 |
93 |
4 |
25 |
PEG |
1.4 |
120 |
80 |
94 |
5 |
20 |
PEG |
1.4 |
120 |
80 |
69 |
6 |
15 |
PEG |
1.4 |
120 |
80 |
52 |
7 |
25 |
DMSO |
1.4 |
120 |
60 |
89 |
8 |
25 |
DMF |
1.4 |
120 |
60 |
90 |
9 |
25 |
H2O |
1.4 |
Reflux |
80 |
64 |
10 |
25 |
EtOH |
1.4 |
Reflux |
80 |
40 |
11 |
25 |
PEG |
1.4 |
100 |
80 |
72 |
12 |
25 |
PEG |
1.4 |
80 |
80 |
44 |
In order to show the efficiency of Ni–SMTU@boehmite than corresponding homogeneous one, the homogeneous catalyst without boehmite nanoparticles (nPr–Si(OMe)3–SMTU–Ni) and also S-methylisothiourea complex of Ni (SMTU–Ni) has been synthesized and their catalytic activity has been compared with Ni–SMTU@boehmite for the synthesis of 5-(2-chlorophenyl)-1H-tetrazole. The results of this comparison are shown in Table 2. As shown in Table 2, catalytic activity of heterogeneous and reusable catalyst (Ni–SMTU@boehmite) is comparable to their corresponding homogeneous ones, because: (1) the boehmite was formed in nanometer-sized particles (20–40 nm), which; when the size of the support is decreased to the nanometer scale, the surface area is substantially increased and the support can be evenly dispersed in the reaction mixture, forming a homogenous emulsion. (2) High-surface-area of boehmite nanoparticles including many hydroxyl groups leads to high catalyst loading capacity. Therefore, as shown in Table 2, these heterogeneous catalyst showed the advantages of both homogeneous (high catalytic activity) and heterogeneous (easy and rapid recoverability and recyclability) catalyst. Also, it is very important that the reaction did not proceed in the absence of catalyst even after long time (Table 2, entry 1).
Table 2 Comparison of Ni–SMTU@boehmite with corresponding homogeneous catalyst in the synthesis of 5-(2-chlorophenyl)-1H-tetrazole under optimized condition
Entry |
Substrate |
Catalyst |
Time (min) |
Yielda (%) |
Isolated yield. |
1 |
2-Chlorobenzonitrile |
— |
80 |
Trace |
2 |
2-Chlorobenzonitrile |
SMTU–Ni |
55 |
91 |
3 |
2-Chlorobenzonitrile |
nPr–Si(OMe)3–SMTU–Ni |
60 |
92 |
4 |
2-Chlorobenzonitrile |
Ni–SMTU@boehmite |
60 |
90 |
After optimizing the reaction conditions, in order to explore the generality and scope of this protocol, various nitriles with NaN3 were employed as substrates for the synthesis of 5-substituted 1H-tetrazole derivatives in the presence of Ni–SMTU@boehmite. The results of this study are summarized in Table 3. These results show that the reactions are equally facile with both electron-donating and electron-withdrawing substituents on the benzonitriles, resulting in good-to-high yields of the corresponding tetrazoles.
Table 3 Synthesis of 5-substituted 1H-tetrazole derivatives in the presence of Ni–SMTU@boehmite
A plausible mechanism for the synthesis of tetrazole derivatives catalyzed by Ni–SMTU@boehmite is shown in Scheme 3.38,39 Initially, catalyst reacts with nitrile to produce the intermediate I; the [3 + 2] cycloaddition reaction between azide and I form the intermediate II; protonation of complex II HCl gives III, which rearranges to produce more stable desired product IV.
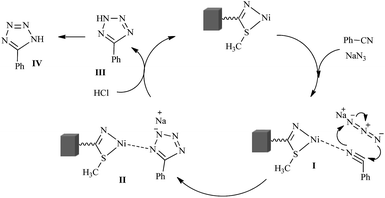 |
| Scheme 3 Plausible mechanism for the synthesis of tetrazoles catalyzed by Ni–SMTU@boehmite. | |
In order to examine the efficiency of these procedures, we compared the results of the model reaction ([2 + 3] cycloaddition of benzonitrile with NaN3) with the previously reported methods in the literatures (Table 4). This catalyst showed short reaction time and good yield than other reported catalysts in literatures. Also Ni–SMTU@boehmite is outstanding in terms of simple preparation, non-toxicity, price, stability and easy separation. As shown in Table 4, many previously reported catalysts have some drawbacks or limitations such as use of homogeneous catalysts that its separation is difficult from the reaction mixture, hazardous organic solvents and long reaction times. While, synthesis of tetrazoles in the presence of Ni–SMTU@boehmite has been carried out in PEG as green solvent with short reaction time. Also some of the previously reported catalysts are more expensive, requires high temperature or inert atmosphere, a lot of time and tedious conditions to prepare. While, boehmite nanoparticles was synthesized in water without inert atmosphere using commercially available materials. In addition, the number recycling of Ni–SMTU@boehmite is more than other catalysts. As shown in Fig. 7, Ni–SMTU@boehmite was reused over 10 runs in synthesis of 2-(1H-tetrazol-5-yl)phenol without any significant loss of its catalytic activity or nickel leaching, which clearly demonstrates the practical reusability of this catalyst. Nickel leaching of Ni–SMTU@boehmite was studied by ICP-PEIS analysis and hot filtration test. Based on the obtained results from ICP-OES analysis, the amount of Ni in fresh catalyst and the recycled catalyst after 10 times recycling is 1.64 mmol g−1 and 1.31 mmol g−1, respectively, which indicated that Ni leaching of this catalyst was very low. In order to examine leaching of nickel in reaction mixture and heterogeneity of this catalyst, we performed hot filtration in the reaction of 2-chlorobenzonitrile with NaN3. In this study we found the yield of product in the half time of the reaction was 46%. Then the reaction was repeated and in half time of the reaction, the catalyst recovered and allowed the filtrate to react further. The yield of reaction in this stage was 50% that confirmed the leaching of nickel hasn't been occurred.
Table 4 Comparison results of Ni–SMTU@boehmite with other catalysts for synthesis of 5-phenyl-1H-tetrazole
Entry |
Catalyst |
Condition |
Time (h) |
Yielda (%) |
Ref. |
Isolated yield. |
1 |
CoY zeolite |
DMF, 120 °C |
14 |
90 |
3 |
2 |
Cu–Zn alloy nanopowder |
DMF, 135 °C |
10 |
95 |
4 |
3 |
B(C6F5)3 |
DMF, 120 °C |
8 |
94 |
9 |
4 |
CAN supported HY-zeolite |
DMF, 110 °C |
4 |
93 |
12 |
5 |
(NH4)Ce(NO3)6 |
DMF, 110 °C |
6 |
97 |
13 |
6 |
Fe3O4@SiO2/salen Cu(II) |
DMF, 120 °C |
7 |
90 |
40 |
7 |
Fe3O4/ZnS HNSs |
DMF, 120 °C |
24 |
81.1 |
41 |
8 |
Mesoporous ZnS |
DMF, 120 °C |
36 |
86 |
42 |
9 |
AgNO3 |
DMF, 120 °C |
5 |
83 |
43 |
10 |
LiB(N3)4 |
NH4OAc (15 mg), DMF/MeOH (9/1), 120 °C |
8 |
86 |
44 |
11 |
Cu(OAc)2 |
DMF, 120 °C |
12 |
98 |
45 |
12 |
CuFe2O4 |
DMF, 120 °C |
12 |
82 |
46 |
13 |
FeCl3–SiO2 |
DMF, 120 °C |
12 |
79 |
47 |
14 |
Nano ZnO/Co3O4 |
DMF, 120–130 °C |
12 |
90 |
48 |
15 |
Ni–SMTU@boehmite |
PEG, 120 °C |
1.33 |
94 |
This work |
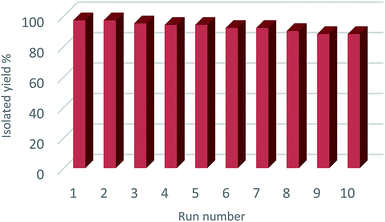 |
| Fig. 7 Recyclability of Ni–SMTU@boehmite in the synthesis of 2-(1H-tetrazol-5-yl)phenol. | |
3 Conclusions
In summary, Ni–SMTU@boehmite as a novel type of recoverable nanocatalyst was prepared by grafting of nickel on modified boehmite nanoparticles by very simple and inexpensive procedure without inert atmosphere. This catalyst was synthesized from inexpensive and commercially available starting materials. This catalyst has been characterized by FT-IR spectroscopy, TGA, XRD, ICP-OES, EDS, SEM and TEM techniques. The advantages of this protocol are the use of an eco-friendly, chemically stable and commercially available materials, the operational simplicity and good to high yields. More important, nano boehmite is new support for heterogenization of homogeneous catalysts, which has several advantages such as high surface area, high stability, ease of surface modification, easy and inexpensive procedure of preparation. Also in this protocol, catalyst was reused for 10 times without Ni leaching or any significant loss of its catalytic activity. Heterogeneity of this catalyst has been studied using hot filtration and ICP-OES techniques. This catalyst showed excellent catalytic activity, high reusability and air or moisture stability for synthesis of 5-substituted 1H-tetrazole derivatives in PEG-400. Additionally, allylic, aliphatic and aromatic nitriles can also be used to synthesis tetrazoles using this catalyst. Thus, this methodology is effective for a wide range of nitriles for the synthesis of 5-substituted 1H-tetrazoles.
4 Experimental
4.1 Preparation of the Ni–SMTU@boehmite
The boehmite nanoparticles was synthesized according to our recently reported procedure via addition of aqueous NaOH solution to aqueous solution of Al(NO3)3·9H2O as drop to drop under vigorous stirring.36 The obtained boehmite nanoparticles (1.5 g) was dispersed in 50 mL toluene by sonication for 30 min, and then 2.5 mL of (3-choloropropyl)triethoxysilane (CPTES) was added to mixture. The reaction mixture was stirred at 40 °C for 24 h. Then, the prepared nanoparticles (nCl–Pr@boehmite) was filtered, washed with ethanol and dried at room temperature. The obtained nCl–Pr@boehmite (1 g) were dispersed in 50 mL ethanol for 20 min, and then S-methylisothiourea hemisulfate salt (2.5 mmol) and potassium carbonate (2.5 mmol) were added to the reaction mixture and stirred for 28 h at 80 °C. Then, the resulting nanoparticles (SMTU@boehmite) was filtered, washed with ethanol and dried at room temperature. The obtained SMTU@boehmite (0.5 g) was dispersed in 25 mL ethanol by sonication for 20 min, and then Ni(NO3)2·6H2O (1 mmol) was added to the reaction mixture. The reaction mixture was stirred at 80 °C for 20 h. The final product (Ni–SMTU@boehmite) was filtered, washed by ethanol and dried at room temperature.
4.2 General procedure for the synthesis of 5-substituted 1H-tetrazoles
A mixture of sodium azide (1.4 mmol) and nitrile (1 mmol) in the presence of 0.025 g of Ni–SMTU@boehmite, was stirred at 120 °C in PEG-400; after completion of the reaction (observed by TLC), the reaction mixture was cooled down, and catalyst was isolated by simple filtration and HCl (4 N, 10 mL) was added to the filtrated solution. The products extracted with ethyl acetate (2 × 10 mL). The organic solvent was dried over anhydrous sodium sulfate, and concentrated to give the crude solid product.
4.3 Selected spectral data
5-Phenyl-1H-tetrazole (Table 2, entry 1). White solid, mp 212–214 °C, FTIR (KBr): νmax/cm: 3436, 2920, 1610, 1559, 1474, 1405, 1252, 1159, 1051, 987, 784, 689, 492. 1H NMR (400 MHz, DMSO, ppm) δ = 7.65–7.59 (m, 3H), 8.08–8.04 (m, 2H).
5-(4-Nitrophenyl)-1H-tetrazole (Table 2, entry 2). Yellow solid, mp 218–220 °C, FTIR (KBr): νmax/cm: 3508, 3101, 2967, 2919, 2854, 2755, 2547, 1646, 1608, 1521, 1439, 1346, 1156, 1101, 1024, 951, 854, 721, 696, 494. 1H NMR (400 MHz, DMSO, ppm) δ = 8.43–8.41 (d, J = 8.8 Hz, 2H), 8.31–8.29 (d, J = 8.8 Hz, 2H).
1-(4-(1H-Tetrazol-5-yl)phenyl)ethanone (Table 2, entry 3). White solid, mp 173–175 °C, FT-IR (KBr): νmax/cm: 3432, 3144, 3079, 3011, 2969, 2921, 2858, 2719, 2625, 2472, 1680, 1570, 1431, 1365, 1271, 1156, 1120, 1081, 1058, 997, 982, 961, 841, 752, 704, 589, 491. 1H NMR (400 MHz, DMSO, ppm) δ = 8.20–8.14 (m, 4H), 2.64 (s, 3H).
5-(4-Bromophenyl)-1H-tetrazole (Table 2, entry 4). White solid, mp 260–262 °C, FTIR (KBr): νmax/cm: 3443, 2956, 2923, 2861, 2766, 2627, 1601, 1423, 1317, 1152, 1055, 998, 842, 734, 562. 1H NMR (400 MHz, DMSO, ppm) δ = 8.00–7.97 (m, 2H), 7.84–7.81 (m, 2H).
5-(3-Chlorophenyl)-1H-tetrazole (Table 2, entry 5). White solid, mp 132–133 °C, FTIR (KBr): νmax/cm: 3454, 3241, 3063, 2972, 2894, 2605, 1972, 1651, 1473, 1445, 1360, 1300, 1246, 1094, 1002, 772, 675, 576. 1H NMR (400 MHz, DMSO, ppm) δ = 8.09–8.00 (m, 1H), 8.04–8.01 (m, 1H), 7.69–7.63 (m, 2H).
2-(1H-Tetrazol-5-yl)phenol (Table 2, entry 7). White solid, mp 224–226 °C, FTIR (KBr): νmax/cm: 3253, 3059, 2933, 2709, 2566, 2359, 1610, 1545, 1475, 1393, 1358, 1294, 1230, 1115, 1068, 946, 808, 742, 680, 541. 1H NMR (400 MHz, DMSO, ppm) δ = 8.02–8.00 (m, 1H), 7.44–7.40 (m, 1H), 7.11–7.08 (m, 1H), 7.03–6.99 (m, 1H), 11.10 (br, 1H), 15.73 (br, 1H).
4-(1H-Tetrazol-5-yl)benzonitrile (Table 2, entry 8). White solid, mp 253 °C, FT-IR (KBr): νmax/cm: 3476, 3150, 3090, 3018, 2923, 2859, 2724, 2624, 2566, 2485, 2232, 1620, 1566, 1494, 1431, 1364, 1275, 1153, 1067, 1028, 847, 749, 695, 552. 1H NMR (400 MHz, DMSO, ppm) δ = 8.24–8.21 (m, 2H), 8.12–8.09 (m, 2H).
5-(3-Nitrophenyl)-1H-tetrazole (Table 2, entry 9). White solid, mp 149–151 °C, FTIR (KBr): νmax/cm: 3852, 3308, 3085, 2975, 2894, 2822, 2752, 1621, 1524, 1348, 1243, 1156, 1076, 1011, 912, 819, 727, 453. 1H NMR (400 MHz, DMSO, ppm) δ = 8.85 (s, 1H), 8.50–8.43 (m, 2H), 7.94–7.90 (m, 1H).
Acknowledgements
This work was supported by the research facilities of Ilam University, Ilam, Iran.
References
- J. Roh, K. Vávrová and A. Hrabálek, Eur. J. Org. Chem., 2012, 6101 CrossRef CAS.
- M. A. K. Zarchi and F. Nazem, J. Appl. Polym. Sci., 2012, 123, 1977 CrossRef CAS.
- V. Rama, K. Kanagaraj and K. Pitchumani, J. Org. Chem., 2011, 76, 9090 CrossRef CAS PubMed.
- G. Aridoss and K. K. Laali, Eur. J. Org. Chem., 2011, 6343 CrossRef CAS.
- S. S. E. Ghodsinia and B. Akhlaghinia, RSC Adv., 2015, 5, 49849 RSC.
- Z. P. Demko and K. B. Sharpless, J. Org. Chem., 2001, 66, 7945 CrossRef CAS PubMed.
- M. A. E. A. Ali Ali El-Remaily and S. K. Mohamed, Tetrahedron, 2014, 70, 270 CrossRef.
- A. Khalafi-Nezhad and S. Mohammadi, RSC Adv., 2013, 3, 4362 RSC.
- S. Kumar Prajapti, A. Nagarsenkar and B. Nagendra Babu, Tetrahedron Lett., 2014, 55, 3507 CrossRef.
- A. Kudelko, K. Jasiak and K. Ejsmont, Monatsh. Chem., 2015, 146, 303 CrossRef CAS PubMed.
- F. Abrishami, M. Ebrahimikia and F. Rafiee, Appl. Organomet. Chem., 2015, 29, 730 CrossRef CAS.
- P. Sivaguru, K. Bhuvaneswari, R. Ramkumar and A. Lalitha, Tetrahedron Lett., 2014, 55, 5683 CrossRef CAS.
- S. Kumar, S. Dubey, N. Saxena and S. K. Awasthi, Tetrahedron Lett., 2014, 55, 6034 CrossRef CAS.
- M. Hajjami and B. Tahmasbi, RSC Adv., 2015, 5, 59194 RSC.
- A. Najafi Chermahini, M. Azadi, E. Tafakori, A. Teimouri and M. Sabzalian, J. Porous Mater., 2016, 23, 441 CrossRef CAS.
- N. Razavi and B. Akhlaghinia, RSC Adv., 2015, 5, 12372 RSC.
- H. Sharghi, S. Ebrahimpourmoghaddam and M. M. Doroodmand, J. Organomet. Chem., 2013, 738, 41 CrossRef CAS.
- G. Qi, W. Zhang and Y. Dai, Res. Chem. Intermed., 2015, 41, 1149 CrossRef CAS.
- A. Fazeli, H. A. Oskooie, Y. S. Beheshtiha, M. M. Heravi, H. Valizadeh and F. F. Bamoharram, Monatsh. Chem., 2013, 144, 1407 CrossRef.
- J. Chen, W. Su, H. Wu, M. Liu and C. Jin, Green Chem., 2007, 9, 972 RSC.
- X. Liu, X. Zhao and M. Lu, J. Organomet. Chem., 2014, 768, 23 CrossRef CAS.
- M. Nikoorazm, A. Ghorbani-Choghamarani and N. Noori, Appl. Organomet. Chem., 2015, 29, 328 CrossRef CAS.
- A. Ghorbani-Choghamarani, B. Tahmasbi and P. Moradi, Appl. Organomet. Chem., 2016, 30, 422 CrossRef CAS.
- A. Ghorbani-Choghamarani, B. Tahmasbi, F. Arghand and S. Faryadi, RSC Adv., 2015, 5, 92174 RSC.
- V. Vatanpour, S. S. Madaenia, L. Rajabi, S. Zinadini and A. A. Derakhshan, J. Membr. Sci., 2012, 401–402, 132 CrossRef CAS.
- M. Mirzaee, B. Bahramian and A. Amoli, Appl. Organomet. Chem., 2015, 29, 593 CrossRef CAS.
- Z. Wu, Y. Mao, M. Song, X. Yin and M. Zhang, Catal. Commun., 2013, 32, 52 CrossRef CAS.
- S. M. Kim, Y. J. Lee, K. W. Jun, J. Y. Park and H. S. Potdar, Mater. Chem. Phys., 2007, 104, 56 CrossRef CAS.
- X. Y. Chen, H. S. Huh and S. W. Lee, Nanotechnology, 2007, 18, 285608 CrossRef.
- M. Thiruchitrambalam, V. R. Palkar and V. Gopinathan, Mater. Lett., 2004, 58, 3063 CrossRef CAS.
- L. Rajabi and A. A. Derakhshan, Sci. Adv. Mater., 2010, 2, 163 CrossRef CAS.
- A. Ghorbani-Choghamarani and B. Tahmasbi, New J. Chem., 2016, 40, 1205 RSC.
- H. Liu, J. Deng and W. Li, Catal. Lett., 2010, 137, 261 CrossRef CAS.
- K. Bahrami, M. M. Khodaei and M. Roostaei, New J. Chem., 2014, 38, 5515 RSC.
- E. Carbonell, E. Delgado-Pinar, J. Pitarch-Jarque, J. Alarcón and E. García-Españ, J. Phys. Chem., 2013, 117, 14325 CAS.
- M. Hajjami, A. Ghorbani-Choghamarani, R. Ghafouri-Nejad and B. Tahmasbi, New J. Chem., 2016, 40, 3066 RSC.
- A. Ghorbani-Choghamarani, Z. Darvishnejad and B. Tahmasbi, Inorg. Chim. Acta, 2015, 435, 223 CrossRef CAS.
- F. Abrishami, M. Ebrahimikia and F. Rafiee, Appl. Organomet. Chem., 2015, 29, 730 CrossRef CAS.
- S. Kumar, S. Dubey, N. Saxena and S. Kumar Awasthi, Tetrahedron Lett., 2014, 55, 6034 CrossRef CAS.
- F. Dehghani, A. R. Sardarian and M. Esmaeilpour, J. Organomet. Chem., 2013, 743, 87 CrossRef CAS.
- Q. Gang, L. Wei and B. Zhining, Chin. J. Chem., 2011, 29, 131 CrossRef.
- L. Lang, H. Zhou, M. Xue, X. Wang and Z. Xu, Mater. Lett., 2013, 106, 443 CrossRef CAS.
- W. X. Wang, H. L. Cai and R. G. Xiong, Chin. Chem. Lett., 2013, 24, 783 CrossRef CAS.
- Y. W. Yao, Y. Zhou, B. P. Lin and C. Yao, Tetrahedron Lett., 2013, 54, 6779 CrossRef CAS.
- P. Mani, A. K. Singh and S. K. Awasthi, Tetrahedron Lett., 2014, 55, 1879 CrossRef CAS.
- B. Sreedhar, A. Suresh Kumar and D. Yada, Tetrahedron Lett., 2011, 52, 3565 CrossRef CAS.
- U. B. Patil, K. R. Kumthekar and J. M. Nagarkar, Tetrahedron Lett., 2012, 53, 3706 CrossRef CAS.
- S. M. Agawane and J. M. Nagarkar, Catal. Sci. Technol., 2012, 2, 1324 CAS.
|
This journal is © The Royal Society of Chemistry 2016 |
Click here to see how this site uses Cookies. View our privacy policy here.