DOI:
10.1039/C6RA01729K
(Paper)
RSC Adv., 2016,
6, 37136-37148
Synthesis of highly functionalized [3]dendralenes and their Diels–Alder reactions displaying unexpected regioselectivity†
Received
20th January 2016
, Accepted 17th March 2016
First published on 30th March 2016
Abstract
Acyclic tetrasubstituted [3]dendralenes stable towards D–A cyclodimerization were prepared by the double olefination of substituted dienyl phosphonates with dimethylsulfonium methylide, followed by H–W–E olefination of the formed butadienylphosphonoacetates with aldehydes. The X-ray structure of a [3]dendralene with a 4-position benzyl substitution revealed that an electron-rich diene exists in the s-trans conformation, leading to the stability. This structural preference of dendralenes leads to an unusual regioselectivity in the D–A reaction, wherein a deactivated diene participated in the process and displayed endo selectivity. The steric bulk of the groups at the 2 and 4 positions govern the regioselectivity and dominates the electronic effects. For the dendralenes possessing a 4-methyl substituent, the electronically rich diene participated in the D–A reaction. These D–A adducts failed to undergo further cycloaddition owing to steric crowding, which resulted in gauche conformation of the diene, as evidenced by X-ray structures of the adducts.
Introduction
Depending upon the type of atom connectivity (unbranched or branched, cyclic or acyclic), four fundamental hydrocarbon families of oligoalkene structures are possible, as presented in Fig. 1. Dendralenes are the first family of the branched oligoalkenes and feature cross-conjugation.1–6 They have not received proper attention in the literature to date, primarily due to their unpredictable stability and lability for Diels–Alder (D–A) cyclodimerization or oligomerization. Recently though, they have gained in popularity as they display unique properties different from normal oligoenes.7–16 This has created interest in various fields, such as polymer chemistry,7–10 electro chemistry,11–13 material chemistry14,15 and theoretical chemistry.16 For synthetic chemists, dendralenes are fascinating molecules because they possess a huge potential, which can be harnessed by subjecting them to tandem D–A reactions, also known as diene-transmissive D–A (DTDA)17 sequences, for the quick generation of complex multicyclic scaffolds from simple acyclic substrates in an atom economical manner. Also, several bioactive natural products18–21 possess dendralenes as their core structures.
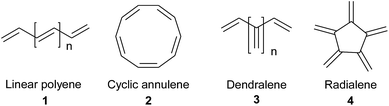 |
| Fig. 1 Fundamental classes of alkenic hydrocarbons. | |
Early synthetic methods for dendralenes comprised various classical approaches for olefin synthesis, namely pyrolysis,22–24 Mitsunobu dehydration,25 β-elimination26 and the thermal decomposition of vinyl sulfolenes,27,28 which often involved harsh reaction conditions. Of late, many groups have reported the synthesis of dendralenes mediated by various transition metals.29–47 The majority of these methods lead to unsubstituted or scantly substituted dendralenes. Some of the approaches lead to [3]dendralenes functionalized in cyclic systems.34–36 Only a handful of methods yield amply substituted acyclic [3]dendralenes, such as the dimerization of allenes,37,38 elimination of 1,3-dienic allylic carbonates39 and enyne metathesis.40–42
Earlier we reported48 a novel olefination methodology using Corey–Chaykovsky dimethylsulfonium methylide (DMSM) 5 (ref. 49) with various activated olefins that provided interesting products.50–57 Later, this methodology was augmented for the sequential double olefination of vinyl phosphonates to provide substituted 1,3-dienes with high regioselectivity and stereoselectivity.51,52 Subsequently, we employed this methodology for the intended synthesis of 1,5-diaryl-2-alkoxycarbonyl [3]dendralenes 6 from dienyl phosphonates 7 via a 1,3-butadien-2-ylphosphonoacetate intermediate 8, as depicted in Scheme 1.58 Unfortunately, the desired [3]dendralenes could not be isolated owing to their high reactivity. Under the reaction conditions, these functionalized [3]dendralenes underwent an in situ D–A cyclodimerization to provide highly functionalized cyclohexenes 9 with excellent regio- and stereo-control. Since then, we have been in the process of optimizing59 the substituents on the [3]dendralene skeleton, which would enhance their stability, thus granting them at our disposal for isolation, characterization and reactivity studies, especially for the D–A reaction. Herein, we report the aforementioned sequential double olefination process on appropriately substituted dienyl phosphonates for the synthesis of highly functionalized [3]dendralenes that are stable against D–A cyclodimerization under ambient conditions as well as their D–A reaction with N-methylmaleimide (NMM) as a representative dienophile.
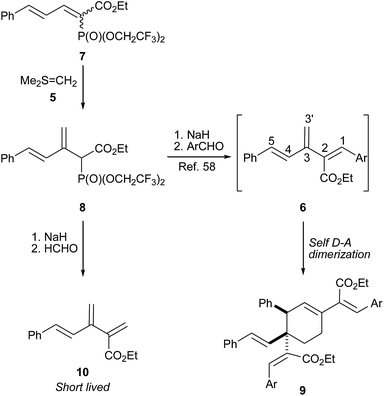 |
| Scheme 1 Synthesis of [3]dendralenes. | |
Results and discussion
Lately, Sherburn and coworkers reported studies pertaining to the stability and reactivity of various thinly substituted dendralenes.60,61 Their findings reveal that odd dendralenes are more reactive in comparison to their even counterparts. This observation has also been corroborated by DFT calculations, which suggest that odd dendralenes prefer a D–A reactive s-cis conformation, whereas even dendralenes predominantly remain in the D–A unreactive s-trans conformation. The study also divulged that the conjugating terminal substituents (at C1 or C5) accelerate the rate of D–A cyclodimerisation of [3]dendralenes contrary to the substituents at C2, C3 and C4, which have little effect on the dimerisation reactivity.60
In cognizance with the above facts, to accomplish our goal of synthesizing functionalized and stable [3]dendralenes, we decided to exclude one of the terminal substituent on our earlier reported58 [3]dendralenes 6 to obtain 2,5-disubstituted [3]dendralenes. The corresponding [3]dendralene 10 was synthesized from dienic phosphonate 7 via a 1,3-butadien-2-ylphosphonoacetate 8 intermediate, and formaldehyde was used as the aldehyde component (Scheme 1). Interestingly, the [3]dendralene 10, although short lived, could be isolated and characterized by 1H NMR. This exemplified the dramatic effect of the substituent on the reactivity and stability of the [3]dendralenes, akin to Sherburn's observation.
For further enhancement of the stability of [3]dendralenes, we decided to add a substituent at the 4-postion of our earlier reported58 [3]dendralenes 6, which would bestow 1,2,4,5-tetrasubstituted [3]dendralenes 11. At the outset, we decided to introduce a benzyl substituent at the 4-position of the reported [3]dendralenes 6. For this, p-anisaldehyde was reacted with 3-phenylpropanal in the presence of a catalytic amount of pyrrolidine and propionic acid,62 which provided the α,β unsaturated aldehyde 14a (Scheme 2). The piperidinium benzoate catalysed Knoevenagel condensation of 14a with ethyl bis-(2,2,2-trifluroethyl)-phosphonoacetate furnished the desired dienyl phosphonate 13a as an inseparable 1/1 mixture of two geometrical isomers with the double bond attached to a phosphonate group as judged from 1H NMR. When this phosphonate 13a was reacted with DMSM 5, which was generated using Me3SI and n-BuLi in THF, the desired olefination took place to give the appropriately substituted butadien-2-ylphosphonoacetate 12a. The phosphonoacetate 12a was then reacted with sodium hydride, followed by benzaldehyde in THF, which provided the 1,2,4,5-tetrasubstituted [3]dendralene 11a. We were gratified to note that the dendralene 11a was stable towards D–A cyclodimerization at ambient temperature. Subsequently, the butadien-2-ylphosphonoacetate 12a was reacted with a few more aldehydes under standard HWE conditions to give the desired 1,2,4,5-tetrasubstituted [3]dendralenes (11b–e) (Scheme 2), with one of the terminal aryl groups (5 position) having an electron donating substituent (4-OMe) in moderate yields but with high stereoselectivity, except for the product 11e, where ethyl glyoxalate was used as the aldehyde component. The dendralene 11e was obtained as a separable mixture of Z and E diastereoisomers (11ea
:
11eb, 60
:
40) for the double bond generated due to the H–W–E reaction.
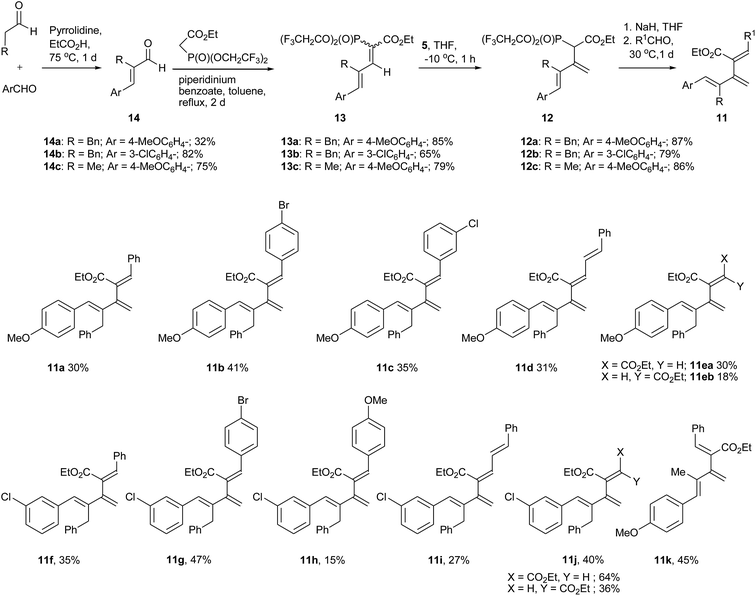 |
| Scheme 2 Synthesis of densely substituted isolable [3]dendralenes. | |
To examine the electronic effect of substitution on the terminal aryl rings on the reactivity of 1,2,4,5-tetrasubstituted [3]dendralenes, we decided to test it with a mildly electron withdrawing 3-chloro substituent at the 5-position aryl ring. To achieve this, 2-benzyl-3-(3-chlorophenyl) prop-2-enal 14b was converted to dienic phosphonate 13b as an inseparable 6
:
4 mixture of two diastereoisomers and then to butadien-2-ylphosphonoacetate 12b, as shown in Scheme 2. This phosphonoacetate 12b was reacted with different aldehydes, to provide another series of 1,2,4,5-tetrasubstituted [3]dendralenes (11f–j) in moderate yields with very high selectivity, except for 11j, which was produced as an inseparable mixture of Z/E diastereoisomers (double bond substituted with ester groups) in a ratio of 64/36. All these product dendralenes are stable towards D–A cyclodimerization at ambient temperature.
Our next goal was to change the 4-position substituent to a sterically less demanding substituent, like a methyl group instead of benzyl. To achieve this, prop-2-enal 14c was converted to 4-methyl substituted [3]dendralene 11k via butadien-2-ylphosphonoacetate 12c, as depicted in Scheme 2. Like in the previous cases, phosphonate 13c was also formed as an inseparable 1/1 mixture of diastereoisomers, and the product dendralene 11k was also stable at room temperature.
The structure of the dendralene 11a was confirmed by single crystal X-ray crystallography (Fig. 2), which also revealed its interesting conformational preferences in the solid state. The dienic part encompassing double bonds at the 1 and 3 positions are in a quasi s-cis conformation, while the diene component covering double bonds at the 3 and 4 positions is in an s-trans conformation. DFT calculations60 showed that in unsubstituted [3]dendralenes, one dienic component exists predominantly in the s-cis conformation while the other dienic unit exists in the s-trans conformation. The present X-ray crystal structure of dendralene 11a confirms this for the first time.
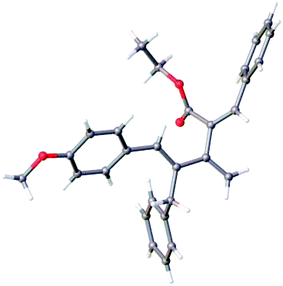 |
| Fig. 2 X-ray structure of 11a. | |
As mentioned earlier, the conjugating terminal substituents (at C1 or C5) are known to have a marked accelerating influence on the cyclodimerisation rate of [3]dendralenes, whereas the substituents at C2, C3 and C4 have little effect on the dimerization reactivity.60,61 In our earlier attempted [3]dendralenes 6 (Scheme 1),58,59 there were two conjugating terminal substituents at C1 and C5 and one substituent at the C2 position. None of those dendralenes could be isolated because they were unstable and underwent D–A cyclodimerisation. In the present study, the [3]dendralene 10 had one conjugating terminal substituent (C5) and one substituent at the C2 position, which was short lived but could be isolated and the 1H NMR spectrum could be recorded as a signature of its formation. Interestingly, [3]dendralenes 11a–11l with two conjugating terminal substituents at C1 and C5 were stabilized by the two substituents at the C2 and C4 positions. Thus, it can be inferred that the substituents at C2 and C4 have a substantial influence on rendering the stability to [3]dendralenes (Fig. 3).
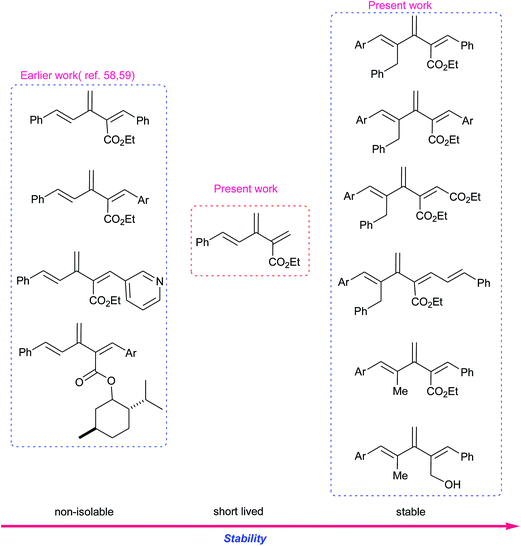 |
| Fig. 3 Stability of substituted [3]dendralenes. | |
After accomplishing our goal of obtaining 1,2,4,5-tetrasubstituted [3]dendralenes 11a–k that are stable towards D–A cyclodimerization, we next aimed to examine their reactivity for the intermolecular D–A reaction with NMM as the dienophile. When 1,5-diaryl-2-ethoxycarbonyl-4-benzyl [3]dendralene 11b was reacted with 1.0 molar equivalent of NMM in refluxing benzene, it furnished a mixture of stereoisomeric D–A adducts, out of which the major stereoisomer 15 (69%) was easily separated from the mixture, whereas the minor isomers could not be identified (Scheme 3). This major isomer 15 was found to be endo-isomer, as confirmed by X-ray crystallography (Fig. 4). It is noticeable that the D–A reaction took place at the diene ‘A’ incorporating double bonds at the 1,3 positions, thus indicating that these two double bonds exist in the s-cis conformation in solution, as was also observed in the solid state by the X-ray structure (Fig. 2). It is noteworthy that the electronically rich diene ‘B’ encompassing double bonds at the 3,4 positions is unable to participate in the D–A reaction owing to its s-trans conformation, which was preferred due to the steric bulk of the benzyl group, which results in a high barrier of s-trans ⇆ s-cis interconversion. This allowed the electronically deactivated diene to participate in the D–A reaction.
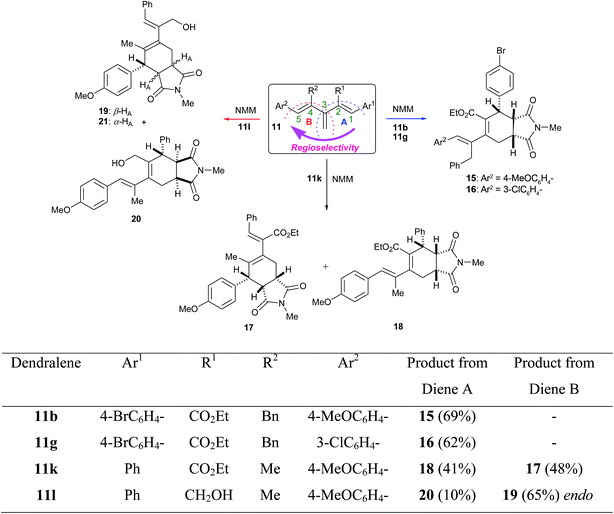 |
| Scheme 3 Diels–Alder reaction of [3]dendralenes. | |
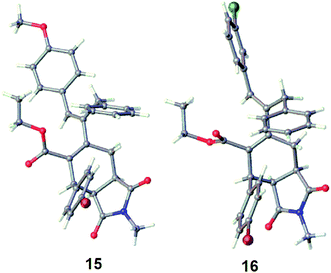 |
| Fig. 4 X-ray structures of 15, 16. | |
In order to examine if any stereoelectronic effect is operating from the conjugating aryl substituents at the 1 or 5 positions on the D–A reaction, [3]dendralene 11g was reacted with 1.0 molar equivalent of NMM in refluxing toluene and furnished a mixture of D–A adducts. From the mixture of products, the major diastereoisomer 16 was obtained in a 62% yield (Scheme 3) as an endo-isomer, which was confirmed by single crystal X-ray crystallography (Fig. 4). The minor isomers could not be identified. Once again, the DA reaction took place at the diene ‘A’ incorporating double bonds at the 1,3 positions, thus indicating that these two double bonds exist in the s-cis conformation in solution state, which is sterically less demanding. The electronic effect of the substituent at the 5-position aryl group did not play any role because the diene ‘B’, incorporating double bonds at the 3,4 positions, comprising this substituent probably preferred the s-trans conformation.
To quell the steric effect, 4-methyl substituted [3]dendralene 11k was reacted with NMM, which resulted in the formation of the regioisomeric adducts 17 and 18 (Scheme 3) in a ratio of 54
:
46, respectively. This suggests that now the steric hindrance was moderated, thus both the dienes ‘A’ and ‘B’ have a very similar preference for the s-cis conformation and could participate in the D–A reaction. The D–A adducts 17 and 18 could not be separated. Thus, their structural analysis was performed on a 54
:
46 mixture of the two regioisomers, where most of the signals were clearly distinguishable. The stereochemistry and regiochemistry of the products 17 and 18 were ascertained on the basis of COSY and ROESY interactions from the inseparable mixture (for detailed interpretations, see the ESI†). For isomer 17, strong ROESY interactions between the protons at δ 3.85 (ArCH) ↔ δ 7.08 (ortho H of Ar), and between δ 6.77 (PhCH) ↔ δ 7.40–7.31 (ortho H of Ph) (Fig. 5) indicate that the diene component comprising double bonds at the 3,4 positions participated in the D–A reaction. The ROESY interaction between protons at δ 3.85 (ArCH) and δ 3.17–3.13 (NCOCHCH2) further supports the endo addition of NMM (Fig. 5). For isomer 18, strong ROESY interactions between protons at δ 4.56 (PhCH) ↔ δ 7.09 (ortho H of Ph) and between δ 6.27 (ArCH) ↔ δ 7.30–7.18 (ortho H of Ar) indicate that the diene component comprising double bonds at the 1,3 positions participated in the D–A reaction, and the interaction between the protons at δ 4.56 (PhCH) ↔ δ 3.23–3.19 (NCOCHCH2) suggests the endo nature of the product (Fig. 5).
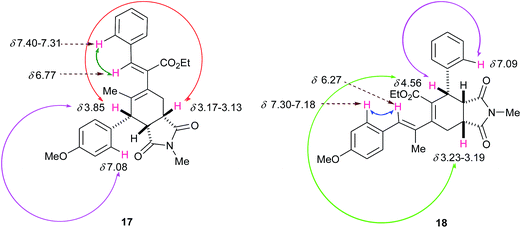 |
| Fig. 5 ROESY interactions of 17 and 18. | |
Despite our repeated efforts, these Diels–Alder adducts 15, 16 and the mixture of 17 and 18 did not undergo further D–A reaction with several dienophiles. This failure could be chiefly attributed to the stereoelectronic effects of the substituents to adopt an s-cis conformation of the dienic system in the D–A adducts, which is a prerequisite for the D–A reaction. The X-ray crystal structures of 15 and 16 also supported this fact, where it can be clearly seen that the diene component is in a gauche conformation. To address the electronic effect, the ester functionality in the dendralene 11k was reduced using DIBAL-H to obtain a new alcoholic [3]dendralene 11l (Scheme 4).
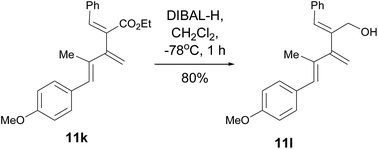 |
| Scheme 4 Preparation of [3]dendralene 11l. | |
When 11l was reacted with 1.0 molar equivalent of NMM in refluxing toluene, it furnished a mixture of three isomers, namely 19, 20 and 21, in a ratio of 80
:
14
:
6 (Scheme 3), respectively, from which all the individual isomers are separated by chromatography. The structure of 19 was established by single crystal X-ray crystallography (Fig. 6). The structure of 21 was assigned by studying the 1H NMR of 19 and 21. Both the spectra showed similarity except that ArCH in 21 was a singlet, thus suggesting a trans relationship of the two protons, viz. ArCH and ArCHCH. The structure of 20 was ascertained on the basis of COSY and ROESY interactions. The positive ROESY interactions between protons at δ 4.47 (PhCH) ↔ δ 7.36 (ortho H of Ph), between δ 6.48 (ArCH) ↔ δ 7.28 (ortho H of Ar), between δ 4.47 (PhCH) ↔ 4.37 (HOCHAHB), and between δ 4.47 (PhCH) ↔ δ 3.79 (HOCHAHB) are indicative of the depicted regiochemistry, whereas the absence of ROESY interactions between the protons at δ 4.47 (PhCH) ↔ δ 2.53–2.45 (NCOCHCH2) suggests the exo nature of the product (Fig. 7). The main difference between structures 18 (Fig. 5) and 20 (Fig. 7) is the stereochemistry at the ring junction. In the case of compound 18, we obtained a positive ROESY peak due to cis disposition of protons at the ring junction and benzylic proton. Therefore, in the case of compound 20, on the basis of an analogy from the absence of a ROESY peak between the protons at δ 4.47 (PhCH) ↔ δ 2.53–2.45 (NCOCHCH2), we concluded that the protons at the ring junction are trans with respect to the benzylic proton. This apparently exo product 20 probably is not due to the exo-mode D–A reaction but due to a small amount of double bond isomerisation during heating, which then underwent endo-selective D–A with NMM. No endo-isomer with this regioselectivity was found in the reaction mixture, which suggests that the diene ‘A’ (Scheme 3) with the depicted stereochemistry was preferred for s-trans conformation. Thus, the stereoselectivity, i.e. the endo
:
exo ratio (19
:
21), was 93
:
7, whereas the regioselectivity (19, 21
:
20) was 86
:
14. It is interesting to note that diene ‘B’ (Scheme 3), i.e. encompassing double bonds at the 3, 4 positions, predominantly participated in the D–A reaction, unlike in dendralenes 11k, 11b and 11g. Hence, there was a switch over of regioselectivity from diene ‘A’ to diene ‘B’ (Scheme 3) as the substituents on the [3]dendralenes were changed.
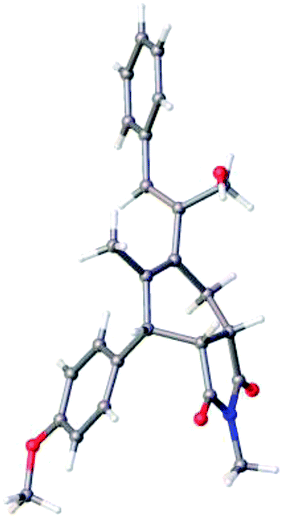 |
| Fig. 6 X-ray structure of 19. | |
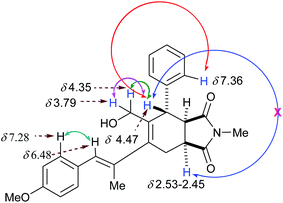 |
| Fig. 7 ROESY interactions of 20. | |
Conclusions
In conclusion, we achieved our goal of the synthesis of stable and highly functionalized [3]dendralenes. The present work was a systematic study encompassing the synthesis of 1,2,4,5-tetrasubstituted [3]dendralenes in acyclic systems, followed by a study of their reactivity patterns. It is evident that substituents at the 2 and 4 positions of [3]dendralenes drastically diminish their reactivity for self-D–A dimerisation reactions, irrespective of their electronic nature (electron donating or withdrawing), hence imparting stability. This is due to the D–A unfavoured s-trans conformation of the dienic components caused by the substituent at these positions. From the D–A reaction studies, it became apparent that the steric effect outweighs electronic activation or deactivation. Sterically hindered 4-benzyl substituted [3]dendralene switched the regioselectivity of the D–A reaction with NMM in favour of the electronically deactivated diene component with ethoxycarbonyl substitution at the 2-position. In the case of 4-methyl substituted [3]dendralene, the electronically rich diene component participated in D–A with NMM. The D–A reactions were highly endo selective. We were unable to engage the first D–A adduct for DTDA sequence with reactive dienophiles. This could be attributed to the steric effect of the dense functionalities, which prevented the diene adopting the s-cis conformation, which is a prerequisite for its success. Nonetheless, these D–A adducts could be promising candidates for biological studies as many of the isoindole derivatives display a myriad of biological properties, such as antimicrobial, antiviral, analgesic, antiinflammatory and antitumor activities.63
Experimental section
General information
All the reactions were performed in oven-dried (120 °C) or flame-dried glass apparatus under a dry N2 or argon atmosphere. Tetrahydrofuran (THF) was dried by distillation from sodium/benzophenone ketyl. n-BuLi (1.6 M in hexane) was purchased from Aldrich. All the aldehydes were purchased from Aldrich and were freshly distilled prior to use. TLC was carried out using Merck silica gel 60 F254 plates. Column chromatography was performed on silica gel (230–400 mesh). 1H NMR spectra were recorded at 200, 300, 500 and 600 MHz; 13C NMR spectra were recorded at 50, 75, 125 and 150 MHz and 31P NMR spectra were recorded on a 121.5 MHz spectrometer using CDCl3, C6D6 and CD3COCD3 as the solvents. The spectra were referenced to residual chloroform (δ 7.25 ppm, 1H; 77.00 ppm, 13C), partially deuterated acetone (δ 2.05 ppm, 1H; 29.9, 206.7 ppm, 13C), partially deuterated benzene (δ 7.16 ppm, 1H; 128.4 ppm, 13C) and H3PO4 as the external reference (δ 0.00 ppm, 31P). IR spectra were carried out on the FT-IR-spectrometer using NaCl discs, and wavenumbers were given in cm−1. Melting points (mp) were uncorrected. A high-resolution mass spectrum was obtained using a high-resolution ESI-TOF mass spectrometer.
General procedure for aldehydes 14a–c. A solution of 3-phenylpropanal (3.2 mL, 24.3 mmol, 1 equiv.), 4-methoxybenzaldehyde (29.5 mL, 243 mmol, 10 equiv.), pyrrolidine (0.6 mL, 7.3 mmol, 0.3 equiv.) and propionic acid (0.55 mL, 7.3 mmol, 0.3 equiv.) was heated at 75 °C for 24 h under an argon atmosphere. Excess 4-methoxybenzaldehyde was distilled out and the residue was purified by column chromatography (petroleum ether–EtOAc; 95
:
5) to provide the aldehyde 14a (1.96 g, 32%) as an orange-coloured viscous liquid. The same procedure was followed for the preparation of compounds 14b and c.
(E)-2-Benzyl-3-(4-methoxyphenyl)acrylaldehyde (14a)64. Rf (hexane–EtOAc, 90
:
10) = 0.35; IR νmax: 3058, 3028, 3002, 2954, 2931, 2908, 2836, 2715, 2566, 1683, 1667, 1624, 1592, 1508, 1495, 1453, 1305, 1252, 1210, 1175, 1148, 1032, 956, 887, 828, 736, 700 cm−1; 1H NMR (200 MHz, CDCl3): δ 9.66 (s, 1H, CHO), 7.46 (d, J = 9.0 Hz, 2H, Ar), 7.44 (s, 1H, ArCH), 7.27–7.19 (m, 5H, Ph), 6.90 (d, J = 8.8 Hz, 2H, Ar), 3.97 (s, 2H, PhCH2), 3.82 (s, 3H, OCH3); 13C NMR (50 MHz, CDCl3): δ 194.9, 161.0, 151.5, 138.2, 137.9, 131.8, 128.4, 127.7, 126.9, 126.0, 114.2, 55.1, 30.1.
(E)-2-Benzyl-3-(3-chlrophenyl)acrylaldehyde (14b)64. Following the general procedure, an isolated yield (1.36 g, 82%) as a yellow-coloured viscous liquid was obtained; Rf (hexane–EtOAc, 90
:
10) = 0.4; IR νmax: 3083, 3061, 3027, 2927, 2826, 2717, 1682, 1627, 1599, 1561, 1494, 1474, 1453, 1394, 1206, 1142, 1077, 956, 890, 784, 737, 697 cm−1; 1H NMR (200 MHz, CDCl3): δ 9.68 (s, 1H, CHO), 7.45–7.11 (m, 10H, Ar, Ph, C
CH), 3.90 (s, 2H, PhCH2); 13C NMR (50 MHz, CDCl3): δ 194.7, 149.4, 141.7, 138.0, 136.1, 134.7, 130.0, 129.7, 129.5, 128.6, 127.9, 127.5, 126.3, 30.3.
(E)-3-(4-Methoxyphenyl)-2-methylacrylaldehyde (14c)65. Following the general procedure, an isolated yield (5.2 g, 75%) as a yellow-coloured viscous liquid was obtained; Rf (hexane–EtOAc, 80
:
20) = 0.48; IR νmax: 3007, 2961, 2935, 2837, 2716, 1682, 1622, 1598, 1571, 1514, 1443, 1407, 1321, 1304, 1258, 1177, 1116, 1034, 1014, 763, 571, 562, 538 cm−1; 1H NMR (300 MHz, CDCl3): δ 9.54 (s, 1H, CHO), 7.53 (d, J = 8.4 Hz, 2H, Ar), 7.19 (s, 1H, ArCH), 6.97 (d, J = 8.4 Hz, 2H, Ar), 3.86 (s, 3H, OCH3), 2.08 (s, 3H, C
CCH3); 13C NMR (150 MHz, CDCl3): δ 195.3, 160.6, 149.7, 136.0, 131.9, 127.8, 114.1, 55.2, 10.7.
General procedure for phosphonates 13a–c. A solution of aldehyde 14a (2.4 g, 9.5 mmol, 1 equiv.), ethyl bis-(2,2,2-trifluroethyl)-phosphonoacetate (3.6 g, 9.5 mmol, 1 equiv.) and piperidinium benzoate (394 mg, 1.9 mmol, 0.2 equiv.) in toluene (25 mL) was heated under reflux for 2 d in a flask fitted with Dean–Stark apparatus. The reaction mixture was then brought to room temperature, diluted with water and extracted with ethyl acetate. The organic extract was concentrated under the reduced pressure, and the residue was purified by column chromatography (petroleum ether–EtOAc; 85
:
15) to give phosphonate 13a as an inseparable mixture of two E/Z isomers (3.15 g, 85%) as a brown-coloured viscous liquid. The same procedure was followed for the preparation of compounds 13b and c.
(2E/Z,4E)-Ethyl 4-benzyl-2-[bis(2,2,2-trifluoroethoxy)phosphoryl]-5-(4-methoxyphenyl)penta-2,4-dienoate (13a). Rf (hexane–EtOAc, 80
:
20) = 0.29; found: C, 53.10; H, 4.20. Calc. for C25H25F6O6P: C, 53.01; H, 4.45%; IR νmax: 3063, 3028, 3002, 2970, 2939, 2907, 1720, 1605, 1579, 1508, 1496, 1455, 1419, 1296, 1251, 1175, 1104, 1071, 1032, 962 cm−1; 1H NMR (600 MHz, CDCl3): δ 7.63 (d, J = 25.8 Hz, 1H, ArC
CCH
C, 2Z), 7.40 (d, J = 25.8 Hz, 1H, ArC
CCH
C, 2E), 7.29–7.12 (m, 14H, Ph, Ar), 6.89 (d, J = 8.4 Hz, 2H, Ar, 2Z), 6.87 (s, 1H, ArCH, 2Z), 6.86 (d, J = 9.0 Hz, 2H, Ar, 2E), 6.83 (s, 1H, ArCH, 2E), 4.34–4.08 (m, 8H, 2 × OCH2CF3), 4.24 (q, J = 7.2 Hz, 2H, OCH2CH3, 2Z), 3.99 (q, J = 7.2 Hz, 2H, OCH2CH3, 2E), 3.98 (s, 2H, PhCH2, 2Z), 3.82 (s, 3H, OCH3, 2Z), 3.80 (s, 3H, OCH3, 2E), 3.79 (s, 2H, PhCH2, 2E), 1.28 (d, J = 7.2 Hz, 3H, OCH2CH3, 2Z), 1.09 (d, J = 7.2 Hz, 3H, OCH2CH3, 2E); 13C NMR (150 MHz, CDCl3): δ 164.3 (d, J = 13.9 Hz, 2Z), 163.8 (d, J = 14.8 Hz, 2E), 160.1, 156.1 (d, J = 6.3 Hz, 2Z), 154.8 (d, J = 7.2 Hz, 2E), 143.1 (2Z), 139.6 (2Z), 138.5 (2E), 138.0 (2Z), 133.5 (d, J = 19.9 Hz, 2E), 133.2 (d, J = 21.3 Hz, 2Z), 131.4 (2E), 131.1 (2Z), 128.9 (2E), 128.4 (2Z), 128.3 (2E), 128.1 (2Z), 127.8 (2E), 126.5 (2E), 126.3 (2Z), 122.4 (dq, J = 275.8, 9.7 Hz), 120.6 (d, J = 186.4 Hz, 2E), 118.5 (d, J = 184.3 Hz, 2Z), 114.0 (2Z), 113.8 (2E), 62.3 (q, J = 37.9 Hz, 2Z), 62.2 (q, J = 37.9 Hz, 2E), 61.8, 55.1, 41.3 (2E), 34.8 (2Z), 13.6 (2E), 13.3 (2Z).
(2E/Z,4E)-Ethyl 4-benzyl-2-[bis(2,2,2-trifluoroethoxy)phosphoryl]-5-(3-chlorophenyl)penta-2,4-dienoate (13b). Following the general procedure, an inseparable mixture 60
:
40 of two E/Z isomers was isolated, yield (1.42 g, 65%) as a brown-coloured viscous liquid; Rf (hexane–EtOAc, 80
:
20) = 0.45; found: C, 50.37; H, 3.59. Calc. for C24H22ClF6O5P: C, 50.50; H, 3.88%; IR νmax: 3064, 2982, 1723, 1594, 1495, 1454, 1419, 1374, 1173, 1070, 963, 879 cm−1; 1H NMR (300 MHz, CDCl3): δ 7.55 (dd, J = 25.5, 1.5 Hz, 1H, ArCHCCH
C, 2Z), 7.36 (dd, J = 25.8, 1.2 Hz, 1H, ArCHCCH
C, 2E), 7.30–7.05 (m, 18H, Ar, Ph), 7.04 (s, 1H, ArCH, 2E), 6.65 (s, 1H, ArCH, 2Z), 4.35–4.12 (m, 10H, 2 × OCH2CF3, OCH2CH3, 2Z), 4.08 (q, J = 7.2 Hz, 2H, OCH2CH3, 2E), 3.91 (s, 2H, PhCH2, 2E), 3.76 (s, 2H, PhCH2, 2Z), 1.27 (t, J = 7.2 Hz, 3H, OCH2CH3, 2Z), 1.15 (t, J = 7.2 Hz, 3H, OCH2CH3, 2E); 13C NMR (75 MHz, CDCl3): δ 164.1 (d, J = 13.6, 2E), 163.4 (d, J = 14.8, 2Z), 155.2 (d, J = 6.5, 2E), 154.7 (d, J = 6.8, 2Z), 139.0 (2E), 137.8 (2E), 137.6 (2Z), 137.4 (2E), 137.1 (2Z), 136.6 (d, J = 21.0, 2E), 135.0 (2Z), 134.4 (d, J = 19.1, 2Z), 129.9 (2E), 129.7 (2Z), 129.3, 129.1, 128.6, 128.3, 127.6 (2Z), 127.1 (2E), 126.9 (2Z), 126.7 (2E), 123.3 (d, J = 185.6, 2Z), 122.4 (dq, J = 266.6, 9.3), 121.3 (d, J = 183.6, 2E), 62.4 (q, J = 37.7), 62.1, 42.0 (2Z), 35.3 (2E), 13.7 (2Z), 13.6 (2E).
(2E/Z,4E)-Ethyl 2-[bis(2,2,2-trifluoroethoxy)phosphoryl]-5-(4-methoxyphenyl)-4-methylpenta-2,4-dienoate (13c). Following the general procedure, an inseparable mixture 1
:
1 of two E/Z isomers was isolated, yield (6.14 g, 79%) as a brown-coloured viscous liquid; Rf (hexane–EtOAc, 80
:
20) = 0.25; found: C, 46.48; H, 3.98. Calc. for C19H21F6O6P: C, 46.54; H, 4.32%; IR νmax: 2965, 2937, 2909, 1720, 1606, 1578, 1509, 1305, 1258, 1175, 1101, 1071, 960 cm−1; 1H NMR (600 MHz, CDCl3): δ 7.63 (d, J = 26.4 Hz, 1H, ArC
CCH
C, 2Z), 7.39 (d, J = 25.8 Hz, 1H, ArC
CCH
C, 2E), 7.34 (d, J = 9.0 Hz, 2H, Ar, 2E), 7.15 (d, J = 8.4 Hz, 2H, Ar, 2Z), 6.94 (s, 1H, ArCH, 2E), 6.92 (d, J = 9.0 Hz, 2H, Ar, 2E), 6.89 (d, J = 8.4 Hz, 2H, Ar, 2Z), 6.87 (s, 1H, ArCH, 2Z), 4.46–4.37 (m, 8H, 2 × OCH2CF3), 4.30 (q, J = 7.2 Hz, 4H, OCH2CH3), 3.84 (s, 3H, OCH3, 2E), 3.82 (s, 3H, OCH3, 2Z), 2.04 (s, 3H, C
CCH3, 2E), 2.03 (s, 3H, C
CCH3, 2Z), 1.33 (t, J = 7.2 Hz, 6H, OCH2CH3); 13C NMR (125 MHz, CDCl3): δ 165.1 (d, J = 13.2 Hz, 2E), 164.7 (d, J = 16.2 Hz, 2Z), 160.1, 156.6 (d, J = 7.2 Hz, 2E), 152.1 (d, J = 7.1 Hz, 2Z), 144.3 (2E), 140.7 (2Z), 131.7, 131.6 (2Z), 131.3 (2E), 128.5 (2Z), 128.3 (2E), 122.5 (dq, J = 266.4, 9.5 Hz), 120.0 (d, J = 189.9 Hz, 2Z), 116.7 (d, J = 187.5 Hz, 2E), 114.0, 62.5 (dq, J = 39.2, 3.6 Hz), 62.0, 55.2, 21.4, 15.6, 13.7.
General procedure for butadien-2-ylphosphonoacetate 12a–c. n-BuLi (0.89 mL, 1.6 M, 1.43 mmol, 3 equiv.) was added drop-wise to a stirred suspension of trimethylsulfonium iodide (292 mg, 1.43 mmol, 3 equiv.) in THF (10 mL) at −10 °C under an argon atmosphere and stirred for 20 min at the same temperature. Later, a solution of dienic phosphonate 13a (270 mg, 0.47 mmol, 1 equiv.) in THF (5 mL) was cannulated into the reaction mixture and stirred for 1 h. The temperature of the reaction mixture was allowed to rise slowly to room temperature, followed by dilution of the reaction mixture with water and extraction with ethyl acetate. The organic layer was concentrated and column chromatography (petroleum ether–EtOAc; 85
:
15) was done to obtain the diene 12a (0.24 g, 87%) as a pale yellow liquid. The same procedure was followed for the preparation of compounds 12b and c.
(4E)-Ethyl 4-benzyl-2-[bis(2,2,2-trifluoroethoxy)phosphoryl]-5-(4-methoxyphenyl)-3-methylene-4-pentenoate 12a. Rf (hexane–EtOAc, 80
:
20) = 0.34; found: C, 53.98; H, 4.93. Calc. for C26H27F6O6P: C, 53.80; H, 4.69%; IR νmax: 3058, 3024, 2969, 2938, 2911, 2840, 1729, 1649, 1602, 1574, 1542, 1510, 1456, 1417, 1369, 1299, 1258, 1173, 1104, 1070, 1032, 965, 878, 844, 699, 660 cm−1; 1H NMR (600 MHz, CDCl3): δ 7.30–7.27 (m, 2H, Ph), 7.21–7.20 (m, 5H, Ph, Ar), 6.94 (s, 1H, ArCH), 6.83 (d, J = 8.4 Hz, 2H, Ar), 5.57 (d, J = 4.8 Hz, 1H, C
CHAHB), 5.51 (d, J = 4.8 Hz, 1H, C
CHAHB), 4.48–4.36 (m, 4H, 2 × OCH2CF3), 4.34 (d, J = 24 Hz, 1H, POCH), 4.17 (q, J = 7.2 Hz, 2H, OCH2CH3), 3.95 (d, J = 16.8 Hz, 1H, PhCHAHB), 3.88 (d, J = 16.8 Hz, 1H, PhCHAHB), 3.79 (s, 3H, OCH3), 1.22 (t, J = 7.2 Hz, 3H, OCH2CH3); 13C NMR (125 MHz, CDCl3): δ 167.1, 158.9, 139.2, 137.7, 135.6, 130.8, 129.9, 129.2 (d, J = 9.4 Hz), 128.5, 128.0, 126.0, 122.8 (dq, J = 181.3, 9.1 Hz), 119.8 (d, J = 8.5 Hz), 113.8, 63.5–62.0 (m), 62.3, 55.1, 48.1 (d, J = 145.3 Hz), 34.6, 13.7; 31P NMR (121.5 MHz, CDCl3): δ 23.4.
(4E)-Ethyl 4-benzyl-2-[bis(2,2,2-trifluoroethoxy)phosphoryl]-5-(3-chlorophenyl)-3-methylene-4-pentenoate (12b). Following the general procedure, an isolated yield (0.18 g, 79%) as a pale yellow liquid was obtained; Rf (hexane–EtOAc, 80
:
20) = 0.25; found: C, 51.37; H, 4.39. Calc. for C25H24ClF6O5P: C, 51.34; H, 4.14%; IR νmax: 3063, 3028, 2968, 2932, 2878, 1734, 1718, 1603, 1594, 1560, 1497, 1472, 1456, 1417, 1297, 1264, 1170, 1100, 1070, 962 cm−1; 1H NMR (200 MHz, CDCl3): δ 7.30–7.15 (m, 9H, Ar, Ph), 6.92 (s, 1H, ArCH), 5.64 (d, J = 4.8 Hz, 1H, C
CHAHB), 5.58 (d, J = 5 Hz, 1H, C
CHAHB), 4.50–4.06 (m, 5H, 2 × OCH2CF3, POCH), 4.17 (q, J = 7.2 Hz, 2H, OCH2CH3), 3.93 (d, J = 16.6 Hz, 1H, PhCHAHB), 3.82 (d, J = 16.4 Hz, 1H, PhCHAHB), 1.22 (t, J = 7.2 Hz, 3H, OCH2CH3); 13C NMR (50 MHz, CDCl3): δ 167.0 (d, J = 1.5 Hz), 138.8 (d, J = 6 Hz), 138.7, 138.6, 137.4 (d, J = 8 Hz), 134.2, 129.8, 129.7, 128.7, 128.6, 128.0, 127.5, 126.5, 126.3, 120.4 (dq, J = 276, 8 Hz) 121.2 (d, J = 9 Hz), 62.8, (dq, J = 32, 6 Hz), 62.5, 48.3 (d, J = 145 Hz), 34.6, 13.8; 31P NMR (121.5 MHz, CDCl3): δ 23.1.
(4E)-Ethyl 2-[bis(2,2,2-trifluoroethoxy)phosphoryl]-5-(4-methoxyphenyl)-4-methyl-3-methylene-4-pentenoate (12c). Following the general procedure, an isolated yield (700 mg, 86%) as a pale yellow liquid was obtained; Rf (hexane–EtOAc, 80
:
20) = 0.3; found: C, 47.79; H, 4.85. Calc. for C20H23F6O6P: C, 47.63; H, 4.60%; IR νmax: 2971, 2938, 2914, 2838, 1730, 1609, 1509, 1460, 1415, 1371, 1302, 1161, 1068, 1033, 960 cm−1; 1H NMR (600 MHz, CDCl3): δ 7.21 (d, J = 8.4 Hz, 2H, Ar), 6.89 (d, J = 8.4 Hz, 2H, Ar), 6.64 (s, 1H, ArCH), 5.60 (d, J = 4.8 Hz, 1H, C
CHAHB), 5.59 (d, J = 6.0 Hz, 1H, C
CHAHB), 4.51–4.35 (m, 4H, 2 × OCH2CF3), 4.38 (d, J = 24 Hz, 1H, POCH), 4.25 (q, J = 7.2 Hz, 1H, OCHAHBCH3), 4.24 (q, J = 7.2 Hz, 1H, OCHAHBCH3), 3.82 (s, 3H, OCH3), 2.04 (s, 3H, C
CCH3), 1.28 (t, J = 7.2 Hz, 3H, OCH2CH3); 13C NMR (125 MHz, CDCl3): δ 167.3, 158.5, 139.4 (d, J = 8.2 Hz), 134.2, 130.5, 129.8, 128.0, 122.4 (dq, J = 270, 28 Hz), 118.0 (d, J = 8.2 Hz), 113.5, 62.6 (dq, J = 8.6, 24.7 Hz), 62.4, 55.1, 48.1 (d, J = 144.4 Hz), 16.1, 13.8; 31P NMR (121.5 MHz, C6D6): δ 23.7.
(E)-Ethyl 2,3-dimethylene-5-phenylpent-4-enoate (10). A solution of diene 8 (0.29 g, 0.63 mmol), in THF (6 mL) was cannulated to a suspension of NaH (0.028 g, 55% in oil, 0.63 mmol) in THF (1 mL) at 0 °C. After 15 min, formaldehyde gas was bubbled through this reaction mixture for 5 min and 10 min. The reaction mixture was diluted with water and extracted with 20% EtOAc in hexane. The organic extract was concentrated under reduced pressure to give 10. Attempts to purify this material by chromatography were not successful due to degradation by dimerization and polymerization. Rf (hexane–EtOAc, 95
:
5) = 0.5; 1H NMR (200 MHz, CDCl3): δ 7.40–7.18 (m, 5H, Ph), 6.89 (d, J = 16.2 Hz, 1H, PhCH), 6.38 (d, J = 1.2 Hz, 1H, EtCO2C
CHAHB), 6.36 (d, J = 16.2 Hz, 1H, PhCH
CH), 5.75 (d, J = 1.2 Hz, 1H, EtCO2C
CHAHB), 5.38 (s, 1H, PhCH
CHC
CHAHB), 5.23 (s, 1H, PhCH
CHC
CHAHB), 4.23 (q, J = 7.2 Hz, 2H, OCH2), 1.28 (t, J = 7.2 Hz, 3H, OCH2CH3).
General procedure for [3]dendralenes 11a–k. A solution of butadien-2-ylphosphonoacetate 12a (190 mg, 0.33 mmol, 1 equiv.) in THF (5 mL) was cannulated to a suspension of sodium hydride (15 mg, 55% in oil, 0.33 mmol, 1 equiv.) in THF (1 mL) under an argon atmosphere and stirred for 5 min. Then, freshly distilled benzaldehyde (34 μL, 0.33 mmol, 1 equiv.) was added and stirred at room temperature for 24 h. The reaction mixture was diluted with water and extracted with ethyl acetate. The organic layer was concentrated under the reduced pressure and purified by column chromatography (petroleum ether–EtOAc; 95
:
5) to obtain the dendralene 11a (42 mg, 30%) as a white solid. The same procedure was followed for the preparation of dendralenes 11b–k.
(2Z,4E)-Ethyl 4-benzyl-2-benzylidene-5-(4-methoxyphenyl)-3-methylenepent-4-enoate (11a). Rf (benzene) = 0.85; mp 92–93 °C; found: C, 82.26; H, 6.83. Calc. for C29H28O3: C, 82.05; H, 6.65%; IR νmax: 3081, 2959, 2923, 2855, 1715, 1604, 1573, 1508, 1494, 1463, 1455, 1370, 1304, 1256, 1210, 1175, 1095, 1029 cm−1; 1H NMR (600 MHz, CDCl3): δ 7.35–7.23 (m, 12H, Ar, 2 × Ph), 6.90 (s, 1H, PhCH), 6.84 (s, 1H, ArCH), 6.84 (d, J = 9.0 Hz, 2H, Ar), 5.27 (s, 1H, C
CHAHB), 5.24 (s, 1H, C
CHAHB), 4.19 (q, 2H, J = 7.2 Hz, OCH2CH3), 3.94 (s, 2H, PhCH2), 3.79 (s, 3H, OCH3), 1.16 (t, J = 7.2 Hz, 3H, OCH2CH3); 13C NMR (150 MHz, CDCl3): δ 169.0, 158.9, 148.7, 139.8, 136.4, 135.8, 135.7, 134.9, 132.4, 130.1, 129.8, 128.7, 128.6, 128.5, 128.4, 126.1, 117.1, 113.9, 61.1, 55.4, 35.5, 14.0. Recrystallization of compound 11a from ethyl acetate/hexane gave crystals suitable for single crystal X-ray analysis.
(2Z,4E)-Ethyl 2-(4-bromobenzylidene)-4-benzyl-5-(4-methoxyphenyl)-3-methylenepent-4-enoate (11b). Following the general procedure, an isolated yield (43 mg, 41%) as a pale yellow liquid was obtained; Rf (benzene) = 0.75; found: C, 69.14; H, 5.57. Calc. for C29H27BrO3: C, 69.19; H, 5.41%; IR νmax: 2956, 2923, 2853, 1715, 1698, 1649, 1607, 1555, 1538, 1508, 1487, 1457, 1369, 1275, 1247, 1179, 1106, 1070, 1036 cm−1; 1H NMR (200 MHz, CDCl3): δ 7.46 (d, 2H, J = 8.4 Hz, Ar), 7.31–7.19 (m, 9H, Ar, Ph), 6.85 (s, 1H, 4-BrC6H4CH), 6.83 (d, J = 8.8 Hz, 2H, Ar), 6.73 (s, 1H, 4-CH3OC6H4CH), 5.25 (s, 1H, C
CHAHB), 5.24 (s, 1H, C
CHAHB), 4.18 (q, J = 7.2 Hz, 2H, OCH2CH3), 3.91 (s, 2H, PhCH2), 3.79 (s, 3H, OCH3), 1.17 (t, J = 7.2 Hz, 3H, OCH2CH3); 13C NMR (150 MHz, CDCl3): δ 168.6, 158.9, 148.6, 139.7, 136.6, 136.3, 134.6, 133.6, 132.5, 131.5, 130.2, 130.1, 129.7, 128.6, 128.5, 126.1, 122.5, 117.4, 113.9, 61.2, 55.3, 35.5, 14.1.
(2Z,4E)-Ethyl 2-(3-chlorobenzylidene)-4-benzyl-5-(4-methoxyphenyl)-3-methylenepent-4-enoate (11c). Following the general procedure, an isolated yield (57 mg, 35%) as a pale yellow liquid was obtained; Rf: (hexane–EtOAc, 95
:
5) = 0.4; found: C, 75.91; H, 5.93. Calc. for C29H27ClO3: C, 75.89; H, 5.93%; IR νmax: 3065, 2924, 2866, 2833, 1717, 1605, 1562, 1510, 1495, 1464, 1453, 1371, 1366, 1336, 1304, 1255, 1206, 1177, 1089, 1034 cm−1; 1H NMR (600 MHz, CDCl3): δ 7.38–7.19 (m, 11H, Ar), 6.87 (s, 1H, 3-ClPhCH), 6.84 (d, J = 9.0 Hz, 2H, 4-OCH3Ph), 6.76 (s, 1H, 4-OCH3PhCH), 5.27 (s, 1H, C
CHAHB), 5.26 (s, 1H, C
CHAHB), 4.20 (q, J = 7.2 Hz, 2H, OCH2CH3), 3.93 (s, 2H, PhCH2), 3.79 (s, 3H, OCH3), 1.18 (t, J = 7.2 Hz, 3H, OCH2CH3); 13C NMR (150 MHz, CDCl3): δ 168.5158.9, 148.4, 139.7, 137.5, 137.3, 136.2, 134.3, 133.2, 132.5, 130.2, 129.7, 129.6, 128.6, 128.5, 128.3, 126.7, 126.1, 117.5, 113.9, 61.3, 55.4, 35.5, 14.0.
(2Z,4E)-Ethyl 2-[(E)-3-benzyl-4-(4-methoxyphenyl)buta-1,3-dien-2-yl]-5-phenylpenta-2,4-dienoate (11d). Following the general procedure, an isolated yield (43 mg, 31%) as a yellow liquid was obtained; Rf (hexane–EtOAc, 90
:
10) = 0.53; found: C, 82.63; H, 6.72. Calc. for C31H30O3: C, 82.64; H, 6.71%; IR νmax: 3062, 3024, 2956, 2928, 2839, 1702, 1609, 1592, 1508, 1495, 1453, 1369, 1301, 1246, 1217, 1175, 1149, 1036, 976 cm−1; 1H NMR (500 MHz, CDCl3): δ 7.86 (dd, J = 15.5, 11.5 Hz, 1H, PhCH
CHCH
C), 7.51 (d, J = 7.0 Hz, 2H, Ar), 7.38–7.17 (m, 10H, 2 × Ph), 6.81 (d, J = 9.0 Hz, 2H, Ar), 6.80 (d, J = 15.5 Hz, 1H, PhCH), 6.76 (s, 1H, ArCH), 6.71 (d, J = 11.5 Hz, 1H, PhCH
CHCH
C), 5.24 (s, 1H, C
CHAHB), 5.17 (s, 1H, C
CHAHB), 4.26 (q, J = 7 Hz, 2H, OCH2CH3), 3.93 (s, 2H, PhCH2), 3.78 (s, 3H, OCH3), 1.29 (t, J = 7 Hz, 3H, OCH2CH3); 13C NMR (125 MHz, CDCl3): δ 167.1, 158.7, 149.0, 141.0, 140.0, 139.7, 136.6, 132.8, 131.6, 129.9, 129.8, 128.6, 128.5, 128.3, 128.2, 127.2, 125.8, 125.5, 116.3, 113.7, 60.5, 55.1, 34.8, 14.2.
Synthesis of 11ea/eb. Following the general procedure, a 6
:
4 mixture of Z/E isomers (1H NMR) was isolated as a colourless liquid. The individual isomers were separated by chromatography.
Diethyl 2-[(E)-3-benzyl-4-(4-methoxyphenyl)buta-1,3-dien-2-yl]maleate (11ea). Isolated yield (55 mg, 30%); Rf (hexane–EtOAc, 80
:
20) = 0.54; found: C, 74.08; H, 6.82. Calc. for C26H28O5: C, 74.26; H, 6.71%; IR νmax: 3059, 2980, 2960, 2932, 2835, 1720, 1610, 1510, 1500, 1453, 1396, 1364, 1333, 1254, 1176, 1092, 1035 cm−1; 1H NMR (500 MHz, CDCl3): δ 7.29–7.25 (m, 4H, Ar, Ph), 7.21–7.18 (m, 3H, Ph), 6.86 (d, J = 9.0 Hz, 2H, Ar), 6.77 (s, 1H, ArCH), 6.00 (s, 1H, CHCO2Et), 5.30 (s, 1H, C
CHAHB), 5.27 (s, 1H, C
CHAHB), 4.31 (q, J = 7.0 Hz, 2H, OCH2CH3), 4.22 (q, J = 7.0 Hz, 2H, OCH2CH3), 3.85 (s, 2H, PhCH2), 3.80 (s, 3H, OCH3), 1.31 (t, 3H, J = 7.0 Hz), 1.30 (t, J = 7.0 Hz, 3H, OCH2CH3); 13C NMR (125 MHz, CDCl3): δ 167.3, 164.9, 158.9, 148.7, 146.0, 139.0, 135.5, 133.0, 129.9, 129.1, 128.4, 126.0, 121.0, 120.1, 113.8, 61.5, 60.9, 55.2, 35.5, 14.0, 13.9.
Diethyl 2-((E)-3-benzyl-4-(4-methoxyphenyl)buta-1,3-dien-2-yl)fumarate (11eb). Isolated yield (33 mg, 18%); Rf (hexane–EtOAc, 80
:
20) = 0.52; IR νmax: 3061, 2981, 2837, 1722, 1607, 1510, 1495, 1391, 1250, 1032 cm−1; 1H NMR (200 MHz, CDCl3): δ 7.28–7.18 (m, 7H, Ar, Ph), 6.77 (d, J = 8.8 Hz, 2H, Ar), 6.41 (s, 1H, ArCH), 5.93 (s, 1H, CHCO2Et), 5.39 (s, 1H, C
CHAHB), 5.02 (s, 1H, C
CHAHB), 4.37 (q, J = 7.0 Hz, 2H, OCH2CH3), 4.16 (q, J = 7.2 Hz, 2H, OCH2CH3), 3.76 (s, 3H, OCH3), 3.52 (s, 2H, PhCH2), 1.37 (t, J = 7.0 Hz, 3H, OCH2CH3), 1.25 (t, J = 7.2 Hz, 3H, OCH2CH3); 13C NMR (50 MHz, CDCl3): δ 167.6, 165.2, 158.8, 147.1, 143.0, 138.7, 135.9, 129.9, 129.8, 129.4, 128.6, 128.3, 126.4, 123.7, 118.3, 113.7, 61.6, 60.9, 55.2, 45.6, 14.0.
(2Z,4E)-Ethyl 4-benzyl-2-benzylidene-5-(3-chlorophenyl)-3-methylenepent-4-enoate (11f). Following the general procedure, an isolated yield (54 mg, 35%) as a colourless liquid was obtained; Rf (hexane–EtOAc, 95
:
5) = 0.5; found: C, 78.62; H, 6.12. Calc. for C28H25ClO2: C, 78.40; H, 5.87%; IR νmax: 3060, 3026, 2981, 2959, 2871, 1716, 1636, 1589, 1556, 1490, 1476, 1453, 1373, 1213, 1095 cm−1; 1H NMR (300 MHz, CDCl3): δ 7.35–7.18 (m, 14H, Ar, 2 × Ph), 6.88 (s, 1H, PhCH), 6.83 (s, 1H, ArCH), 5.33 (s, 1H, C
CHAHB), 5.29 (s, 1H, C
CHAHB), 4.21 (q, J = 7.2 Hz, 2H, OCH2CH3), 3.90 (s, 2H, PhCH2), 1.17 (t, J = 7.2 Hz, 3H, OCH2CH3); 13C NMR (75 MHz, CDCl3): δ 168.7, 148.0, 139.6, 139.3, 139.0, 135.5, 135.3, 135.0, 134.2, 131.1, 129.6, 128.8, 128.5, 128.4, 128.3, 127.2, 126.6, 126.2, 118.0, 61.1, 35.4, 13.9.
(2Z,4E)-Ethyl 2-(4-bromobenzylidene)-4-benzyl-5-(3-chlorophenyl)-3-methylenepent-4-enoate (11g). Following the general procedure, an isolated yield (240 mg, 47%) as a pale yellow liquid was obtained; Rf (hexane–EtOAc, 90
:
10) = 0.75; found: C, 66.50; H, 4.92. Calc. for C28H24BrClO2: C, 66.22; H, 4.76%; IR νmax: 3061, 3026, 2979, 2926, 1720, 1591, 1561, 1486, 1453, 1371, 1343, 1302, 1276, 1211, 1073, 1046, 1010 cm−1; 1H NMR (200 MHz, CDCl3): δ 7.47 (d, J = 8.6 Hz, 2H, Ar), 7.36–7.15 (m, 11H, Ar, Ph), 6.82 (s, 1H, ArCH), 6.70 (s, 1H, ArCH), 5.29 (s, 1H, C
CHAHB), 5.28 (s, 1H, C
CHAHB), 4.18 (q, J = 7 Hz, 2H, OCH2CH3), 3.87 (s, 2H, PhCH2), 1.17 (t, J = 7 Hz, 3H, OCH2CH3); 13C NMR (50 MHz, CDCl3): δ 168.4, 147.9, 139.4, 139.1, 138.9, 136.0, 134.4, 134.2, 133.7, 131.5, 131.1, 130.1, 129.6, 128.8, 128.5, 128.4, 127.3, 126.6, 126.2, 122.5, 118.2, 61.2, 35.4, 13.9.
(2Z,4E)-Ethyl 2-(4-methoxybenzylidene)-4-benzyl-5-(3-chlorophenyl)-3-methylenepent-4-enoate (11h). Following the general procedure, an isolated yield (28 mg, 15%) as a colourless liquid was obtained; Rf (hexane–EtOAc, 95
:
5) = 0.28; IR νmax: 3061, 3029, 2956, 2930, 2838, 1714, 1709, 1601, 1565, 1507, 1453, 1376, 1295, 1255, 1201, 1178, 1097, 962 cm−1; 1H NMR (300 MHz, CDCl3): δ 7.34–7.17 (m, 11H, Ar, Ph), 6.88 (d, J = 8.7 Hz, 2H, Ar), 6.86 (s, 1H, ArCH), 6.75 (s, 1H, ArCH), 5.29 (s, 1H, C
CHAHB), 5.26 (s, 1H, C
CHAHB), 4.23 (q, J = 7.2 Hz, 2H, OCH2CH3), 3.89 (s, 2H, PhCH2), 3.84 (s, 3H, OCH3), 1.21 (t, J = 7.2 Hz, 3H, OCH2CH3); 13C NMR (75 MHz, CDCl3): δ 169.0, 159.8, 148.4, 139.7, 139.3, 139.1, 134.8, 134.2, 133.1, 132.2, 140.0, 130.3, 129.6, 128.8, 128.5, 128.4, 127.2, 126.6, 126.1, 117.6, 113.8, 61.0, 55.3, 35.4, 14.0; HRMS (ESI-TOF) m/z: [M + H]+ calcd for C29H28ClO3 459.1721; found 459.1723.
(2Z,4E)-Ethyl 2-[(E)-3-benzyl-4-(3-chlorophenyl)buta-1,3-dien-2-yl]-5-phenylpenta-2,4-dienoate (11i). Following the general procedure, an isolated yield (37 mg, 27%) as a yellow liquid was obtained; Rf (hexane–EtOAc, 95
:
5) = 0.54; found: C, 79.25; H, 5.80. Calc. for C30H27ClO2: C, 79.19; H, 5.98%; IR νmax: 3058, 3027, 2979, 2929, 2869, 1705, 1615, 1592, 1561, 1496, 1476, 1447, 1367, 1317, 1304, 1221, 1206, 1159, 1150, 1096, 1045, 1033 cm−1; 1H NMR (300 MHz, CDCl3): δ 7.86 (dd, J = 15.6, 11.4 Hz, 1H, PhCH
CHCH), 7.53–7.50 (m, 2H, Ar), 7.39–7.11 (m, 12H, Ar, 2 × Ph), 6.82 (d, J = 15.6 Hz, 1H, PhCH
CHCH), 6.73 (s, 1H, ArCH), 6.70 (dd, J = 11.4, 0.9 Hz, 1H, PhCH
CHCH
C), 5.31 (s, 1H, C
CHAHB), 5.23 (s, 1H, C
CHAHB), 4.27 (q, J = 7.2 Hz, 2H, OCH2CH3), 3.89 (s, 2H, PhCH2), 1.31 (t, J = 7.2 Hz, 3H, OCH2CH3); 13C NMR (75 MHz, CDCl3): δ 167.0, 148.6, 141.5, 140.2, 139.8, 139.6, 139.2, 136.6, 134.2, 132.3, 130.4, 129.5, 128.8, 128.5, 128.2, 127.3, 127.1, 126.6, 126.1, 125.4, 117.7, 60.7, 34.8, 14.3.
Diethyl 2-[(E)-3-benzyl-4-(3-chlorophenyl)buta-1,3-dien-2-yl]maleate (11j). Following the general procedure, an isolated yield (54 mg, 40%) as a colourless liquid was obtained. The product was contaminated with 36% of diethyl 2-[(E)-3-benzyl-4-(3-chlorophenyl)buta-1,3-dien-2-yl]fumarate and could not be separated. Rf (hexane–EtOAc, 95
:
5) = 0.26; found: C, 70.80; H, 5.98. Calc. for C25H25ClO4: C, 70.67; H, 5.93%; IR νmax: 3063, 3026, 2979, 2934, 2906, 2871, 1723, 1615, 1591, 1562, 1241, 1182, 1100, 1027 cm−1; 1H NMR (500 MHz, CDCl3): δ 7.34–7.14 (m, 18H, Ar, Ph), 6.75 (s, 1H, ArCH, Z), 6.40 (s, 1H, ArCH, E), 5.99 (s, 1H, CHCO2Et, Z), 5.91 (s, 1H, CHCO2Et, E), 5.44 (s, 1H, C
CHAHB, E), 5.35 (s, 1H, C
CHAHB, Z), 5.30 (s, 1H, C
CHAHB, Z), 5.06 (s, 1H, C
CHAHB, E), 4.40 (q, J = 7.0 Hz, 2H, OCH2CH3, E), 4.33 (q, J = 7.0 Hz, 2H, OCH2CH3, Z), 4.24 (q, J = 7.0 Hz, 2H, OCH2CH3, Z), 4.19 (q, J = 7.0 Hz, 2H, OCH2CH3, E), 3.81 (s, 2H, PhCH2, Z), 3.58 (s, 2H, PhCH2, E), 1.39 (t, J = 7.0 Hz, 3H, OCH2CH3, E), 1.33 (t, J = 7.0 Hz, 3H, OCH2CH3, Z), 1.32 (t, J = 7.0 Hz, 3H, OCH2CH3, Z), 1.28 (t, J = 7.0 Hz, 3H, OCH2CH3, E); 13C NMR (125 MHz, CDCl3): δ 167.3 (E), 167.2 (Z), 164.9 (E), 164.8 (Z), 148.3 (Z), 146.9 (E), 145.4 (Z), 142.4 (E), 139.9 (E), 138.9 (Z), 138.5 (Z), 138.4 (Z), 138.0 (E), 137.7 (E), 134.3 (Z), 134.1 (E), 131.8 (Z), 129.6 (E), 129.4 (Z), 128.9 (E), 128.8 (Z), 128.6 (E), 128.5, 127.4 (Z), 127.2 (E), 126.6 (Z), 126.5 (E), 126.3 (Z), 123.9 (E), 121.1 (Z), 121.0 (E), 118.5 (E), 61.6, 61.0, 45.5 (E), 35.6 (Z), 14.1, 14.0.
(2Z,4E)-Ethyl 2-benzylidene-5-(4-methoxyphenyl)-4-methyl-3-methylenepent-4-enoate (11k). Following the general procedure, an isolated yield (100 mg, 45%) as a colourless liquid was obtained; Rf (hexane–EtOAc, 95
:
5) = 0.42; found: C, 79.52; H, 6.89. Calc. for C23H24O3: C, 79.28; H, 6.94%; IR νmax: 3070, 3036, 2364, 2326, 1962, 1812, 1717, 1611, 1509, 1479, 1251, 1214, 1177, 1036 cm−1; 1H NMR (300 MHz, CDCl3): δ 7.36–7.26 (m, 7H, Ar, Ph), 6.91 (d, J = 8.4 Hz, 2H, Ar), 6.85 (s, 1H, PhCH), 6.62 (s, 1H, ArCH), 5.37 (s, 1H, C
CHAHB), 5.32 (s, 1H, C
CHAHB), 4.22 (q, J = 6.9 Hz, 2H, OCH2CH3), 3.84 (s, 3H, OCH3), 2.13 (s, 3H, C
CCH3), 1.17 (t, J = 6.9 Hz, 3H, OCH2CH3); 13C NMR (75 MHz, CDCl3): δ 169.1, 158.3, 150.5, 135.6, 135.5, 134.7, 130.4, 129.7, 128.5, 128.3, 115.3, 113.6, 61.0, 55.3, 17.0, 13.9.
(2Z,4E)-2-Benzylidene-5-(4-methoxyphenyl)-4-methyl-3-methylenepent-4-en-1-ol (11l). To a solution of dendralene 11k (151 mg, 0.43 mmol) in dichloromethane (10 mL), DIBAL-H (1.74 mL, 1.0 M in cyclohexane, 1.73 mmol) was added at −78 °C under an argon atmosphere, and the solution was stirred for 1 h at the same temperature. The temperature of the reaction mixture was raised to 0 °C, stirred for another 1 h and finally allowed to attain room temperature. The reaction mixture was quenched with dil. HCl and extracted with dichloromethane. The organic layer was concentrated under reduced pressure and the residue was purified by column chromatography (petroleum ether–EtOAc; 90
:
10) to obtain the dendralene 11l (105 mg, 80%) as a colourless liquid. Rf (hexane–EtOAc, 80
:
20) = 0.4; found: C, 82.15; H, 7.32. Calc. for C21H22O2: C, 82.32; H, 7.24%; IR νmax: 3426, 3058, 3034, 2951, 2933, 2838, 1607, 1511, 1480, 1441, 1303, 1248, 1177, 1034 cm−1; 1H NMR (300 MHz, CD3COCD3): δ 7.49 (d, J = 7.5 Hz, 2H, Ph), 7.37–7.25 (m, 5H, Ar, Ph), 6.90 (d, J = 8.7 Hz, 2H, Ar), 6.64 (s, 1H, PhCH), 6.53 (s, 1H, ArCH), 5.27 (s, 1H, C
CHAHB), 5.24 (s, 1H, C
CHAHB), 4.36 (d, J = 5.4 Hz, 2H, CH2OH), 3.89 (t, J = 5.4 Hz, 1H, CH2OH), 3.78 (s, 3H, OCH3), 2.05 (s, 3H, C
CCH3); 13C NMR (75 MHz, CD3COCD3): δ 155.6, 143.2, 138.3, 136.8, 132.8, 131.6, 130.3, 129.3, 128.3, 114.7, 113.6, 60.3, 55.8, 17.6.
(3aSR,4SR,7aRS)-Ethyl 4-(4-bromophenyl)-2,3,3a,4,7,7a-hexahydro-6-[(E)-1-(4-methoxyphenyl]-3-phenylprop-1-en-2-yl)-2-methyl-1,3-dioxo-1H-isoindole-5-carboxylate (15). A solution of dendralene 11b (60 mg, 0.12 mmol, 1 equiv.) and NMM (15 mg, 0.14 mmol, 1 equiv.) in benzene (3 mL) was heated under reflux for 3 d. The reaction mixture was then concentrated on a rotary evaporator and the residue was filtered through a small silica gel column to obtain the D–A adducts (57 mg, 78%), which on careful chromatography on silica gel provided the major adduct 15 (50 mg, 69%) as a white solid. Rf (hexane–EtOAc, 70
:
30) = 0.36; mp 119 °C; found: C, 66.28; H, 5.39; N, 2.05. Calc. for C34H32BrNO5: C, 66.45; H, 5.25; N, 2.28%; IR νmax: 3062, 3023, 2979, 2954, 2932, 2838, 1775, 1702, 1606, 1510, 1488, 1434, 1380, 1281, 1253, 1220, 1178, 1122, 1075, 1025, 1008, 969 cm−1; 1H NMR (500 MHz, CDCl3): δ 7.30 (d, J = 8.5 Hz, 2H, Ar), 7.29–7.23 (m, 5H, Ph), 7.19 (d, J = 8.0 Hz, 2H, Ar), 6.90 (d, 2H, J = 8.5 Hz, Ar), 6.63 (d, J = 8.5 Hz, 2H, Ar), 6.45 (s, 1H, ArCH), 4.46 (d, J = 7.5 Hz, 1H, ArCHCH), 4.10 (q, J = 7.5 Hz, 1H, OCHAHBCH3), 4.09 (q, J = 7.5 Hz, 1H, OCHAHBCH3), 4.06 (d, J = 15.0 Hz, 1H, PhCHAHB), 3.83 (d, J = 17.0 Hz, 1H, PhCHAHB), 3.81 (s, 3H, OCH3), 3.07 (t, J = 7.5 Hz, 1H, ArCHCH), 2.72–2.67 (m, 1H, COCHCHAHB), 2.65 (dd, J = 18.5, 6 Hz, 1H, COCHCHAHB), 2.53 (dd, J = 18.5, 10.5 Hz, 1H, COCHCHAHB), 2.40 (s, 3H, NCH3), 1.16 (t, J = 7.5 Hz, 3H, OCH2CH3); 13C NMR (125 MHz, CDCl3): δ 178.7, 177.0, 167.2, 158.8, 150.1, 140.4, 138.6, 135.1, 131.4, 130.3, 130.1, 129.5, 129.2, 128.4, 127.7, 126.6, 121.5, 113.9, 60.9, 55.3, 45.1, 41.9, 37.6, 37.0, 28.7, 24.0, 14.1. Recrystallization of compound 15 from ethylacetate/hexane gave crystals suitable for single crystal X-ray analysis.
(3aSR,4SR,7aRS)-Ethyl 4-(4-bromophenyl)-6-[(E)-1-(3-chlorophenyl)-3-phenylprop-1-en-2-yl]-2,3,3a,4,7,7a-hexahydro-2-methyl-1,3-dioxo-1H-isoindole-5-carboxylate (16). A solution of dendralene 11g (155 mg, 0.3 mmol, 1 equiv.) and NMM (34 mg, 0.3 mmol, 1 equiv.) in toluene (5 mL) was heated under reflux for 2 d. The reaction mixture was then concentrated on a rotary evaporator and the residue was filtered through a small silica gel column to obtain the D–A adducts (148 mg, 78%), which on careful chromatography on silica gel provided the major adduct 16 (118 mg, 62%) as a white solid. Rf (hexane–EtOAc, 80
:
20) = 0.16; mp 140–141 °C; found: C, 64.13; H, 4.77; N, 2.09. Calc. for C33H29BrClNO4: C, 64.04; H, 4.72; N, 2.26%; IR νmax: 3024, 2977, 2929, 2849, 1778, 1703, 1591, 1562, 1486, 1434, 1382, 1276, 1220, 1124, 1074, 1047, 1010 cm−1; 1H NMR (200 MHz, CDCl3): δ 7.37–7.19 (m, 11H, Ar, Ph), 6.64 (d, J = 8.4 Hz, 2H, Ar), 6.44 (s, 1H, ArCH), 4.49 (d, J = 7.2 Hz, 1H, ArCHCH), 4.14 (q, J = 7.2 Hz, 1H, OCHACHBCH3), 4.13 (q, J = 7.0 Hz, 1H, OCHACHBCH3), 4.05 (d, J = 15.4 Hz, 1H, PhCHACHB), 3.84 (d, J = 14.6 Hz, 1H, PhCHACHB), 3.08 (t, J = 7.6 Hz, 1H, ArCHCH), 2.75–2.46 (m, 3H, COCHCH2), 2.42 (s, 3H, NCH3), 1.20 (t, J = 7.2 Hz, 3H, OCH2CH3); 13C NMR (50 MHz, CDCl3): δ 178.6, 176.9, 166.6, 149.9, 143.6, 138.4, 138.1, 135.0, 134.3, 131.4, 130.2, 129.7, 129.6, 128.8, 128.6, 128.5, 127.3, 126.9, 126.8, 126.2, 121.5, 61.0, 45.0, 41.7, 37.4, 37.1, 28.9, 24.0, 14.1. Recrystallization of compound 16 from ethanol gave crystals suitable for single crystal X-ray analysis.
(2Z)-Ethyl 2-((3aRS,4RS,7aSR)-2,3,3a,4,7,7a-hexahydro-4-(4-methoxyphenyl)-2,5-dimethyl-1,3-dioxo-1H-isoindol-6-yl)-3-phenylacrylate (17) and (3aSR,4SR,7aRS)-ethyl 2,3,3a,4,7,7a-hexahydro-6-[(E)-1-(4-methoxyphenyl)prop-1-en-2-yl]-2-methyl-1,3-dioxo-4-phenyl-1H-isoindole-5-carboxylate (18). A solution of dendralene 11k (126 mg, 0.36 mmol, 1 equiv.) and NMM (41 mg, 0.36 mmol, 1 equiv.) in toluene (3 mL) was heated under reflux for 1 d. The reaction mixture was then concentrated on a rotary evaporator and chromatographed to obtain the D–A adducts as an inseparable 54
:
46 mixture of 17/18 (149 mg, 89%). Rf (hexane–EtOAc, 70
:
30) = 0.3; found: C, 73.07; H, 6.56; N, 3.07. Calc. for C28H29NO5: C, 73.18; H, 6.36; N, 3.05%; IR νmax: 3023, 2981, 2940, 2906, 2837, 1780, 1713, 1696, 1610, 1511, 1437, 1382, 1255, 1175, 1035, 760, 697 cm−1; 1H NMR (500 MHz, CDCl3): δ 7.40–7.31 (m, 5H, Ph, 17), 7.30–7.18 (m, 5H, Ar, Ph, 18), 7.09 (d, J = 7.0 Hz, 2H, Ph, 18), 7.08 (d, J = 8.5 Hz, 2H, Ar, 17), 6.92 (d, J = 8.5 Hz, 2H, Ar, 18), 6.81 (d, J = 9.0 Hz, 2H, Ar, 17), 6.77 (s, 1H, PhCH, 17), 6.27 (s, 1H, ArCH, 18), 4.56 (d, J = 7.5 Hz, 1H, PhCH, 18), 4.21 (q, J = 7.0 Hz, 2H, OCH2CH3, 17), 4.03 (q, J = 7.0 Hz, 1H, OCH2CH3, 18), 3.85 (d, J = 5.0 Hz, 1H, ArCH, 17), 3.85 (s, 3H, OCH3, 18), 3.79 (s, 3H, OCH3, 17), 3.39 (t, J = 8.0 Hz, 1H, PhCHCH, 18), 3.33 (t, J = 9.0 Hz, 1H, ArCHCH, 17), 3.23–3.15 (m, 3H, COCHCHAHB, 18, COCHCH2, 17), 3.08–2.98 (m, 1H, COCHCHAHB, 17), 2.82 (dd, J = 20.0, 12.0 Hz, 1H, COCHCHAHB, 18), 2.73 (dd, J = 18.0, 11.5 Hz, 1H, COCHCHAHB, 17), 2.47 (s, NCH3, 3H, 17), 2.40 (s, 3H, NCH3, 18), 2.15 (d, J = 0.5 Hz, 3H, C
CCH3, 18), 1.81 (s, 3H, C
CCH3, 17), 1.19 (t, J = 7.0 Hz, 3H, OCH2CH3, 17), 1.09 (t, J = 7.0 Hz, 3H, OCH2CH3, 18); 13C NMR (50 MHz, CDCl3): δ 179.5 (17), 179.0 (18), 178.0 (17), 177.2 (18), 168.1 (17), 167.7 (18), 158.9 (17), 158.4 (18), 150.6 (18), 137.6 (17), 136.8 (18), 136.6 (17), 135.5 (17), 134.6 (17), 134.3 (17), 130.1, 130.0, 129.9, 129.8, 128.6, 128.4, 128.3, 128.2, 127.6, 127.0, 125.9, 113.7 (17), 113.6 (18), 61.0 (17), 60.6 (18), 55.2 (18), 55.1 (17), 46.2 (18), 45.2 (17), 45.1 (17), 42.0 (18), 37.5 (18), 37.4 (17), 29.7 (18), 26.6 (18), 25.9 (17), 23.9 (17), 23.8 (18), 20.3 (17), 17.4 (18), 13.8 (17). Structures were confirmed from the mixture by COSY and ROESY NMR (for details, see the ESI†).
Synthesis of compounds 19, 20 and 21. A solution of dendralene 6l (48 mg, 0.16 mmol, 1 equiv.) and NMM (21 mg, 0.19 mmol, 1 equiv.) in toluene (3 mL) was heated under reflux for 1 d. The reaction mixture was then concentrated on a rotary evaporator and the residue, on careful chromatography on silica gel, provided the D–A adducts 19 (52 mg, 65%) as a white solid, 20 (8 mg, 10%) as foam and 21 (3 mg, 4%) as a gummy solid (combined yield: 79%).
(3aRS,4RS,7aSR)-3a,4,7,7a-Tetrahydro-6-[(Z)-1-hydroxy-3-phenylprop-2-en-2-yl]-4-(4-methoxyphenyl)-2,5-dimethyl-2H-isoindole-1,3-dione (19). Rf (hexane–EtOAc, 60
:
40) = 0.21; mp 117–118 °C; IR νmax: 3443, 3059, 3021, 2936, 2906, 2853, 2840, 1777, 1694, 1610, 1507, 1434, 1386, 1249, 1178, 1135, 1034 cm−1; 1H NMR (500 MHz, CDCl3): δ 7.39–7.36 (m, 2H, Ph), 7.30–7.28 (m, 3H, Ph), 7.07 (d, J = 8.5 Hz, 2H, Ar), 6.78 (d, J = 8.5 Hz, 2H, Ar), 6.48 (s, 1H, PhCH), 4.53 (d, J = 12.5 Hz, 1H, CHAHBOH), 4.49 (d, J = 12.5 Hz, 1H, CHAHBOH), 3.82 (d, J = 7.0 Hz, 1H, ArCHCH), 3.75 (s, 3H, OCH3), 3.31 (t, J = 8.5 Hz, 1H, ArCHCH), 3.19–3.14 (m, 1H, COCHCH2), 3.02 (d, J = 18.0 Hz, 1H, COCHCHAHB), 2.73 (dd, J = 18.0, 11.5 Hz, 2H, COCHCHAHB), 2.45 (s, 3H, NCH3), 1.80 (s, 3H, C
CCH3); 13C NMR (125 MHz, CDCl3): δ 179.9, 178.2, 158.8, 141.3, 136.3, 132.4, 132.0, 130.6, 130.0, 129.0, 128.7, 128.3, 127.2, 113.7, 60.3, 55.1, 46.0, 45.2, 37.3, 25.7, 23.8, 20.2. Recrystallization of compound 19 from ethylacetate/hexane gave crystals suitable for single crystal X-ray analysis.
(3aRS,4SR,7aSR)-3a,4,7,7a-Tetrahydro-5-(hydroxymethyl)-6-[(E)-1-(4-methoxyphenyl)prop-1-en-2-yl]-2-methyl-4-phenyl-2H-isoindole-1,3-dione (20). Rf (hexane–EtOAc, 60
:
40) = 0.17; found: C, 74.60; H, 6.67; N, 3.26. Calc. for C26H27NO4: C, 74.80; H, 6.52; N, 3.35%; IR νmax: 3457, 3035, 2925, 2854, 1700, 1510, 1434, 1251, 1034 cm−1; 1H NMR (500 MHz, C6D6): δ 7.36 (d, J = 7.5 Hz, 2H, Ph), 7.28 (d, J = 8.5 Hz, 2H, Ar), 7.17 (t, J = 7.5 Hz, 2H, Ph), 7.07 (t, J = 7.5 Hz, 1H, Ph), 6.94 (d, J = 8.5, 2H, Ar), 6.48 (s, 1H, ArCH), 4.47 (d, J = 7 Hz, 1H, PhCH), 4.35 (d, J = 12 Hz, 1H, CHACHBOH), 3.79 (dd, J = 12, 2 Hz, 1H, CHACHBOH), 3.46 (s, 3H, OCH3), 3.18 (m, 2H, COCHCHAHB, CH2OH), 3.02 (t, J = 7.5 Hz, 1H, PhCHCH), 2.53–2.45 (m, 2H, COCHCHAHB), 2.39 (s, 3H, NCH3), 1.96 (s, 3H, C
CCH3); 13C NMR (125 MHz, CDCl3): δ 179.6, 177.9, 158.3, 140.3, 137.4, 135.6, 132.1, 130.1, 129.6, 128.9, 128.4, 127.8, 127.5, 113.6, 62.2, 55.2, 45.4, 41.8, 37.4, 25.3, 23.7, 17.8.
(3aSR,4RS,7aRS)-3a,4,7,7a-Tetrahydro-6-[(Z)-1-hydroxy-3-phenylprop-2-en-2-yl]-4-(4-methoxyphenyl)-2,5-dimethyl-2H-isoindole-1,3-dione (21). Rf (hexane–EtOAc, 60
:
40) = 0.4; IR νmax: 3498, 3019, 2932, 1774, 1697, 1610, 1510, 1441, 1250, 1034, 755 cm−1; 1H NMR (500 MHz, CDCl3): δ 7.37–7.25 (m, 7H, Ar, Ph), 6.91 (d, J = 8.5 Hz, 2H, Ar), 6.34 (s, 1H, PhCH), 4.39 (d, J = 13.5 Hz, 1H, CHAHBOH), 4.37 (d, J = 13.0 Hz, 1H, CHAHBOH), 4.13 (s, 1H, ArCH), 3.81 (s, 3H, OCH3), 3.40 (d, J = 8.5 Hz, 1H, ArCHCH), 3.12 (t, J = 8.0 Hz, 1H, COCHCHAHB), 3.01 (s, 3H, NCH3), 2.70 (d, J = 15.5 Hz, 1H, COCHCHAHB), 2.44–2.39 (m, 1H, COCHCH2), 1.92 (d, J = 2.0 Hz, 3H, C
CCH3); 13C NMR (125 MHz, CDCl3): δ 180.8, 179.4, 158.3, 140.7, 136.2, 134.1, 133.5, 131.3, 130.7, 128.8, 128.3, 128.2, 127.2, 114.3, 59.7, 55.2, 47.1, 45.7, 39.6, 28.1, 25.1, 21.0.
Acknowledgements
Mr Gonna Somu Naidu is thankful to Council of Scientific and Industrial Research (CSIR), New Delhi for a research fellowship. X-ray crystallographic data for compound 16 was collected at the X-ray diffraction facility, IIT, Indore.
References
- H. Hopf, in Classics in Hydrocarbon Chemistry: Syntheses, Concepts, Perspectives, Wiley-VCH, Weinheim, 2000, ch. 7, p. 103 Search PubMed.
- H. Hopf, Angew. Chem., Int. Ed., 2001, 40, 705 CrossRef CAS.
- H. Hopf, Nature, 2009, 460, 183 CrossRef CAS PubMed.
- H. Hopf and M. S. Sherburn, Angew. Chem., Int. Ed., 2012, 51, 2298 CrossRef CAS PubMed.
- E. G. Mackay and M. S. Sherburn, Pure Appl. Chem., 2013, 85, 1227 CrossRef CAS.
- M. S. Sherburn, Acc. Chem. Res., 2015, 48, 1961 CrossRef CAS PubMed.
- W. J. Bailey, J. Economy and M. E. Hermes, J. Org. Chem., 1962, 27, 3295 CrossRef CAS.
- R. C. Blume, U.S. Pat. 3,860,669, 1973.
- C. D. Diakoumakos and J. A. Mikroyannidis, J. Appl. Polym. Sci., 1994, 53, 201 CrossRef CAS.
- K. Tekenaka, S. Amamoto, H. Kishi, H. Takeshita, M. Miya and T. Shiomi, Macromolecules, 2013, 46, 7282 CrossRef.
- A. L. Kanibolotsky, J. C. Forgie, G. J. McEntee, M. M. A. Talpur, P. J. Skabara, T. D. J. Westgate, J. J. W. McDouall, M. Auinger, S. J. Coles and M. B. Hursthouse, Chem.–Eur. J., 2009, 15, 11581 CrossRef CAS PubMed.
- R. R. Amaresh, D. Liu, T. Konovalova, M. V. Lakshmikantham, M. P. Cava and L. D. Kispert, J. Org. Chem., 2001, 66, 7757 CrossRef CAS PubMed.
- M. R. Bryce, M. A. Coffin, P. J. Skabara, A. J. Moore, A. S. Batsanov and J. A. K. Howard, Chem.–Eur. J., 2000, 6, 1955 CrossRef CAS.
- Y. Kajiwara, S. Hino and Y. Misaki, Bull. Chem. Soc. Jpn., 2012, 85, 830 CrossRef CAS.
- M. Hasegawa, M. Watanabe and Y. Misaki, J. Synth. Org. Chem., Jpn., 2013, 71, 1268 CrossRef CAS.
- U. Fleischer, W. Kutzelnigg, P. Lazzeretti and V. Muhlenkamp, J. Am. Chem. Soc., 1994, 116, 5298 CrossRef CAS.
- O. Tsuge, E. Wada and S. Kanemasa, Chem. Lett., 1983, 12, 1525 CrossRef.
- B. S. Radovic, M. Careri, A. Mangia, M. Musci, M. Gerboles and E. Anklam, Food Chem., 2001, 72, 511 CrossRef CAS.
- M. Rahman, M. Yusaf, J. U. Chowdhury and M. A. Wahab, Bangladesh J. Sci. Ind. Res., 1987, 22, 152 Search PubMed.
- M. A. Sefton, I. L. Francis and P. J. Williams, J. Food Sci., 1994, 59, 142 CrossRef CAS.
- A. Arnone, R. Cardillo, V. Dimodugno and G. Nasini, J. Chem. Soc., Perkin Trans. 1, 1989, 1995 RSC.
- W. J. Bailey and J. Economy, J. Am. Chem. Soc., 1955, 77, 1133 CrossRef CAS.
- A. T. Blomquist and J. A. Verdol, J. Am. Chem. Soc., 1955, 77, 1806 CrossRef CAS.
- H. Priebe and H. Hopf, Angew. Chem., Int. Ed. Engl., 1982, 21, 286 Search PubMed.
- S. Woo, N. Squires and A. G. Fallis, Org. Lett., 1999, 1, 573 CrossRef CAS.
- T. A. Bradford, A. D. Payne, A. C. Willis, M. N. Paddon-Row and M. S. Sherburn, J. Org. Chem., 2010, 75, 491 CrossRef CAS PubMed.
- S. Fielder, D. D. Rowan and M. S. Sherburn, Angew.
Chem., Int. Ed., 2000, 39, 4331 CrossRef CAS.
- J. I. G Cadogan, S. Cradock, S. Gillam and I. Gosney, J. Chem. Soc., Chem. Commun., 1991, 114 RSC.
- A. D. Payne, G. Bojase, M. N. Paddon-Row and M. S. Sherburn, Angew. Chem., Int. Ed., 2009, 48, 4836 CrossRef CAS PubMed.
- T. A. Bradford, A. D. Payne, A. C. Willis, M. N. Paddon-Row and M. S. Sherburn, Org. Lett., 2007, 9, 4861 CrossRef CAS PubMed.
- N. A. Miller, A. C. Willis, M. N. Paddon-Row and M. S. Sherburn, Angew. Chem., Int. Ed., 2007, 46, 937 CrossRef CAS PubMed.
- G. Bojase, A. D. Payne, A. C. Willis and M. S. Sherburn, Angew. Chem., Int. Ed., 2008, 47, 910 CrossRef CAS PubMed.
- A. D. Payne, A. C. Willis and M. S. Sherburn, J. Am. Chem. Soc., 2005, 127, 12188 CrossRef CAS PubMed.
- N. Thies and E. Haak, Angew. Chem., Int. Ed., 2015, 54, 4097 CrossRef CAS PubMed.
- K. M. Brummondo, H. Chen, P. Sill and L. You, J. Chem. Soc., 2002, 124, 15186 CrossRef.
- S. Brase, H. Wertal, D. Frank, D. Vidovic and A. Meijere, Eur. J. Org. Chem., 2005, 4167 CrossRef CAS.
- M. Arisawa, T. Sugihara and M. Yamaguchi, Chem. Commun., 1998, 2615 RSC.
- T. Miura, T. Biyajima, T. Toyoshima and M. Murakami, Beilstein J. Org. Chem., 2011, 7, 578 CrossRef CAS PubMed.
- K. Beydoun, H.-J. Zhang, B. Sundararaju, B. Demerseman, M. Achard, Z. Xi and C. Bruneau, Chem. Commun., 2009, 6580 RSC.
- H. Srour, K. Abidi, Z. Sahli, B. Sundararaju, N. Hamdi, M. Achard and C. Bruneau, ChemCatChem, 2011, 3, 1876 CrossRef CAS.
- J. L. Notre, A. A. Martinez, P. H. Dixneuf and C. Bruneau, Tetrahedron, 2003, 59, 9425 CrossRef.
- B. K. D. Kim, Y. Do and S. Chang, Org. Lett., 2003, 5, 3041 CrossRef PubMed.
- H. Wang, B. Beiring, D. G. Yu, K. D. Collins and F. Glorius, Angew. Chem., Int. Ed., 2013, 52, 12430 CrossRef CAS PubMed.
- C. J. Reider, K. J. Winberg and F. G. West, J. Org. Chem., 2011, 76, 50 CrossRef PubMed.
- H. L. Shimp, A. Hare, M. McLaughlin and G. C. Micalizio, Tetrahedron, 2008, 64, 3437 CrossRef PubMed.
- S. Kim, D. Seomoon and P. H. Lee, Chem. Commun., 2009, 1873 RSC.
- C. S. Chin, H. Lee, H. Park and M. Kim, Organometallics, 2002, 21, 3889 CrossRef CAS.
- S. K. Ghosh, R. Singh and S. M. Date, Chem. Commun., 2003, 636 RSC.
- E. J. Corey and M. Chaykovsky, J. Am. Chem. Soc., 1965, 87, 1353 CrossRef CAS.
- S. M. Date, R. Singh and S. K. Ghosh, Org. Biomol. Chem., 2005, 3, 3369 CAS.
- S. M. Date and S. K. Ghosh, Angew. Chem., Int. Ed., 2007, 46, 386 CrossRef CAS PubMed.
- S. M. Date and S. K. Ghosh, Bull. Chem. Soc. Jpn., 2004, 77, 2099 CrossRef CAS.
- R. Singh and S. K. Ghosh, Org. Lett., 2007, 9, 5071 CrossRef CAS PubMed.
- R. Singh and S. K. Ghosh, Tetrahedron, 2010, 66, 2284 CrossRef CAS.
- R. Singh, G. C. Singh and S. K. Ghosh, Tetrahedron Lett., 2005, 46, 4719 CrossRef CAS.
- R. Singh, G. C. Singh and S. K. Ghosh, Eur. J. Org. Chem., 2007, 5376 CrossRef CAS.
- S. K. Ghosh, R. Singh and G. C. Singh, Eur. J. Org. Chem., 2004, 4141 CrossRef CAS.
- R. Singh and S. K. Ghosh, Chem. Commun., 2011, 47, 10809 RSC.
- R. Singh and S. K. Ghosh, Tetrahedron: Asymmetry, 2014, 25, 57 CrossRef CAS.
- M. N. Paddon-Row and M. S. Sherburn, Chem. Commun., 2012, 48, 832 RSC.
- H. Toombs-Ruane, E. L. Pearson, M. N. Paddon-Row and M. S. Sherburn, Chem. Commun., 2012, 48, 6639 RSC.
- A. Erkkila and P. M. Pihko, J. Org. Chem., 2006, 71, 2538 CrossRef PubMed.
- K. Speck and T. Magauer, Beilstein J. Org. Chem., 2013, 9, 2048 CrossRef PubMed.
- S. Kiyooka, H. Fujimoto, M. Mishima, S. Kobayashi, K. M. Uddin and M. Fujio, Tetrahedron Lett., 2003, 44, 927 CrossRef CAS.
- N. Lahmar, J. Aatar, T. B. Ayed, H. Amri and M. Bellassoued, J. Organomet. Chem., 2006, 691, 3018 CrossRef CAS.
Footnote |
† Electronic supplementary information (ESI) available: Copies of the 1H NMR spectra for compounds 10–21, 13C NMR spectra for 11–21, 31P NMR of 12a–c, COSY and ROESY for compounds (17 & 18), 20, and X-ray crystallographic data for compounds 11a, 15, 16 and 19. CCDC 1427878, 1427914, 1427900 and 1427915. For ESI and crystallographic data in CIF or other electronic format see DOI: 10.1039/c6ra01729k |
|
This journal is © The Royal Society of Chemistry 2016 |