DOI:
10.1039/C6RA03103J
(Paper)
RSC Adv., 2016,
6, 38430-38436
Efficient removal of Cd(II) ions from aqueous solutions via visible capturing
Received
2nd February 2016
, Accepted 9th April 2016
First published on 11th April 2016
Abstract
A new type of cotton fiber with double functions of visual detection and efficient adsorption of cadmium (Cd(II)) ions in aqueous solutions was successfully prepared via the surface immobilization of 5,10,15,20-tetrakis(1-methyl-4-pyridinio)porphyrin tetra(p-toluenesulfonate) (TPTP). The prepared multi-functional cotton fibers (denoted as C-TPTP fiber) were able to exhibit efficient capture ability for Cd(II) ions with obvious color changes, which confirmed the sensitive detection capability for Cd(II) in the adsorption process. The pH effect, the adsorption kinetics and isotherms of the C-TPTP fiber were also investigated, together with the corresponding color detections for the Cd(II) adsorption performances. The results showed that the C-TPTP fiber reached adsorption equilibrium within 1 hour with a maximum adsorption capacity of 0.4265 mmol g−1 as determined from the Langmuir isotherm model. The color of the C-TPTP fiber changed from brownish to dark green with the increase of the Cd(II) adsorption capacity. The C-TPTP fiber prepared in this paper shows great potential for the early warning and efficient removal of heavy metal ions.
1. Introduction
Heavy metal pollution in water bodies has posed a great threat to human health and ecological safety.1,2 Among these heavy metals, cadmium (Cd) mainly originated from the effluents discharged by electroplating, metallurgy and battery industries, and has been listed as a priority pollutant by the USA Environmental Protection Agency.3 Cadmium ions as a teratogenic and carcinogenic substance can accumulate in the human body and cause renal dysfunction, osteomalacia, fertility damage and other diseases.4,5 Since the presence of cadmium ions does not significantly affect the color change of the water body, it is quite difficult to detect the cadmium ions by visual inspection. Therefore, it is quite necessary and promising to develop a type of adsorptive material that can not only identify the presence of cadmium ions in water, but also effectively remove them from water.
The available methods for the removal of heavy metal ions mainly include chemical precipitation, electrochemical treatment, reverse osmosis, adsorption, etc.6–8 The adsorption has been considered as one of the most efficient methods with many advantages including low cost, high efficiency, good selectivity and recyclability. As the adsorption media, adsorbents play an important role and can be prepared with various materials. The biological materials are often used as the substrate materials of the adsorbents which are capable of adsorbing copper, lead, mercury and other heavy metal ions in the water body.9 Among various biological materials, cotton fiber is a very promising adsorbent substrate since it is a natural polymer that is widely found in nature, cheap and readily available. The hydroxyl groups on the surface of the cotton fiber provide active sites for the grafting of various functional groups capable of adsorbing heavy metal ions, which can greatly improve the adsorption performance of the cotton fiber. For instance, Zheng et al. fabricated amino functionalized cotton fiber with the excellent adsorption capacity of 12.73 mg g−1 for Cd(II) ions.10 In view of the excellent surface modification property of the cotton fiber, a growing number of researchers have been engaged in the study of the cotton fiber as a practical adsorbent.
In recent years, rapid in situ detection of heavy metal ions in water or wastewater has aroused great interest among researchers. The study of the optical sensor is particularly attractive, since it has unique advantages over other detection methods, e.g. fluorescence analysis system,11 and it can facilitate easy and rapid identification of heavy metal ions in water body through color change without complex operations or equipment. Optical sensors can be fixed on various substrates.12 Some essays have already reported that diphenyl carbazion, dithizone and tetraphenylporphyrin tetrasulfonic acid were grafted onto the porous monolithic silicon to realize the detection of Pb2+, Cr6+ and Hg2+ respectively.13,14 These studies indicated that it is quite promising to fix the organic optical sensor groups on the matrix material for the fast detection of heavy metal ions in the water body. Among various optical sensors, porphyrin as a synthetic dye possesses the spatial structure exactly accessible for cadmium ions to form metal complex, which can efficiently absorb specific wavelength of the visible light and then present proper color change.15 As a result, the appropriate substrate materials anchored with porphyrin indicator can be used for the in situ detection of Cd(II) in aqueous solution.
Heavy metal adsorption and detection are in constant development, and the combination of these two techniques is the future trend to fulfill the demands of industrial applications. In this paper, the combination of these two techniques was realized by anchoring porphyrin molecules onto the cotton fiber substrate to prepare a multi-functional cotton fiber (C-TPTP fiber). To the best of knowledge, such combination has not been reported so far, and the prepared C-TPTP fiber was able to be applied for the detection and adsorption of Cd(II) ions. The Cd(II) ion adsorption performances and the visible color changes of the C-TPTP fiber under various adsorption conditions were investigated. This study would provide more insight into the preparation of multi-functional adsorbents for the early warning and efficient removal of heavy metal ions.
2. Experimental
2.1 Materials
Cotton fibers were provided by Qingbai Plastic Products Factory. 2-Bromoisobutyryl bromide (BIBB, 98%), cadmium nitrate tetrahydrate (99%) and cadmium nitrate standard solution (AR) were purchased from Shanghai Aladdin Reagent Co., Ltd. 5,10,15,20-Tetrakis(1-methyl-4-pyridinio)porphyrin tetra(p-toluenesulfonate) (hereinafter referred to as TPTP) was purchased from Sigma-Aldrich Chemical Reagent Co., Ltd. Dichloromethane (DCM, AR), sodium hydroxide (NaOH, AR), hydrochloric acid (HCl, AR), acetone (AR) and methanol (99.9%) were purchased from Tianjin Baishi Chemical Industry Co., Ltd.
2.2 Immobilization of BIBB
First, 0.3 g of cotton fibers and 15 mL of DCM were added into a test tube, which was then placed into an ice-water bath. Afterwards, 3 mL of BIBB was slowly pipetted into the test tube, followed by 1 mL of pyridine. Then the test tube was placed onto a magnetic stirrer and the reaction mixture was stirred at the rotation speed of 300 rpm for 24 h at 20 °C. After the completion of the reaction, the brominated cotton (denoted as C–Br) was taken out, repeatedly rinsed with deionized water, soaked in acetone until it turned white, and finally dried with argon and stored in a dry box for future use.
2.3 Immobilization of TPTP on C–Br fiber
C–Br was soaked in 7 mg L−1 of TPTP solution with the solid–liquid ratio of 1
:
1. The reaction was carried out for 12 h at the rotation speed of 200 rpm at 20 °C. After completion of the reaction, the modified cotton fiber was taken out and repeatedly rinsed with deionized water until the absorbance of the TPTP in the washing liquid cannot be detected by the UV-visible spectrophotometer (UV-3200, Mapada, Shanghai). Then the modified cotton fiber (denoted as C-TPTP) was dried in vacuum and stored in a dry box for future use. The anchored TPTP amount on C–Br fiber was calculated according to the changed concentrations of TPTP solution before and after reaction, which were measured by the UV-visible spectrophotometer.
2.4 Surface characterization
The chemical composition of the cotton fiber samples were analyzed using an Infrared Spectrometer (FTIR-8300PCS, Shimadzu, Japan). The surface morphologies of the cotton fiber samples were observed using a Scanning Electron Microscope (SEM) (S-3400N (II), Hitachi, Japan). The surface element recognition and the corresponding atomic percentage for each element were examined using X-ray Photoelectron Spectroscopy (XPS, Thermo ESCALAB 250Xi, USA).
2.5 Batch adsorption test
Adsorption experiments were carried out to determine the pH effect, adsorption kinetics and adsorption isotherms of the C-TPTP during the Cd(II) adsorption process. The Cd(II) ion concentrations in the solutions before and after the adsorption process were measured by the Inductively Coupled Plasma Optical Emission Spectrometer (ICP-OES, Optima 2100DV, PerkinElmer). The Cd(II) adsorption capacity was calculated according to eqn (1): |
 | (1) |
where q (mmol g−1) is the adsorption capacity, C0 and C (mmol L−1) are the Cd(II) initial and final concentrations in solution, respectively, V (L) is the volume of Cd(II) ion solution, and m (g) is the weight of the C-TPTP fiber. The color changes of the C-TPTP samples after Cd(II) ion adsorption were recorded by taking photographs under specific adsorption conditions.
For the investigation of pH effect, 7 C-TPTP samples (20 mg each) were placed in 20 mL of 4 mM cadmium nitrate solutions at pH values of 1–7, respectively. Cd(II) ion (in Cd(NO3)2 form) is the predominant species in this pH range studied in 4 mM Cd(NO3)2 solutions, as determined by MINEQL+ software.16 The adsorption experiments were allowed for 12 h at 20 °C (adsorption period far more than that to reach the adsorption equilibrium).
For the investigation of the adsorption kinetics, 500 mg of C-TPTP was placed in 500 mL of 4 mM cadmium nitrate solution at pH 7. The adsorption was carried out at the rotation speed of 150 rpm at 20 °C. Samples were taken at specific time intervals during the adsorption process. The concentrations of the samples were determined by ICP-OES.
For the investigation of the adsorption isotherm, C-TPTP samples (20 mg each) were placed into a series of 20 mL cadmium nitrate solutions with concentrations ranging from 0.2 mM to 4.0 mM at pH 7. The adsorption was allowed for 12 hours at the rotation speed of 150 rpm at 20 °C.
3. Results and discussion
3.1 Functionalization of the cotton fiber
The schematic diagram of the surface functionalization of the cotton fiber is shown in Fig. 1. The matrix of the cotton fiber is mainly cellulose, possessing a large number of hydroxyl groups, which can be esterified by BIBB to produce the C–Br fibers. Based on the relatively strong reaction activity of C–Br bond of the C–Br fibers, TPTP can be immobilized onto the surface of C–Br fiber to produce C-TPTP fiber, by the formation of the C–N covalent bond from the reaction of the C–Br bond of C–Br fiber with the N–H bond of TPTP, releasing the molecule of HBr. With the advantage of the color change during porphyrin–Cd(II) ion complexation, C-TPTP fiber is able to detect the Cd(II) ions and remove them from aqueous solutions. The specific removal principle for Cd(II) ions is shown in Fig. 1B, where one porphyrin molecule on C-TPTP chelates one Cd(II) ion in its cavity, and the C-TPTP fiber exhibits green color based on this complexation reaction. Therefore, the C-TPTP fiber possesses dual function and can be used for the detection and adsorption of Cd(II) ions.
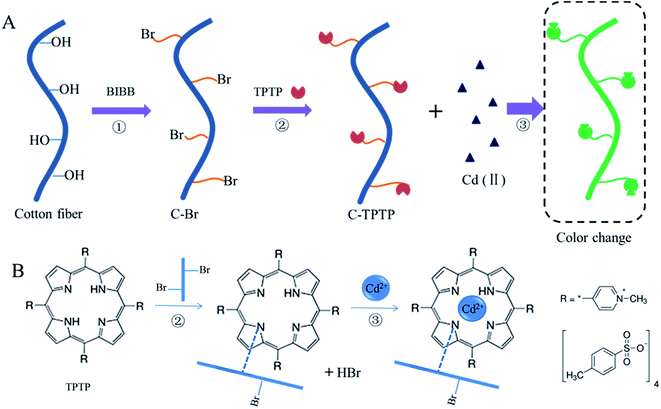 |
| Fig. 1 Schematic diagram of (A) experimental process, and (B) mechanism of immobilization of TPTP and adsorption of Cd(II) ions. | |
3.2 Characterization of cotton fibers
FTIR spectra were used to characterize the chemical composition of cotton fiber before and after modification. As shown in Fig. 2, the new peak at 1730 cm−1 was attributed to the ester bond (–COO–), which belongs to the esterification reaction between BIBB and hydroxyl groups of the pristine cotton fiber.15 The new peak at 1580 cm−1 was characteristic of the bending vibration of the secondary amine, which should be attributed to the porphyrin molecules. The peaks at 1090 cm−1 and 560 cm−1 were the characteristic absorption peaks of R–SO3− groups, which is part of the toluenesulfonate groups of TPTP.15 These characteristic peaks proved that porphyrin was successfully immobilized onto the surface of the cotton fiber.
 |
| Fig. 2 FTIR spectra of the pristine cotton fiber and C-TPTP fiber. | |
Fig. 3 showed the XPS spectra of the pristine and the modified cotton fibers, and the specific atomic percentages (denoted as atomic%) for each element were listed in Table 1. The pristine cotton fiber was mainly composed of carbon and oxygen elements. The Br element (4.77%) appeared in the spectrum of C–Br fiber, suggesting the BIBB was successfully immobilized on the fiber surface. For the spectrum of C-TPTP fiber, the content of Br decreased to 1.48% as compared to C–Br fiber. On the other hand, the N and S elements belonging to TPTP appeared on the C-TPTP fiber surface. These results indicated that TPTP was successfully immobilized onto the cotton fiber surface by the formation of C–N bond and the release of the HBr molecule, as shown in Fig. 1. According to the results of UV measurement, the amount of anchored TPTP on the cotton fibers was 6.78 mg g−1.
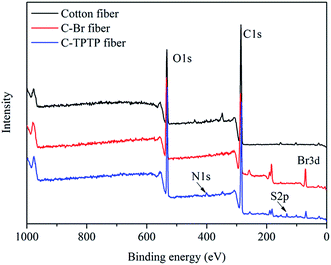 |
| Fig. 3 XPS spectra of pristine and modified cotton fibers. | |
Table 1 Surface element composition (in atomic percentage) of all the cotton fibers as derived from the XPS characterization results
Element |
Atomic% |
Pristine cotton fiber |
C–Br fiber |
C-TPTP fiber |
C |
80.17 |
69.70 |
69.69 |
O |
19.73 |
25.53 |
25.55 |
Br |
0 |
4.77 |
1.48 |
N |
0 |
0 |
2.61 |
S |
0 |
0 |
0.59 |
The surface morphologies of cotton fiber before and after modification were shown in Fig. 4. The pristine cotton fiber was flat-ribbon type with a smooth surface. After the immobilization of TPTP, it seems that a rough layer was covered on the C-TPTP fiber surface. It should be noted that the form of fibers had not been changed during the modification process.
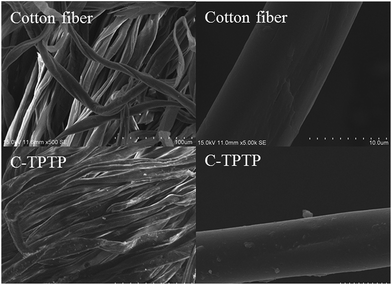 |
| Fig. 4 SEM images of the pristine cotton fiber and C-TPTP fiber. | |
3.3 pH effect
The pH effect was investigated for the adsorption of Cd(II) ions with C-TPTP fibers, shown in Fig. 5. It can be seen that the Cd(II) ion adsorption capacity of the C-TPTP fiber increased with the increase of the pH values. Furthermore, the color of C-TPTP fiber gradually turned from the initial brownish to dark green, with the increase of the pH values. Therefore, the color change of the C-TPTP fiber showed a direct correlation with the adsorption capacity. In other words, the more amount of Cd(II) ions adsorbed, the more dark green observed.17 The pH effect on the adsorption capacity should be attributed to the extent of protonated porphyrin at different pH values.18 The secondary amine groups would be protonated to gain positive charges in the acidic environment, exhibiting the exclusion effect to heavy metal cations due to the electrostatic repulsive force. In contrast, porphyrin molecules would show lower degree of protonation with strong chelating effect to cadmium ions in the relatively neutral environment, exhibiting a better chelation effect to Cd(II) ions in aqueous solution. Therefore, higher pH values favor the adsorption of Cd(II) ions on C-TPTP fibers with darker green colors.
 |
| Fig. 5 Adsorption of Cd(II) onto C-TPTP fibers at different pH values (the inserted pictures are digital photos of C-TPTP fibers after the adsorption of Cd(II) ions at different pHs). | |
3.4 Adsorption kinetics
Fig. 6 showed the Cd(II) ion adsorption kinetic behaviors of the pristine cotton fiber, C–Br fiber and C-TPTP fiber. It can be seen that there was almost no adsorption of Cd(II) ions on the pristine cotton fiber and the C–Br fiber. In contrast, the C-TPTP fiber exhibited an enhanced adsorption performance to Cd(II) ions. The Cd(II) ion adsorption kinetics of C-TPTP showed a rapid increase in the initial 20 minutes, followed by the steady increase, and finally reached equilibrium within 60 minutes. The adsorption of Cd(II) ions was mainly due to the porphyrin functional groups immobilized on the cotton fiber surface. It should be noticed that the color of C-TPTP fiber showed a gradual change during the adsorption process, and finally exhibited dark green at the adsorption equilibrium based on the complexation of the Cd(II) ion with porphyrin.15 The Cd(II) ion adsorption kinetics of the C-TPTP fiber was further fitted with Pseudo-First-Order (PFO) and Pseudo-Second-Order (PSO) kinetic models,19,20 with the relevant model equations and the calculated parameters shown in Table 2. It can be seen from the fitting results in Table 2 that PSO model gave much better fit to the adsorption kinetics as compared with the PFO model, with the R2 value of 0.9988. The equilibrium adsorption capacity (qe) derived from the PSO model was 0.3703 mmol g−1, which was also closer to the experimental value (0.3380 mmol g−1) listed in Fig. 6.
 |
| Fig. 6 Cd(II) adsorption kinetics of the pristine cotton fiber, C–Br fiber and C-TPTP fiber (the inserted pictures are digital photos of C-TPTP fibers at different time intervals during the Cd(II) ion adsorption process). | |
Table 2 Equations and parameters of the adsorption kinetics models fitted to the Cd(II) ion adsorption kinetics with C-TPTP fibera
Kinetics model |
Model equation |
Model parameters |
Parameter values |
qt (mmol g−1) and qe (mmol g−1) are the adsorption capacity at time t (min) and at adsorption equilibrium, respectively. k1 (min−1) and k2 (g mmol−1 min−1) are the rate constants of the PFO and PSO models, respectively. R2 is the correlation coefficient for the relevant kinetics model equations. |
PFO |
ln(qe − qt) = ln qe − k1t |
k1 (min−1) |
0.0427 |
qe (mmol g−1) |
0.2078 |
R2 |
0.9306 |
PSO |
t/qt = 1/(k2qe2) + t/qe |
k2 (g mmol−1 min−1) |
0.4962 |
qe (mmol g−1) |
0.3703 |
R2 |
0.9988 |
3.5 Adsorption isotherms
The Cd(II) ion adsorption isotherms of the C-TPTP fiber were examined and the results were shown in Fig. 7. The adsorption isotherm showed a steady increase with the increase of the Cd(II) ion equilibrium concentrations, and finally reached the plateau when the Cd(II) ion equilibrium concentration was greater than 3 mmol L−1. The C-TPTP fibers exhibited different colors at different adsorption equilibrium stages, due to the different adsorption capacity of the Cd(II) ions, and the colors changed gradually from the initial brownish to dark green. Therefore, the C-TPTP fibers with different colors can be used as the indicator to quantify the cadmium ion concentrations in the feed solution.
 |
| Fig. 7 Cd(II) ion adsorption isotherms of the C-TPTP fiber (the inserted pictures are digital photos of C-TPTP fibers at Cd(II) ion adsorption equilibrium from solutions with different initial Cd(II) ion concentrations). | |
The Cd(II) ion adsorption isotherms were fitted with Langmuir and Freundlich isotherm model,21,22 with the model equations and the calculated parameters shown in Table 3. It can be inferred that the Langmuir adsorption isotherm model gave a better fit to the Cd(II) ion adsorption isotherm of C-TPTP fibers with the R2 value of about 0.99, suggesting a monolayer adsorption on C-TPTP fibers. These results were consistent with the surface structure of the C-TPTP fiber, as the porphyrin molecules immobilized covered the cotton fiber surface in a monolayer form to capture the Cd(II) ions, so that one Cd(II) ion chelated with one porphyrin molecule in its cavity in the monolayer. In addition, the maximum adsorption capacity was 0.4265 mmol g−1 calculated from Langmuir model, and this removal efficiency of C-TPTP is higher than that of the reported adsorbents listed in Table 4. Therefore, C-TPTP fibers could be used as an efficient adsorbent for the removal and detection of cadmium ions from aqueous solution.
Table 3 Equations and parameters of the Langmuir and Freundlich models fitted to Cd(II) ion adsorption isotherm with C-TPTP fibersa
Langmuir model |
Freundlich model |
For Langmuir model, qe (mmol g−1) and qm (mmol g−1) are the equilibrium and maximum adsorption capacity, respectively. Ce (mmol L−1) is the equilibrium concentration, and KL (L mmol−1) is a Langmuir model constant. For the Freundlich model, qe and Ce have the same definitions as those of the Langmuir model, KF (mmol1−n Ln g−1) and n are Freundlich constants, which are related to the adsorbent capacity and the adsorption intensity of the adsorbent, respectively. |
 |
qe = KFCen |
qm (mmol g−1) |
KL (L mmol−1) |
R2 |
n |
KF (mmol1−n Ln g−1) |
R2 |
0.4265 |
1.7810 |
0.9894 |
0.2844 |
0.2641 |
0.9230 |
Table 4 Comparison of the Cd(II) adsorption amount of C-TPTP with that of the other adsorbents
Materials |
pH |
The maximum adsorption amount of Cd2+ (mmol g−1) |
Reference |
Sulphuric acid-treated wheat bran |
5.4 |
0.3835 |
23 |
Rice hulls |
7 |
0.1900 |
24 |
Heat-treated bentonite |
7.9 |
0.1468 |
25 |
Sphagnum moss peat |
|
0.0516 |
26 |
Sporopollenin |
7 |
0.0146 |
27 |
Rice husk ash |
6 |
0.0552 |
28 |
Dead biomass |
6 |
0.1807 |
29 |
Olive cake |
4 |
0.0805 |
30 |
C-TPTP |
7 |
0.4265 |
This work |
4. Conclusions
In this paper, the cotton fiber was successfully immobilized with TPTP molecules by a convenient surface modification method. The surface modified cotton fiber (C-TPTP) was endowed with dual functions for the detection and adsorption of Cd(II) ions. The results showed that the adsorption was pH dependent, and the highest adsorption capacity was achieved at pH 7. The adsorption kinetics reached equilibrium within 60 min and was best described by the Pseudo-Second-Order (PSO) kinetics model. The adsorption isotherm was best fitted with the Langmuir model, with the calculated maximum adsorption capacity of 0.4265 mmol g−1. The obvious color change was observed from the initial brownish to dark green, with the increase of the adsorption capacity. The C-TPTP fiber reported in this study could be used as an efficient adsorbent for the removal of Cd(II) ions in aqueous solutions with visible indicator function.
Acknowledgements
This work was financially supported by the National Natural Science Foundation of China (21307083), and Shenzhen Science and Technology Foundations (JCYJ20140418095735550, KQCX20140519103908550, and JCYJ20150324140036861).
References
- S. Hasan, A. Krishnaiah, T. K. Ghosh, D. S. Viswanath, V. M. Boddu and E. D. Smith, Adsorption of divalent cadmium (Cd(II)) from aqueous solutions onto chitosan-coated perlite beads, Ind. Eng. Chem. Res., 2006, 45, 5066–5077 CrossRef CAS.
- T. K. Naiya, A. K. Bhattacharya and S. K. Das, Adsorptive removal of Cd(II) ions from aqueous solutions by rice husk ash, Environ. Prog. Sustainable Energy, 2009, 28, 535–546 CrossRef CAS.
- R. E. Cameron, in Guide to Site and Soil Description for Hazardous Waste Site Characterization: Metals, U.S. Environmental Protection Agency, Washington, DC, 1992, vol. 1 Search PubMed.
- G. L. Rorrer, T. Y. Hsien and J. D. Way, Synthesis of porous-magnetic chitosan beads for removal of cadmium ions from waste-water, Ind. Eng. Chem. Res., 1993, 32, 2170–2178 CrossRef CAS.
- W. S. Wan Ngah and M. A. K. M. Hanafiah, Removal of heavy metal ions from wastewater by chemically modified plant wastes as adsorbents: a review, Bioresour. Technol., 2008, 99, 3935–3948 CrossRef CAS PubMed.
- N. Kongsricharoern and C. Polprasert, Chromium removal by a bipolar electro-chemical precipitation process, Water Sci. Technol., 1996, 34, 109–116 CrossRef CAS.
- C. L. Lai and S. H. Lin, Electrocoagulation of chemical mechanical polishing (CMP) wastewater from semiconductor fabrication, Chem. Eng. J., 2003, 95, 205–211 CrossRef CAS.
- J. J. Qin, M. N. Wai, M. H. Oo and F. S. Wong, A feasibility study on the treatment and recycling of a wastewater from metal plating, J. Membr. Sci., 2002, 208, 213–221 CrossRef CAS.
- S. E. Bailey, T. J. Olin, R. M. Bricka and D. D. Adrian, A review of potentially low-cost sorbents for heavy metals, Water Res., 1999, 33, 2469–2479 CrossRef CAS.
- L. Zheng, Z. Dang, X. Yi and H. Zhang, Equilibrium and kinetic studies of adsorption of Cd(II) from aqueous solution using modified corn stalk, J. Hazard. Mater., 2010, 176, 650–656 CrossRef PubMed.
- I. Klimant and M. Otto, A fiber optical sensor for heavy-metal ions based on immobilized xylenol orange, Mikrochim. Acta, 1992, 108, 11–17 CrossRef CAS.
- S. A. El-Safty, A. A. Ismail, H. Matsunaga, T. Hanaoka and F. Mizukami, Optical nanoscale pool-on-surface design for control sensing recognition of multiple cations, Adv. Funct. Mater., 2008, 18, 1485–1500 CrossRef CAS.
- T. Balaji, S. A. El-Safty, H. Matsunaga, T. Hanaoka and F. Mizukami, Optical sensors based on nanostructured cage materials for the detection of toxic metal ions, Angew. Chem., Int. Ed., 2006, 45, 7202–7208 CrossRef CAS PubMed.
- A. A. Ensafi, A. K. Far and S. Meghdadi, Highly selective optical sensor for mercury assay based on covalent immobilization of 4-hydroxy salophen on a triacetyl-cellulose membrane, Sens. Actuators, B, 2008, 133, 84–90 CrossRef CAS.
- L. Zhang, Y. H. Zhao and R. Bai, Development of a multifunctional membrane for chromatic warning and enhanced adsorptive removal of heavy metal ions: application to cadmium, J. Membr. Sci., 2011, 379, 69–79 CrossRef CAS.
- W. D. Schecher and D. C. McAvoy, MINEQL+: Chemical Equilibrium Modeling System, Version 4.5 for Windows, Environmental Research Software, Hallowell, ME, 2003 Search PubMed.
- K. Kalyanasundaram, Photochemistry of Polypyridine and Porphyrin Complexes, Academic Press, Boston, 1992 Search PubMed.
- S. A. El-Safty, D. Prabhakaran, A. A. Ismail, H. Matsunaga and F. Mizukami, Nanosensor design packages: a smart and compact development for metal ions sensing responses, Adv. Funct. Mater., 2007, 17, 3731–3745 CrossRef CAS.
- Y. S. Ho and G. McKay, Pseudo-second order model for sorption processes, Process Biochem., 1999, 34, 451–465 CrossRef CAS.
- L. Zheng, Z. Dang, X. Yi and H. Zhang, Equilibrium and kinetic studies of adsorption of Cd(II) from aqueous solution using modified corn stalk, J. Hazard. Mater., 2010, 176, 650–656 CrossRef CAS PubMed.
- S. L. Luo, X. J. Li, L. Chen, J. L. Chen, Y. Wan and C. B. Liu, Layer-by-layer strategy for adsorption capacity fattening of endophytic bacterial biomass for highly effective removal of heavy metals, Chem. Eng. J., 2014, 239, 312–321 CrossRef CAS.
- S. L. Luo, X. L. Xu, G. Y. Zhou, C. B. Liu, Y. H. Tang and Y. T. Liu, Amino siloxane oligomer-linked graphene oxide as an efficient adsorbent for removal of Pb(II) from wastewater, J. Hazard. Mater., 2014, 274, 145–155 CrossRef CAS PubMed.
- A. Ozer and H. B. Pirincci, The adsorption of Cd(II) ions on sulphuric acid-treated wheat bran, J. Hazard. Mater., 2006, 137, 849–855 CrossRef CAS PubMed.
- D. Roy, P. N. Greenlaw and B. S. Shane, Adsorption of heavy metals by green algae and groundrice hulls, J. Environ. Sci. Health, Part A: Environ. Sci. Eng., 1993, 28, 37–50 Search PubMed.
- E. G. Pradas, M. V. Sanchez, F. C. Cruz, M. S. Viciana and M. F. Perez, Adsorption of cadmium and zinc from aqueous solution on natural and activated bentonite, J. Chem. Technol. Biotechnol., 1994, 59, 289–295 CrossRef.
- J. K. McLelland and C. A. Rock, Pretreating land-fill leachate with peat to remove metals, Water, Air, Soil Pollut., 1988, 379, 203–215 Search PubMed.
- N. Unlu and M. Ersoz, Adsorption characteristics of heavy metal ions onto a low cost biopolymeric sorbent from aqueous solutions, J. Hazard. Mater., 2006, 136, 272–280 CrossRef PubMed.
- V. C. Srivastava, I. D. Mall and I. M. Mishra, Characterization of mesoporous rice husk ash (RHA) and adsorption kinetics of metal ions from aqueous solution onto RHA, J. Hazard. Mater., 2006, 134, 257–267 CrossRef CAS PubMed.
- G. Yan and T. Viraraghavan, Heavy-metal removal from aqueous solution by fungus Mucor rouxii, Water Res., 2003, 37, 4486–4496 CrossRef CAS PubMed.
- S. Doyurum and A. C. Elik, Pb(II) and Cd(II) removal from aqueous solutions by olive cake, J. Hazard. Mater., 2006, 138, 22–28 CrossRef CAS PubMed.
|
This journal is © The Royal Society of Chemistry 2016 |
Click here to see how this site uses Cookies. View our privacy policy here.