DOI:
10.1039/C6RA01158F
(Paper)
RSC Adv., 2016,
6, 24797-24807
Synthesis of 1,3,4-oxadiazoles as promising anticoagulant agents
Received
14th January 2016
, Accepted 25th February 2016
First published on 26th February 2016
Abstract
In the present study, a series of 2,5-disubstituted-1,3,4-oxadiazole derivatives (4a–4k) were designed and subjected to molecular docking simulation studies onto the enzymes vitamin K epoxide reductase (PDB: 3KP9) and factor Xa (PDB: 1NFY) to visualize their binding affinity towards the said target proteins. In silico ADME studies highlighted that the designed compounds are safe enough and have the potential to be considered as drug like molecules, indicating the appreciable ADME property & probable toxicity of the designed compounds. The title compounds were synthesized from (benzo[d]oxazol-2-yl)methanamine and evaluated for in vitro radical scavenging properties and ex vivo anticoagulant activity. The results of the ex vivo anticoagulant evaluation highlighted that the compounds exhibited a significant increase in prothrombin time and clotting time, and a minimal increase in the activated partial thromboplastin time, indicating that the compounds can be considered for promising anticoagulant therapy. The results of the radical scavenging experiments indicated that the compounds have substantial antioxidant activity.
1. Introduction
Myocardial infarction due to arterial thromboembolism (ATE) is currently the leading cause of death by cardiovascular diseases (CVDs) in developed and developing countries. Cardioembolic events like myocardial infarction and ischemic stroke are primarily due to ATE.1 The recurrent ATE episodes even after treatment by the clinically available anticoagulant medications for the management of ATE disorders indicate the therapeutic shortcomings of the present anticoagulant therapy. During the past decade, the management of acute myocardial infarction disorder has changed, as prevention of complications has been the cornerstone for treatment.2 Anticoagulant therapy plays an important role in the management thromboembolic disorders, employed for both prevention and treatment of ATE. In clinical practices, anticoagulants have marked advantage in the prophylaxis of thrombotic events in both pre- and post-surgery.3 For many decades, 4-hydroxycoumarin anticoagulants majorly warfarin and acenocoumarol, have been the core of anticoagulation therapy and are generally known as antivitamin K or vitamin K epoxide reductase (VKOR) inhibitors.4 These 4-hydroxycoumarin anticoagulants inhibits the oxidation–reduction cycle of vitamin K in the liver and resulting in accumulation of abnormal form of coagulation protein; des-γ-carboxyprothrombin, known as proteins induced by vitamin K antagonism II (PIVKA-II) and inhibiting the coagulation process.5 Clinical studies highlight the advantage of VKOR inhibitors in the prevention of both primary and secondary venous thromboembolism, prevention of systemic embolism in patients with artificial heart valves or in arrhythmic condition like atrial fibrillation. The VKOR inhibitors, which produce their anticoagulant effect by inhibiting the activation of factor II, VII, IX, and X, have the marked advantage due to the inhibition of factor X and II. Factor X occupies a central position at the junction of the extrinsic and intrinsic system, and the activation of factor X is universal in both the pathways. At the same time, inhibition of activation of the factor II (prothrombin) to factor IIa (thrombin), leads to the inhibition of the fibrin formation from fibrinogen. As thrombin is the common end point in both the pathways, it leads to a stable clot formation. The 4-hydroxycoumarin anticoagulants have marked clinical drawback; these agents require frequent monitoring, challenging standardization of laboratory processes, dose response, diet-drug and drug–drug interactions, narrow therapeutic range, therapeutic drug monitoring, purple toe syndrome, eclampsia, risk of haemorrhage and genetic polymorphisms that affect vitamin K antagonist metabolism. These major clinical drawbacks and entire therapeutic shortcomings of these 4-hydroxycoumarin anticoagulants indicate the need for better anticoagulant agents with clinical advantage. The selective direct inhibition of factor Xa protein has marked significance in the management of clinical condition like deep vein thrombosis, pulmonary embolism and also towards prevention of stroke due to systemic embolism. Activation of factor X to factor Xa via the intrinsic and extrinsic pathways play a central role in the cascade of blood coagulation. The factor Xa directly converts prothrombin to thrombin through the prothrombinase complex, and eventually leads to fibrin clot formation and activation of platelets by thrombin. An activated factor X will probably generate more than thousand molecules of thrombin highlighting the amplification significance of the coagulation cascade. Whilst, the prothrombinase bound factor Xa will produce an explosive burst of thrombin generation with more than three hundred thousand fold activity towards the generation of thrombin in comparison to the free factor Xa. Thus selective inhibition of factor Xa will definitely help in controlling the amplified burst of thrombin production and direct inhibitor of factor Xa also exhibits better efficacy, improved safety and thus being beneficial.
Apart from ATE, atherosclerosis is also another primary reason for the development of CVDs. Oxidative stress is termed as the main cause that triggers the atherosclerotic process leading to the development of atherosclerosis plug. The reactive oxygen species, which are continuously produced during cellular metabolism, are regulated and neutralized by the endogenous antioxidant system. An abnormal production of ROS in the physiological system due to environmental factors, lifestyle habits etc. results in a situation called “oxidative stress”. A chronic situation of this oxidative stress leads to cellular damage by altering the hereditary DNA and resulting in lifestyle clinical disorders including CVDs.6 In view of these above fact, the development of an anticoagulant molecule with significant radical scavenging property highlight its dual pharmacological advantage, prevention of CVDs events by reducing the process of atherosclerotic plaque formation due to radical scavenging property and management of CVDs disorders by its anticoagulant activity. The molecular docking application studies have great importance in developing new chemical entities as therapeutic agents. The advantage of understanding the probable protein–ligand interaction at the target enzyme site highlights the ease and depth of ligand interaction. Molecular docking studies impart knowledge about the probable structural modification on the parent moiety via potential substitution that would results in appropriate derivatives with better interaction. The molecular docking studies also has advantage in identification of the right protocol for pharmacological screening and helping to narrow down biological evaluation procedure and in turn minimize the use of animals for pharmacological screening. In the present investigation, we have designed a small library of nitrogen-containing heterocyclic compounds and the designed molecules were docked onto VKOR and factor Xa enzymes, based on the docking scores and interactions best ranked molecules were selected for the synthesis. The synthesized molecules were evaluated for in vitro radical scavenging property by 2,2′-diphenyl-1-picryl hydrazyl radical scavenging assay method and ex vivo anticoagulant activity by blood coagulation study by measuring for an increase in prothrombin time, activated partial thromboplastin time and clotting time. The results highlight that 1,3,4-oxadiazole moiety provides a scaffold on which pharmacophores can be arranged to afford potent and selective drugs as anticoagulants.
2. Results and discussion
2.1. Chemistry
Synthesis of the intermediates and the target compounds (benzo[d]oxazol-2-yl)-N-[(5-subtituted-1,3,4-oxadiazol-2-yl)methyl]methanamine; 4a–4k were accomplished according to the steps depicted (Scheme 1). The synthesis of the title compounds were referred from reported literatures.7–9 The starting material (benzo[d]oxazol-2-yl)methanamine (1) was synthesized by nucleophilic addition of 2-amino phenol with glycine.8 The N-substitution of the benzoxazole derivative 1 with ethyl 2-chloroacetate in the presence of anhydrous potassium carbonate to yield the ester derivative, ethyl 2-[(benzo[d]oxazol-2-yl)methylamino]acetate (2).10 The ester derivative 2 on reaction with an excess of hydrazine monohydrate resulted in acyl hydrazide derivative, 2-[(benzo[d]oxazol-2-yl)methylamino]acetohydrazide (3).7 The acylhydrazide derivative 3 was condensed with appropriate heteroaryl/aryl/aliphatic carboxylic acids in presence of phosphorous oxychloride to undergo dehydrative cyclization and to obtain 1,3,4-oxadiazole derivatives; (benzo[d]oxazol-2-yl)-N-[(5-substituted-1,3,4-oxadiazol-2-yl)methyl]methanamine (4a–4j).7 The mercapto derivative, 5-{[(benzo[d]oxazol-2-yl)methylamino]methyl}-1,3,4-oxadiazole-2-thiol (4k) was prepared by reacting the acylhydrazide 3 with carbon disulphide in the presence of potassium hydroxide.9 In the 1H NMR spectra of N-[(benzo[d]oxazol-2-yl)methyl](5-phenyl-1,3,4-oxadiazol-2-yl)methanamine (4a), the methylene protons bridge between benzoxazole moiety and amino group has resonated at δ 3.6 ppm as doublet and the methylene protons between amino group and oxadiazole moiety as doublet has resonated at δ 3.8 ppm. Furthermore in the proton NMR spectrum, the absence of hydrazide protons at 11.7 and 4.8 ppm, respectively confirms the cyclization and formation of the 1,3,4-oxadiazole moiety. In the IR spectrum of the compound 4a, the absence of the characteristic absorption peak corresponding to the carbonyl group stretching at 1650–1750 cm−1 attributes the formation of the oxadiazole derivative. The 13C NMR spectra of compound 4a, the chemical shifts observed at δ 163.5 and 167.2 ppm can be attributed to C2 and C5 of oxadiazole moiety, respectively. Furthermore, signals at δ 46.3 and 49.7 ppm were assigned to the methylene carbon bridge between the benzoxazole moiety and amino group and between the amino group and oxadiazole moiety, respectively. The mass spectra characterization of the compound 4a, exhibits a M+1 molecular ion peak at 307 m/z and two major fragment ion peaks at 147 and 159 m/z, confirming the formation of the oxadiazole derivative. The ion peak 147 m/z and which is also the base peak was attributed to the 2-aminomethyl benzoxazole fragment. The ion peak 159 m/z may be attributed to the 5-phenyl 1,3,4-oxadiazole fragment. In the 1H NMR spectrum of N-[(benzo[d]oxazol-2-yl)methyl][5-(2-aminophenyl)-1,3,4-oxadiazol-2-yl]methanamine (4d), the amino group protons at the ortho position in the phenyl moiety at position C5 of the oxadiazole nucleus has resonated at δ 5.4 ppm as a singlet. The 13C NMR spectra of compound 4d, the chemical shift at δ 162.1 ppm was assigned for the C2 substituted with the amino group of the phenyl moiety. The mass spectra of the compound 4d, confirms the formation of the derivative by the presence of M+1 molecular ion peak at 322 m/z, and a ion peak at 174 m/z, which can be attributed to the oxadiazole fragment. The base peak 147 m/z was attributed to the 2-aminomethyl benzoxazole fragment. The IR spectrum of compound 4d exhibited a strong absorption at 1266 cm−1 indicating the stretching of C–O–C of the oxadiazole moiety. In the IR spectrum of 5-{[(benzo[d]oxazol-2-yl)methylamino]methyl}-1,3,4-oxadiazole-2-thiol (4k), the absorption band at 2587 cm−1 corresponding to mercapto group stretching and the absorption band at 1283 cm−1 can be attributed to the stretching vibration of C–O–C of the oxadiazole moiety. In proton NMR spectra of the compound 4k, the mercapto proton has resonated at δ 12.9 ppm, and in the 13C NMR spectra of compound 4k, chemical shift at δ 166.2 and 174.7 ppm can be attributed to C2 and C5 of the oxadiazole moiety, which in agreement for the formation of the molecule.
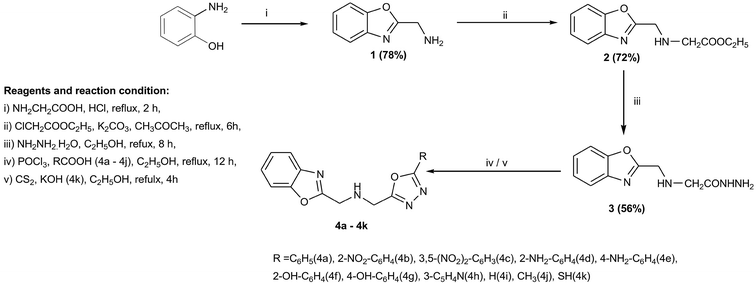 |
| Scheme 1 Synthetic strategy of designed compounds. | |
2.2. In silico ADME results
All the compounds had appropriate values towards Lipinski parameters for biological efficacy with zero to one Lipinski violation. Indicating that the designed compounds compile with Lipinski rules of the five and would have good oral bioavailability. The molecular weight of each ligand was within the range of 230 to 396 D. They also had moderate to high predicted oral availability based on percentage ABS ranging between 51 to 82%. Generally, a compound needs a score less than 5 for lipophilicity, the lipophilicity data suggested that the compounds were optimally lipophilic in nature ranging from −0.04 to 2.35. The designed compounds exhibited a TPSA value less than 140 Å (except compound 4c), highlighting the permeability of the compounds into the cellular plasma membrane. A positive value of drug scores indicates that the molecules contain predominant pharmacophoric groups, which are often found in the active pharmaceutical ingredient. The designed compounds showed positive values in the drug score calculation, the values ranged from 0.34 to 0.90. The results indicate that the designed 1,3,4-oxadiazole have potential as new drug candidates. Table 1 represents the calculated % ABS, TPSA and Lipinski parameters of the synthesized compounds.
Table 1 Predicted ADME, Lipinski parameters and molecular properties of the synthesized compounds
Sl No. |
Cpd |
% ABSa |
TPSAc |
RTBc |
HBAc |
HBDc |
log Pc |
MW |
Violationsc |
log Sb |
Drug likenessb |
Drug scoreb |
Data calculated as per the eqn (1). Molecular property as obtained from OSIRIS property explorer software. Molecular property as obtained from Molinspiration online property calculation toolkit. |
1 |
4a |
82 |
76.98 |
5 |
6 |
1 |
2.35 |
306.32 |
0 |
−3.96 |
2.65 |
0.78 |
2 |
4b |
67 |
122.81 |
6 |
9 |
1 |
2.26 |
351.32 |
0 |
−4.42 |
−5.02 |
0.38 |
3 |
4c |
51 |
168.63 |
7 |
12 |
1 |
2.19 |
396.32 |
1 |
−4.88 |
−8.22 |
0.34 |
4 |
4d |
73 |
103.01 |
5 |
7 |
3 |
1.78 |
321.34 |
0 |
−4.03 |
−0.13 |
0.59 |
5 |
4e |
73 |
103.01 |
5 |
7 |
3 |
1.43 |
321.34 |
0 |
−4.03 |
1.11 |
0.71 |
6 |
4f |
75 |
97.21 |
5 |
7 |
2 |
2.08 |
322.32 |
0 |
−3.66 |
2.15 |
0.79 |
7 |
4g |
75 |
97.21 |
5 |
7 |
2 |
1.87 |
322.32 |
0 |
−3.66 |
1.16 |
0.73 |
8 |
4h |
78 |
89.88 |
5 |
7 |
1 |
1.28 |
307.31 |
0 |
−3.16 |
2.85 |
0.86 |
9 |
4i |
82 |
76.98 |
4 |
6 |
1 |
0.43 |
230.23 |
0 |
−1.81 |
2.09 |
0.9 |
10 |
4j |
82 |
76.98 |
4 |
6 |
1 |
−0.04 |
244.25 |
0 |
−1.44 |
2.08 |
0.9 |
11 |
4k |
82 |
76.98 |
4 |
6 |
1 |
0.95 |
262.29 |
0 |
−2.69 |
−0.18 |
0.67 |
2.3. Molecular docking results
The molecular modeling technique was used to explore, predict and understand the protein/enzyme interactions with our designed 1,3,4-oxadiazoles and also to visualize the probable binding. The docking study was performed using the SYBYL-X 2.1. Wherein, the 2,5-disubstituted-1,3,4-oxadiazoles were docked into the active site of the selected enzymes. In order to visualize the importance of possible anticoagulant property, the compounds were docked onto factor Xa (PDB ID: 1NFY) and VKOR (PDB ID: 3KP9) highlighting its possible interaction with factor Xa and VKOR. Since, 1NFY and 3KP9 have been well established and reported for docking analysis to understand the anticoagulant importance.11,12
The docking study of the 1,3,4-oxadiazoles onto the active site of the enzyme factor Xa (1NFY) were encouraging, as the 1,3,4-oxadiazoles were exhibiting a good docking score and considerable lower crash score in comparison to the co-crystallized ligand. The co-crystallized ligand, RPR132747 exhibited a docking and crash score of 6.2033 and −2.2025 kcal M−1 respectively, with the main hydrophobic interactions with the surrounding residues Cys191, Gly216, Ala190, and Trp215, strongly contributed to the stabilization. The hydrogen and the nitrogen of the imine function exhibited hydrogen bond interaction with the residues Cys191·H and Cys191·O at a distance of 2.887 and 1.988 Å, respectively. The proton of the amino group exhibited hydrogen bond interaction with the residue Trp215 at a distance of 2.512 Å. The oxygen of the keto function of piperazin-2-one moiety exhibited hydrogen bond interaction with the residue Gly218 at a distance of 2.617 Å. The docking study revealed that the 1,3,4-oxadiazole derivatives possessed high affinity towards factor Xa; 1NFY (Fig. 1). The 1,3,4-oxadiazoles; 4a–4k exhibited a docking score 5.7482 to 8.2495 kcal M−1. Compound 4d (2-aminophenyl) exhibited the highest docking score (8.2495 kcal M−1), the hydrogen of the amino bridge and the hydrogen of the of amino group at the ortho position (C2) of the phenyl moiety were exhibiting hydrogen bond interaction with Gly216 residue at a distance of 1.907 and 2.003 Å, respectively. The oxygen of 1,3,4-oxadiazole was involved in hydrogen bond interaction with Gly216 residue at a distance of 1.885 Å. Compound 4j (methyl) exhibited the lowest docking score (5.7482 kcal M−1).
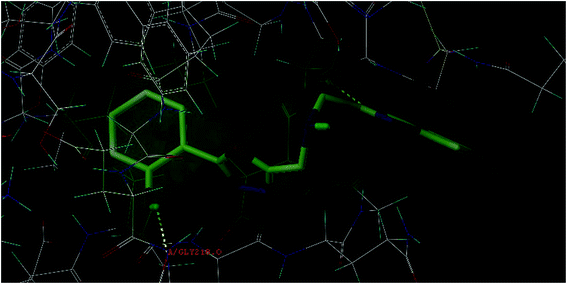 |
| Fig. 1 Docking confirmation of compound 4f against factor Xa. | |
The docking study of the designed 2,5-disubstituted 1,3,4-oxadizoles onto the active site of the enzyme VKOR (3KP9) were considerable. The co-crystallized ligand, U-10 in the a chain of the protein exhibited a docking score and crash score of 11.0519 and −4.2664 kcal M−1 respectively, with the main hydrophobic interactions with the surrounding residues Arg63, Cys133, Phe114, Ala73, Gly76 and Thr170, strongly contributed to the stabilization. The docking study revealed that the 1,3,4-oxadiazole derivatives possessed substantial affinity towards 3KP9 (Fig. 2). The 1,3,4-oxadiazoles 4a–4k exhibited docking score in the range of 5.7315 to 7.5942 kcal M−1. Compound 4f (2-hydroxyphenyl) exhibited the highest docking score (7.5942 kcal M−1) and compound 4i (H) exhibited the lowest docking score (5.7315 kcal M−1). None of the designed 1,3,4-oxadiazoles exhibited crash score of greater than −4.5 kcal M−1. A crash score greater than −4.5 kcal M−1, highlights inappropriate penetration of the ligand into the binding site of 1NFY and 3KP9. The docking score of 1,3,4-oxadizoles; 4a–4k along with their crash score and polar score are presented in Table 2.
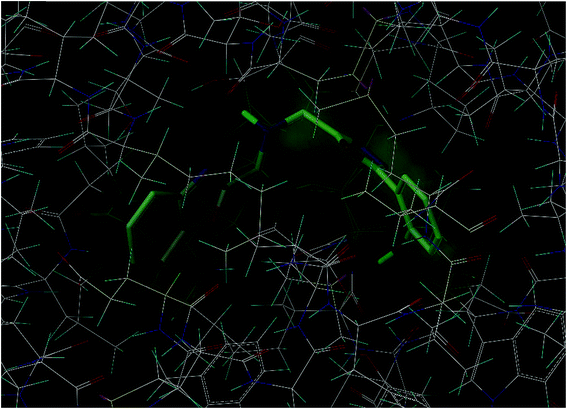 |
| Fig. 2 Docking confirmation of compound 4f against VKOR. | |
Table 2 Docking score of 1,3,4-oxadiazole derivatives 4a–4k
Sl No. |
Compound |
Docking score towards 1NFYa |
Docking score towards 3KP9a |
Total score |
Crash score |
Polar score |
Total score |
Crash score |
Polar score |
Data expressed in kcal M−1 as obtained from the docking utility of SYBYL 2.1 software. |
1 |
4a |
7.8420 |
−0.9808 |
1.2296 |
6.3371 |
−0.9439 |
0.0000 |
2 |
4b |
6.7809 |
−1.3056 |
1.9362 |
7.3146 |
−0.5267 |
0.0002 |
3 |
4c |
7.6432 |
−0.4919 |
3.394 |
7.2228 |
−1.2568 |
0.0015 |
4 |
4d |
8.2495 |
−1.12 |
2.8877 |
7.4090 |
−0.8013 |
0.0225 |
5 |
4e |
6.8824 |
−0.8197 |
0.9712 |
7.1937 |
−0.6164 |
1.0696 |
6 |
4f |
7.1389 |
−0.2259 |
1.3741 |
7.5942 |
−0.7832 |
0.5217 |
7 |
4g |
7.6132 |
−1.024 |
2.3548 |
6.9707 |
−1.0720 |
0.9974 |
8 |
4h |
6.9597 |
−1.0125 |
1.1459 |
6.6382 |
−0.4538 |
0.0000 |
9 |
4i |
5.7623 |
−1.0396 |
1.8386 |
5.7315 |
−1.0953 |
1.0290 |
10 |
4j |
5.7482 |
−0.2411 |
2.0757 |
6.8402 |
−1.4975 |
0.6091 |
11 |
4k |
6.1310 |
−1.0475 |
1.6415 |
6.1449 |
−0.6815 |
1.1785 |
12 |
RPR132747 |
6.2033 |
−2.2025 |
0.8011 |
— |
13 |
U-10 |
— |
11.0519 |
−4.2664 |
0.0003 |
The molecular docking studies indicated that the designed 1,3,4-oxadiazole have better affinity to inhibit factor Xa than VKOR, with comparative higher docking score and lower crash score. This relative selectivity towards factor Xa is advantages, since clinical studies highlight that in comparison to VKOR inhibitors like warfarin, factor Xa inhibitors like rivaroxaban have marked advantage, as factor Xa inhibitors significantly reduced the primary composite endpoint of stroke and non-CNS systemic embolism, and the major secondary endpoints like myocardial infarction and vascular death are also reduced considerably by the factor Xa inhibitors. In addition, the other clinical drawback like requirement to monitor the PT values and TDM are minimal with respect to selective and direct factor Xa inhibitors.
2.4. In vitro radical scavenging evaluation
The 1,3,4-oxadiazole derivatives; 4a–4k were evaluated for their radical scavenging property at 25 μM strength by adopting DPPH radical assay method and employing ascorbic acid as the reference standard. The results of the radical scavenging activity were significant and the compounds 4a–4k exhibited ranging from 29.5 to 80.8% in comparison ascorbic acid with a radical scavenging efficacy of 71.7%. The results of the radical scavenging evaluation indicated that the compounds with electron donating substitution; at the ortho/para position of the phenyl moiety exhibited better radical scavenging activity than that of the reference standard, which may be attributed to the resonance stabilized intermediate formation. The statistical analysis of the compounds 4a–4k for their radical scavenging property indicated that the tested compounds exhibited significance value of P < 0.001 highlighting the confidence interval of 99.9% with respect to reference standard ascorbic acid. The radical scavenging data of the test compounds 4a–4k at 25 μM strength are expressed as the mean ± SEM in Table 3.
Table 3 The radical scavenging efficacy of the compounds 4a–4k
Sl No. |
Compounds |
Percentage free radical scavenging activitya,b |
Trial 1 |
Trial 2 |
Trial 3 |
Mean ± SEM |
Results are expressed as the mean values from three parallel experiments ± SEM. Data was analyzed by Dunnett's test compared with reference drug ascorbic acid. n = 3; (***) equals P < 0.001. |
1 |
4a |
48.18 |
49.20 |
47.25 |
48.21 ± 0.56*** |
2 |
4b |
29.43 |
30.83 |
28.17 |
29.48 ± 0.77*** |
3 |
4c |
33.08 |
34.40 |
31.88 |
33.12 ± 0.73*** |
4 |
4d |
72.20 |
72.75 |
71.70 |
72.22 ± 0.30 |
5 |
4e |
80.75 |
81.13 |
80.41 |
80.76 ± 0.21*** |
6 |
4f |
71.32 |
71.89 |
70.81 |
71.34 ± 0.31 |
7 |
4g |
76.23 |
76.70 |
75.80 |
76.24 ± 0.26*** |
8 |
4h |
55.22 |
56.10 |
54.42 |
55.25 ± 0.49*** |
9 |
4i |
39.87 |
41.06 |
38.80 |
39.91 ± 0.65*** |
10 |
4j |
36.98 |
38.22 |
35.85 |
37.02 ± 0.68*** |
11 |
4k |
38.11 |
39.33 |
37.00 |
38.15 ± 0.67*** |
12 |
Ascorbic acid |
72.45 |
70.94 |
71.70 |
71.70 ± 0.44 |
2.5. Ex vivo anticoagulant activity
The literature studies highlight the antithrombotic importance of 1,3,4-oxadiazole and reports also discussed the structural requirement.13,14 The 1,3,4-oxadiazole derivatives 4a–4k at a cumulative dose of 25 mg kg−1 were evaluated for an increase in CT, PT and aPTT values towards blood coagulation activity. Acenocoumarol at a cumulative dose of 1 mg kg−1 was used as the reference standard for PT and unfractionated heparin at a single dose of 500 IU kg−1 was used as the reference standard for aPTT and CMC was employed as the negative control. The test compounds exhibited substantial anticoagulant activity with respect to increasing in CT, PT, and aPTT measure values. The statistical analysis of the compounds anticoagulant efficacy indicated that the compounds exhibited a significance value of P < 0.001 highlighting the confidence interval of 99.9% with respect to increasing in CT, PT, and aPPT values, respectively with respect to the negative control.
2.6. Measure for an increase in prothrombin time (PT)
The anticoagulant activity for the increase in PT by the test compounds 4a–4k highlighted that the compounds exhibited significant increase in PT values ranging from 14.73 to 30.83 s with respect to the negative control (12.23 ± 0.12 s) and in comparison to that of the reference standard acenocoumarol (30.03 ± 0.67 s). The increase in PT measure value highlights the effect of the test compounds on the exogenous pathway of coagulation. Compound 4k; the mercapto derivative of 1,3,4-oxadiazoles exhibited a significant increase in PT (30.83 ± 0.15 s) equivalent to that of the reference standard acenocoumarol and was found to be the most potent among the tested 1,3,4-oxadiazole series. The significant increase in the prothrombin time of compound 4k may be attributed to the possible tautomeric shift from thiol to thione isomer, resulting in the inhibition of VKOR enzyme and exhibiting an anticoagulant effect. Followed by the mercapto derivative, the nitro derivatives were exhibiting a significant increase in PT measure values, compound 4b (4-nitrophenyl) and 4c (3,5-dinitrophenyl) exhibited a PT measure value of 27.67 ± 0.27 and 26.67 ± 0.23 s, respectively. The anticoagulant activity data highlights that oxadiazole compounds with aryl substitution at position C5; 4a–4h have better efficacy than that of the compounds; 4i and 4j indicating the necessitate for bulky substitution at C5. Moreover, phenyl substitution at C5 of oxadiazole with electron withdrawing function; 4b and 4c have considerable anticoagulant property in comparison to other aryl substituted derivatives; 4a and 4d–4h. The increase in PT values of the test compounds with respect to the negative control and the reference standard at the end of 12 hours after the administration of the last dose were statistically analyzed using the repeated measures ANOVA with Dunnett's test and the data are presented in Table 4.
Table 4 Ex vivo anticoagulant activity data of the compounds 4a–4k
Sl No. |
Compounds |
Mean PT values (s) ± SEMa |
Mean CT values (s) ± SEMa |
Mean aPPT values (s) ± SEMa |
Data was analyzed by Dunnett's test compared with the negative control. n = 6; (***) equals P < 0.001. |
1 |
Control |
12.03 ± 0.12 |
9.80 ± 0.06 |
27.87 ± 0.12 |
2 |
4a |
21.10 ± 0.25*** |
14.33 ± 0.19*** |
32.13 ± 0.15*** |
3 |
4b |
27.67 ± 0.27*** |
17.70 ± 0.21*** |
31.67 ± 0.29*** |
4 |
4c |
26.67 ± 0.23*** |
16.47 ± 0.09*** |
32.37 ± 0.26*** |
5 |
4d |
24.03 ± 0.28*** |
16.17 ± 0.22*** |
32.70 ± 0.06*** |
6 |
4e |
23.07 ± 0.54*** |
15.73 ± 0.45*** |
32.53 ± 0.48*** |
7 |
4f |
23.20 ± 0.44*** |
16.63 ± 0.35*** |
33.20 ± 0.21*** |
8 |
4g |
20.50 ± 0.12*** |
14.07 ± 0.18*** |
32.33 ± 0.32*** |
9 |
4h |
21.70 ± 0.21*** |
15.97 ± 0.18*** |
31.83 ± 0.35*** |
10 |
4i |
14.73 ± 0.09*** |
13.00 ± 0.06*** |
32.57 ± 0.19*** |
11 |
4j |
15.03 ± 0.15*** |
14.17 ± 0.12*** |
33.07 ± 0.09*** |
12 |
4k |
30.83 ± 0.15*** |
23.57 ± 0.19*** |
33.00 ± 0.15*** |
13 |
Acenocoumarol |
30.03 ± 0.67*** |
22.53 ± 0.50*** |
|
14 |
Unfractionated heparin |
|
35.43 ± 0.20*** |
72.63 ± 0.56*** |
2.7. Measure for an increase in clotting time (CT)
The anticoagulant activity for the increase in CT values of the test compounds 4a–4k highlighted that the compounds exhibited significant increase in CT measure values as that of negative control (9.80 ± 0.06 s), in comparison to reference standards acenocoumarol (22.53 ± 0.50 s) and unfractionated heparin (35.43 ± 0.20 s) employed. The measure for increase in CT highlights the cumulative role of the compounds acting via both; exogenous and endogenous pathway of coagulation. Hence, both acenocoumarol and unfractionated heparin were employed as reference standard. The test compounds 4a–4k exhibited a CT measure values in the range from 13.00 to 23.57 s. The mercapto derivative 4k exhibited a significant increase in CT valves (23.57 ± 0.19 s) highest among the tested 1,3,4-oxadiazoles, similar to that of PT measure values, higher than that of the reference standard employed. The increase in CT values of the test compounds at the end of 12 hours after the administration of the last dose were compared with the negative control and the reference standards acenocoumarol and unfractionated heparin, the data were statistically analyzed using the repeated measures ANOVA with Dunnett's test and the data are presented in Table 4.
2.8. Measure for an increase in activated partial thromboplastin time (aPTT)
The anticoagulant activity for the increase in aPPT values of the test compounds 4a–4k highlighted that the compounds produced a minimal increase in aPPT measure values ranging from 31.67 to 33.20 s, in comparison to reference standard unfractionated heparin (73.08 ± 0.86 s) and as that of negative control (27.27 ± 0.55 s). The measure for increase in aPPT highlights the effect of compounds on the endogenous pathway of coagulation. The results were not substantial, as the mercapto derivative 4k, which exhibited a significant increase in PT (30.83 ± 0.15 s) and CT valves (23.57 ± 0.19 s) failed to produce a significant increase in aPPT measure values (33.00 ± 0.15 s). The aPPT measure values of the test compounds at the end of 12 hours after the administration of the last dose were compared with the negative control and the reference standard unfractionated heparin. The data were statistically analyzed using the repeated measures ANOVA with Dunnett's test and the data are presented in Table 4.
3. Experimental section
3.1. In silico ADME studies
Most of drug candidates fail in clinical trials due to poor ADME properties. Screening for drug likeliness and for ADME properties of a molecule are important for drug development. Since, oral bioavailability is considered to play an important role for the development of bioactive molecules as therapeutic agents, which is essential for rational drug design. According to Lipinski rule of five, molecules that breach more than one of these rules may have difficulties with bioavailability. The Lipinski rule of five establishes some structural parameters in relevance to the oral bioavailability profile of the molecules and is extensively adapted in designing compounds.15 The in silico studies were performed for Lipinski parameters, as well as for the topological polar surface area (TPSA), the percentage of absorption (% ABS) and the drug-likeness and drug score were included for the designed 1,3,4-oxadiazoles in order to verify that these compounds exhibit a optimal theoretical oral bioavailability potential. The drug-likeness and drug score were calculated using the OSIRIS property explorer software and the lipophilicity and TPSA were calculated using Molinspiration online property calculation toolkit (http://www.molinspiration.com). The percent of absorption (% ABS) was estimated according to the eqn (1).
Calculation of percent of absorption
|
% ABS = 109 − (0.345 × TPSA)
| (1) |
TPSA was also calculated using Molinspiration online property calculation toolkit according to the fragment-based method reported. Polar surface area, together with lipophilicity is an important property of a molecule which indicates the ease of transport across biological membranes. A high TPSA values indicates poor absorption of a drug and in turn highlights low bioavailability.
3.2. Molecular docking studies
Docking studies were undertaken using the SYBYL-X 2.1 docking program in order to gain insight into the binding mode of the designed compounds and elucidate the impact of structural differences on the selected protein towards their inhibitory activities. Crystallographic data of the selected enzyme with its co-crystallized ligand were downloaded from Brookhaven Protein Data Bank (www.rcsb.org) and were used for docking studies. The X-ray crystal structure of protein enzymes were defined and established with both hydrophobic active site and hydrogen bond interaction amino acid residuals. The protein was prepared using a protein preparation module in SYBYL-X 2.1, where bond orders were assigned, water and other residues were removed, hydrogen atoms were added and the protein model was charged with molecular mechanics force fields (MMFF94s). Ligands, 1,3,4-oxadiazoles were drawn in Chemsketch and molecules were converted into 3D structures using the ligand preparation utility of SYBYL-X 2.1 and the ligands energy were minimized by applying MMFF94s in SYBYL-X 2.1.
Molecular docking simulations were performed in order to distinguish the basic receptor–ligand interactions. The optimized 3D-structures of 1,3,4-oxadiazoles were docked within 10 Å radius to find the most optimal binding pose of each ligand. The docking algorithm performs a series of hierarchical searches for locations of possible ligand affinity within the binding site of the enzyme. The docked poses of geometrically optimized ligands were ranked with total scores, highlighting the affinity to the enzyme core and the most optimal binding pose of each ligand were analyzed in order to untangle the essential parameters in terms of direct- (hydrogen bonds) and indirect (hydrophobic) interactions governing binding disparities among the series of the compounds. The score of the docked molecules were obtained, which indicate the affinity of the molecules towards the enzyme core. To envisage the anticoagulant property of the molecules, VKOR and factor Xa enzymes were selected as the target.16 The compounds were docked along with the co-crystallized ligands and the interactions like hydrogen bonding were visualized. The protein structure of 1NFY and 3KP9 is well established with a hydrophobic active site and the proteins were determined at 2.1 and 3.6 Å resolution, respectively. The bound conformations of 4-({4-[(6-chloro-1-benzothien-2-yl)sulfonyl]-2-oxopiperazine-1-yl}methyl)benzenecarboximidamide (RPR132747) and Ubiquinone-10 (U-10) were used as controls in order to define the active site in FXa and VKOR, respectively.
3.3. Synthesis of designed molecules
Melting points were determined in open glass capillaries and are uncorrected. TLC was used to assess the progress/completion of reactions and the purity of the synthesized compounds using ethyl acetate and hexane (4
:
1) as the solvent system and iodine vapors as a visualizing agent. The IR spectra were recorded using Shimadzu FTIR-8400 instrument by KBr disc pellet technique and only noteworthy absorption levels (cm−1) are listed. 1H NMR and 13C NMR spectra were recorded using Brucker AC-400 MHz FT NMR spectrophotometer at 400 MHz with deuterated dimethyl sulfoxide (DMSO-d6) as solvent and tetra methyl silane (TMS) as internal standard (chemical shifts in δ, ppm). The splitting patterns were designated as follows: s: singlet; d: doublet; q: quartet; m: multiplet. The LCMS was recorded using Shimadzu LCMS-2010A instrument by ESI. The elementary analysis was recorded using Thermo Finnigan FLASH EA 1112 CHNS analyzer and values found are within 0.4% of theoretical values.
3.3.1. Synthesis of (benzo[d]oxazol-2-yl)methanamine (1). A solution of 2-aminophenol (13 g, 0.12 mol) and glycine (18 g, 0.24 mol) in xylene (50 mL) was heated to reflux with stirring for 2 h. The progress of the reaction was monitored by TLC. On completion of the reaction, the mixture was cooled to room temperature and the pH was adjusted to 8 using 1 N sodium hydroxide solution to obtain off-white colored product 2. The product was recrystallized using rectified spirit as the solvent. Yield: 78%. Mp: 176–177 °C. Rf: 0.68 (ethyl acetate and hexane; 4
:
1). IR (KBr, cm−1) 3369 (NH2), 3047 (
C–H), 1593 (C
N). 1H NMR (400 MHz, DMSO-d6, δ, ppm): 7.4–6.9 (m, 4H, Ar-H), 3.6 (d, J = 12.1 Hz, 2H, CH2), 2.4 (s, 2H, NH2).
3.3.2. Synthesis of ethyl 2-[(benzo[d]oxazol-2-yl)methylamino]acetate (2). The mixture of benzoxazole-2-methanamine derivative 2 (14.8 g, 0.10 mol), ethyl 2-chloroacetate (18.3 mL, 0.15 mol) and activated anhydrous potassium carbonate (12.0 g) in dichloromethane (50.0 mL) were heated to reflux with stirring for 6 h. The progress of the reaction was monitored by TLC. On completion of the reaction, the mixture was cooled to room temperature and filtered. The filtrate was concentrated and poured into the crushed ice to obtain the solid ester product 3. The product was recrystallized using rectified spirit as the solvent. Yield: 72%. Mp: 121–123 °C. Rf: 0.71 (ethyl acetate and hexane; 4
:
1). IR (KBr, cm−1) 3384 (NH), 3030 (C
C), 1737 (C
O), 1633 (C
N). 1H NMR (400 MHz, DMSO-d6, δ, ppm): 7.6–7.2 (m, 4H, Ar-H), 4.3 (q, J = 6.4 Hz, 2H,
), 3.8 (d, J = 11.3 Hz, 2H,
), 3.4 (d, J = 16.6 Hz, 2H,
), 2.6 (s, 1H, CH2![[N with combining low line]](https://www.rsc.org/images/entities/char_004e_0332.gif)
), 1.1 (t, J = 14.8 Hz, 3H, CH3).
3.3.3. Synthesis of 2-[(benzo[d]oxazol-2-yl)methylamino]acetohydrazide (3). The solution of ester derivative 3 (18.6 g, 0.08 mol) and hydrazine monohydrate (10.0 mL, 0.20 mol) in ethanol (30 mL) were heated to reflux for 8 h. The progress of the reaction was monitored by TLC. On completion of the reaction, the mixture was cooled to room temperature and allowed to cool in refrigerator overnight to precipitate the acylhydrazide 4 the product was recrystallized using rectified spirit as a solvent. Yield: 56%. Mp: 212–213 °C. Rf: 0.74 (ethyl acetate and hexane; 4
:
1). IR (KBr, cm−1) 3398–3450 (NH2, NH), 3045 (C
C), 1718 (C
O), 1627 (C
N), 1432 (C–O–C). 1H NMR (400 MHz, DMSO-d6, δ, ppm): 11.7 (s, 1H, CO![[N with combining low line]](https://www.rsc.org/images/entities/char_004e_0332.gif)
), 7.3–6.8 (m, 4H), 4.8 (s, 2H,
), 3.7 (d, J = 7.2 Hz, 2H,
), 3.6 (d, J = 9.7 Hz, 2H,
), 2.6 (s, 1H, CH2![[N with combining low line]](https://www.rsc.org/images/entities/char_004e_0332.gif)
).
3.3.4. General procedure for the synthesis of N-[(benzo[d]oxazol-2-yl)methyl](5-substituted-1,3,4-oxadiazol-2-yl)methanamine (4a–4k). To the solution of acetohydrazide 4 (2.2 g, 0.01 mol) and carboxylic acid derivatives (0.01 mol) in ethanol (25.0 mL), phosphorous oxychloride (1.6 mL, 0.01 mol) was added slowly in an exhaustion chamber and heated to reflux for 8–12 h. The progress of the reaction was monitored by TLC. On completion of the reaction, the mixture was cooled to room temperature and crushed ice was added to obtain oxadiazole derivative (4a–4j). The product was recrystallized using rectified spirit as a solvent.
3.3.4.1. N-[(Benzo[d]oxazol-2-yl)methyl](5-phenyl-1,3,4-oxadiazol-2-yl)methanamine (4a). Yield: 76%. Mp: 233–234 °C. Rf: 0.75 (ethyl acetate and hexane; 4
:
1). IR (KBr, cm−1) 3242 (NH), 3026 (C
C), 1592 (C
N), 1424 (C–O–C). 1H NMR (400 MHz, DMSO-d6, δ, ppm): 7.3–6.9 (m, 9H, Ar-H), 3.8 (d, J = 12.1 Hz, 2H,
), 3.6 (d, J = 14.6 Hz, 2H,
), 2.3 (s, 1H, CH2![[N with combining low line]](https://www.rsc.org/images/entities/char_004e_0332.gif)
). 13C NMR (100 MHz, DMSO-d6, δ, ppm): 167.2 (C5 of oxadiazole), 163.5 (C2 of oxadiazole), 159.5–119.7 (aromatic carbons), 49.7 (oxadiazole-
H2NHCH2), 46.3 (benzoxazole-
H2NHCH2). MS (m/z, %): 307 (60) [M+1]. Anal. calcd for C17H14N4O2: C, 66.66; H, 4.61; N, 18.29. Found: C, 66.74; H, 4.72; N, 18.31.
3.3.4.2. N-[(Benzo[d]oxazol-2-yl)methyl][5-(2-nitrophenyl)-1,3,4-oxadiazol-2-yl]methanamine (4b). Yield: 72%. Mp: 226–227 °C. Rf: 0.69 (ethyl acetate and hexane; 4
:
1). IR (KBr, cm−1) 3256 (NH), 3021 (C
C), 1643 (C
N), 1542 (N–O, NO2), 1431 (C–O–C). 1H NMR (400 MHz, DMSO-d6, δ, ppm): 7.7–7.4 (m, 8H, Ar-H), 3.8 (d, J = 14.1 Hz, 2H,
), 3.6 (d, J = 12.8 Hz, 2H,
), 2.4 (s, 1H, CH2![[N with combining low line]](https://www.rsc.org/images/entities/char_004e_0332.gif)
). 13C NMR (100 MHz, DMSO-d6, δ, ppm): 168.3 (C5 of oxadiazole), 164.5 (C2 of oxadiazole), 161.3–121.6 (aromatic carbons), 49.2 (oxadiazole-
H2NHCH2), 44.3 (benzoxazole-
H2NHCH2). MS (m/z, %): 352 (75) [M+1]. Anal. calcd for C17H13N5O4: C, 58.12; H, 3.73; N, 19.93. Found: C, 58.46; H, 3.79; N, 20.13.
3.3.4.3. N-[(Benzo[d]oxazol-2-yl)methyl[[5-(3,5-dinitrophenyl)-1,3,4-oxadiazol-2-yl]methanamine (4c). Yield: 68%. Mp: 293–295 °C. Rf: 0.74 (ethyl acetate and hexane; 4
:
1). IR (KBr, cm−1) 3253 (NH), 3019 (C
C), 1607 (C
N), 1519, 1522 (NO2), 1436 (C–O–C). 1H NMR (400 MHz, DMSO-d6, δ, ppm): 8.4–7.6 (m, 7H, Ar-H), 3.6 (d, J = 11.4 Hz, 2H,
), 3.4 (d, J = 09.1 Hz, 2H,
), 2.4 (s, 1H, CH2![[N with combining low line]](https://www.rsc.org/images/entities/char_004e_0332.gif)
). 13C NMR (100 MHz, DMSO-d6, δ, ppm): 164.5 (C5 of oxadiazole), 160.7 (C2 of oxadiazole), 158.6–116.7 (aromatic carbons), 45.1 (oxadiazole-
H2NHCH2), 42.3 (benzoxazole-
H2NHCH2). MS (m/z, %): 397 (80) [M+1]. Anal. calcd for C17H12N6O6: C, 51.52; H, 3.05; N, 21.21. Found: C, 51.61; H, 3.39; N, 21.46.
3.3.4.4. N-[(Benzo[d]oxazol-2-yl)methyl][5-(2-aminophenyl)-1,3,4-oxadiazol-2-yl]methanamine (4d). Yield: 57%. Mp: 231–233 °C. Rf: 0.62 (ethyl acetate and hexane; 4
:
1). IR (KBr, cm−1) 3324 (NH2), 3249 (NH), 3021 (C
C), 1612 (C
N), 1266 (C–O–C). 1H NMR (400 MHz, DMSO-d6, δ, ppm): 8.9–8.3 (m, 8H, Ar-H), 5.4 (s, 2H,
), 3.7 (d, J = 12.6 Hz, 2H,
), 3.6 (d, J = 9.4 Hz, 2H,
), 2.4 (s, 1H, CH2![[N with combining low line]](https://www.rsc.org/images/entities/char_004e_0332.gif)
). 13C NMR (100 MHz, DMSO-d6, δ, ppm): 164.2 (C5 of oxadiazole), 162.1 (C2 of oxadiazole), 161.9–119.3 (aromatic carbons), 46.9 (oxadiazole-
H2NHCH2), 42.3 (benzoxazole-
H2NHCH2). MS (m/z, %): 322 (45) [M+1]. Anal. calcd for C17H16N6O: C, 63.54; H, 4.71; N, 21.79. Found: C, 63.98; H, 4.76; N, 21.87.
3.3.4.5. N-[(Benzo[d]oxazol-2-yl)methyl][5-(4-aminophenyl)-1,3,4-oxadiazol-2-yl]methanamine (4e). Yield: 68%. Mp: 257–259 °C. Rf: 0.59 (ethyl acetate and hexane; 4
:
1). IR (KBr, cm−1) 3318 (NH2), 3251 (NH), 3024 (C
C), 1589 (C
N), 1276 (C–O–C). 1H NMR (400 MHz, DMSO-d6, δ, ppm): 8.2–7.8 (m, 8H, Ar-H), 3.7 (d, J = 19.2 Hz, 2H,
), 3.6 (d, J = 14.8 Hz, 2H,
), 2.5 (s, 1H, CH2![[N with combining low line]](https://www.rsc.org/images/entities/char_004e_0332.gif)
). 13C NMR (100 MHz, DMSO-d6, δ, ppm): 167.5 (C5 of oxadiazole), 164.1 (C2 of oxadiazole), 159.3–112.7 (aromatic carbons), 43.2 (oxadiazole-
H2NHCH2), 41.6 (benzoxazole-
H2NHCH2). MS (m/z, %): 321 (35) [M+]. Anal. calcd for C17H15N5O2: C, 63.54; H, 4.71; N, 21.79. Found: C, 63.46; H, 4.89; N, 22.12.
3.3.4.6. N-[(Benzo[d]oxazol-2-yl)methyl][5-(2-hydroxyphenyl)-1,3,4-oxadiazol-2-yl]methanamine (4f). Yield: 57%. Mp: 234–235 °C. Rf: 0.60 (ethyl acetate and hexane; 4
:
1). IR (KBr, cm−1) 3343 (O–H), 3239 (NH), 3027 (C
C), 1611 (C
N), 1409 (C–O), 1272 (C–O–C). 1H NMR (400 MHz, DMSO-d6, δ, ppm): 10.2 (s, 1H, OH), 7.9–7.6 (m, 8H, Ar-H), 3.7 (d, J = 11.9 Hz, 2H,
), 3.4 (d, J = 7.4 Hz, 2H,
), 2.8 (s, 1H, CH2![[N with combining low line]](https://www.rsc.org/images/entities/char_004e_0332.gif)
). 13C NMR (100 MHz, DMSO-d6, δ, ppm): 166.1 (C5 of oxadiazole), 164.6 (C2 of oxadiazole), 161.3–121.2 (aromatic carbons), 46.5 (oxadiazole-
H2NHCH2), 43.2 (benzoxazole-
H2NHCH2). MS (m/z, %): 323 (40) [M+1]. Anal. calcd for C17H14N4O3: C, 63.35; H, 4.38; N, 17.38. Found: C, 63.31; H, 4.35; N, 17.26.
3.3.4.7. N-[(Benzo[d]oxazol-2-yl)methyl][5-(4-hydroxyphenyl)-1,3,4-oxadiazol-2-yl]methanamine (4g). Yield: 61%. Mp: 217–218 °C. Rf: 0.55 (ethyl acetate and hexane; 4
:
1). IR (KBr, cm−1) 3326 (O–H), 3241 (NH), 3027 (C
C), 1593 (C
N), 1411 (C–O), 1278 (C–O–C). 1H NMR (400 MHz, DMSO-d6, δ, ppm): 9.8 (s, 1H, OH), 8.4–7.5 (m, 8H, Ar-H), 3.7 (d, J = 7.2 Hz, 2H,
), 3.6 (d, J = 11.5 Hz, 2H,
), 2.5 (s, 1H, CH2![[N with combining low line]](https://www.rsc.org/images/entities/char_004e_0332.gif)
). 13C NMR (100 MHz, DMSO-d6, δ, ppm): 164.5 (C5 of oxadiazole), 162.8 (C2 of oxadiazole), 159.1–117.9 (aromatic carbons), 43.2 (oxadiazole-
H2NHCH2), 41.6 (benzoxazole-
H2NHCH2). MS (m/z, %): 322 (30) [M+]. Anal. calcd for C17H14N4O3: C, 63.35; H, 4.38; N, 17.38. Found: C, 63.18; H, 4.22; N, 17.62.
3.3.4.8. N-[(Benzo[d]oxazol-2-yl)methyl][5-(pyridin-3-yl)-1,3,4-oxadiazol-2-yl]methanamine (4h). Yield: 68%. Mp: 267–269 °C. Rf: 0.74 (ethyl acetate and hexane; 4
:
1). IR (KBr, cm−1) 3241 (NH), 3025 (C
C), 1624 (C
N), 1455 (C–O–C). 1H NMR (400 MHz, DMSO-d6, δ, ppm): 8.3–7.7 (m, 8H, Ar-H), 3.7 (d, J = 11.4 Hz, 2H,
), 3.5 (d, J = 12.2 Hz, 2H,
), 2.4 (s, 1H, CH2![[N with combining low line]](https://www.rsc.org/images/entities/char_004e_0332.gif)
). 13C NMR (100 MHz, DMSO-d6, δ, ppm): 164.4 (C5 of oxadiazole), 163.1 (C2 of oxadiazole), 161.6–117.3 (aromatic carbons), 46.9 (oxadiazole-
H2NHCH2), 44.1 (benzoxazole-
H2NHCH2). MS (m/z, %): 308 (38) [M+1]. Anal. calcd for C16H13N5O2: C, 62.53; H, 4.26; N, 22.79. Found: C, 62.72; H, 4.56; N, 23.11.
3.3.4.9. N-[(Benzo[d]oxazol-2-yl)methyl](1,3,4-oxadiazol-2-yl)methanamine (4i). Yield: 82%. Mp: 241–242 °C. Rf: 0.64 (ethyl acetate and hexane; 4
:
1). IR (KBr, cm−1) 3248 (NH), 3028 (C
C), 1581 (C
N), 1274 (C–O–C). 1H NMR (400 MHz, DMSO-d6, δ, ppm): 8.3 (s, 1H, ![[C with combining low line]](https://www.rsc.org/images/entities/char_0043_0332.gif)
oxadiazole), 7.9–7.6 (m, 4H, Ar-H), 3.7 (d, J = 12.6 Hz, 2H,
), 3.5 (d, J = 10.6 Hz, 2H,
), 2.3 (s, 1H, CH2![[N with combining low line]](https://www.rsc.org/images/entities/char_004e_0332.gif)
). 13C NMR (100 MHz, DMSO-d6, δ, ppm): 167.2 (C5 of oxadiazole), 163.5 (C2 of oxadiazole), 159.1–120.7 (aromatic carbons), 47.6 (oxadiazole-
H2NHCH2), 42.6 (benzoxazole-
H2NHCH2). MS (m/z, %): 230 (32) [M+]. Anal. calcd for C11H10N4O2: C, 57.39; H, 4.38; N, 24.34. Found: C, 57.58; H, 4.61; N, 24.47.
3.3.4.10. N-[(Benzo[d]oxazol-2-yl)methyl](5-methyl-1,3,4-oxadiazol-2-yl)methanamine (4j). Yield: 84%. Mp: 256–257 °C. Rf: 0.68 (ethyl acetate and hexane; 4
:
1). IR (KBr, cm−1) 3245 (NH), 3023 (C
C), 1597 (C
N), 1478 (C–O–C). 1H NMR (400 MHz, DMSO-d6, δ, ppm): 7.9–7.4 (m, 4H, Ar-H), 3.8 (d, J = 12.5 Hz, 2H,
), 3.6 (d, J = 09.1 Hz, 2H,
), 2.4 (s, 1H, CH2![[N with combining low line]](https://www.rsc.org/images/entities/char_004e_0332.gif)
), 1.9 (s, 3H, CH3). 13C NMR (100 MHz, DMSO-d6, δ, ppm): 169.8 (C2 of oxadiazole), 162.4 (C5 of oxadiazole), 154.1–121.2 (aromatic carbons), 47.8 (oxadiazole-
H2NHCH2), 43.6 (benzoxazole-
H2NHCH2), 27.6 (CH3 of 5-methyloxadiazole). MS (m/z, %): 244 (45) [M+]. Anal. calcd for C12H12N4O2: C, 59.01; H, 4.95; N, 22.94. Found: C, 58.92; H, 4.66; N, 22.96.
3.3.4.11. Synthesis of 5-{[(benzo[d]oxazol-2-yl)methylamino]methyl}-1,3,4-oxadiazole-2-thiol (4k). To a solution of the acylhydrazide 4 (2.2 g, 0.01 mol) in ethanol (30.0 mL), equimolar quantities of carbon disulphide (3.8 mL, 0.05 mol) and potassium hydroxide (2.8 g, 0.05 mol) were added. The contents were heated to reflux for 4 h. The progress of the reaction was monitored by TLC. On completion of the reaction, distilled water was added followed by neutralization with dilute HCl to obtain the solid mercapto product 4k. The product separated out was filtered and recrystallized using rectified spirit as a solvent. Yield: 58%. Mp: 287–288 °C. Rf: 0.53 (ethyl acetate and hexane; 4
:
1). IR (KBr, cm−1) 3342 (NH), 3087 (C
C), 2587 (SH), 1620 (C
N), 1283 (C–O–C). 1H NMR (400 MHz, DMSO-d6, δ, ppm): 12.9 (s, 1H, SH), 8.1–7.6 (m, 4H, Ar-H), 3.9 (d, J = 14.7 Hz, 2H,
), 3.4 (d, J = 10.8 Hz, 2H,
), 2.3 (s, 1H, CH2![[N with combining low line]](https://www.rsc.org/images/entities/char_004e_0332.gif)
). 13C NMR (100 MHz, DMSO-d6, δ, ppm): 174.7 (C-SH, C2 of oxadiazole), 166.2 (C5 of oxadiazole), 156.9–121.4 (aromatic carbons), 49.3 (oxadiazole-
H2NHCH2), 45.2 (benzoxazole-
H2NHCH2). MS (m/z, %): 263 (45) [M+1]. Anal. calcd for C11H10N4O2S: C, 50.37; H, 3.84; N, 21.36; S, 12.23. Found: C, 50.62; H, 4.11; N, 21.53; S, 12.49.
3.4. In vitro radical scavenging activity
The in vitro radical scavenging activity of the newly synthesized 1,3,4-oxadiazoles were screened by DPPH radical scavenging assay method.17 To the 2 mL solutions of synthesized compounds (25 μM), 2 mL DPPH solution (25 μM) was added into the test tube. The solution was incubated at 37 °C for 30 min and the absorbance of each solution was measured at 517 nm against the reagent blank solution. The ascorbic acid (25 μM) was used as the reference standard. The experimental values obtained for DPPH radical scavenging assays were statistically analyzed. The percent free radical scavenging activity was calculated according to the eqn (2).
Calculation of percent of radical scavenging activity
|
 | (2) |
3.5. Ex vivo blood coagulation test
The anticoagulant activity of the newly synthesized 1,3,4-oxadiazoles was evaluated by blood coagulation test method18,19 using groups of Albino rats of Wistar strain of both sex, weighing 120–140 g each and 6 animals per group. The first group served as the untreated control and was given 0.5% CMC orally. The second groups were designated as the positive control for an increase in PT measure value and received a cumulative dose of 1 mg kg−1 (0.25 mg kg−1 at 12 hours interval) acenocoumarol (Acitrom® Piramal Healthcare). The third groups were designated as the positive control for an increase in aPTT measure value and received a single dose of 500 IU kg−1 (12 hours prior blood sample withdrawal) unfractionated heparin (Declot® Zydus Cadila). The test compounds at a cumulative dose of 25 mg kg−1 (6.25 mg kg−1 at 12 hours interval) suspended in 0.5% CMC was given orally. The blood samples were withdrawn at the end of 12th hour after the administration of last dose of the reference standards and the test compounds. The animals were anesthetized by intravenous injection of 60 mg kg−1 thiopental sodium (Intraval sodium® Piramal Healthcare). The caudal caval vein was exposed by a midline incision and 1.8 mL of blood was collected into a plastic syringe containing 0.2 mL of 100 mM citrate buffer pH 4.5. The blood sample was immediately agitated and centrifuged in a plastic tube at 1500 rpm for 10 min. The plasma so obtained was transferred to another plastic tube and the coagulation tests for the determination of PT and aPTT were performed within three hours. The citrated plasma was coagulated by the addition of coagulating agent and the time for the clot formation was determined in the coagulometer, which highlights the time in s required for the coagulation of the treated and the untreated plasma sample.
3.6. Prothrombin time (PT)
An aliquot of 0.1 mL of citrated plasma was incubated for 1 min at 37 °C. The coagulation process was initiated by the addition of 0.2 mL of human thromboplastin (Thromborel S® Siemens). The coagulometer (CA-600 Blood Coagulometer® Sysmex) was started and the time for the clot formation was determined in s. The increase in PT measure value highlights the effect of the test compounds on the exogenous pathway of coagulation.
3.7. Activated partial thromboplastin time (aPTT)
To 0.1 mL of citrated plasma, 0.1 mL of human placenta (Dade Actin® Siemens) was added and the mixture was incubated for 2 min at 37 °C. The coagulation process is initiated by the addition of 0.1 mL of 25 mM calcium chloride solution. The coagulometer (CA-600 Blood Coagulometer® Sysmex) was started and the time for the clot formation was determined in s. The increase in aPTT measure value highlights the effect of compounds on the endogenous pathway of coagulation.
3.8. Clotting time (CT)
A drop of blood from the treated animal was drawn onto a clean dry glass slide. One end of the capillary tube was dipped into blood drop gently and up to 3/4 of the capillary was allowed to be filled with blood. A small piece of the capillary was broken at regular time intervals until fibrin thread appeared at the broken end of the capillary tube. The clotting time of blood was determined by recording time interval between dipping the blood and the first appearance of fibrin thread at the broken ends of the capillary tube using a stopwatch.
4. Conclusions
The in silico studies indicated that the designed compounds possessed optimal parameters for being an active pharmaceutical ingredient. Molecular docking studies were encouraging and highlighted that the designed compounds were exhibiting a better integration with the target protein and with considerably low crash score. Based on docking results best ranked eleven new benzoxazole encompassing 1,3,4-oxadiazoles (4a–4k) were synthesized, characterized and evaluated for in vitro radical scavenging property and ex vivo anticoagulant activity. The radical savaging efficacy evaluated by DPPH radical scavenging assay method were promising and the compounds exhibited substantial radical scavenging activity. The measure for CT, PT and aPTT values towards anticoagulant activity were encouraging. The compounds exhibited significant increase in CT and PT measure values with respect to the negative control and in comparison to the reference standards employed. The compounds exhibited a minimal increase in aPTT measure values with respect to the negative control and in comparison unfractionated heparin employed as the reference standard. The considerable antithrombotic activity of the 1,3,4-oxadiazoles suggests that these compounds might possibly act as FXa inhibitors, possibly contributing in part to their anticoagulant efficacy.
Acknowledgements
We express our gratitude to the JSS College of Pharmacy, Mysuru and JSS University for providing us all necessary facilities. We sincerely offer our gratitude to Chairman and Staff, NMR research Center, IISc, Bangalore for providing NMR spectra and to the Chairman and staff, USIC, Karnataka University, Dharwad for providing mass spectra.
References
- J. F. Meschia, D. a. Miller and T. G. Brott, Mayo Clin. Proc., 2002, 77, 542–551 CrossRef PubMed.
- C. J. Murray and A. D. Lopez, Lancet, 1997, 349, 1436–1442 CrossRef CAS.
- T. Groth and W. Wagenknecht, Biomaterials, 2001, 22, 2719–2729 CrossRef CAS PubMed.
- D. Cain, S. M. Hutson and R. Wallin, J. Biol. Chem., 1997, 272, 29068–29075 CrossRef CAS PubMed.
- J. Hirsh, J. Dalen, D. R. Anderson, L. Poller, H. Bussey, J. Ansell and D. Deykin, Chest, 2001, 119, 8S–21S CrossRef CAS PubMed.
- P. A. Wolf, R. D. Abbott and W. B. Kannel, Arch. Intern. Med., 1987, 147, 1561–1564 CrossRef CAS PubMed.
- H. Kumar, S. A. Javed, S. A. Khan and M. Amir, Eur. J. Med. Chem., 2008, 43, 2688–2698 CrossRef CAS PubMed.
- G. Giorgioni, B. Accorroni, A. D. Stefano, G. Marucci, A. Siniscalchi and F. Claudi, Med. Chem. Res., 2005, 14, 57–73 CrossRef CAS.
- S. V. Bhandari, K. G. Bothara, M. K. Raut, A. A. Patil, A. P. Sarkate and V. J. Mokale, Bioorg. Med. Chem., 2008, 16, 1822–1831 CrossRef CAS PubMed.
- R. Singh, G. V. Pujar, M. N. Purohit and V. M. Chandrashekar, Med. Chem. Res., 2013, 22, 2163–2173 CrossRef CAS.
- K. M. Amin, N. M. Abdel Gawad, D. E. Abdel Rahman and M. K. M. El Ashry, Bioorg. Chem., 2014, 52, 31–43 CrossRef CAS PubMed.
- W. Li, S. Schulman, R. J. Dutton, D. Boyd, J. Beckwith and T. A. Rapoport, Nature, 2010, 463, 507–512 CrossRef CAS PubMed.
- K. Bethge, H. H. Pertz and K. Rehse, Arch. Pharm., 2005, 338, 78–86 CrossRef CAS PubMed.
- B. Vishwanathan, B. M. Gurupadayya and K. V. Sairam, Bangl. J. Pharmacol., 2016, 11, 67–74 CrossRef.
- Y. H. Zhao, M. H. Abraham, J. Le, A. Hersey, C. N. Luscombe, G. Beck, B. Sherborne and I. Cooper, Pharm. Res., 2002, 19, 1446–1457 CrossRef CAS.
- S. Maignan, J. P. Guilloteau, Y. M. Choi-Sledeski, M. R. Becker, W. R. Ewing, H. W. Pauls, A. P. Spada and V. Mikol, J. Med. Chem., 2003, 46, 685–690 CrossRef CAS PubMed.
- L. Ma, Y. Xiao, C. Li, Z.-L. Xie, D.-D. Li, Y.-T. Wang, H.-T. Ma, H.-L. Zhu, M.-H. Wang and Y.-H. Ye, Bioorg. Med. Chem., 2013, 21, 6763–6770 CrossRef CAS PubMed.
- O. M. Abdelhafez, K. M. Amin, R. Z. Batran, T. J. Maher, S. A. Nada and S. Sethumadhavan, Bioorg. Med. Chem., 2010, 18, 3371–3378 CrossRef CAS PubMed.
- D. Couri and W. D. Wosilait, Biochem. Pharmacol., 1966, 15, 1349–1360 CrossRef CAS.
|
This journal is © The Royal Society of Chemistry 2016 |