DOI:
10.1039/C6RA04218J
(Paper)
RSC Adv., 2016,
6, 64344-64356
In vitro and in vivo assessment of novel pH-sensitive interpenetrating polymer networks of a graft copolymer for gastro-protective delivery of ketoprofen†
Received
17th February 2016
, Accepted 20th June 2016
First published on 28th June 2016
Abstract
The pH-sensitive polyacrylamide-grafted-tamarind seed polysaccharide (PAam-g-TSP) copolymer was synthesized under microwave irradiation in the presence of ceric ammonium nitrate in order to initiate the reaction. The reaction mixture was subjected to hydrolysis in alkaline medium. The copolymer was characterized with the help of FTIR, 1H-NMR and elemental analysis techniques. Furthermore, pH-sensitive IPN microbeads were prepared from these polymers in combination with sodium alginate through a dual crosslinking process and used for gastro-protective delivery of ketoprofen. The IPN microbeads were tested in vitro and in vivo and showed pH-dependent swelling/shrinking with the changing pH of the medium. Drug release was higher in 7.4 pH medium (about 83%), but it was lower in pH 1.2 medium (about 17%). The in vivo pharmacokinetics, anti-inflammatory activity and stomach histopathological evaluation performed on Wistar rats suggested that the microbeads are pH-sensitive and they were able to target ketoprofen to the intestine, resulting in diminished adverse effects in the stomach.
Introduction
In view of their favorable characteristics such as nontoxicity, being economical, biodegradability and ease of availability, polysaccharides are the most preferred biomaterials over synthetic polymers. These polysaccharides can be easily tailor-made to provide useful properties for applications in drug delivery.1,2 Recently, due to the broad range of smart polymeric materials that are responsive to changes in external pH, temperature, electric field, etc., they are the key components in many biomedical applications.3 Grafted copolymers are particularly useful in these areas due to their remarkable properties. Graft copolymer comprises of a macromolecular network in which one or more of a block are well connected as the side chains to the main backbone of a polymer, generating many interesting properties.4,5 After grafting, the host polymer attains many desired properties of the grafted monomer linkages.6 In particular, grafting of PAam chains onto TSP has resulted in a new copolymer that holds many combined properties of both the natural and synthetic polymers. Through hydrolysis of amide groups of PAam, chemical conversion of graft copolymer to ionic form occurs in solution, leading to the formation of a pH-sensitive copolymer,7 having many useful properties of a material required in drug delivery area.8,9
Of late, IPN based systems resulting from the blending of two or more polymers in which at least one polymer is synthesized and/or crosslinked in the immediate presence of another polymer possess useful properties.10,11 IPNs of polysaccharides especially help to maintain a constant drug concentration through its network cage such that the length of drug release can be easily extended to the convenient level.12,13 The IPNs of hydrolyzed PAam-g-TSP and NaAlg are considered to be advantageous since the two crosslinked polymers exist as three dimensional pH-sensitive networks through which enhanced free volume of the matrix can be achieved for ease of drug encapsulation and by improving the mechanical strength properties of the biomaterial. Hitherto, no literature reports are accessible on the use of IPNs of hydrolyzed PAam-g-TSP and NaAlg polymers in the area of drug delivery.
In this study, we have made an intensive effort to develop such matrices. TSP was obtained from seeds of Tamarindus indica Linn., a predominant tropical tree that grows mostly in Indian and South East Asian regions. TSP is being used as thickener, stabilizer and gelling agent in food production.14 The composition of TSP is (1→4)-β-D-glucan backbone substituted with the side chains of α-D-xylopyranose and β-D-galactopyranosyl (1→2)-α-D-xylopyranose linked (1→6) to the glucose residues,15 with a molecular weight ranging from 2.5 × 105 to 6.5 × 105.16 It is known to be non-carcinogenic, mucoadhesive, biocompatible and being used for drug delivery application.
NaAlg is another natural polysaccharide composed of 1,4-linked-β-D-mannuronic acid (M) and α-L-guluronic acid (G) residues. The ratio between the residues17 of β-D-mannuronic acid and that of α-L-guluronic acid (M/G) is generally 2
:
1. NaAlg is widely used in food manufacturing as gelling agent. In aqueous media, where multi-valent cations exist, NaAlg undergoes ionic gelation through exchange of sodium ions with other multivalent cations. Covalent crosslinking of NaAlg can therefore be achieved by treating with glutaraldehyde (GA) in smaller concentrations to find its use in modified forms for various drug delivery applications.18,19
Ketoprofen, a NSAID is used for treating musculoskeletal as well as joint disorders. It readily absorbs through the GIT and has short half-life of 2 h. It is reported to show adverse effects including ulceration, gastric irritation hemorrhage in stomach after oral dosing. Therefore, this useful drug has to be protected with a biopolymer such as the one developed here to control its release in the stomach for effective management of joint disorders and to maintain the patient compliance at the same time.20
In our earlier study, we have reported pH-sensitive IPNs of graft copolymers for controlled and targeted delivery of drugs.21–24 In continuation of these studies, we now extend our efforts to develop pH-sensitive graft copolymeric IPNs as microbeads in the size of 913 ± 3 μm. Herein, we report the synthesis of IPN microbeads from hydrolyzed PAam-g-TSP copolymer and NaAlg for controlled delivery of ketoprofen avoiding its release in the stomach. These IPN microbeads possess –COOH functional groups that can be un-ionized in the acidic stomach pH, to show small quantity of drug release, but when these microbeads are in ionized state in intestinal alkaline pH, results in a much greater release of ketoprofen. This may perhaps help to reduce the side effects of ketoprofen in the stomach. The formulations are new to the literature and are well characterized using a variety of analytical techniques as well as in vitro and in vivo experiments.
Materials and methods
Chemicals
Ketoprofen was received from Rhone-Poulenc (Mumbai, India). Tamarind seed powder was purchased locally; sodium alginate (Approximate MW 240
000 and G/M ratio 1
:
2), calcium chloride dehydrate, GA (25% w/v) and sodium hydroxide were all procured from s.d. fine chemicals (Mumbai, India). Acrylamide, ceric ammonium nitrate, potassium dihydrogen phosphate, acetonitrile (HPLC grade) and methanol (HPLC grade) were obtained from HiMedia Laboratories Pvt. Ltd. (Mumbai, India). Distilled water was used throughout the study and all chemicals used were of analytical reagent grade samples without purification.
Preparation of tamarind seed polysaccharide
To 20 g of TSP, cold distilled water (200 ml) was added to obtain slurry, which was added to 800 ml distilled water in a glass flask under boiling. Under constant stirring, boiling was continued for 30 min using rotamantle (2RML Q-19A, Remi, Mumbai, India). The resultant solution was set aside overnight, centrifuged for 20 min at 5000 rpm (R-8C DX Centrifuge, Remi, Mumbai, India), supernatant liquid was taken and added to twofold volume of absolute ethanol. The resulting precipitate was washed consecutively with petroleum ether, diethyl ether, absolute alcohol and subjected for drying at 50 °C in a Petriplate. The product was preserved for further use.25
Microwave assisted synthesis of pH-sensitive PAam-g-TSP copolymer
Synthesis of PAam-g-TSP was done using a microwave assisted irradiation process.26 Two grams of TSP was soaked in 70 ml of distilled water at 40 °C in a reaction vessel for overnight under constant stirring at 50 rpm speed. In a 10 ml each distilled water, accurately weighed amounts of Aam monomer (8.53 g) and 0.5 g of CAN were dissolved separately and then mixed with TSP solution. The reaction was carried out in a microwave oven (GMG 17E 07 SLGX, Godrej, India) by irradiating at 900 W. At specific time intervals, when the boiling of TSP solution started (∼70 °C), irradiation was interrupted and the solution was cooled in chilled water. The process of irradiation followed by cooling was carried out until the polymer was converted into a gel. The reaction mixture was set aside for 1 h to complete the reaction, after which it was added to methanol and kept for 24 h. Further steps include addition of excess amount of methanol and distilled water to the reaction mixture, filtered and dried for 12 h at 50 °C. The resultant grafted copolymer was powdered and stored properly further use.
The % grafting efficiency of the derived graft copolymer was determined as:
|
 | (1) |
where
W0,
W1 and
W2 are weights of the native TSP, grafted copolymer, and Aam, respectively.
Alkaline hydrolysis of PAam-g-TSP copolymer
Precisely weighed quantity (2 g) of PAam-g-TSP was mixed with 100 ml of 0.9 M sodium hydroxide solution with continuous stirring in a glass flask and the reaction was performed for 1 h at 75 °C on a thermostatically controlled water bath. Temperature of the reaction mixture was reduced to 25 °C, 200 ml of methanol was added and retained for 12 h to remove any excess water. The filtered product was then washed thoroughly using methanol, dried overnight at 50 °C and preserved in a desiccator before further use.
Characterization of PAam-g-TSP
FTIR analysis was performed on the native TSP, PAam-g-TSP and alkaline hydrolyzed PAam-g-TSP using FTIR spectrophotometer (Nicolet, Model Magna 550, USA) by potassium bromide pellet method. Sample pellets were made by triturating along with potassium bromide and were pressed under a hydraulic pressure of 600 kg. The samples were scanned in the range of 450–4000 cm−1.
1H-NMR experiments were done on native TSP, Aam and PAam-g-TSP using TMS (internal standard). The 10 mg dm−3 of samples were solubilized in DMSO at 60 °C and then the spectra were recorded using Varian spectrophotometer (Mercury plus 300 MHz NMR, WA, USA).
The % nitrogen, carbon and hydrogen were estimated to confirm the grafting reaction. Native TSP, PAam-g-TSP copolymer and alkaline hydrolyzed PAam-g-TSP copolymers were tested using CHN analyzer (Flash EA 1112, Thermo Finnigan, Italy).
Preparation of IPN microbeads
Polymer solutions containing native TSP with NaAlg or combination of PAam-g-TSP with NaAlg or NaAlg alone (total polymer concentration 4% w/v) were prepared in double distilled water. Ketoprofen equivalent to 20% or 40% of dry weight of polymer was added to the polymer solution and stirred at 50 rpm to obtain uniform dispersion. A 20 ml of this dispersion was taken into a hypodermic syringe and extruded little by little into calcium chloride (5% or 10% w/v) solution through a needle (#23) under continuous stirring. The resultant ionically crosslinked microbeads were segregated and washed continuously with distilled water, then dried to ambient condition for 24 h followed by drying another 10 h at 40 °C. Further, these microbeads were crosslinked using GA (4–8% w/w of dry polymer) in the presence of 1 N HCl solution at 50 °C for 30 min, and thoroughly washed with distilled water to eliminate the un-reacted GA followed by overnight drying at 40 °C. Complete elimination of un-reacted GA was proved by the negative test for washings with 2,4-dinitrophenyl hydrazine. Detailed compositions of these IPN microbeads are given in Table 1.
Table 1 Composition of pH-sensitive IPN microbeads
Microbeads |
H-PAam-g-TSP (% w/v) |
NaAlg (% w/v) |
TSP (% w/v) |
Ketoprofena (% w/w) |
CaCl2 (% w/v) |
GAa (% w/w) |
% w/w of dry polymer. |
T1 |
— |
4 |
— |
20 |
5.0 |
— |
T2 |
0.5 |
3.5 |
— |
20 |
5.0 |
— |
T3 |
1.0 |
3.0 |
— |
20 |
5.0 |
— |
T4 |
1.5 |
2.5 |
— |
20 |
5.0 |
— |
T5 |
1.5 |
2.5 |
— |
40 |
5.0 |
— |
T6 |
1.5 |
2.5 |
— |
20 |
10 |
— |
T7 |
1.5 |
2.5 |
— |
20 |
10 |
4 |
T8 |
1.5 |
2.5 |
— |
20 |
10 |
6 |
T9 |
1.5 |
2.5 |
— |
20 |
10 |
8 |
T10 |
— |
2.5 |
1.5 |
20 |
10 |
8 |
Scanning electron microscopy (SEM)
IPN microbeads coated with platinum were analyzed for surface morphology at room temperature under accelerated voltage of 10–40 kV using SEM (JEOL, JSM-6360, Kyoto, Japan).
Size of microbeads
Average size of the minimum number of 100 microbeads for each batch was determined within an accuracy of ±0.001 mm with the help of digital micrometer (MDC-25S Mitutoyo, Tokyo, Japan).
Drug encapsulation efficiency
Each batch of microbeads were accurately weighed and soaked in 50 ml phosphate buffer of pH 7.4 for 12 h at 37 °C. Then the soaked microbeads were triturated and heated at 40 °C for 1 h followed by centrifugation at 2000 rpm using a laboratory centrifuge (R-8C DX Centrifuge, Remi, Mumbai, India) to achieve complete extraction of the drug into the supernatant media. The absorbance of this supernatant media was recorded using UV-visible spectrophotometer (UV-1800, Shimadzu, Japan) at the wave-length of 260 nm and then DEE was estimated.
Thermogravimetric analysis
TGA was carried out on native NaAlg, PAam-g-TSP and IPN microbeads in the presence of dynamic nitrogen ambiance at the heating rate of 10 °C min−1 and 50 ml min−1 flow rate from 0–600 °C temperature range using a thermogravimetric analyzer (DuPont-9900, USA).
Differential scanning calorimetric analysis
DSC experiment was done on pristine ketoprofen, placebo IPN microbeads and drug-loaded IPN microbeads between the temperature ranges 0 to 300 °C at 10 °C min−1 step in a nitrogen ambiance using with the help differential scanning calorimeter (DSC Q20 V24.4 Build116, TA Instruments, USA). The samples were sealed in aluminum crucibles having perforated lids, prior to the test and the reference was an empty aluminum crucible.
X-ray diffraction analysis
X-ray diffractograms were obtained over the 2θ range of 0–90° using X-ray diffractometer (Rigaku Miniflex II, Japan and Philips, PW-171) for pristine ketoprofen, placebo microbeads and drug loaded microbeads.
Pulsatile swelling study
To confirm pH-sensitive nature of the IPN microbeads, pulsatile swelling studies were performed on the IPN microbeads. To do this, in 25 ml each of pH 1.2 and pH 7.4 buffer solutions, 20 mg of IPN microbeads were kept at 37 °C. The microbeads have undergone shrinkage and swelling by altering the pH from 1.2 to 7.4. To do this, microbeads were removed from the buffer solutions at specific time intervals, the surface-adhered liquid droplets were removed by carefully pressing in between tissue paper wraps and their weights were determined with the help of an electronic microbalance (Model BL-220H, Shimadzu, Japan). This cyclic measurement of swelling/shrinking from pH 7.4 to pH 1.2 then again to pH 7.4 was performed in order to prove the pH-dependent reversible swelling/shrinking behavior of microbeads; however, this does not reflect changes in pH during transit of microbeads all the way through gastrointestinal tract. Then, % swelling (Q) was calculated as: |
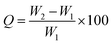 | (2) |
where W1 is dry weight of the microbeads and W2 is swollen weight.
In vitro drug release study
In vitro release of drug was performed on the IPN microbeads using a dissolution test apparatus (Electrolab TDT-08P, Mumbai, India) by taking 900 ml each of 1.2 and 7.4 pH buffer solutions at 37 ± 0.5 °C using 100 rpm paddle rotation speed. At fixed time intervals, equal aliquots (5 ml) of the sample was collected and replenished with an equivalent amount of the respective buffer solution. After filtration of the samples on a 0.45 μm membrane filter, the concentration of drug was determined by UV-visible spectrophotometer (UV-1800, Shimadzu, Japan) at the fixed wavelength of 260 nm.
In vivo pharmacokinetic evaluation
The formulation T9 has shown adequate in vitro performance, which was selected for pharmacokinetic evaluation. Wistar rats were divided into two groups with six rats each and were accustomed to the laboratory atmosphere by providing with sufficient food and water. Rats were kept fasting overnight prior for conducting these experiments except water was fed. Rats in the first group were orally administered with pristine ketoprofen (18 mg kg−1 in 0.5%, w/v solution of NaCMC), while the second group of rats were administered with T9 microbeads containing the drug equivalent to 18 mg kg−1 of ketoprofen in 0.5% w/v solution of NaCMC. The pristine ketoprofen was administered as suspension in NaCMC using oral rat feeding needle. The volume of drug solution administered was in the range of 0.5 to 1 ml depending upon body weight of animals. For administration of T9 microbeads, soft transparent tube with 3 mm inner diameter was inserted with the help of mouth gauze; microbeads were placed into the tube and pushed with about 2 ml solution of NaCMC. At different intervals of time, blood was withdrawn from the rat's orbital sinus and subjected to centrifugation using a laboratory centrifuge (C24 Centrifuge, Sigma, Germany) for 20 min at 3000 rpm speed to isolate the plasma. The isolated plasma was then preserved at −72 °C for estimating ketoprofen concentration using the HPLC method.
Plasma ketoprofen concentration was assessed by a modified reverse phase (RP) HPLC method using Shimadzu HPLC consisting of a system controller, pump, variable-wavelength UV/Visible detector and auto-injector with LC Solution software. Reverse phase C18 column (Genesis, USA; particle size 5 m; 250 mm × 4.6 mm; maintained at 25 °C) was used. Combination of acetonitrile and phosphate buffer (pH 3, 10 mM) in the ratio of 50
:
50 was used as a mobile phase. The flow rate, injection volume and maximum wavelength used were 1 ml min−1, 50 μl and 260 nm, respectively. A sample of 90 μl of plasma was blended with 10 μl of lercanidipine (internal standard) solution (50 μg ml−1) and the mixture was vortexed for 2 min. After repeated addition of cold acetonitrile (300 μl), the mixture was vortexed (2 min) and centrifuged for 10 min at 4 °C under 10
000 rpm speed. The resultant supernatant fluid was analyzed by HPLC and the plasma ketoprofen concentration was evaluated using the calibration curve. Retention time of the drug and internal standard (lercanidipine) were found to be 9.8 and 16.3 min, respectively. Both the peak patterns were suitable without any interference from the plasma matrix during retention time of any of the peaks, which were resolved appropriately. The validation was conducted for bio-analytical method with a recovery of >82% established as the reproducible state.
The data have shown as mean ± SD. Various pharmacokinetic parameters like AUC, Cmax, Tmax, t1/2 and Ke were computed using PK solutions 2.0™. The results pharmacokinetic studies were evaluated using student's t-test (Graph Pad Prism Software). A value of p < 0.05 was considered statistically significant.
In vivo anti-inflammatory study
Wistar rats were divided into three groups with six rats in each group. Rats were under fasting overnight prior for conducting the experiments except water was fed. One ml solution of NaCMC (0.5%, w/v) was administered to group I (control group), ketoprofen equivalent to body weight of 18 mg kg−1 was administered to group II rats (standard group) and T9 microbeads equivalent to drug, 18 mg kg−1 body weight were administered to group III rats (test group) orally using 1 ml solution of NaCMC.27 Carrageenan-induced paw edema method was used for evaluating the anti-inflammatory activity.28 The inflammation was produced by injecting 1% w/v of carrageenan solution (0.1 ml) in sub-plantar region of rat's left hind paw. Before 30 min of carrageenan injection, pristine ketoprofen and T9 microbeads were orally administered. Mercury displacement technique in plethysmometer was used to measure the rat's paw volume to calculate % reduction in the edema.
Examination of stomach histopathology of rats
After termination of anti-inflammatory study, the separated stomachs from the sacrificed rats were washed with normal saline and stored in 10% formalin. Stomach tissues were processed by dehydration using xylene and ethanol.29 After processing, tissues were inserted in paraffin and about 5 micron thick paraffin sections were cut and staining was done using hematoxylin and eosin (H&E). The photographs were taken using binocular light microscope (CXRIII, Labomed, Mumbai, India).
Live subject statement
All animal experiments were performed in compliance with the relevant laws and institutional guidelines; the institutional animal ethics committee (IAEC) of BLDEA's College of Pharmacy, Vijayapur has approved the animal experiments as per the committee for purpose of control and supervision of experiments on animals (CPCSEA) guidelines (Reg. no. 1076/C/07).
Results and discussion
Synthesis of PAam-g-TSP
PAam-g-TSP copolymer was synthesized via microwave-irradiation technique using CAN as an initiator to generate the free-radical sites onto TSP. Capturing electrons from –OH groups of TSP via electron deficient CAN resulted in the formation of new Ce–O bonds that undergo breaking due to microwave irradiation, leading to the creation of free radical sites on which graft reaction was initiated. Further, the grafted copolymer was subjected to hydrolysis in the presence of 0.9 M sodium hydroxide solution giving a pH-sensitive copolymer due to the conversion of –CONH2 groups of PAam into –COONa groups as shown in Scheme 1. The grafted PAam-g-TSP copolymer showed a grafting efficiency of 95.86%.
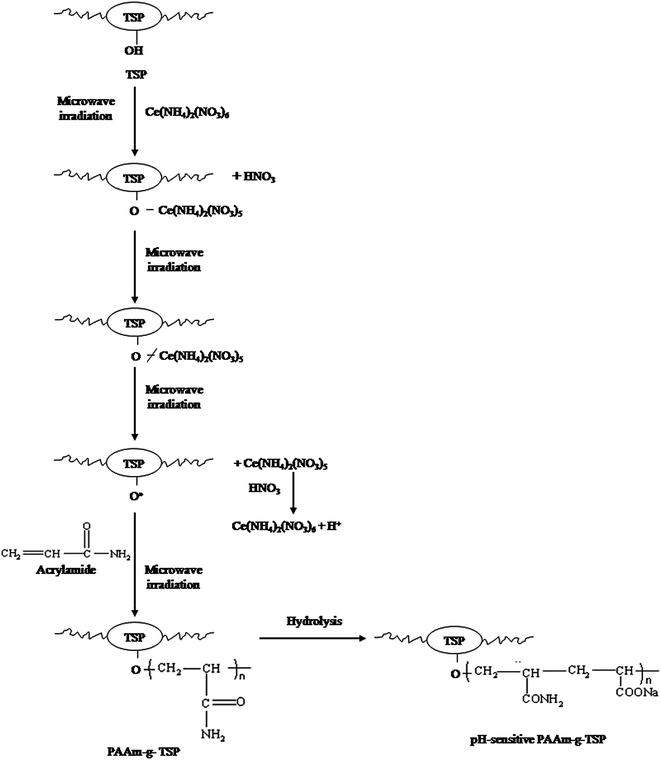 |
| Scheme 1 Microwave assisted synthesis of pH-sensitive PAam-g-TSP copolymer. | |
Characterization of PAam-g-TSP
FTIR analysis on neat TSP, PAam-g-TSP and hydrolyzed PAam-g-TSP copolymer was carried out (Fig. S1, as shown under ESI†). The neat TSP showed peaks at 3415 cm−1 and ∼2927 cm−1 due to –OH stretching vibrations of the carbohydrates and –CH2 asymmetric stretching vibrations of cyclic aldehydes, respectively. Because of the deformation of C
O groups of TSP, two peaks have appeared at 1618 and 1638 cm−1. The peak at 1416 cm−1 is the result of –CH and –CH2 in-plane bending in TSP, while a peak at 1118 cm−1 is attributed to C–O stretching vibrations. In case of PAam-g-TSP, the peaks at 3414 and 3236 cm−1 are due to NH stretching vibrations of the amides and OH groups. Because of alkyl chain's CH stretching, a peak at 2926 cm−1 appeared, but the primary amide groups of TSP appeared at 1638, 1617 and 1415 cm−1, suggesting successful grafting. In the spectra of hydrolyzed PAam-g-TSP, the peak at 3415 cm−1 is due to –OH stretching vibrations, while the peak at 2924 cm−1 is due to aliphatic –CH stretching vibrations. The peaks observed at 1617, 1638 and 1414 cm−1 are attributed to COO− groups. The alkaline hydrolysis of PAam-g-TSP was confirmed by the non-appearance of a peak at 3236 cm−1 that was seen in the PAam-g-TSP, suggesting the absence of N–H bond.
The 1H-NMR analysis of Aam, TSP and PAam-g-TSP was performed (Fig. S2, as shown under ESI†). The 1H shifts for TMS and DMSO are seen at ∼0 and ∼2.5 ppm, respectively. The Aam (A) exhibited chemical shift at ∼3.4 ppm due to protons of –CH
CO– (C is attached to O) groups, while 1H shifts are seen around ∼5.6 and ∼6.2 ppm that correspond to CH2 and CH groups, respectively.30 The CONH2 protons resonated at ∼7.0 and 7.6 ppm. Similar chemical shifts were also reported by Liu et al.,31 where CONH2 protons were observed at 7.0 and 7.7 ppm. While TSP (B) exhibits 1H shift at ∼0.9 ppm is due to methyl protons, shifts at ∼1.2 and ∼2.5 ppm are due to the protons of CH2 and CH groups. Shift at ∼3.4 ppm is due to the protons of OH groups and the shift at ∼5.3 ppm is because of glycosidic bonds (C–O–C). These data are in accordance with previous literature.32,33 But in case of PAam-g-TSP (C), along with the chemical shifts of TSP at ∼0.9 ppm (methyl protons), at ∼1.2 and ∼2.5 ppm (protons of CH2 and CH groups), at ∼3.4 ppm (protons of OH groups), new signals at ∼7.0 and 7.6 ppm are recorded due to the protons of CONH2 groups of PAam-g-TSP and also the signals which were appeared at ∼5.6 and ∼6.2 ppm in the spectra of TSP are completely disappeared. This confirms Aam grafting onto TSP. Similar observations are also available in the literature.34
Elemental analysis data given in Table 2 show 2.29% nitrogen, 41.82% carbon and 7.03% hydrogen in TSP, suggesting the existence of trace amount of proteins. On the other hand, 14.70% nitrogen, 43.62% carbon and 7.63% hydrogen are present in PAam-g-TSP, due to –CONH2 groups of polyacrylamide present on the backbone of TSP. However, a reduction of 3.87% in nitrogen is observed in hydrolyzed PAam-g-TSP due to the transformation of –CONH2 into –COONa during hydrolysis. All these data confirm the grafting and alkaline hydrolysis reactions.
Table 2 Elemental analysis results
Codes |
% nitrogen |
% carbon |
% hydrogen |
TSP |
2.29 |
41.82 |
7.03 |
PAam-g-TSP |
14.70 |
43.62 |
7.63 |
Hydrolyzed PAam-g-TSP |
3.87 |
28.99 |
5.73 |
IPN microbeads
By introducing polymer–drug dispersion drop wise into CaCl2 solution, ionic crosslinking has occurred between the chains of NaAlg as a result of replacement of Na+ ions with Ca2+ ions of the carboxylate group. The secondary chain of NaAlg conjugates with Ca2+ forms a linkage and spherically shaped microbeads encapsulated with un-crosslinked PAam-g-TSP as well as ketoprofen were formed in the presence of Ca2+ ions together with NaAlg chains. This upon covalent crosslinking with GA led to the formation of acetal structure due to the reaction of aldehydic groups of GA and –OH groups of NaAlg along with PAam-g-TSP, resulting in the IPN formation.
SEM, size and DEE
Fig. 1 depicts the surface morphology of IPN microbeads (T3 and T9) as analyzed by SEM that showed spherical nature. Ionically crosslinked (T3) microbeads showed rough and dense surfaces, while T9 that was formulated by both ionic and covalent crosslinking showed somewhat smooth surfaces. The smoothness might have been contributed by the shrinkage of microbeads at higher crosslinking as the microbeads undergo shrinking at higher crosslinking, resulting in smoother surface.35
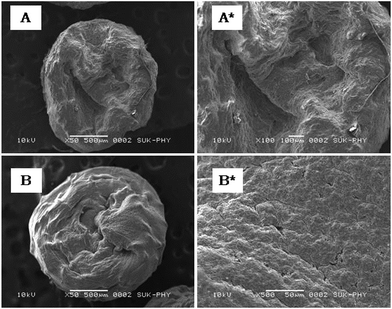 |
| Fig. 1 SEM photographs of T3 IPN microbeads (A) and T9 IPN microbeads (B). A* and B* correspond to their surfaces. | |
The size of microbeads ranged between 779 and 913 μm (see Table 3), which increased with increasing PAam-g-TSP and ketoprofen concentrations. As the quantities of PAam-g-TSP and ketoprofen were increased, the viscosity of polymer solution increased and larger droplets were formed while extruding through a needle during the preparation of microbeads, resulting in increased size, though the size was reduced with increase in concentration of calcium ions. The size of IPN microbeads was reduced due to crosslinking with GA because of rapid polymer chain shrinkage producing smaller and stiffer IPN microbeads at higher crosslink density.36
Table 3 Determination of average size, DEE and release exponent (n) of IPN microbeads
IPN microbeads |
Average size (μm) |
DEE (%) |
n |
pH 1.2 |
pH 7.4 |
T1 |
876 ± 2 |
77.38 ± 0.60 |
0.89 |
0.72 |
T2 |
881 ± 1 |
79.76 ± 0.50 |
0.87 |
0.70 |
T3 |
892 ± 3 |
82.14 ± 0.25 |
0.85 |
0.68 |
T4 |
902 ± 2 |
85.71 ± 0.61 |
0.83 |
0.65 |
T5 |
913 ± 3 |
88.69 ± 1.74 |
0.81 |
0.62 |
T6 |
879 ± 1 |
83.92 ± 0.40 |
0.85 |
0.63 |
T7 |
834 ± 1 |
80.95 ± 0.30 |
0.88 |
0.74 |
T8 |
803 ± 1 |
78.75 ± 0.95 |
0.89 |
0.77 |
T9 |
779 ± 1 |
76.19 ± 0.75 |
0.99 |
0.80 |
T10 |
771 ± 1 |
74.32 ± 0.93 |
0.86 |
0.71 |
The DEE of IPN microbeads ranged between 76% and 88% (Table 3). The DEE is higher for microbeads prepared using higher Ca2+ concentration than those prepared with lower Ca2+ concentration. With lower Ca2+ concentration, a loose network is likely to be formed due to inadequate crosslinking, as a result of which leakage of drug occurs during the production of microbeads. While at larger quantity of Ca2+, the IPN matrix becomes stiff and seepage of drug is small leading to higher DEE. Whereas at high PAam-g-TSP concentration, DEE increased because of the increase in the size of microbeads.25
TG analysis
TGA data of NaAlg, PAam-g-TSP and T9 IPN microbeads displayed in Fig. 2 show weight loss by NaAlg in two phases i.e., one at 42.69% noted up to 250 °C and a second loss of 60% up to 600 °C. The initial weight loss is the result of free and bound moisture evaporation from the polymer, while higher weight loss might be due to polymer degradation. TGA thermogram is in accordance with earlier report, where weight loss of NaAlg was also seen in two stages; the first stage of weight loss was seen up to 300 °C and second stage of weight loss occurred37 up to 650 °C. However, weight loss for PAam-g-TSP was in one phase i.e., 31% at 175 °C, which increased to 62% after 600 °C. Similar results were also reported for PAAm grafted guar gum copolymer, where one stage weight loss was observed, the initial weight loss was small and then it became constant.38 The T9 microbeads demonstrated the weight losses in two stages i.e., one up to 17% at 180 °C and another up to 60.5% at 600 °C. The residual mass of T9 microbeads was high compared PAam-g-TSP, indicating that the IPN microbeads are thermally more stable than PAam-g-TSP. In the IPN microbeads, NaAlg and PAam-g-TSP chains are knotted with crosslinking agents; therefore the thermal stability is more which confirms the IPN formation.
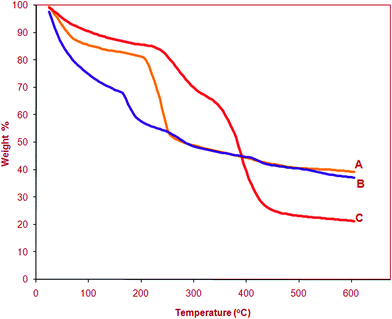 |
| Fig. 2 TGA thermograms of NaAlg (A), PAam-g-TSP (B) and drug free T9 IPN microbeads (C). | |
DSC analysis
The DSC data for pristine ketoprofen, placebo T9 microbeads and drug-loaded T9 microbeads depicted in Fig. 3 show an endothermic peak at 94 °C for ketoprofen, which at its melting point. The placebo T9 microbeads showed two endothermic peaks, one broad peak at 94 °C, which might be due to the bound and unbound moisture losses from the IPN matrix, and other at 187 °C due to thermal decomposition. Drug-loaded T9 microbeads also exhibited two peaks, one peak at 94 °C, and other at 195 °C, due to thermal decomposition. The intensity of peak at 94 °C is high compared to placebo T9 microbeads, which can be assigned to existence of surface-adhered drug in its partial crystalline nature as well as the loss of bound and unbound moisture.
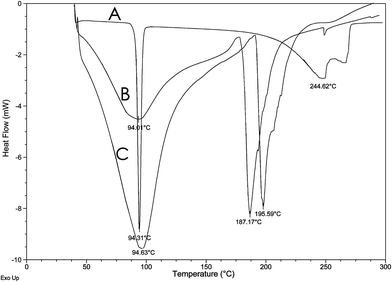 |
| Fig. 3 DSC thermograms of pristine ketoprofen (A), drug free T9 IPN microbeads (B) and drug loaded T9 IPN microbeads (C). | |
Eerikainen et al.,39 have reported that the solubility limit of ketoprofen in the polymer matrix determines the degree of crystallinity. Eudragit L nanoparticles containing 33% (w/w) drug showed no peaks related to ketoprofen indicating the amorphous form of drug. For nanoparticles loaded with 50% (w/w) drug, an endothermic peak was observed at 94 °C, suggesting the crystalline state of drug, since the solubility limit of drug exceeded in polymer matrix and the excess drug formed crystals. In our study, the solubility limit of ketoprofen in polymer network did not exceed as drug loading was only 20% (w/w); hence the drug exists in amorphous form in the IPN matrix.
XRD analysis
X-ray diffraction analysis of pristine ketoprofen, placebo T9 microbeads and drug-loaded T9 microbeads shown at Fig. 4, suggest that for pristine ketoprofen, peaks are observed between 2θ of 5° and 25° relating to the existence of drug in the crystalline states. However, no peaks are observed for placebo T9 and drug-loaded T9 microbeads between 2θ of 5° and 25° as both the diffractograms are almost identical, indicating molecular level dispersion of drug.
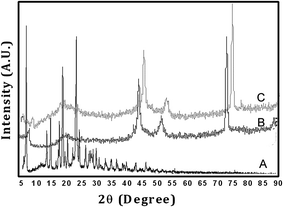 |
| Fig. 4 XRD diffractograms of pristine ketoprofen (A), drug free T9 IPN microbeads (B) and drug loaded T9 IPN microbeads (C). | |
Tamilvanan and Sa40 have reported that microparticles containing 20% (w/w) indomethacin did not show any peaks related to drug; at this low concentration, the drug was dispersed at molecular level and exists as solid solution in polymer network. However, from 30% (w/w) loading onwards, indomathacin existed in crystalline form. Therefore, in our study also, the drug loaded T9 microbeads containing 20% (w/w) ketoprofen did not show peaks between 2θ of 5° and 25°, suggesting molecular level dispersion of drug. Another possibility is that the XRD peaks depend on size of crystals; in case of drug-loaded T9 microbeads, the characteristics peaks of ketoprofen might have been overlapped with the noise of polymer matrix in which the drug is encapsulated, which is not easy to assess at the detection limit of crystal size. It suggests the molecular level dispersion of ketoprofen in the polymer network. Similar results are also available in the literature.41
pH-Sensitive swelling behavior
Swelling data of the microbeads in buffers of pH 1.2 and 7.4 are shown in Fig. 5. The microbeads formulated with NaAlg alone (T1) as well as native TSP (T10) are found to be non pH-responsive, whereas IPN microbeads prepared using PAam-g-TSP with NaAlg were pH-responsive. A reversible swelling-shrinkage was observed by changing the pH of the medium between 1.2 and 7.4. Such a response could be due to carboxyl groups of PAam-g-TSP making the chains more attracted towards water molecules. In alkaline media, ionization of carboxyl groups of microbeads might have occurred and due to increase in osmotic pressure of the inner core, increased swelling was observed.42,43
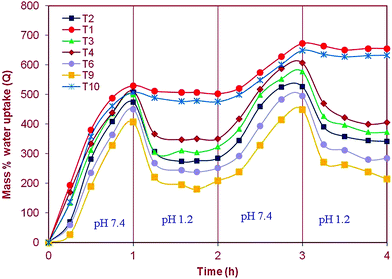 |
| Fig. 5 Pulsatile swelling behavior of IPN microbeads in solutions of different pH. | |
In vitro drug release characteristics
The results of in vitro drug release depicted in Fig. 6, show that T1 microbeads prepared by using NaAlg alone released the drug quickly, but drug release in 7.4 pH media is around 92% in 6 h. On the other hand, in 1.2 pH media, drug release is around 41% within the first 2 h. However, the drug release from microbeads of native TSP with NaAlg (T10) is much faster with 91.1% release in 6 h in buffer media of pH 7.4, while 39.9% release occurred within 2 h in pH 1.2 as shown in Fig. 6B. This suggests that microbeads prepared using native TSP and NaAlg did not slow down the drug release in acidic pH of the stomach. The IPN microbeads of PAam-g-TSP with NaAlg (T3 to T9) showed pH-sensitivity to control the drug release up to 12 h, depending on the nature of the formulation (Fig. 6A).
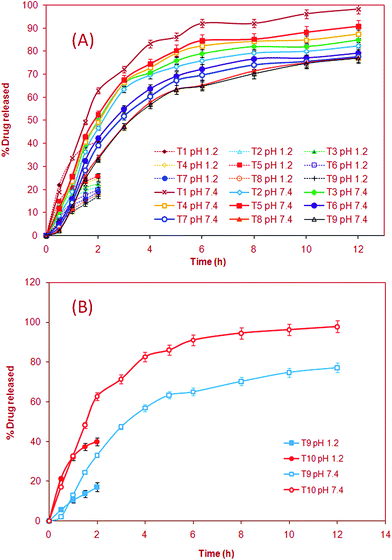 |
| Fig. 6 In vitro drug release profiles of IPN microbeads prepared using PAam-g-TSP with NaAlg (A) and native TSP with NaAlg (B). | |
Drug release in pH 1.2 buffer media is slower than that in buffer of pH 7.4 media due to increased swelling of IPN microbeads in alkaline pH condition due to the ionization of –COO− groups. Additionally, drug release is found to be dependent on the amount of PAam-g-TSP as well as the extent of crosslinking. For instance, a decrease in drug release is observed with increase in concentration of the crosslinking agent. Additionally, at increased amount of PAam-g-TSP copolymer, drug release also increased. On the contrary, with increase in initial drug loading, drug release was enhanced. This may be due to increase in initial drug loading that might have decreased the amount of polymer, thereby forming a weaker IPN matrix, thereby facilitating increased drug release.44 The IPN microbeads having covalent crosslinking (T7 to T9) exhibit retarded drug release compared to those of the ionically crosslinked microbeads (T2 to T6). The T9 formulation with the dual crosslinking showed 17% drug release in acidic environment, but 83% of drug was released in alkaline media with a controlled rate.
The release mechanism from the IPN matrix was computed using the empirical equation by estimating the K and n parameters of eqn (3)45
|
 | (3) |
here
Mt represents the drug released at time
t, and
M∞ refers to total amount of drug loaded in the formulation. The exponent
n value indicates the nature of drug release mechanism. The values of
n are calculated by employing a statistical package and these are found to vary between 0.81 and 0.91 in pH 1.2 solution confirming the Case II transport, while in 7.4 pH solution, the
n values ranged between 0.62 and 0.79, suggesting the dominance of anomalous transport (see
Table 3). The
n values increase with increasing extent of crosslinking.
In vivo pharmacokinetics evaluation
In vivo pharmacokinetic data are presented in Table 4 and displayed in Fig. 7. Following the oral administration, a rapid absorption of pristine ketoprofen was observed as indicated by a low Tmax value of 1 h compared to T9 IPN microbeads of 6 h. The Cmax value of IPN microbeads was smaller than that of pristine ketoprofen, because of its slower absorption from the IPN microbeads. The IPN microbeads exhibited longer elimination half-life (t1/2) of 4.44 h compared to pristine ketoprofen, which was 4.39 h, indicating the slower drug elimination from the T9 IPN microbeads. The elimination rate constant of IPN microbeads was found to be 0.156 ± 0.012 h−1, while it was 0.158 ± 0.009 h−1 for pristine ketoprofen. The IPN microbeads have shown increased AUC compared to pristine ketoprofen, suggesting its improved bioavailability from T9 IPN microbeads due to controlled release of drug up to ≈10 h. Our study is in conformity with the report of Abd El-Gawad et al.,46 where they observed rapid absorption of ketoprofen from control (conventional) tablets in albino rabbits after oral administration, peak plasma concentration reached within 1.3 h (Tmax), while for the test (controlled release) tablets, the Tmax was 5.7 h. Thus the decreased Cmax, increased Tmax and AUC suggests controlled release of ketoprofen from test tablets compared to control tablets.
Table 4 Pharmacokinetic parameters for pristine ketoprofen (PD) and T9 IPN microbeadsa
Sl. No |
Pharmacokinetic parameters |
Pristine ketoprofen |
T9 IPN microbeads |
Results are mean ± SD, n = 6. AUC, area under the curve; Cmax, maximum plasma concentration; Ke, elimination rate constant; Tmax, time for maximum plasma concentration; t1/2, half-life. |
1 |
Cmax (μg mL−1) |
5.20 ± 0.31 |
4.60 ± 0.38 |
2 |
Tmax (h) |
1.00 ± 0.00 |
6.00 ± 0.00 |
3 |
AUC0–∞ (μg h mL−1) |
35.10 ± 3.12 |
45.30 ± 0.29 |
4 |
AUC0–t (μg h mL−1) |
34.30 ± 3.01 |
46.30 ± 0.34 |
5 |
MRT (h) |
6.20 ± 0.46 |
8.30 ± 0.54 |
6 |
t1/2 (h) |
4.39 ± 0.39 |
4.44 ± 0.35 |
7 |
Ke (h−1) |
0.158 ± 0.009 |
0.156 ± 0.012 |
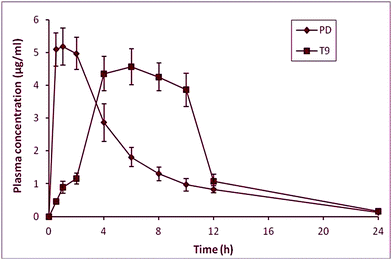 |
| Fig. 7 Plasma concentration of pristine ketoprofen (PD) and T9 IPN microbeads in rats after oral administration. Results are expressed as mean ± SD; n = 6. | |
An abrupt rise in plasma concentration was observed after oral administration of pristine ketoprofen, which started declining after 2 h, but T9 IPN microbeads have shown small quantity of drug in the plasma up to 2 h than the pristine ketoprofen. This suggests the rapid absorption of ketoprofen and quicker appearance in the plasma within 2 h as well as its quick elimination. However, in case of T9 IPN microbeads, a negligible drug release was found in the gastric environment, where the microbeads remained un-ionized, thereby showing lower swelling with the release of ketoprofen. The pharmacokinetic parameters of T9 microbeads are significantly different from pristine ketoprofen (p < 0.05), suggesting gastro-protective effect of IPN microbeads.
In vivo anti-inflammatory study
The data of anti-inflammatory study shown in Fig. 8, suggests that the pristine ketoprofen caused 90% inhibition of paw edema within 2 h and % edema inhibition declined to reach 6.54% at the end of 12 h. The T9 IPN microbeads demonstrated a lower % edema inhibition (23%) initially for 2 h and at the end of 7th h, it gradually increased to 91.1% and after the 12th h, it again decreased to 46%. This suggests that pristine ketoprofen has exerted a greater action in the first 2 h and then, it was eliminated from the blood. On the other hand, from the pH-sensitive T9 IPN microbeads, a small quantity of ketoprofen was released in acidic environment of the stomach showing a large quantity of drug being released in alkaline environment of the intestine. These results are in agreement with the literature report, where polyacrylamide grafted xanthan hydrogel beads showed similar pharmacodynamics in rats after oral administration.47,48
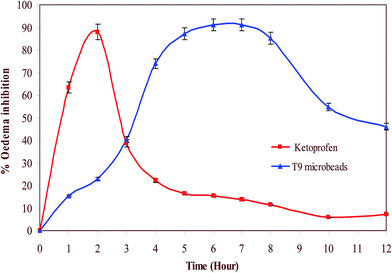 |
| Fig. 8 Anti-inflammatory activity of pristine ketoprofen and pH-sensitive T9 microbeads in rats. | |
Stomach histopathology of rats
Fig. 9 and Table 5 summarize the results of stomach histopathology. There were no indications of ulcer/hemorrhages; mucosal layer was normal in the control rats. The surface epithelium and gastric glands of this group were intact, which remained unchanged, whereas in rats administered with pristine ketoprofen, ulcers measuring about 1.97 ± 0.72 mm were noticed with observable hemorrhage. Notable erosion of mucosa associated with congestion, oedema and perforations were also observed, but the surface epithelium appeared to be unusual and gastric glands were imperfect. For the rats treated with T9 microbeads, ulcers with minimal dimensions of 0.79 ± 0.45 mm and very mild perforation, congestion, oedema and mucosal erosion were observed. However, there were no signs of hemorrhage and necrosis. From the above observations, adverse effects such as ulcers, hemorrhage and erosion of mucosa reported for ketoprofen were considerably decreased after the administration of T9 IPN microbeads. Similar histopathological changes were also noticed for ibuprofen-loaded alginate beads after oral administration in mice.29
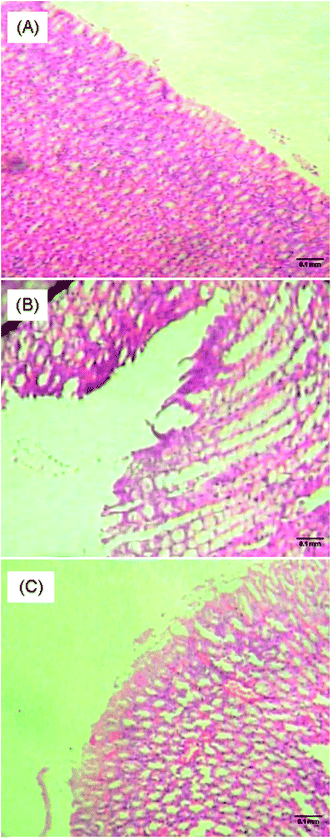 |
| Fig. 9 Stomach histopathology of control rats (A), rats treated with pristine ketoprofen (B) and rats treated with T9 IPN microbeads (C) (Haematoxylin-Eosin, 100×). Size bar = 0.1 mm. | |
Table 5 Results of stomach histopathologya
Sl. No |
Parameters |
Rat groups |
Group I treated with NaCMC solution |
Group II treated with pristine ketoprofen |
Group III treated with T9 IPN microbeads |
Values are mean ± S.D. of six animals in each group. Score: 0: Nil; 1: Mild; 2: appreciable; 3: severe; 4: very severe. |
1 |
Ulcer number |
0 |
6.0 ± 0.9 |
2.0 ± 0.7 |
2 |
Ulcer size in mm |
0 |
1.97 ± 0.72 |
0.79 ± 0.45 |
3 |
Perforations |
0 |
2 |
1 |
4 |
Hemorrhage |
0 |
3 |
0 |
5 |
Congestion |
0 |
3 |
1 |
6 |
Oedema |
0 |
3 |
1 |
7 |
Necrosis |
0 |
3 |
0 |
8 |
Erosion of gastric mucosa |
0 |
4 |
1 |
Conclusion
The pH-sensitive PAam-g-TSP copolymer synthesized using microwave irradiation was successfully developed in the form of IPN microbeads and characterized by 1H-NMR, FTIR, elemental analysis and thermogravimetry. The pH-sensitive IPN microbeads were prepared using PAam-g-TSP copolymer with NaAlg by dual crosslinking method for controlled delivery of ketoprofen avoiding its release in stomach environment. The prepared microbeads exhibited pH-dependent swelling/shrinkage trends as the pH of external medium was altered. Large quantity of drug release was observed in buffer media of pH 7.4 (about 83%), while the release was quite small in pH 1.2 media (17%). The in vivo pharmacokinetics, anti-inflammatory activity and stomach histopathology studies performed on Wistar rats revealed successful delivery of ketoprofen to the intestine, resulting in reduced adverse effects in the stomach.
Abbreviations
FTIR | Fourier transform infrared |
1H-NMR | Proton nuclear magnetic resonance |
PAam-g-TSP | Polyacrylamide-grafted-tamarind seed polysaccharide |
IPN | Interpenetrating polymer network |
PAAm | Polyacrylamide |
TSP | Tamarind seed polysaccharide |
NaAlg | Sodium alginate |
NSAID | Non-steroidal anti-inflammatory drug |
GIT | Gastrointestinal tract |
Aam | Acrylamide |
CAN | Ceric ammonium nitrate |
TMS | Tetramethylsilane |
DMSO | Dimethylsulfoxide |
GA | Glutaraldehyde |
SEM | Scanning electron microscopy |
DEE | Drug encapsulation efficiency |
TGA | Thermogravimetric analysis |
DSC | Differential scanning calorimetry |
XRD | X-ray diffraction |
NaCMC | Sodium carboxymethylcellulose |
Acknowledgements
The financial support by Vision Group on Science and Technology, Government of Karnataka, India under K-FIST (L2) programme 2012–13 to the Department of Pharmaceutical Technology is highly appreciated. We thank Dr B. G. Mulimani, Hon'ble Vice-chancellor, BLDE University, Management of BLDE Association, Vijayapur and College of Pharmaceutical Sciences, Manipal University, Manipal for providing facilities to carry out this work.
References
- Y. Qiu and K. Park, Environment-sensitive hydrogels for drug delivery, Adv. Drug Delivery Rev., 2001, 53, 321–339 CrossRef CAS PubMed.
- T. R. Bhardwaj, M. Kanwar, R. Lal and A. Gupta, Natural gums and modified natural gums as sustained-release carriers, Drug Dev. Ind. Pharm., 2000, 26, 1025–1038 CrossRef CAS PubMed.
- K. S. Soppimath, T. M. Aminabhavi, A. M. Dave, S. G. Kumbar and W. E. Rudzinski, Stimulus responsive “smart” hydrogels as novel drug delivery systems, Drug Dev. Ind. Pharm., 2002, 28, 957–974 CrossRef CAS PubMed.
- V. D. Athawale and S. C. Rathi, Graft polymerization: starch as a model substrate, J. Macromol. Sci., Rev. Macromol. Chem. Phys., 1999, 39, 445–480 CrossRef.
- M. J. Zohuriaan-Mehr, Advances in chitin and chitosan modification through graft copolymerization: A comprehensive review, Iran. Polym. J., 2005, 14, 235–265 CAS.
- M. D. Kurkuri, S. G. Kumbar and T. M. Aminabhavi, Synthesis and characterization of polyacrylamide-grafted-sodium alginate copolymeric membranes and their use in pervaporation separation of water and tetrahydrofuran mixtures, J. Appl. Polym. Sci., 2002, 86, 272–281 CrossRef CAS.
- H. Kaur and R. R. Chatterjee, Interpenetrating hydrogel networks. 2. swelling and mechanical properties of the gelatin-polyacrylamide interpenetrating networks, Macromolecules, 1990, 23, 4868–4871 CrossRef CAS.
- S. K. Rath and R. P. Singh, On the characterization of grafted and ungrafted starch, amylose, and amylopectin, J. Appl. Polym. Sci., 1998, 70, 1795–1810 CrossRef CAS.
- S. A. Agnihotri and T. M. Aminabhavi, Novel interpenetrating network chitosan-poly(ethylene oxide-g-acrylamide) hydrogel microspheres for the controlled release of capecitabine, Int. J. Pharm., 2006, 324, 103–115 CrossRef CAS PubMed.
- T. T. Hsieh, K. H. Hsieh, G. P. Simon and C. Jiu, Polymer networks of 2-hydroxyethyl methacrylate-terminated polyurethane and polyurethane, Polymer, 1999, 40, 3153–3163 CrossRef CAS.
- J. D. Kosmala, D. B. Henthorn and L. B. Peppas, Preparation of interpenetrating networks of gelatin and dextran as degradable biomaterials, Biomaterials, 2000, 21, 2019–2023 CrossRef CAS PubMed.
- A. P. Rokhade, N. B. Shelke, S. A. Ptil and T. M. Aminabhavi, Novel interpenetrating polymer network microspheres of chitosan and methylcellulose for controlled release of theophylline, Carbohydr. Polym., 2007, 69, 678–687 CrossRef CAS.
- S. A. Agnihotri and T. M. Aminabhavi, Development of novel interpenetrating network gellan gum-poly(vinyl alcohol) hydrogel microspheres for the controlled release of carvedilol, Drug Dev. Ind. Pharm., 2005, 31, 491–503 CrossRef CAS PubMed.
- M. J. Gidley, P. J. Lillford, D. W. Rowlands, P. Lang, M. Dentini, V. Crescenzi, M. Edwards, C. Fanutti and J. S. G. Reid, Structure and solution properties of tamarind seed polysaccharide, Carbohydr. Res., 1991, 214, 299–314 CrossRef CAS PubMed.
- R. Sahoo, S. Sahoo and P. L. Nayak, Release behavior of anticancer drug paclitaxel from tamarind seed polysaccharide galactoxyloglucan, Eur. J. Sci. Res., 2010, 47, 197–206 Search PubMed.
- J. Zhang, X. Shengjun, Z. Shengtang and D. Zhaoli, Preparation and characterization of tamarind gum/sodium alginate composite gel beads, Iran. Polym. J., 2008, 17, 899–906 CAS.
- M. A. Masuelli and C. O. Illanes, Review of the characterization of sodium alginate by intrinsic viscosity measurements. Comparative analysis between conventional and single point methods, Int. J. Biomater. Res. Eng., 2014, 1, 1–11 Search PubMed.
- A. Blandino, M. Macias and D. Cantero, Glucose oxidase release from calcium alginate gel capsules, Enzyme Microb. Technol., 2000, 27, 319–324 CrossRef CAS PubMed.
- C. Bregni, J. Degrossi, R. Garcia, M. C. Lamas, R. Firenstein and M. D'Aquino, Alginate microspheres of Bacillus subtilis, Ars Pharm., 2000, 41, 245–320 CAS.
- G. F. Palmieri, G. Bonacucina, P. Martino and S. Martelli, J. Microencapsulation, 2002, 19, 111–119 CrossRef CAS PubMed.
- R. V. Kulkarni, R. Boppana, G. Krishna Mohan, S. Mutalik and N. V. Kalyane, pH-responsive interpenetrating network hydrogel beads of poly(acrylamide)-g-carrageenan and sodium alginate for intestinal targeted drug delivery: Synthesis, in vitro and in vivo evaluation, J. Colloid Interface Sci., 2012, 367, 509–517 CrossRef CAS PubMed.
- R. Boppana, G. Krishna Mohan, U. Nayak, S. Mutalik, B. Sa and R. V. Kulkarni, Novel pH-sensitive IPNs of polyacrylamide-g-gum ghatti and sodium alginate for gastro-protective drug delivery, Int. J. Biol. Macromol., 2015, 75, 133–143 CrossRef CAS PubMed.
- R. V. Kulkarni and B. Sa, Evaluation of pH-sensitivity and drug release characteristics of (Polyacrylamide-grafted-Xanthan)–carboxymethyl cellulose-based pH-sensitive interpenetrating network hydrogel beads, Drug Dev. Ind. Pharm., 2008, 34, 1406–1414 CrossRef CAS PubMed.
- R. V. Kulkarni and B. Sa, Novel pH-sensitive interpenetrating network hydrogel beads of carboxymethylcellulose–(Polyacrylamide-grafted-Alginate) for controlled release of ketoprofen: Preparation and characterization, Curr. Drug Delivery, 2008, 5, 256–264 CrossRef CAS.
- R. V. Kulkarni, S. Mutalik, B. S. Mangond and U. Y. Nayak, Novel interpenetrated polymer network microbeads of natural polysaccharides for modified release of water soluble drug: In-vitro and in-vivo evaluation, J. Pharm. Pharmacol., 2012, 64, 530–540 CrossRef CAS PubMed.
- P. Rani, G. Sen, S. Mishra and U. Jha, Microwave assisted synthesis of polyacrylamide grafted gum ghatti and its application as flocculant, Carbohydr. Polym., 2012, 89, 275–281 CrossRef CAS PubMed.
- M. N. Ghosh, Fundamentals of Experimental Pharmacology, Hilton and Company, India, 2005, p. 192 Search PubMed.
- C. A. Winter, E. A. Risley and G. W. Nuss, Carrageenin-induced edema in hind paw of the rat as an assay for anti-iflammatory drugs, Proc. Soc. Exp. Biol. Med., 1962, 111, 544–547 CrossRef CAS PubMed.
- B. Arica, S. Calis, P. Atilla, N. T. Durlu and N. Caker, In vitro and in vivo studies of ibuprofen-loaded biodegradable alginate beads, J. Microencapsulation, 2005, 22, 153–165 CrossRef CAS PubMed.
- O. Demchenko, T. Zheltonozhskaya, A. Turov, M. Tsapka and V. Syromyatnikov, Poly(vinyl alcohol)-graft-polyacrylamide with different grafts number and length as studied by 1H-NMR spectroscopy, Mol. Cryst. Liq. Cryst., 2005, 427, 225–233 Search PubMed.
- A. Liu, S. Mao, M. Liu, Y. Zhang, F. Wu, M. Li, E. Wang, G. Cheng and Y. Du, 1H NMR study on microstructure of a novel acrylamide/methacrylic acid template copolymer in aqueous solution, Colloid Polym. Sci., 2007, 285, 381–388 CAS.
- A. R. Madgulkar, M. R. Bhalekar, K. D. Asgaonkar and A. A. Dikpati, Synthesis and characterization of a novel mucoadhesive derivative of xyloglucan, Carbohydr. Polym., 2016, 135, 356–362 CrossRef CAS PubMed.
- A. Real, D. Wallander, A. Maciel, G. Cedillo and H. Zoza, Graft copolymerization of ethyl acrylate onto tamarind kernel powder and evaluation of its biodegradability, Carbohydr. Polym., 2015, 117, 11–18 CrossRef PubMed.
- Y. Kaneko, S. Sato, J. Kadokawa and N. Iyi, Synthesis of organic-inorganic hybrid hydrogels using rodlike polysiloxane having acrylamido groups as a new cross-linking agent, J. Mater. Chem., 2006, 16, 1746–1750 RSC.
- R. Boppana, R. V. Kulkarni, S. S. Mutalik, C. Mallikarjun Setty and B. Sa, Interpenetrating network hydrogel beads of carboxymethylcellulose and egg albumin for controlled release of lipid lowering drug, J. Microencapsulation, 2010, 27, 337–344 CrossRef CAS PubMed.
- R. V. Kulkarni, V. V. Nagathan, P. R. Biradar and A. A. Naikawadi, Simvastatin loaded composite polyspheres of gellan gum and carrageenan: In vitro and in vivo evaluation, Int. J. Biol. Macromol., 2013, 57, 238–244 CrossRef CAS PubMed.
- C. X. Liang and K. Hirabayashi, Improvements of the physical-properties of fibroin membranes with sodium alginate, J. Appl. Polym. Sci., 1992, 45, 1937–1943 CrossRef CAS.
- K. S. Soppimath and T. M. Aminabhavi, Water transpost and drug release study from cross-linked polyacrylamide grafted guar gum hydrogel microspheres for the controlled release application, Eur. J. Pharm. Biopharm., 2002, 53, 87–98 CrossRef.
- H. Eerikainen, L. Peltonen, J. Raula, J. Hirvonen and E. I. Kauppinen, Nanoparticles containing ketoprofen and acrylic polymers prepared by an aerosol flow reactor method, AAPS PharmSciTech, 2004, 5, 68 CrossRef PubMed.
- S. Tamilvanan and B. Sa, Studies on in vitro release behavior of indomethacin-loaded polystyrene microparticles, Int. J. Pharm., 2000, 201, 187–197 CrossRef CAS PubMed.
- A. P. Rokhade, S. A. Agnihotri, S. A. Patil, N. N. Mallikarjuna, P. V. Kulkarni and T. M. Aminabhavi, Semi-interpenetrating polymer network microspheres of gelatin and sodium carboxymethyl cellulose for controlled release of ketorolac tromethamine, Carbohydr. Polym., 2006, 65, 243–252 CrossRef CAS.
- K. S. Soppimath, A. R. Kulkarni and T. M. Aminabhavi, Chemically modified Polyacrylamide-g-guar gum based cross-linked anionic microgels as pH-sensitive drug delivery systems: Preparation and characterization, J. Controlled Release, 2001, 75, 331–345 CrossRef CAS PubMed.
- K. Chaturvedi, K. Ganguli, A. R. Kulkarni, M. N. Nadagouda, J. Stowbridge, W. E. Rudzinski and T. M. Aminabhavi, Ultrasmall fluorescent bile acid conjugated PHB-PEG block copolymeric nanoparticles: Synthesis, characterization and cellular uptake, RSC Adv., 2013, 3, 7064–7070 RSC.
- R. V. Kulkarni, F. S. Patel, H. M. Nanjappaiah and A. A. Naikawadi, In vitro and in vivo evaluation of novel interpenetrated polymer network microparticles containing repaglinide, Int. J. Biol. Macromol., 2014, 69, 514–522 CrossRef CAS PubMed.
- P. L. Ritger and N. A. Peppas, A simple equation for description of solute release. II. Fickian and anomalous release from swellable devices, J. Controlled Release, 1987, 5, 37–42 CrossRef CAS.
- A. E.-G. H. Abd El-Gawad, E. M. Ramadan, O. A. Soliman and R. M. Yusif, Formulation and in-vivo study of ketoprofen tablets Prepared using chitosan interpolymer complexes, Bull. Pharm. Sci., Assiut Univ., 2012, 35, 1–16 CAS.
- R. V. Kulkarni and B. Sa, Enteric delivery of ketoprofen through functionally modified poly(acrylamide-grafted-xanthan)-based pH-sensitive hydrogel beads: Preparation, in vitro and in vivo evaluation, J. Drug Targeting, 2008, 16, 167–177 CrossRef CAS PubMed.
- R. C. Mundargi, S. A. Patil, S. A. Agnihotri and T. M. Aminabhavi, Development of polysaccharide-based colon targeted drug delivery system for the treatment of amoebiasis, Drug Dev. Ind. Pharm., 2007, 33, 255–264 CrossRef CAS PubMed.
Footnote |
† Electronic supplementary information (ESI) available. See DOI: 10.1039/c6ra04218j |
|
This journal is © The Royal Society of Chemistry 2016 |